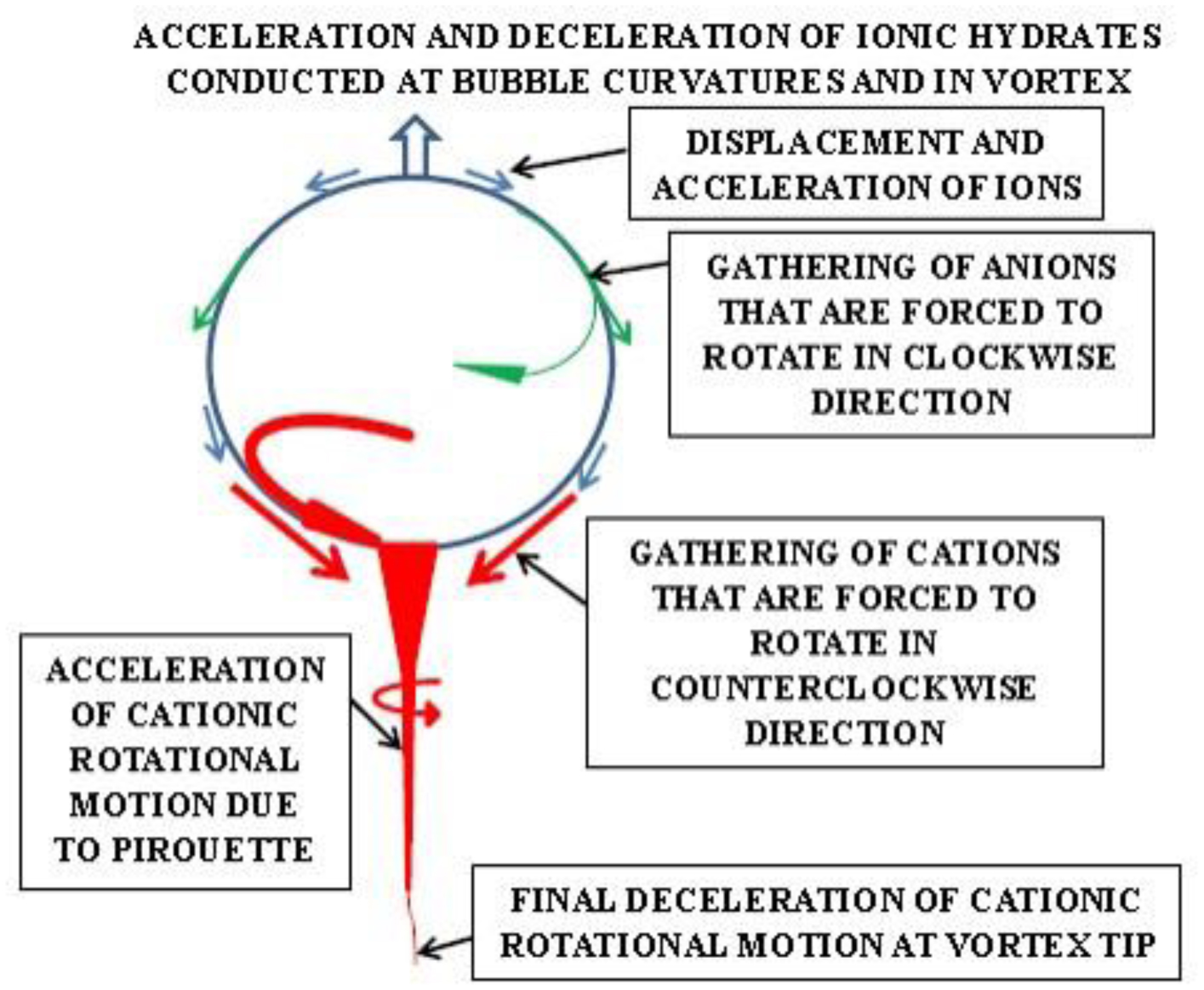
Photographic investigations of rising bubbles in seawater revealed that each bubble may conduct a single or bi-spiraling motion, which resemble architecture of RNA or DNA respectively. The rotational motion results from acceleration of ionic hydrates, which are separated to anionic and cationic domains at the upper and bottom curvatures of the bubble. Afterwards, rotational motion undergoes further acceleration in the bubble upper vortex, followed by deceleration at the vortex tip. During that phase, the spiraling motion cause significant friction that result in polarization of electronegative atoms of H, C, N, O and P. These may be simultaneously arranged around a whirling cationic strands and form phosphate groups, ribose and nitrogen bases equipped with H2 and H3 rotors. It is hypothesized that such hydrogen rotors may operate as generators of electrons, which may be detached from valence shells of electropositive atoms. Then, electrons may flow via nitrogen bases and deoxyribose or ribose to phosphate groups. Next, the negatively charged edges of phosphate groups may attract cationic hydrates and energize their rotational motion in the grooves, then causing also its spiraling projection outward. That may be responsible for replication of nucleotides and its arrangement along the cationic flow into RNA or DNA polymers, in the same manner as originally produced by rising bubbles. Moreover, it points that hydrogen rotors may generate energy needed for viability as well as interact with all physical and chemical fields.
