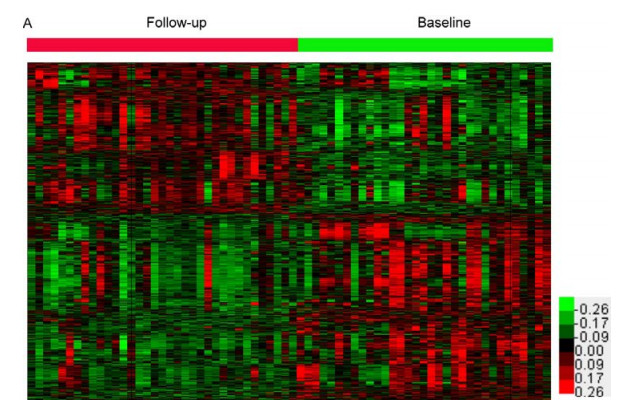
Citation: Chunxiang Fan, Zouqin Huang, Binbin Chen, Baojin Chen, Qi Wang, Weidong Liu, Donghai Yu. Comprehensive analysis of key lncRNAs in ischemic stroke[J]. Mathematical Biosciences and Engineering, 2020, 17(2): 1318-1328. doi: 10.3934/mbe.2020066
[1] | Rongxing Qin, Lijuan Huang, Wei Xu, Qingchun Qin, Xiaojun Liang, Xinyu Lai, Xiaoying Huang, Minshan Xie, Li Chen . Identification of disulfidptosis-related genes and analysis of immune infiltration characteristics in ischemic strokes. Mathematical Biosciences and Engineering, 2023, 20(10): 18939-18959. doi: 10.3934/mbe.2023838 |
[2] | Yonghua Xue, Yiqin Ge . Construction of lncRNA regulatory networks reveal the key lncRNAs associated with Pituitary adenomas progression. Mathematical Biosciences and Engineering, 2020, 17(3): 2138-2149. doi: 10.3934/mbe.2020113 |
[3] | Ming-Xi Zhu, Tian-Yang Zhao, Yan Li . Insight into the mechanism of DNA methylation and miRNA-mRNA regulatory network in ischemic stroke. Mathematical Biosciences and Engineering, 2023, 20(6): 10264-10283. doi: 10.3934/mbe.2023450 |
[4] | Yong Ding, Jian-Hong Liu . The signature lncRNAs associated with the lung adenocarcinoma patients prognosis. Mathematical Biosciences and Engineering, 2020, 17(2): 1593-1603. doi: 10.3934/mbe.2020083 |
[5] | Lishui Shen, Xiaofeng Hu, Ting Chen, Guilin Shen, Dong Cheng . Integrated network analysis to explore the key mRNAs and lncRNAs in acute myocardial infarction. Mathematical Biosciences and Engineering, 2019, 16(6): 6426-6437. doi: 10.3934/mbe.2019321 |
[6] | Ziyu Wu, Sugui Wang, Qiang Li, Qingsong Zhao, Mingming Shao . Identification of 10 differently expressed lncRNAs as prognostic biomarkers for prostate adenocarcinoma. Mathematical Biosciences and Engineering, 2020, 17(3): 2037-2047. doi: 10.3934/mbe.2020108 |
[7] | Sisi Qi, Youyu Sheng, Ruiming Hu, Feng Xu, Ying Miao, Jun Zhao, Qinping Yang . Genome-wide expression profiling of long non-coding RNAs and competing endogenous RNA networks in alopecia areata. Mathematical Biosciences and Engineering, 2021, 18(1): 696-711. doi: 10.3934/mbe.2021037 |
[8] | Yun-xiang Li, Shi-ming Wang, Chen-quan Li . Four-lncRNA immune prognostic signature for triple-negative breast cancer. Mathematical Biosciences and Engineering, 2021, 18(4): 3939-3956. doi: 10.3934/mbe.2021197 |
[9] | Jie Chen, Jinggui Chen, Bo Sun, Jianghong Wu, Chunyan Du . Integrative analysis of immune microenvironment-related CeRNA regulatory axis in gastric cancer. Mathematical Biosciences and Engineering, 2020, 17(4): 3953-3971. doi: 10.3934/mbe.2020219 |
[10] | Beibei Zhu, Yue Mao, Mei Li . Identification of functional lncRNAs through constructing a lncRNA-associated ceRNA network in myocardial infarction. Mathematical Biosciences and Engineering, 2021, 18(4): 4293-4310. doi: 10.3934/mbe.2021215 |
Ischemic stroke (IS) is a leading cause of mortality and disability worldwide [1]. However, the treatments for ischemic stroke remained inadequate. The mechanisms underlying ischemic stroke are still not completely understood. In the past decades, several miRNAs and protein coding genes had been demonstrated to be related to the progression of stroke. For example, the expression levels of miRNA-221-3p, miRNA-382-5p, miRNA-4271 in serum and circulating exosomal miRNA-223 could serve as biomarkers for stroke [2]. Inhibition of miRNA-27b enhances neurogenesis via AMPK activation in a mouse ischemic stroke model [3]. Underlying mechanisms of stroke could be useful to develop new therapeutic biomarkers for Ischemic stroke.
