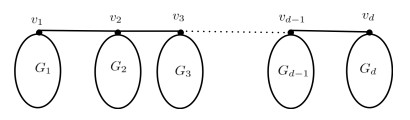
This paper presents a nonlinear robust H-infinity control strategy for improving trajectory following performance of autonomous ground electric vehicles (AGEV) with active front steering system. Since vehicle trajectory dynamics inherently influenced by various driving maneuvers and road conditions, the main objective is to deal with the trajectory following control challenges of parametric uncertainties, system nonlinearities, and external disturbance. The AGEV system dynamics and its uncertain vehicle trajectory following system are first modeled and constructed, in which parameter uncertainties related to the physical limits of tire are considered and handled, then the control-oriented vehicle trajectory following augmented system with dynamic error is developed. The resulting nonlinear robust H-infinity state-feedback controller (NHC) of vehicle trajectory-following system is finally designed by H-infinity performance index and nonlinear compensation under AGEV system requirements, and solved utilizing a set of linear matrix inequalities derived from quadratic H-infinity performance and Lyapunov stability. Simulations for double lane change and serpentine scenes are carried out to verify the effectiveness of the proposed controller with a high-fidelity, CarSim®, full-vehicle model. It is found from the results that the proposed NHC provides improved vehicle trajectory following performance compared with the linear quadratic regulator (LQR) controller and robust H-infinity state-feedback controller (RHC).
Citation: Xianjian Jin, Qikang Wang, Zeyuan Yan, Hang Yang. Nonlinear robust control of trajectory-following for autonomous ground electric vehicles with active front steering system[J]. AIMS Mathematics, 2023, 8(5): 11151-11179. doi: 10.3934/math.2023565
[1] | Ali Al Khabyah . Mathematical aspects and topological properties of two chemical networks. AIMS Mathematics, 2023, 8(2): 4666-4681. doi: 10.3934/math.2023230 |
[2] | Zhibin Du, Ayu Ameliatul Shahilah Ahmad Jamri, Roslan Hasni, Doost Ali Mojdeh . Maximal first Zagreb index of trees with given Roman domination number. AIMS Mathematics, 2022, 7(7): 11801-11812. doi: 10.3934/math.2022658 |
[3] | Zeeshan Saleem Mufti, Ali Tabraiz, Qin Xin, Bander Almutairi, Rukhshanda Anjum . Fuzzy topological analysis of pizza graph. AIMS Mathematics, 2023, 8(6): 12841-12856. doi: 10.3934/math.2023647 |
[4] | Ali N. A. Koam, Ali Ahmad, Azeem Haider, Moin A. Ansari . Computation of eccentric topological indices of zero-divisor graphs based on their edges. AIMS Mathematics, 2022, 7(7): 11509-11518. doi: 10.3934/math.2022641 |
[5] | Muhammad Kamran Jamil, Muhammad Imran, Aisha Javed, Roslan Hasni . On the first general Zagreb eccentricity index. AIMS Mathematics, 2021, 6(1): 532-542. doi: 10.3934/math.2021032 |
[6] | Muhammad Umar Mirza, Rukhshanda Anjum, Maged Z. Youssef, Turki Alsuraiheed . A comprehensive study on fuzzy and crisp graph indices: generalized formulae, proximity and accuracy analysis. AIMS Mathematics, 2023, 8(12): 30922-30939. doi: 10.3934/math.20231582 |
[7] | Edil D. Molina, José M. Rodríguez-García, José M. Sigarreta, Sergio J. Torralbas Fitz . On the Gutman-Milovanović index and chemical applications. AIMS Mathematics, 2025, 10(2): 1998-2020. doi: 10.3934/math.2025094 |
[8] | Zhen Lin . The biharmonic index of connected graphs. AIMS Mathematics, 2022, 7(4): 6050-6065. doi: 10.3934/math.2022337 |
[9] | Chenxu Yang, Meng Ji, Kinkar Chandra Das, Yaping Mao . Extreme graphs on the Sombor indices. AIMS Mathematics, 2022, 7(10): 19126-19146. doi: 10.3934/math.20221050 |
[10] | Milica Anđelić, Tamara Koledin, Zoran Stanić . Notes on Hamiltonian threshold and chain graphs. AIMS Mathematics, 2021, 6(5): 5078-5087. doi: 10.3934/math.2021300 |
This paper presents a nonlinear robust H-infinity control strategy for improving trajectory following performance of autonomous ground electric vehicles (AGEV) with active front steering system. Since vehicle trajectory dynamics inherently influenced by various driving maneuvers and road conditions, the main objective is to deal with the trajectory following control challenges of parametric uncertainties, system nonlinearities, and external disturbance. The AGEV system dynamics and its uncertain vehicle trajectory following system are first modeled and constructed, in which parameter uncertainties related to the physical limits of tire are considered and handled, then the control-oriented vehicle trajectory following augmented system with dynamic error is developed. The resulting nonlinear robust H-infinity state-feedback controller (NHC) of vehicle trajectory-following system is finally designed by H-infinity performance index and nonlinear compensation under AGEV system requirements, and solved utilizing a set of linear matrix inequalities derived from quadratic H-infinity performance and Lyapunov stability. Simulations for double lane change and serpentine scenes are carried out to verify the effectiveness of the proposed controller with a high-fidelity, CarSim®, full-vehicle model. It is found from the results that the proposed NHC provides improved vehicle trajectory following performance compared with the linear quadratic regulator (LQR) controller and robust H-infinity state-feedback controller (RHC).
A molecular graph in chemical graph theory is the graphical representation of the structural formula of a chemical compound in which the vertices represent atoms and edges represent chemical bond between those atoms. A topological index of a molecular graph G is a real number which characterizes the topology of G. Also it is invariant under graph automorphism. Topological indices have been widely used in Quantitative Structure-Activity Relationship (QSAR) and Quantitative Structure-Property Relationship (QSPR) studies. It has application in many folds, to name a few areas, biochemistry, nanotechnology, pharmacology. Bond energy is a measure of bond strength of a chemical compound. The distance between two atoms is considered as the bond length between them. The higher the bond energy, the smaller is the bond length between those atoms. The recently introduced 2-degree based topological invariants, analogous to novel graph invariants (Zagreb indices), namely leap Zagreb indices, may be applied in studying such bond energy between atoms in a molecular graph of a chemical compound.
Throughout this paper, G=(V,E) represents a connected molecular graph with the vertex set V(G) and the edge set E(G). Let the number of vertices and edges of G be n and m respectively. The degree of a vertex v in G is the number of vertices adjacent to v in G and denoted by deg(v:G). The 2-degree (or the second-degree) of a vertex v in G is the number of vertices which are at distance two from v in G and denoted by d2(v:G). The Zagreb indices, namely, the first and second Zagreb indices, are the most important and oldest molecular structure descriptors. These indices have been studied extensively in the field of Mathematical Chemistry [3,4,5]. Recently, the concept of Forgotten topological index also known as F-index have attracted many researchers which results in over 100 research articles related to F-index. A.M.Naji et al. [13] have recently introduced and studied some properties of a new topological invariant called Leap Zagreb indices. They are defined as follows:
Definition 1. (ⅰ) The first leap Zagreb index LM1(G) of a graph G is equal to the sum of squares of the second degrees of the vertices, LM1(G)=∑u∈V(G)d2(u)2.