Citation: Roman Marks, Piotr H. Pawłowski. Rotational-electric principles of RNA/DNA and viability[J]. AIMS Biophysics, 2023, 10(3): 385-400. doi: 10.3934/biophy.2023023
[1] | Roman Marks . Bubble mediated polymerization of RNA and DNA. AIMS Biophysics, 2022, 9(2): 96-107. doi: 10.3934/biophy.2022009 |
[2] | Moataz M. Fahmy, Sohier M. El-Kholey, Seham Elabd, Mamdouh M. Shawki . Effect of changing the alternating electric current frequency on the viability of human liver cancer cell line (HEPG2). AIMS Biophysics, 2025, 12(1): 1-13. doi: 10.3934/biophy.2025001 |
[3] | István P. Sugár . Electric energies of a charged sphere surrounded by electrolyte. AIMS Biophysics, 2021, 8(2): 157-164. doi: 10.3934/biophy.2021012 |
[4] | Alexander G. Volkov, Yuri B. Shtessel . Electrotonic signal transduction between Aloe vera plants using underground pathways in soil: Experimental and analytical study. AIMS Biophysics, 2017, 4(4): 576-595. doi: 10.3934/biophy.2017.4.576 |
[5] | Alexander G. Volkov, Yuri B. Shtessel . Propagation of electrotonic potentials in plants: Experimental study and mathematical modeling. AIMS Biophysics, 2016, 3(3): 358-379. doi: 10.3934/biophy.2016.3.358 |
[6] | Arsha Moorthy, Alireza Sarvestani, Whitney Massock, Chamaree de Silva . Twisting and extension: Application of magnetic tweezers to DNA studies. AIMS Biophysics, 2023, 10(3): 317-346. doi: 10.3934/biophy.2023020 |
[7] | Piotr H. Pawłowski, Piotr Zielenkiewicz . The role of electric charge in SARS-CoV-2 and other viral infections. AIMS Biophysics, 2024, 11(2): 166-188. doi: 10.3934/biophy.2024011 |
[8] | Richard C Petersen . Free-radicals and advanced chemistries involved in cell membrane organization influence oxygen diffusion and pathology treatment. AIMS Biophysics, 2017, 4(2): 240-283. doi: 10.3934/biophy.2017.2.240 |
[9] | Thomas D. Christian, William H. Konigsberg . Single-molecule FRET reveals proofreading complexes in the large fragment of Bacillus stearothermophilus DNA polymerase I. AIMS Biophysics, 2018, 5(2): 144-154. doi: 10.3934/biophy.2018.2.144 |
[10] | Massimo Fioranelli, Maria Grazia Roccia, Aroonkumar Beesham, Dana Flavin, M. Ghaeni, Faissal AZIZ . A model for considering effects of extremely low frequency electromagnetic fields on quail embryonic cells. AIMS Biophysics, 2022, 9(3): 198-207. doi: 10.3934/biophy.2022017 |
Photographic investigations of rising bubbles in seawater revealed that each bubble may conduct a single or bi-spiraling motion, which resemble architecture of RNA or DNA respectively. The rotational motion results from acceleration of ionic hydrates, which are separated to anionic and cationic domains at the upper and bottom curvatures of the bubble. Afterwards, rotational motion undergoes further acceleration in the bubble upper vortex, followed by deceleration at the vortex tip. During that phase, the spiraling motion cause significant friction that result in polarization of electronegative atoms of H, C, N, O and P. These may be simultaneously arranged around a whirling cationic strands and form phosphate groups, ribose and nitrogen bases equipped with H2 and H3 rotors. It is hypothesized that such hydrogen rotors may operate as generators of electrons, which may be detached from valence shells of electropositive atoms. Then, electrons may flow via nitrogen bases and deoxyribose or ribose to phosphate groups. Next, the negatively charged edges of phosphate groups may attract cationic hydrates and energize their rotational motion in the grooves, then causing also its spiraling projection outward. That may be responsible for replication of nucleotides and its arrangement along the cationic flow into RNA or DNA polymers, in the same manner as originally produced by rising bubbles. Moreover, it points that hydrogen rotors may generate energy needed for viability as well as interact with all physical and chemical fields.