Long non-coding RNAs (lncRNAs) were a type of lncRNAs, whose length was longer than 200 bps and had no protein coding function. Emerging studies indicated lncRNAs played crucial roles in the progression of human diseases. LncRNAs modulated downstream targets through epigenetic, transcriptional and posttranscriptional levels. A few lncRNAs were found to be associated with the progression of stroke. For example, LncRNA-N1LR promoted neuroprotection against ischemic stroke probably by suppressing p53 phosphorylation [4]. LncRNA H19 promoted neuroinflammation in ischemic stroke by driving HDAC1-Dependent M1 Microglial Polarization [5]. Several bioinformatics analyses constructed lncRNAs related to regulatory networks in ischemic stroke. Zheng et al. identified LINC00265, LINC00473, NEXN-AS1, HCG17, and MEG8 as important lncRNAs biomarkers in ischemic stroke [6]. However, the roles of lncRNAs in stroke remained unclear.
In present study, we analyzed a public dataset GSE37587 [7] to identify differentially expressed lncRNAs and mRNAs after stroke recovery in ischemic stroke patients. Next, a lncRNA co-expression network was constructed to identify key lncRNAs related to stroke recovery. Bioinformatics analysis was conducted to explore the potential functions of these lncRNAs in stroke.
We analyzed the GSE37587 dataset to identify differently expressed mRNAs and lncRNAs after stroke recovery, which was downloaded from the GEO database (www.ncbi.nlm.nih.gov/geo/). A total of 34 ischemic stroke patients within 24 hours from known onset of symptom and again at 24-48 hours after onset were included in the GSE37587 dataset. We used two ways of paired t-test to identify key genes. Genes having fold changes ≥ 2 and P values < 0.05 were selected as of significantly differential expression.
To identify functions of DEGs in IS, we performed GO function enrichment analysis in 3 functional ontologies: biological process (BP), cellular component (CC) and molecular function (MF). KEGG pathway enrichment analysis was also performed to identify pathways enriched in IS using DAVID system (https://david.ncifcrf.gov/). The p-value was calculated by hypergeometric distribution and a pathway with p < 0.05 was considered as significant.
The number of genes in the critical modules was put in the order from high to low gene significance scores. Then, functional enrichment analysis was conducted on these genes in these modules. GO Biological Process term and Kyoto Encyclopedia of Genes and Genomes (KEGG) pathway analyses were conducted using Cytoscape plug-in ClueGo. Functional enrichment analysis was based on the cut-off value of P-value < 0.05.
In this study, the Pearson correlation coefficient of differentially expressed gene (DEG)-lncRNA pairs was calculated according to the expression value of them. The co-expressed DEG-lncRNA pairs with the absolute value of Pearson correlation coefficient ≥ 0.5 were selected and the co-expression network was established by using Cytoscape software.