(ⅱ) The second leap Zagreb index LM2(G) of a graph G is equal to the sum of the products of the second degrees of pairs of adjacent vertices, LM2(G)=∑uv∈E(G)d2(u)d2(v).
(ⅲ) The third leap Zagreb index LM3(G) of a graph G is equal to the sum of the products of the degree with the second degree of every vertex in G, LM3(G)=∑u∈V(G)deg(u)d2(u)
Subsequently, Z. Shao et al. [18] generalized the results of Naji et al.[13] for trees and unicyclic graphs and determined upper and lower bounds on leap Zagreb indices and characterized extremal graphs. Basavanagoud et al.[2] computed exact values for first and second leap hyper Zagreb indices of some nano structures. V. R. Kulli [7,8,9] introduced and studied various leap indices. Shiladhar et al.[17] computed leap Zagreb indices of wind mill graphs. Most recently, Naji et al.[14] have studied some properties of leap graphs.
Azari et al.[1] found formulae for first and second Zagreb indices of bridge and chain graphs. Nilanjan De [15,16] computed F-index and hyper Zagreb index of bridge and chain graphs. Jerline et al. [6] obtained exact values for harmonic index of bridge and chain graphs. E. Litta et al. [10] worked on modified Zagreb indices of bridge graphs. Mohanad Ali et al. [11] computed F-leap index of some special classes of bridge and chain graphs. Zhang et al.[12] worked on Edge-Version Atom-Bond Connectivity and Geometric Arithmetic Indices of generalized bridge molecular graphs. Motivated by their results, we compute exact values for the first and third leap Zagreb indices of bridges and chain graphs. Also we discuss some applications related to these indices in the last section of this paper. First, we recall the definitions of bridge and chain graphs from [1] as follows:
Definition 2. Let {Gi}di=1 be a set of finite pairwise disjoint graphs with distinct vertices vi∈V(Gi). The bridge graph B1=B1(G1,G2,…,Gd;v1,v2,v3,…,vd) of {Gi}di=1 with respect to the vertices {vi}di=1 as shown in Figure 1, is the graph obtained from the graphs G1,G2,…,Gd by connecting the vertices vi and vi+1 by an edge for all i=1,2,…,d−1.
Definition 3. The bridge graph B2=B2(G1,G2,…,Gd;v1,w1,v2,w2,…,vd,wd) of {Gi}di=1 with respect to the vertices {vi,wi}di=1 as shown in Figure 2, is the graph obtained from the graphs G1,G2,G3,…,Gd by connecting the vertices wi and vi+1 by an edge for all i=1,2,…,d−1.
Definition 4. The chain graph C=C(G1,G2,…,Gd;v1,w1,v2,w2,…,vd,wd) of {Gi}di=1 with respect to the vertices {vi,wi}di=1 as shown in Figure 3, is the graph obtained from the graphs G1,G2,…,Gd by identifying the vertices wi and vi+1 for all i=1,2,…,d−1.
The following lemma gives the 2-degree of any arbitrary vertex in the bridge graph B1.
Lemma 5. Let G1,G2,⋯,Gd be d≥5 connected graphs. Then the 2-degree of any arbitrary vertex u in the bridge graph B1 formed by these graphs is as follows:
d2(u:B1)={ν1+μ2+1,ifu=v1νd+μd−1+1,ifu=vdν2+μ1+μ3+1,ifu=v2νd−1+μd+μd−2+1,ifu=vd−1νi+μi−1+μi+1+2,,ifu=vi,3≤i≤d−2d2(u:G1)+1,ifu∈NG1(v1)d2(u:Gd)+1,ifu∈NGd(vd)d2(u:Gi)+2,ifu∈NGi(vi),2≤i≤d−1d2(u:Gi),ifu∈V(Gi)∖NGi[vi],1≤i≤d, | (2.1) |
where νi=d2(vi:Gi) and μi=deg(vi:Gi),1≤i≤d.
Next, we compute the first leap Zagreb index of the type-Ⅰ bridge graph B1.
Let Si=∑u∈NGi(vi)d2(u:Gi), 1≤i≤d.
Theorem 6. LM1(B1)=d∑i=1LM1(Gi)+d−1∑i=2[(μi−1+μi+1+1)2+2νi(μi−1+μi+1+1)+4Si+8μi]+2d−2∑i=3νi+2(S1+Sd)+(μ1+μd−2μ3−2μd−2)+(μ2+1)(μ2+2ν1+1)+(μd−1+1)(μd−1+2νd+1)+3d−12.
Proof. By virtue of Lemma 5
LM1(B1)=∑u∈V(B1)d2(u:B1)2=(ν1+μ2+1)2+(νd+μd−1+1)2+(ν2+μ1+μ3+1)2+(νd−1+μd+μd−2+1)2+d−2∑i=3(νi+μi−1+μi+1+2)2+∑u∈NG1(v1)(d2(u:G1)+1)2+∑u∈NGd(vd)(d2(u:Gd)+1)2+d−1∑i=2∑u∈NGi(vi)(d2(u:Gi)+2)2+d∑i=1∑u∈V(Gi)∖NGi[vi]d2(u:Gi)2 |
=ν21+(μ2+1)2+2ν1(μ2+1)+ν2d+(μd−1+1)2+2νd(μd−1+1)+ν22+(μ1+μ3+1)2+2ν2(μ1+μ3+1)+ν2d−1+(μd+μd−2+1)2+2νd−1(μd+μd−2+1)+d−2∑i=3[(νi+1)2+2(νi+1)(μi−1+μi+1+1)+(μi−1+μi+1+1)2]+∑u∈NG1(v1)[d2(u:G1)2+2d2(u:G1)]+μ1+∑u∈NGd(vd)[d2(u:Gd)2+2d2(u:Gd)]+μd+d−1∑i=2∑u∈NGi(vi)[d2(u:Gi)2+4d2(u:Gi)]+4d−1∑i=2μi+d∑i=1∑u∈V(Gi)∖NGi[vi]d2(u:Gi)2 |
=d∑i=1LM1(Gi)+d−1∑i=2[(μi−1+μi+1+1)2+2νi(μi−1+μi+1+1)+4Si+8μi]+2d−2∑i=3νi+2(S1+Sd)+(μ1+μd−2μ2−2μ3−2μd−2−2μd−1)+(μ2+1)(μ2+2ν1+1)+(μd−1+1)(μd−1+2νd+1)+3d−12. |
Thus the result follows.