Air-sea interactions cause breaking waves that disperse immense number of bubbles in the surface water [1]–[4]. After generation, bubbles rise through the water column and promote solubility of trapped tropospheric gases as e.g. nitrogen, oxygen, carbon dioxide and methane. Next, dissolved gases may contribute to respiration, photosynthesis, oxidation of matter, and evade back to air [5],[6]. In addition, it was discovered that bubbles in seawater may polarize electronegative elements and condense them along the single spiraling cationic flow or bi-spiraling cationic and anionic flows. Such processes are activated in the bubble vortices, during the narrowing of motion. That is causing a pirouette increase of revolutions, which beforehand have been generated at bubble curvatures by 105 fold [7],[8]. As a consequence, the rotational energy is extremely focused, causing friction and polarization of molecules and elements dissolved in the water. Finally, at the subatomic scale, the rotational processing lead to subtraction of electrons from electropositive elements and gathering them by electronegative atoms as H, C, N, O and P. In that way continuously polarized electronegative elements undergo attraction and condensation along the spiraling cationic flow forming highly electronegative blocks of molecules and macromolecules [8].
Other experiments unveiled that water molecules themselves behave as perfect rotors. That unique feature was revealed by rotary detection discs suspended in air over the evaporating water surfaces [9]. The laboratory investigations showed that evaporating water molecules gathers significant rate of energy, which is transferred to air. That, rotational energy called “latent heat of vaporization” was classified as resulting from rotational motion of H–H arms operating in H2O, which start to whirl at the moment of evaporation [9].
Experimental evidences revealing the helicoid-like movements of rising bubbles were obtained using photography method [10]. The movements consist of a cationic flow that is aggregated on the bubble bottom half sphere and anionic flow that is generated on the upper half sphere [7],[10],[11]. Helical architecture of a single cationic motion resembles configuration that is typical for of RNA, while cationic/anionic motion shows typical double-helix-like structure. Further investigations revealed that each rising bubbles may conduct a counterclockwise rotational motion in the bottom half sphere and/or clockwise at bubble upper half sphere, in the Northern Hemisphere [10].
Both experimental research lines revealed the importance of hydrogen rotors operating in water molecules. The same rotational energy is also operative in the case of cationic hydrates, which are surrounded by many water molecules exposing their hydrogen rotors outward [8]. Therefore, the combination of results obtained from experimental observations of swirling bubbles and evaporating water molecules were adopted to explain the rotational-electric principles that are activated during the process of RNA and DNA replication.
In this paper we further explore experimental observations that revealed the rotational motion of rising bubbles (chapter 2). Particular attention is given to acceleration and deceleration of cationic rotational motion (chapter 3); which explains the rotational-electric principles of formation and functioning of phosphate group (section 3.1), formation of deoxyribose/ribose and its electric functions (3.2), formation and functioning of nitrogen bases possessing hydrogen rotors (3.3) and rotational-electric system of storage information in nucleotides (3.4). Chapter 4 presents rotational-electric principles of self-replication. Discussion (chapter 5) is divided to geochemical investigations (5.1), electric charge investigations (5.2) and the importance of rotational motion (5.3).