In order to provide insight into the potential mechanisms associated with the stroke recovery, we analyzed the GSE37587 dataset to identify differently expressed mRNAs and lncRNAs after stroke recovery (shown in Figure 1). A total of 34 ischemic stroke patients within 24 hours from known onset of symptom and again at 24–48 hours after onset were included in the GSE37587 dataset. Genes having fold changes ≥ 2 and P values < 0.05 were selected as of significantly differential expression. Totally, 3546 mRNAs were identified as differently expressed mRNAs. Among them, 1686 mRNAs were found to be up-regulated and 1654 mRNAs were found to be down-regulated in follow-up stroke groups compared to baseline stroke groups. Hierarchical clustering showed systematic variations in the expression of mRNAs after stroke recovery.
The expression values of heatmap are scaled by mRNAs and lncRNAs. The samples with Follow-up and Baseline are represented by the bands with red and green, respectively.
Next, we identified differently expressed lncRNAs after stroke recovery. We found 19 lncRNAs were dysregulated. Our results showed that FAM10A6, CDRT15P, MGC10997, FAM10A4, EPR1, RNF5P1, HLA-H, NAG18, LOC338799, RNF126P1, SIGLECP3 were up-regulated and KLKP1, CYP2B7P1, LOC432369, FTHL8, UBE2MP1 and DLEU1 were down-regulated in follow-up stroke groups compared to baseline stroke groups (Figure 2).
Dot-plot assayed for the expression levels of the 17 expressed lncRNAs between Follow-up and Baseline samples. (A) FAM10A6, (B) CDRT15P, (C) MGC10997, (D) FAM10A4, (E) EPR1, (F) RNF5P1, (G) HLA-H, (H) NAG18, (I) LOC338799, (J) RNF126P1, (K) SIGLECP3 were up-regulated and (L) KLKP1, (M) CYP2B7P1, (N) LOC432369, (O) FTHL8, (P) UBE2MP1 and (Q) DLEU1 were down-regulated. The baseline and follow-up samples are green and red. *P < 0.05, **P < 0.01, ***P < 0.001.
Next, we constructed dysregulated lncRNA-mRNA co-expression networks to reveal the potential roles of lncRNAs in stroke recovery. Only the lncRNA-mRNA pairs with the absolute value of Pearson correlation coefficient ≥ 0.45 were selected for network construction. As present in Figure 3, 24 lncRNAs, 1668 mRNAs and 3542 edges were included in this network.
Three lncRNAs, including DLEU1, LOC432369, LOC338799 were identified to play crucial roles in this network. More than 350 mRNAs were regulated by these lncRNAs.
Based on co-expression networks, we performed bioinformatics analysis for differentially expressed lncRNAs by using the set of co-expressed mRNAs. The biological processes analysis showed lncRNAs were involved in regulating mRNA nonsense-mediated decay, translation, cell-cell adhesion, proteasome-mediated ubiquitin, mRNA splicing, via spliceosome, protein polyubiquitination, viral process, autophagy, rRNA processing, and macroautophagy (Figure 4A). Molecular function analysis showed these lncRNAs were involved in regulating protein binding, poly(A) RNA binding, structural constituent of ribosome, RNA binding, cadherin binding involved in cell-cell adhesion, retinoid X receptor binding, identical protein binding, chaperone binding, protein serine/threonine phosphatase activity, and ubiquitin-protein transferase activity (Figure 4B).. Cell competent analysis showed these lncRNAs were associated with cytosol, membrane, nucleoplasm, ribosome, mitochondrion, mitochondrial inner membrane, nucleus, extracellular exosome, cytoplasm, and cell-cell adherens junction (Figure 4C).
KEGG pathway analysis showed these lncRNAs were associated with ribosome, oxidative phosphorylation, ubiquitin mediated proteolysis, biosynthesis of amino acids, spliceosome, carbon metabolism, viral carcinogenesis, 2-oxocarboxylic acid metabolism, proteasome, and lysosome (Figure 4D).
The present study identified DLEU1, LOC432369, LOC338799 as key lncRNAs in ischemic stroke by regulating more than 350 mRNAs. However, the potential roles of these lncRNAs in ischemic stroke remained to be further investigated.