Corollary 7. If G1=G2=⋯=Gd=G in a bridge graph B1, then LM1(B1)=dLM1(G)+(4d−6)μ2+(4d−8)ν+(12d−26)μ+(4d−4)(νμ+S)+4d−12, where S=∑u∈NG(v)d2(u:G).
Lemma 8. [1] The degree of an arbitrary vertex u of the bridge graph B1,d≥5 is given by:
deg(u:B1)={μ1+1,ifu=v1μd+1,ifu=vdμi+2,ifu=vi,2≤i≤d−1deg(u:Gi),ifu∈V(Gi)∖{vi},1≤i≤d, | (2.2) |
where μi=deg(vi:Gi),1≤i≤d.
Next, we compute the third leap Zagreb index of the type-Ⅰ bridge graph B1 Let us denote si=∑u∈NGi(vi)deg(u:Gi), 1≤i≤d.
Theorem 9. LM3(B1)=d∑i=1LM3(Gi)+(s1+sd)+2d−1∑i=2si+d∑i=1(2νi+6μi)+2d∑i=2(μi−1μi)−2(μ2+μd−1)−(ν1+νd)−3(μ1+μd)+4d−10.
Proof. By virtue of Lemma 5 and 8
LM3(B1)=∑u∈v(B1)d2(u)deg(u)=(ν1+μ2+1)(μ1+1)+(ν2+μ1+μ3+1)(μ2+2)+(νd+μd−1+1)(μd+1)+(νd−1+μd+μd−2+1)(μd−1+2)+d−2∑i=3(νi+μi−1+μi+1+2)(μi+2)+∑u∈NG1(v1)(d2(u:G1)+1)(deg(u:G1))+∑u∈NGd(vd)(d2(u:Gd)+1)(deg(u:Gd))+d−1∑i=2∑u∈NGi(vi)(d2(u:Gi)+2)(deg(u:Gi))+d∑i=1∑u∈V(Gi)∖NGi[vi](d2(u:Gi))(deg(u:Gi)) |
=(ν1μ1+ν1+μ2μ1+μ2+μ1+1)+(ν2μ2+2ν2+μ1μ2+2μ1+μ3μ2+2μ3+μ2+2)+(νdμd+νd+μd−1μd+μd−1+μd+1)+(νd−1μd−1+2νd−1+μdμd−1+2μd+μd−2μd−1+2μd−2+μd−1+2)+d−2∑i=3(νiμi+2νi+μi−1μi+2μi−1+μi+1μi+2μi+1+2μi+4)+∑u∈NG1(v1)(d2(u:G1)deg(u:G1)+deg(u:G1))+∑u∈NGd(vd)(d2(u:Gd)deg(u:Gd)+deg(u:Gd))+d−1∑i=2∑u∈NGi(vi)(d2(u:Gi)deg(u:Gi)+2deg(u:Gi))+d∑i=1∑u∈V(Gi)∖NGi[vi]d2(u:Gi)deg(u:Gi) |
Thus the result follows.
Corollary 10. If G1=G2=⋯=Gd=G in a bridge graph B1, then LM3(B1)=dLM3(G)+2(d−1)(s+ν+μ2)+2μ(3d−5)+4d−10, where s=∑u∈NG(v)deg(u:G).
For any two nonempty sets A and B, AΔB denotes the symmetric difference of A and B and defined as AΔB=(A∖B)∪(B∖A)=(A∪B)∖(A∩B). First, we obtain the 2-degree of any arbitrary vertex in the type-Ⅱ bridge graph B2 as follows:
Lemma 11. Let G1,G2,⋯,Gd be d≥5 triangle free connected graphs. Then 2-degree of any arbitrary vertex u in the bridge graph B2 formed by these graphs is as follows:
d2(u:B2)={d2(u:G1),ifu∈V(G1)∖NG1[w1]d2(u:G1)+1,ifu∈NG1(w1)d2(u:Gi),ifu∈V(Gi)∖{NGi[vi]∪NGi[wi]},2≤i≤d−1d2(u:Gd),ifu∈V(Gd)∖NGd[vd]d2(u:Gd)+1,ifu∈NGd(vd)d2(u:Gi)+1,ifu∈(NGi(vi)ΔNGi(wi)),2≤i≤d−1d2(u:Gi)+2,ifu∈NGi(vi)∩NGi(wi),2≤i≤d−1δi+μi+1,ifu=wi,1≤i≤d−1νi+λi−1,ifu=vi,2≤i≤d. | (2.3) |
where νi=d2(vi:Gi),μi=deg(vi:Gi);2≤i≤d,δi=d2(wi:Gi),λi=deg(wi:Gi);1≤i≤d−1.
Next, we compute the first leap Zagreb index of type-Ⅱ bridge graph B2.
Let us denote S′1=∑u∈NG1(w1)d2(u:G1) and Sd=∑u∈NGd(vd)d2(u:Gd)
Theorem 12. LM1(B2)=d∑i=1LM1(Gi)+2(S′1+Sd)+(λ1+μd)+d−1∑i=2∑u∈NGi(vi)ΔNGi(wi)[2d2(u:Gi)+1]+4d−1∑i=2∑u∈NGi(vi)∩NGi(wi)[d2(u:Gi)+1]+d−1∑i=1(μ2i+1+2δiμi+1)+d∑i=2(λ2i−1+2νiλi−1).
Proof.
LM1(B2)=∑u∈V(B2)d2(u:B2)2=∑u∈V(G1)∖NG1[w1]d2(u:G1)2+d−1∑i=2 ∑u∈V(Gi)∖{NGi[vi]∪NGi[wi]}d2(u:Gi)2+∑u∈V(Gd)∖NGd[vd]d2(u:Gd)2+∑u∈NG1(w1)(d2(u:G1)+1)2+d−1∑i=2∑u∈NGi(vi)ΔNGi(wi)(d2(u:Gi)+1)2+∑u∈NGd(vd)(d2(u:Gd)+1)2+d−1∑i=2∑u∈NGi(vi)∩NGi(wi)(d2(u:Gi)+2)2+d−1∑i=1(δi+μi+1)2+d∑i=2(νi+λi−1)2 |
=LM1(G1)−δ21−∑u∈NG1(w1)d2(u:G1)2+d−1∑i=2[∑u∈V(Gi)d2(u:Gi)2−∑u∈N(vi)∪N(wi)d2(u:Gi)2−ν2i−δ2i]+LM1(Gd)−ν2d−∑u∈NGd(vd)d2(u:Gd)2+∑u∈NG1(w1)d2(u:G1)2+2∑u∈NG1(w1)d2(u:G1)+λ1+d−1∑i=2[∑u∈NGi(vi)ΔNGi(wi)[d2(u:Gi)2+2d2(u:Gi)+1]]+∑u∈NGd(vd)[d2(u:Gd)2+2d2(u:Gd)+1]+d−1∑i=2[∑u∈NGi(vi)∩NGi(wi)[d2(u:Gi)2+4d2(u:Gi)+4]]+d−1∑i=1[δ2i+2δiμi+1+μ2i+1]+d∑i=2[ν2i+2νiλi−1+λ2i−1] |
Thus,
LM1(B2)=d∑i=1LM1(Gi)+2(S′1+Sd)+(λ1+μd)+d−1∑i=2∑u∈NGi(vi)ΔNGi(wi)[2d2(u:Gi)+1]+4d−1∑i=2∑u∈NGi(vi)∩NGi(wi)[d2(u:Gi)+1]+d−1∑i=1(μ2i+1+2δiμi+1)+d∑i=2(λ2i−1+2νiλi−1). |
Corollary 13. If G1=G2=⋯,Gd=G, in a bridge graph B2, then LM1(B2)=dLM1(G)+λ+μ+2(S+S′)+(d−2)∑u∈NG(v)ΔNG(w)(2d2(u:G)+1)+4(d−2)∑u∈NG(v)∩NG(w)(d2(u:G)+1)+(d−1)[μ2+λ2]+2(d−1)[δμ+νλ], where S=∑u∈NG(w)d2(u:G) and S′=∑u∈NG(v)d2(u:G).