All observations on rotational behavior of rising bubbles that are considered in this study were obtained in the Northern Hemisphere and base mainly on the laboratory investigations. These revealed that during the interception of ionic hydrates into bubble convex and concave curvature; ions are immediately fractionated to anionic and cationic domains and simultaneously accelerated. As a result, anionic-dextrorotary rotational motion is continuously generated at bubbles upper half sphere, while cationic-levorotary motion is developing at lower half sphere and is continued in bubble vortices (see Figure 1).
Bubble tracers were captured by photography methods using upward directed light in time of 1/5 to 1/30 s. These, typically showed a few circuits like rotational features, which afterwards were scaled [7],[8],[10]. In addition, beside the photography records, also the formation of cationic counterclockwise rotational motion that develops within the bubble vortices was confirmed by rotary detection discs. During that experiments discs were exposed to streams of vortex-jet droplets produced by bursting bubbles and developed levorotary motion in the Northern Hemisphere [11].
The processing of ions incorporated into the bubble wall along with separation into anionic and cationic domains and pirouette acceleration, followed by deceleration of cationic motion is presented in Figure 1.
After the initial acceleration of ionic motion at bubble external wall, further enhancement in rotational motion take place within the bubble vortex due to pirouette effect (Figure 1). That effect results in focusing energy, which is simultaneously dissipated by friction and generation of heat along with a Larmor electromagnetic radiation [12]. In turn, gradual deceleration of rotational motion is induced and goes from large ionic hydrates, via heavier atoms of P then is continued on relatively smaller atoms of O, N, and C and is terminated on the smallest atoms of H. During that phase hydrogen atoms are centrifugally displaced outwards.
Considering that the convergent motion of cationic hydrates may be pulled to a distance of c. 10 times of bubble diameter, it indicates that cationic rotation may play a dominating role [7]. Thus, the cationic motion is inducing significant friction between atoms and polarization of electronegative elements, from which blocks of nucleotides may be continuously braided [8]. If that processing is undisturbed, the negatively charged blocks of nucleotides may be steadily attracted towards cationic flow and then arranged into elongated, spiral polymers of RNA or DNA.
Continuous displacement and acceleration of ions along the rising bubble curvatures results in separation of ions into anionic and cationic domains within upper and bottom half spheres. The principle of separation is based on mass differences between anions and cations. Since, anions are relatively heavier than cations, these are more resistant to displacement, thus are gathered within the upper half sphere of rising bubble. These conduct outward motion, therefore may be dispersed in the vicinity of bubble equator. In contrast, cationic hydrates are lighter thus may be drawn under rising bubble and conduct strong convergent motion, which may form bubble vortex [11]. The estimated number of helical circuits conducted at the tip of vortex may be of a 105 s−1 range, indicating a high potential of such cationic flow to polarize elements drawn into vortex and catalyze electronegative blocks of polar molecules, which then may be continuously arranged into elongated polymers [7].
It is expected that during deceleration of cationic-rotational motion proceeded at the tip of bubble vortex the heavier phosphorus atom with an atomic mass of 30.97 u may first become centrally positioned, then encased by four O atoms with a mass of 15.99 u and three H atoms of 1 u. Thus, the process of phosphorus group formation (H3PO4) may rely on combination of at least two factors: atomic masses and electronegativity of atoms drown into the bubble vortex, as illustrated in Figures 1 and 2.
Since, the centrally positioned phosphorus has three electron shells, in which the outer is occupied by only 5 electrons (of total 18) it suggests that P may function as capacitor and router for electrons. Moreover, considering that the third, covalent shell of P offers accommodation of 13 more electrons, it indicates the highest electronegative potential of phosphorus, among other elements found in RNA and DNA. Therefore, the flow of electrons, if generated by nitrogen bases (see subchapter 3.2) may be responsible for attraction of cationic hydrates towards groove and rutting electrons from phosphate group to cations. The flow of electrons is indicated by red vectors and red rotor, which illustrate the electrical function of phosphate group (see Figure 2).