We next performed a bioinformatics analysis of these lncRNAs in ischemic stroke based on their co-expressing mRNAs using Cytoscape's ClueGo plug-in (Figure 5). The pathways with p ≤ 0.05 were considered to be significant. Our results showed DLEU1 was associated with oxidative phosphorylation, spliceosome, ribosome, protein processing in endoplasmic reticulum, ubiquitin-mediated proteolysis. LOC432369 was associated with oxidative phosphorylation. LOC338799 was associated with clathrin-dependent endocytosis, the establishment of organelle localization and ribonucleoprotein complex assembly. These analyses indicated that these pathways were referred to GO analysis.
LncRNAs had been demonstrated as key regulators involved in human disease progression [8]. Emerging studies reported lncRNAs played their roles in human diseases by modulating downstream targets through epigenetic, transcriptional and posttranscriptional levels. For instance, lncRNA Lnczc3h7a binds to TRIM25 and promotes RIG-I-mediated antiviral innate immune responses [9]. LncRNA MALAT1 interacted with and inactivated the pro-metastatic transcription factor TEAD to suppress breast cancer metastasis [10]. Stroke is one of the leading causes of death and disability worldwide. A few lncRNAs were found to be associated with the progression of stroke. For example, LncRNA-N1LR promoted neuroprotection against ischemic stroke probably by suppressing p53 phosphorylation. Suppressing of MALAT1 attenuated neuronal cell death though inhibiting Beclin1-dependent autophagy in ischemic stroke [11]. LncRNA-1810034E14Rik reduced microglial activation in experimental ischemic stroke [12]. Of note, several bioinformatics analyses revealed several lncRNAs related to regulatory networks in ischemic stroke. However, the roles of lncRNAs in stroke remained unclear.
The present study found 19 lncRNAs were dysregulated after stroke recovery. Moreover, we constructed a lncRNAs-mRNAs co-expression network, including 24 lncRNAs, 1668 mRNAs and 3542 edges. Bioinformatics analysis revealed these lncRNAs were involved in regulating multiple biological processes and pathways, such as mRNA nonsense-mediated decay, translation, cell-cell adhesion. Of note, we identify 3 key lncRNAs that were associated with the recovery progression of stroke, including DLEU1, LOC432369, LOC338799. Our results showed DLEU1 was associated with oxidative phosphorylation, spliceosome, ribosome, protein processing in endoplasmic reticulum, ubiquitin-mediated proteolysis. Of note, ubiquitin mediated proteolysis had been reported to play a crucial role in the development of stroke. For example, Wu et al, found upregulated genes in stroke patients were found to be involved in the MyD88-independent pathway and in UBE2V1-TRAF6 ubiquitin-mediated proteolysis [13]. Cheng et al. found Smoking regulating genes in blood of patients with ischemic stroke was also involved in regulating ubiquitin-mediated proteolysis [14]. LOC432369 was associated with oxidative phosphorylation. Very interestingly, a recent study showed that oxidative phosphorylation was related to stroke. Biffi et al. found that genetic variation of oxidative phosphorylation genes was related to stroke [15]. LOC338799 was associated with clathrin-dependent endocytosis, establishment of organelle localization and ribonucleoprotein complex assembly.
Among these lncRNAs, DLEU1 was reported as an important regulator in the progression of multiple human diseases, such as cancers, Sezary syndrome, and sclerosis [16,17]. DLEU1 played as an oncogenetic lncRNAs in multiple cancers, such as bladder cancer, cervical cancer, non-small cell lung cancer, pancreatic ductal adenocarcinoma, colorectal cancer, oral cancer, and endometrial cancer. For example, DLEU1 promotes the development of endometrial cancer by sponging miR-490 to regulate SP1 expression [16]. DLEU1 promotes tumorigenesis of endometrial carcinoma by targeting mTOR. The present study showed DLEU1 was involved in regulating oxidative phosphorylation and ubiquitin-mediated proteolysis by regulating ATP6V0A2 and ATP5I. LOC338799 [18], known as LINC01089, had been reported to be related to syndromic intellectual disability and breast cancer [19]. LOC338799 is a novel metastasis inhibiting lncRNA suppressed by EGF and downregulated in agammaessive breast cancer [20]. A microdeletion in LOC338799 was associated with syndromic intellectual disability [21]. This study showed LOC338799 was involved in the regulation of clathrin-dependent endocytosis, ribonucleoprotein complex assembly in the stroke.