In what follows next, we compute the third leap Zagreb index of B2.
Lemma 14. The degree of an arbitrary vertex u of the bridge graph B2, d≥5 is given by:
deg(u:B2)={deg(u:G1),ifu∈V(G1)∖{w1}deg(u:Gd),ifu∈V(Gd)∖{vd}deg(u:Gi),ifu∈V(Gi)∖{vi,wi},2≤i≤d−1λi+1,ifu=wi,1≤i≤d−1μi+1,ifu=vi,2≤i≤d. | (2.4) |
where μi=deg(vi:Gi);2≤i≤d,λi=deg(wi:Gi);1≤i≤d−1.
Theorem 15. LM3(B2)=d∑i=1LM3(Gi)+∑u∈NG1(w1)deg(u:G1)+∑u∈NGd(vd)deg(u:Gd)+d−1∑i=2∑u∈NGi(wi)∖NGi(vi)deg(u:Gi)+d−1∑i=2∑u∈NGi(vi)∖NGi(wi)deg(u:Gi)+d−1∑i=2∑u∈NGi(vi)∩NGi(wi)2deg(u:Gi)+d−1∑i=12μi+1λi+d−1∑i=1μi+1+d∑i=2λi−1+d∑i=1(δi+νi)−ν1−δd.
Proof. By virtue of Lemma 2.7 and 2.10
LM3(B2)=∑u∈V(B2)d2(u)deg(u)=∑u∈V(G1)∖NG1[w1]d2(u:G1)deg(u:G1)+d−1∑i=2∑u∈V(Gi)∖{NGi[vi]∪NGi[wi]}d2(u:Gi)deg(u:Gi)+∑u∈V(Gd)∖NGd[vd]d2(u:Gd)deg(u:Gd)+∑u∈NG1(w1)(d2(u:G1)+1)(deg(u:G1))+d−1∑i=2∑u∈NGi(wi)∖NGi(vi)(d2(u:Gi)+1)(deg(u:Gi))+d−1∑i=2∑u∈NGi(vi)∖NGi(wi)(d2(u:Gi)+1)(deg(u:Gi))+∑u∈NGd(vd)(d2(u:Gd)+1)(deg(u:Gd))+d−1∑i=2∑u∈NGi(vi)∩NGi(wi)(d2(u:Gi)+2)(deg(u:Gi))+d−1∑i=1(δi+μi+1)(λi+1)+d∑i=2(νi+λi−1)(μi+1) |
Thus the result follows.
Corollary 16. If G1=G2=⋯=Gd=G in a bridge graph B2, then LM3(B2)=dLM3(G)+∑u∈NG(w)deg(u:G)+∑u∈NG(v)deg(u:G)+(d−2)(∑u∈NG(w)∖NG(v)deg(u:G)+∑u∈NG(v)∖NG(w)deg(u:G)+∑u∈NG(v)∩NG(w)2deg(u:G))+(d−1)(2μλ+μ+λ)+d(δ+ν)−(ν+δ).
In the following lemma, we obtain the 2-degree of any vertex in the chain graph C.
Lemma 17. Let G1,G2,⋯,Gd, d≥5 be C3-free connected graphs and let C=C(G1,G2,⋯,Gd;w1,v2,w2,v3,⋯,wd−1,vd) be the chain graph formed using these graphs. Then the 2-degree of any vertex u in C is given as follows:
d2(u:C)={d2(u:G1),ifu∈V(G1)∖NG1[w1]d2(u:G1)+μ2,ifu∈NG1(w1)d2(u:Gd),ifu∈V(Gd)∖NGd[vd]d2(u:Gd)+λd−1,ifu∈NGd(vd)d2(u:Gi),ifu∈V(Gi)∖{NGi[wi]∪NGi[vi]},2≤i≤d−1d2(u:Gi)+μi+1,ifu∈NGi(wi)∖NGi(vi),2≤i≤d−1d2(u:Gi)+λi−1,ifu∈NGi(vi)∖NGi(wi),2≤i≤d−1d2(u:Gi)+λi−1+μi+1,ifu∈NGi(vi)∩NGi(wi),2≤i≤d−1δi+νi+1,ifu=wi=vi+1,1≤i≤d−1, | (2.5) |
where νi=d2(vi:Gi),μi=deg(vi:Gi),λi=deg(wi:Gi) and δi=d2(wi:Gi) for all 1≤i≤d.
Now, we compute the first leap Zagreb index of the chain graph C by applying Lemma 17.
Theorem 18. For the chain graph C,
LM1(C)=d∑i=1LM1(Gi)+∑u∈NG1(w1)[2μ2d2(u:G1)+μ22]+∑u∈NGd(vd)[2λd−1d2(u:Gd)+λ2d−1]+d−1∑i=2 ∑u∈NGi(wi)∖NGi(vi)[2μi+1d2(u:Gi)+μ2i+1]+d−1∑i=2 ∑u∈NGi(vi)∖NGi(wi)[2λi−1d2(u:Gi)+λ2i−1]+2d−1∑i=2 ∑u∈NGi(vi)∩NGi(wi)[λi−1d2(u:Gi)+μi+1d2(u:Gi)+λi−1μi+1]+d−1∑i=2 ∑u∈NGi(vi)∩NGi(wi)(λ2i−1+μ2i+1)+2d−1∑i=1δiνi+1. |
Proof. By Lemma 17, we have
LM1(C)=∑u∈V(C)d2(u:C)2=∑u∈V(G1)∖NG1[w1]d2(u:G1)2+∑u∈NG1(w1)[d2(u:G1)+μ2]2+∑u∈V(Gd)∖NGd[vd]d2(u:Gd)2+∑u∈NGd(vd)[d2(u:Gd)+λd−1]2+d−1∑i=2 ∑u∈V(Gi)∖{NGi[vi]∪NGi[wi]}d2(u:Gi)2+d−1∑i=2 ∑u∈NGi(wi)∖NGi(vi)[d2(u:Gi)+μi+1]2+d−1∑i=2 ∑u∈NGi(vi)∖NGi(wi)[d2(u:Gi)+λi−1]2+d−1∑i=2 ∑u∈NGi(vi)∩NGi(wi)[d2(u:Gi)+λi−1+μi+1]2+d−1∑i=1[δi+νi+1]2 |
=LM1(G1)−∑u∈NG1(w1)[d2(u:G1)2]−δ21+∑u∈NG1(w1)[d2(u:G1)2+2d2(u:G1)μ2+μ22]+LM1(Gd)−∑u∈NGd(vd)d2(u:Gd)2−ν2d+∑u∈NGd(vd)[d2(u:Gd)2+2λd−1d2(u:Gd)+λ2d−1]+d−1∑i=2∑u∈V(Gi)d2(u:Gi)2−d−1∑i=2 ∑u∈NGi[vi]∪NGi[wi]d2(u:Gi)2+d−1∑i=2 ∑u∈NGi(wi)∖NGi(vi)[d2(u:Gi)2+2μi+1d2(u:Gi)+μ2i+1]+d−1∑i=2 ∑u∈NGi(vi)∖NGi(wi)[d2(u:Gi)2+2λi−1d2(u:Gi)+λ2i−1]+d−1∑i=2∑u∈NGi(vi)∩NGi(wi)[d2(u:Gi)2+2λi−1d2(u:Gi)+2μi+1d2(u:Gi)+2λi−1μi+1+λ2i−1+μ2i+1]+d−1∑i=1[δ2i+ν2i+1]+2d−1∑i=1δiνi+1 |
=d∑i=1LM1(Gi)+∑u∈NG1(w1)[2μ2d2(u:G1)+μ22]+∑u∈NGd(vd)[2λd−1d2(u:Gd)+λ2d−1]+d−1∑i=2 ∑u∈NGi(wi)∖NGi(vi)[2μi+1d2(u:Gi)+μ2i+1]+d−1∑i=2 ∑u∈NGi(vi)∖NGi(wi)[2λi−1d2(u:Gi)+λ2i−1]+2d−1∑i=2 ∑u∈NGi(vi)∩NGi(wi)[λi−1d2(u:Gi)+μi+1d2(u:Gi)+λi−1μi+1]+d−1∑i=2 ∑u∈NGi(vi)∩NGi(wi)(λ2i−1+μ2i+1)+2d−1∑i=1δiνi+1. |
Corollary 19. In a chain graph C, if G1=G2=⋯=Gd=G, then LM1(C)=dLM1(G)+∑u∈NG(w)[2μd2(u:G)+μ2]+∑u∈NG(v)[2λd2(u:G)+λ2]+(d−2)∑u∈NG(w)∖NG(v)[2μd2(u:G)+μ2]+(d−2)∑u∈NG(v)∖NG(w)[2λd2(u:G)+λ2]+2(d−2)∑u∈NG(v)∩NG(w)[λd2(u:G)+μd2(u:G)+λμ]+(d−2)∑u∈NG(v)∩NG(w)(λ2+μ2)+2(d−1)δν.
Lemma 20. Let G1,G2,⋯,Gd, d≥5 be C3-free connected graphs and let C=C(G1,G2,⋯,Gd;w1,v2,w2,v3,⋯,wd−1,vd) be the chain graph formed using these graphs. Then the degree of any vertex u in C is given as follows:
deg(u:C)={deg(u:G1),ifu∈V(G1)∖{w1}deg(u:Gd),ifu∈V(Gd)∖{vd}deg(u:Gi),ifu∈V(Gi)∖{vi,wi},2≤i≤d−1λi+μi+1,ifu=wi=vi+1,1≤i≤d−1, | (2.6) |
where μi=deg(vi:Gi),λi=deg(wi:Gi) for all 1≤i≤d
Finally, we compute the third leap Zagreb index of the chain graph C by applying Lemma 17 and 2.16.
Theorem 21. LM3(C)=d∑i=1LM3(Gi)+∑u∈NG1(w1)μ2deg(u:G1)+∑u∈NGd(vd)λd−1deg(u:Gd)+d−1∑i=2∑u∈NGi(wi)∖NGi(vi)μi+1deg(u:Gi)+d−1∑i=2∑u∈NGi(vi)∖NGi(wi)λi−1deg(u:Gi)+d−1∑i=2∑u∈NGi(vi)∩NGi(wi)(λi−1deg(u:Gi)+μi+1deg(u:Gi))+d−1∑i=1(δiμi+1+νi+1λi).
Proof. By virtue of Lemma 17 and 20
LM3(C)=∑u∈V(C)d2(u)deg(u)=∑u∈V(G1)∖NG1[w1]d2(u:G1)deg(u:G1)+∑u∈NG1(w1)(d2(u:G1)+μ2)deg(u:G1)+∑u∈V(Gd)∖NGd[vd]d2(u:Gd)deg(u:Gd)+∑u∈NGd(vd)(d2(u:Gd)+λd−1)deg(u:Gd)+d−1∑i=2∑u∈V(Gi)∖{NGi[wi]∪NGi[vi]}d2(u:Gi)deg(u:Gi)+d−1∑i=2∑u∈NGi(wi)∖NGi(vi)(d2(u:Gi)+μi+1)deg(u:Gi)+d−1∑i=2∑u∈NGi(vi)∖NGi(wi)(d2(u:Gi)+λi−1)deg(u:Gi)+d−1∑i=2∑u∈NGi(vi)∩NGi(wi)(d2(u:Gi)+λi−1+μi+1)deg(u:Gi)+d−1∑i=1(δi+νi+1)(λi+μi+1). |
Thus the result follows.
Corollary 22. In a chain graph C, if G1=G2=⋯=Gd=G, then LM3(C)=dLM3(G)+∑u∈NG(w)μdeg(u:G)+∑u∈NG(v)λdeg(u:G)+(d−2)(∑u∈NG(w)∖NG(v)μdeg(u:G)+∑u∈NG(v)∖NG(w)λdeg(u:G)+∑u∈NG(v)∩NG(w)(λ+μ)deg(u:G))+(d−1)(δμ+νλ).
In this section, we determine the first and third leap Zagreb indices of some molecular graph structures. Two vertices v and w of a hexagon H (C6) (please refer Figure 4) are said to be in
(ⅰ) ortho-position, if they are adjacent in H
(ⅱ) meta-position, if they are distance two in H
(ⅲ) para-position, if they are distance three in H.