Several limitations of this study should be noted. First, further validation of key mRNAs and lncRNAs in clinical samples could strengthen the importance of this manuscript. Second, the molecular functions of key mRNAs and lncRNAs in regulating stroke recovery remained unclear. The further investigation of their functional roles and underlying mechanisms in stroke recovery were still need.
In conclusion, the present study identified 19 lncRNAs related to stroke recovery by analyzing a public dataset GSE37587. A co-expression network included 24 lncRNAs, 1668 mRNAs and 3542 edges were constructed in present study. Bioinformatics analysis showed these lncRNAs were involved in regulating multiple biological processes and pathways, such as mRNA nonsense-mediated decay, translation, cell-cell adhesion. Three lncRNAs, including DLEU1, LOC432369, and LOC338799, were identified as key lncRNAs in stroke. Bioinformatics showed DLEU1 was involved in regulating oxidative phosphorylation, and ubiquitin-mediated proteolysis. LOC432369 was associated with oxidative phosphorylation. LOC338799 was associated with clathrin-dependent endocytosis, establishment of organelle localization and ribonucleoprotein complex assembly. We thought this study could provide useful information to understand the mechanisms underlying stroke progression.
This work is supported by Clinical Traditional Chinese Medicine Characteristic Discipline of Shanghai, Pudong New Area (Grant No. PDZY-2018-0612), Three-year Action Plan for the Further Development of Traditional Chinese Medicine in Shanghai (Grant No. ZY (2018-2020) -FWTX-8012), Academic Leaders Training Program of Pudong Health Bureau of Shanghai (Grant No. PWRD2018-08), The Outstanding Clinical Discipline Project of Shanghai Pudong (Grant No. PWYgy2018-04), Acupuncture and Moxibustion Characteristic Discipline of Clinical Traditional Chinese Medicine in Pudong New Area (Grant No. PDZY-2018-0610).
The authors declare no financial conflicts of interest.
[1] | A. Arboixand and J. Alio, Cardioembolic Stroke: Clinical Features, Specific Cardiac Disorders and Prognosis, Curr. Cardiol. Rev., 6 (2010), 150-161. |
[2] | S. Sabour, The Diagnostic Value of Serum miRNA-221-3p, miRNA-382-5p, and miRNA-4271 in Ischemic Stroke: Methodological Issue to Avoid Misinterpretation, J. Stroke Cerebrovasc. Dis., 26 (2017), 1161. |
[3] | Z. Wang, Y. Yuan, Z. Zhang, et al., Inhibition of miRNA-27b enhances neurogenesis via AMPK activation in a mouse ischemic stroke model, FEBS Open Bio., 9 (2019), 859-869. |
[4] | Z. Wu, P. Wu, X. Zuo, et al., Erratum to: LncRNA-N1LR Enhances Neuroprotection Against Ischemic Stroke Probably by Inhibiting p53 Phosphorylation, Mol. Neurobiol., 54 (2017), 7670-7685. |
[5] | J. Wang, B. Cao, D. Han, et al., Long Non-coding RNA H19 Induces Cerebral Ischemia Reperfusion Injury via Activation of Autophagy, Aging Dis., 8 (2017), 71-84. |
[6] | J. A. Saugstad, Non-Coding RNAs in Stroke and Neuroprotection, Front. Neurol., 6 (2015), 50. |
[7] | T. L. Barr, R. L. Vangilder, S. L. Rellick, et al., A Genomic Profile of the Immune Response to Stroke With Implications for Stroke Recovery, Biol. Res. Nurs., 17 (2015), 248-256. |
[8] | X. Wan, W. Huang, S. Yang, et al., Identification of androgen-responsive lncRNAs as diagnostic and prognostic markers for prostate cancer, Oncotarget, 7 (2016), 60503-60518. |