We connect h≥5 ortho-hexagons to form a polyphenyl chain denoted by Oh as follows:
One can observe that the Polyphenyl chain Oh shown in Figure 5 is a B1 type bridge graph. Therefore, from Corollary 7, we get
LM1(Oh)=hLM1(G)+(4h−6)μ2+(4h−8)ν+(12h−26)μ+(4h−4)[νμ+∑u∈NG(v)d2(u:G)]+4h−12=24h+(4h−6)(4)+(4h−8)(2)+(12h−26)(2)+(4h−4)(4)+(4h−4)(4)+4h−12=108h−136. |
Similarly,
From Corollary 10, we get
LM3(Oh)=24h+(2h−2)(2)+(2h−2)(2)+2(2)(3h−5)+2(h−1)(2+4)+4h−10=60h−50 |
The polyphenyl chain Mh is formed by connecting h≥5 meta-hexagons as shown in Figure 6.
The polyphenyl chain Ph is formed by connecting h≥5 para-hexagons as shown in the following Figure 7.
It is clear that the Polyphenyl chains Mh and Ph are type-Ⅱ bridge graphs B2.
Using Corollary 2.9, we get
LM1(Mh)=hLM1(G)+λ+μ+2∑u∈NG(w)d2(u:G)+(h−2)[∑u∈NG(w)∖NG(v)(2d2(u:G)+1)]+(h−2)∑u∈NG(v)∖NG(w)(2d2(u:G)+1)+4(h−2)∑u∈NG(v)∩NG(w)(d2(u:G)+1)+2∑u∈NG(v)d2(u:G)+(h−1)μ2+2(h−1)δμ+2(h−1)νλ+(h−1)λ2=24h+4+2(4)+(h−2)[2(2)+1]+(h−2)[2(2)+1]+4(h−2)(2+1)+2(4)+(h−1)(4)+4(h−1)(4)+(h−1)(4) |
Thus LM1(Mh)=70h−48.
Similarly, by Corollary 13, we have
LM1(Ph)=24h+4+2(4)+(h−2)[2(4)+2]+(h−2)(8+2)+4(h−2)(0)+2(4)+(h−1)(4)+8(h−1)+8(h−1)+(h−1)(4) |
Therefore, LM1(Ph)=68h−44.
Using Corollary 2.12, we get
LM3(Mh)=24h+8+(h−2)8+(h−1)12+h(4)−4=48h−24 |
LM3(Ph)=24h+8+(h−2)8+(h−1)12+4h−4=48h−24. |
Next, we shall see an application related to the chain graph C. The spiro-chain SPC4(d,3) is a chain graph formed using d≥5 copies of the cycle C4.
Here the number 3 in the construction denotes the position of the vertices v and w in the spiro-chain (refer Figure 8).
The spiro-chain SPC6(d,4) is a chain graph formed using d≥5 copies of the cycle C6 or hexagon where the vertices v and w are connected in the 4th position (refer Figure 9).
By applying Corollary 19, we get
LM1(SPC4(d,3))=dLM1(G)+∑u∈NG(w)[2μd2(u:G)+μ2]+∑u∈NG(v)[2λd2(u:G)+λ2]+(d−2)∑u∈NG(w)∖NG(v)[2μd2(u:G)+μ2]+(d−2)∑u∈NG(v)∖NG(w)[2λd2(u:G)+λ2]+2(d−2)∑u∈NG(v)∩NG(w)[λd2(u:G)+μd2(u:G)+λμ]+(d−2)∑u∈NG(v)∩NG(w)(λ2+μ2)+2(d−1)δν=54d−66. |
Similarly, from Corollary 19, we have LM1(SPC6(d,4))=80d−56.
By applying Corollary 22, we get
LM3(SPC4(d,3))=8d+2(2+2)+2(2+2)+(d−2)(16)+(d−1)(4)=28d−20 |
Similarly, from Corollary 22, we have LM3(SPC6(d,4))=48d−24.
We have computed exact values of one of the recent topological invariants namely first and third leap Zagreb indices for bridge and chain graphs. It is worth mentioning that computing second leap Zagreb index of bridges and chain graphs has not yet addressed and interested researchers may work on it. Also these indices need to be explored for several other interesting graph structures arising from mathematical chemistry and other branches of science.
The authors wish to thank the referees for their careful reading of the manuscript and valuable suggestions. This work was supported in part by the National Key Technologies R & D Program of China under Grant 2017YFB0802300, 2018YFB0904205, in part by the Key Laboratory of Pattern Recognition and Intelligent Information Processing, Institutions of Higher Education of Sichuan Province under Grant MSSB-2020-12.
The authors declare that no competing interests exist.
[1] |
G. Demesure, M. Defoort, A. Bekrar, D. Trentesaux, M. Djemaï , Decentralized motion planning and scheduling of AGVs in an FMS, IEEE Trans. Ind. Informat., 14 (2018), 1744–1752. https://doi.org/10.1109/TII.2017.2749520 doi: 10.1109/TII.2017.2749520
![]() |
[2] |
S. Riazi, K. Bengtsson, B. Lennartson, Energy optimization of large-scale AGV systems, IEEE Trans. Autom. Sci. Eng., 18 (2021), 638–649. https://doi.org/10.1109/TASE.2019.2963285 doi: 10.1109/TASE.2019.2963285
![]() |
[3] |
V. Digani, L. Sabattini, C. Secchi, C. Fantuzzi, Ensemble coordination approach in multi-AGV systems applied to industrial warehouses, IEEE Trans. Autom. Sci. Eng., 12 (2015), 922–934. https://doi.org/10.1109/TASE.2015.2446614 doi: 10.1109/TASE.2015.2446614
![]() |
[4] |
T. Wang, Y. Xu, S. Ahipasaoglu, C. Courcoubetis, Ex-post max-min fairness of generalized AGV mechanisms, IEEE Trans. Autom. Control, 62 (2017), 5275–5281. https://doi.org/10.1109/TAC.2016.2632424 doi: 10.1109/TAC.2016.2632424
![]() |
[5] |
S. Hoshino, J. Ota, A. Shinozaki, H. Hashimoto, Hybrid design methodology and cost-effectiveness evaluation of AGV transportation systems, IEEE Trans. Autom. Sci. Eng., 4 (2007), 360–372. https://doi.org/10.1109/TASE.2006.887162 doi: 10.1109/TASE.2006.887162
![]() |
[6] |
S. Lu, C. Xu, R. Zhong, An active RFID tag-enabled locating approach with multipath effect elimination in AGV, IEEE Trans. Autom. Sci. Eng., 13 (2016), 1333–1342. https://doi.org/10.1109/TASE.2016.2573595 doi: 10.1109/TASE.2016.2573595
![]() |
[7] |
H. Gao, J. Zhu, X. Li, Y. Kang, J. Li, H. Su, Automatic parking control of unmanned vehicle based on switching control algorithm and backstepping, IEEE /ASME Trans. Mech., 27 (2022), 1233–1243. https://doi.org/10.1109/TMECH.2020.3037215 doi: 10.1109/TMECH.2020.3037215
![]() |
[8] |
M. Graf Plessen, D. Bernardini, H. Esen, A. Bemporad, Spatial-based predictive control and geometric corridor planning for adaptive cruise control coupled with obstacle avoidance, IEEE Trans. Control. Syst. Technol., 26 (2018), 38–50. https://doi.org/10.1109/TCST.2017.2664722 doi: 10.1109/TCST.2017.2664722
![]() |
[9] |
R. Utriainen, M. Pöllänen, H. Liimatainen, The safety potential of lane keeping assistance and possible actions to improve the potential, IEEE Trans. Intell. Veh., 5 (2020), 556–564. https://doi.org/10.1109/TIV.2020.2991962 doi: 10.1109/TIV.2020.2991962
![]() |
[10] |
W. Li, Q. Li, S. Li, R. Li, Y. Ren, W. Wang, Indirect shared control through non-zero sum differential game for cooperative automated driving, IEEE Trans. Intell. Transp. Syst., 23 (2022), 15980–15992. https://doi.org/10.1109/TITS.2022.3146895 doi: 10.1109/TITS.2022.3146895
![]() |
[11] |
X. Jin, J. Wang, X. He, Z. Yan, L. Xu, C. Wei, et al., Improving vibration performance of electric vehicles based on in-wheel motor-active suspension system via robust finite frequency control, IEEE Trans. Intell. Transp. Syst., 24 (2023), 1631–1643. https://doi.org/10.1109/TITS.2022.3224609 doi: 10.1109/TITS.2022.3224609
![]() |
[12] |
X. Jin, J. Wang, Z. Yan, L. Xu, G. Yin, N. Chen, Robust vibration control for active suspension system of in-wheel-motor-driven electric vehicle via μ-synthesis methodology, ASME J. Dyn. Sys. Meas. Control, 144 (2022), 051007. https://doi.org/10.1115/1.4053661 doi: 10.1115/1.4053661
![]() |
[13] |
X. Jin, Q. Wang, Z. Yan, H. Yang, J. Wang, G. Yin, A learning-based evaluation for lane departure warning system considering driving characteristics, Proc. Inst. Mech. Eng. D-J. Aut., 2022. https://doi.org/10.1177/09544070221140973 doi: 10.1177/09544070221140973
![]() |
[14] |
K. Nam, H. Fujimoto, Y. Hori, Advanced motion control of electric vehicles based on robust lateral tire force control via active front steering, IEEE/ASME Trans. Mech., 19 (2014), 289–299. https://doi.org/10.1109/TMECH.2012.2233210 doi: 10.1109/TMECH.2012.2233210
![]() |
[15] |
H. Wang, Y. Tian, H. Xu, Neural adaptive command filtered control for cooperative path following of multiple underactuated autonomous underwater vehicles along one path, IEEE Trans. Syst, Man, Cybern. Syst., 52 (2022), 2966–2978. https://doi.org/10.1109/TSMC.2021.3062077 doi: 10.1109/TSMC.2021.3062077
![]() |
[16] |
Y. Wang, B. Nguyen, H. Fujimoto, Y. Hori, Multirate estimation and control of body slip angle for electric vehicles based on onboard vision system, IEEE Trans. Ind. Electron., 61 (2014), 1133–1143. https://doi.org//10.1109/TIE.2013.2271596 doi: 10.1109/TIE.2013.2271596
![]() |
[17] |
G. Wang, Y. Liu, S. Li, Y. Tian, N. Zhang, G. Cui, New integrated vehicle stability control of active front steering and electronic stability control considering tire force reserve capability, IEEE Trans. Veh. Technol., 70 (2021), 2181–2195. https://doi.org//10.1109/TVT.2021.3056560 doi: 10.1109/TVT.2021.3056560
![]() |
[18] |
J. Cho, K. Huh, Active front steering for driver's steering comfort and vehicle driving stability, Int. J. Automt. Technol., 20 (2019), 589–596. https://doi.org/10.1007/s12239-019-0056-1 doi: 10.1007/s12239-019-0056-1
![]() |
[19] |
P. Falcone, F. Borrelli, J. Asgari, H. Tseng, D. Hrovat, Predictive active steering control for autonomous vehicle systems. IEEE Trans. Control Syst. Technol., 15 (2007), 566–580. https://doi.org/10.1109/TCST.2007.894653 doi: 10.1109/TCST.2007.894653
![]() |
[20] |
T. Faulwasser, R. Findeisen, Nonlinear model predictive control for constrained output path following, IEEE Trans. Autom. Control, 61 (2016), 1026–1039. https://doi.org/10.1109/TAC.2015.2466911 doi: 10.1109/TAC.2015.2466911
![]() |
[21] |
P. Liljeback, I. Haugstuen, K. Pettersen, Path following control of planar snake robots using a cascaded approach, IEEE Trans. Control Syst. Technol., 20 (2012), 111–126. https://doi.org/10.1109/TCST.2011.2107516 doi: 10.1109/TCST.2011.2107516
![]() |
[22] |
A. Hladio, C. Nielsen, D. Wang, Path following for a class of mechanical systems, IEEE Trans. Control Syst. Technol., 21 (2013), 2380–2390. https://doi.org/10.1109/TCST.2012.2223470 doi: 10.1109/TCST.2012.2223470
![]() |
[23] |
S. Mobayen, Robust tracking controller for multivariable delayed systems with input saturation via composite nonlinear feedback, Nonlinear. Dyn., 76 (2014), 827–838. https://doi.org/10.1007/s11071-013-1172-5 doi: 10.1007/s11071-013-1172-5
![]() |
[24] |
V. Ghaffari, Optimal tuning of composite nonlinear feedback control in time-delay nonlinear systems, J. Franklin Inst., 357 (2020), 1331–1356. https://doi.org/10.1016/j.jfranklin.2019.12.024 doi: 10.1016/j.jfranklin.2019.12.024
![]() |
[25] |
S. Yu, X. Li, H. Chen, F. Allgöwer, Nonlinear model predictive control for path following problems, Int. J. Robust Nonlinear Control, 25 (2015), 1168–1182. https://doi.org/10.1002/rnc.3133 doi: 10.1002/rnc.3133
![]() |
[26] |
J. Chen, Z. Shuai, H. Zhang, W. Zhao, Path following control of autonomous four-wheel-independent-drive electric vehicles via second-order sliding mode and nonlinear disturbance observer techniques, IEEE Trans. Ind. Electron., 68 (2021), 2460–2469. https://doi.org/10.1109/TIE.2020.2973879 doi: 10.1109/TIE.2020.2973879
![]() |
[27] |
Z. Liu, X. Chen, Adaptive sliding mode security control for stochastic Markov jump cyber-physical nonlinear systems subject to actuator failures and randomly occurring injection attacks, IEEE Trans. Ind. Informat., 2022. https://doi.org/10.1109/TII.2022.3181274 doi: 10.1109/TII.2022.3181274