[9] | H. Lin, M. Jiang, L. Liu, et al., The long noncoding RNA Lnczc3h7a promotes a TRIM25-mediated RIG-I antiviral innate immune response, Nat. Immunol., 2019 (2019), 1. |
[10] | C. H. Liand Y. Chen, Insight Into the Role of Long Noncoding RNA in Cancer Development and Progression, Int. Rev. Cell Mol. Biol., 326 (2016), 33-65. |
[11] | H. Yang, X. Xi, B. Zhao, et al., KLF4 protects brain microvascular endothelial cells from ischemic stroke induced apoptosis by transcriptionally activating MALAT1, Biochem. Biophys. Res. Commun., 495 (2018), 2376-2382. |
[12] | X. Zhang, X. L. Zhu, B. Y. Ji, et al., LncRNA-1810034E14Rik reduces microglia activation in experimental ischemic stroke, J. Neuroinflammation, 16 (2019), 75. |
[13] | D. Wu, Y. C. G. Lee, H. C. Liu, et al., Identification of TLR downstream pathways in stroke patients. Clin. Biochem., 46 (2013):1058-1064. |
[14] | X. Wang, S. Cheng, V. H. Brophy, et al., A Meta-Analysis of Candidate Gene Polymorphisms and Ischemic Stroke in 6 Study Populations: Association of Lymphotoxin-Alpha in Nonhypertensive Patients. Stroke, 40 (2009):683-695. |
[15] | C. D. Anderson, A. Biffi, M. A. Nalls, et al., Common Variants Within Oxidative Phosphorylation Genes Influence Risk of Ischemic Stroke and Intracerebral Hemorrhage. Stroke, 44 (2013):612-619. |
[16] | T. Liu, Z. Han, H. Li, et al., LncRNA DLEU1 contributes to colorectal cancer progression via activation of KPNA3, Mol. Cancer, 17 (2018), 118. |
[17] | J. Wang, D. Pappas, P. L. De Jager, et al., Modeling the Cumulative Genetic Risk for Multiple Sclerosis from Genome-Wide Association Data, Genome Med., 3 (2011), 3. |
[18] | T. Kim, S. J. Choi, Y. H. Lee, et al., Gene expression profile predicting the response to anti-TNF treatment in patients with rheumatoid arthritis; analysis of GEO datasets, Jt. Bone Spine, 81 (2014), 325-330. |
[19] | G. W. E. Santen, M. Kriek and H. Van Attikum, SWI/SNF complex in disorder: SWItching from malignancies to intellectual disability, Epigenetics, 7 (2012), 1219-1224. |
[20] | A. Saschen, M. R. Aure, L. Leibovich, et al., LIMT is a novel metastasis inhibiting lncRNA suppressed by EGF and downregulated in aggressive breast cancer, Embo Mol. Med., 8 (2016), 1052-1064. |
[21] | J. D. J. Labonne, T. Graves, Y. Shen, et al., A microdeletion at Xq22.2 implicates a glycine receptor GLRA4 involved in intellectual disability, behavioral problems and craniofacial anomalies, BMC Neurol., 16 (2016), 132. |
1. | Daowei Zhang, Jiawen Wu, Shenghai Zhang, Jihong Wu, Identification of Immune Infiltration-Related ceRNAs as Novel Biomarkers for Prognosis of Patients With Primary Open-Angle Glaucoma, 2022, 13, 1664-8021, 10.3389/fgene.2022.838220 | |
2. | Suresh L. Mehta, Anil K. Chokkalla, Raghu Vemuganti, Noncoding RNA crosstalk in brain health and diseases, 2021, 149, 01970186, 105139, 10.1016/j.neuint.2021.105139 | |
3. | Haoran Guo, Xueran Kang, Ying Xu, Chengbin Wang, Chi Wang, Ginkgo biloba active compounds can modulate the development of acute mountain sickness and ischemic stroke as discovered by network pharmacology and molecular docking, 2024, 2, 2834-443X, 178, 10.1002/ila2.58 | |
4. | Meiying Yang, Yufang Gu, LncRNA DLEU1 promotes angiogenesis in diabetic foot ulcer wound healing by regulating miR-96-5p, 2024, 193, 0021-1265, 241, 10.1007/s11845-023-03471-x | |
5. | Ebenezer Oladiemji Awotoro, Makolo Angela, Computational pipeline for long non-coding RNA sequence data to identify differential expressed genes, 2024, 14, 2141-2464, 1, 10.5897/JBSA2022.0120 |