![]() |
[28] |
X. Zhao, Z. Liu, B. Jiang, C. Gao, Switched controller design for robotic manipulator via neural network-based sliding mode approach, IEEE Trans. Circuits Syst. II: Exp. Briefs, 70 (2022), 561–565. https://doi.org/10.1109/TCSII.2022.3169475 doi: 10.1109/TCSII.2022.3169475
![]() |
[29] |
B. Xu, F. Sun, Y. Pan, B. Chen, Disturbance observer based composite learning fuzzy control of nonlinear systems with unknown dead zone, IEEE Trans. Syst. Man. Cybern. Syst., 47 (2017), 1854–1862. https://doi.org/10.1109/TSMC.2016.2562502 doi: 10.1109/TSMC.2016.2562502
![]() |
[30] |
H. A. Hagras, A hierarchical type-2 fuzzy logic control architecture for autonomous mobile robots, IEEE Trans. Fuzzy. Syst., 12 (2004), 524–539. https://doi.org/10.1109/TFUZZ.2004.832538 doi: 10.1109/TFUZZ.2004.832538
![]() |
[31] |
J. Cervantes, W. Yu, S. Salazar, I. Chairez, Takagi-Sugeno dynamic neuro-fuzzy controller of uncertain nonlinear systems, IEEE Trans. Fuzzy. Syst., 25 (2017), 1601–1615. https://doi.org/10.1109/TFUZZ.2016.2612697 doi: 10.1109/TFUZZ.2016.2612697
![]() |
[32] |
Y. Wu, L. Wang, J. Zhang, F. Li, Path following control of autonomous ground vehicle based on nonsingular terminal sliding mode and active disturbance rejection control, IEEE Trans. Veh. Technol., 68 (2019), 6379–6390. https://doi.org/10.1109/TVT.2019.2916982 doi: 10.1109/TVT.2019.2916982
![]() |
[33] | M. Islam, Y. He, An optimal preview controller for active trailer steering systems of articulated heavy vehicles, SAE Tech. Pap., 2011, 1–14. https://doi.org/10.4271/2011-01-0983 |
[34] |
T. Ding, Y. Zhang, G. Ma, Z. Cao, X. Zhao, B. Tao, Trajectory tracking of redundantly actuated mobile robot by MPC velocity control under steering strategy constraint, Mechatronics, 84 (2022), 102779. https://doi.org/10.1016/j.mechatronics.2022.102779 doi: 10.1016/j.mechatronics.2022.102779
![]() |
[35] |
H. Moradi, G. Vossoughi, M. R. Movahhedy, H. Salarieh, Suppression of nonlinear regenerative chatter in milling process via robust optimal control, J. Process Control, 23 (2013), 631–648. https://doi.org/10.1016/j.jprocont.2013.02.006 doi: 10.1016/j.jprocont.2013.02.006
![]() |
[36] |
Y. Fu, B. Li, J. Fu, Multi-model adaptive switching control of a nonlinear system and its applications in a smelting process of fused magnesia, J. Process Control, 115 (2022), 67–76. https://doi.org/10.1016/j.jprocont.2022.04.009 doi: 10.1016/j.jprocont.2022.04.009
![]() |
[37] |
S. Fahmy, S. Banks, Robust H∞ control of uncertain nonlinear dynamical systems via linear time-varying approximations, Nonlinear Anal., 63 (2005), 2315–2327. https://doi.org/10.1016/j.na.2005.03.030 doi: 10.1016/j.na.2005.03.030
![]() |
[38] |
G. Ju, Y. Wu, W. Sun, Adaptive output feedback asymptotic stabilization of nonholonomic systems with uncertainties, Nonlinear Anal., 71 (2009), 5106–5117. https://doi.org/10.1016/j.na.2009.03.088 doi: 10.1016/j.na.2009.03.088
![]() |
[39] |
E. Jafari, T. Binazadeh, Observer-based improved composite nonlinear feedback control for output tracking of time-varying references in descriptor systems with actuator saturation, ISA Trans., 91 (2019), 1–10. https://doi.org/10.1016/j.isatra.2019.01.035 doi: 10.1016/j.isatra.2019.01.035
![]() |
[40] |
S. Mobayen, An LMI-based robust tracker for uncertain linear systems with multiple time-varying delays using optimal composite nonlinear feedback technique, Nonlinear Dyn., 80 (2015), 917–927. https://doi.org/10.1007/s11071-015-1916-5 doi: 10.1007/s11071-015-1916-5
![]() |
[41] |
V. Ghaffari, S. Mobayen, D. Ud, T. Rojsiraphisal, M. T. Vu, Robust tracking composite nonlinear feedback controller design for time-delay uncertain systems in the presence of input saturation, ISA Trans., 129 (2022), 88–99. https://doi.org/10.1016/j.isatra.2022.02.029 doi: 10.1016/j.isatra.2022.02.029
![]() |
[42] |
X. Jin, Z. Yan, G. Yin, S. Li, C. Wei, An adaptive motion planning technique for on-road autonomous driving, IEEE Access, 9(2020), 2655–2664. https://doi.org/10.1109/ACCESS.2020.3047385 doi: 10.1109/ACCESS.2020.3047385
![]() |
[43] |
B. Paden, M. Čáp, S. Yong, D. Yershov, E. Frazzoli, A survey of motion planning and control techniques for self-driving urban vehicles, IEEE Trans. Intell. Veh., 1 (2016), 33–55. https://doi.org/10.1109/TIV.2016.2578706 doi: 10.1109/TIV.2016.2578706
![]() |
1. | Xiujun Zhang, Zhiqiang Zhang, Natarajan Chidambaram, Subhasri Jaganathan, Narasimhan Devadoss, Vignesh Ravi, On degree and distance-based topological indices of certain interconnection networks, 2022, 137, 2190-5444, 10.1140/epjp/s13360-022-03010-0 | |
2. | Kinkar Chandra Das, Sourav Mondal, Zahid Raza, On Zagreb connection indices, 2022, 137, 2190-5444, 10.1140/epjp/s13360-022-03437-5 | |
3. | Sourav Mondal, Kinkar Chandra Das, Zagreb connection indices in structure property modelling, 2023, 69, 1598-5865, 3005, 10.1007/s12190-023-01869-5 | |
4. | Deepa Balasubramaniyan, Natarajan Chidambaram, Vignesh Ravi, Muhammad Kamran Siddiqui, QSPR analysis of anti‐asthmatic drugs using some new distance‐based topological indices: A comparative study, 2024, 124, 0020-7608, 10.1002/qua.27372 | |
5. | Yaşar NACAROĞLU, ON LEAP ZAGREB INDICES OF A SPECIAL GRAPH OBTAINED BY SEMIGROUPS, 2023, 6, 2618-5660, 16, 10.33773/jum.1333260 |