The documented formation of sub-bubble cationic vortices unveiled the system of continuous electrical polarization of elements dissolved in seawater and related rotational arrangement of them into electronegative rings composed of C, O and H. During that processing, four atoms of C (each of 12.01 u) and one atom of O (15.99 u) might be closed into (COCCC) ring that is encased by four atoms of H (1 u), three O–H groups and one group of C–H2–O–H. Such configuration confirms that rotational forcing might be responsible for positioning atoms of H, outward of C and O, see Figure 3.
Such configuration suggests that the process of deoxyribose or ribose formation might result from fast revolving cationic hydrate (Na)(OH2)n, that close i.e. (rotationally catalyze) flatted ring containing beforehand polarized electronegative atoms of C, O and H. As a result, the ring composed of C and O may share the outer electron shells forming electrical circuit, as illustrated in Figure 2. In there, the inflow of electrons through H-O switch (left panel) is marked by bold red arrow. Then, the excess electron is expected to excite (COCCC) ring and shift position of H atom exposed by outer CH2–O–H group, allowing further transfer of electron to phosphate group (right panel). Continuity in such flow of electrons is needed to ensure steady supply of negative charge for deoxyribose or ribose. Then, the flow of electrons may interact with cationic hydrates, forcing their motion in the RNA or DNA grooves, as illustrated by bold red arrows in Figure 2.
Deceleration of rotational motion conducted at tip of bubble vortex is expected to incorporate both cationic motion and compensative motion of anionic hydrates. Thus, the rotational motion around cationic hydrate is expected to close smaller pentagonal rings while an anionic one may close larger hexagonal rings [8]. However, during the final deceleration of whirling motion also a hydrogen groups of N–H2 (amine) and C–H3 (methyl) may be positioned outward and take over the remaining rotational energy (as indicated by red rotors in Figures 4, 5, 6 and 7). In that way, each of the nitrogen bases (beside uracil) may be equipped with H–H or H–H–H rotor that can generate electrons by frequent replenishing covalent shell of H by second electron. Afterwards, the hydrogen atom that accepted electron become rotationally balanced; but overloaded H will tends to donate the excess electron to the shared valence shell of N or C and then to C incorporated to ringed nitrogen base. Next, the electrons may be transferred to ribose or deoxyribose and then to phosphorus in phosphate group. Finally, that flow of electrons is expected to attract highly mobile cationic hydrates [8]. As a result, the cationic atmosphere [13] can be continuously energized; forming dynamic flow in RNA/DNA grooves and causing projection of spiraling and rotating cationic flow outside the grooves (Figure 2).
Considering that the narrowing of rotational motion conducted in the bubble vortex goes from the mm to pm range scale, it suggest that molecules assembled in such vortices should base on the dominating i.e. rotational motion in counterclockwise direction, conducted by cationic hydrates (as illustrated in Figures 4, 5, 6 and 7), in case of motion observed from above in the Northern Hemisphere [7],[10]. The directions of anionic and cationic rotations refer to directions observed at the rising bubble walls, as illustrated in Figure 1. Thus, it is expected that counterclockwise motion is also conducted by hydrogen rotors operating at the ringed nitrogen bases.
It is inferred that single electrons revolving in each of H atoms allow frequent expositions of proton and promote accommodation of second electron by each of the hydrogen atom operating as rotor. Then, the excess electron may immediately drop to covalent shell of nearest electronegative atom of nitrogen or carbon. Therefore, the frequent dropping of electrons by each of two or three hydrogen atoms operating as rotor may generate flow of electrons and induce electric current.
In addition, it is expected that hydrogen rotors operating at nitrogen bases memorize the original bubble rotational-electric feature, which then may be regenerated by H2 or H3 rotors. Therefore, we may regard the generation and flow of electrons as process that might be responsible for replication of continuous cationic motion in RNA/DNA grooves. More precisely, the generation of single stranded rotational motion may be responsible for reactivation of cationic flow during replication of RNA, while bi-stranded cationic and anionic/cationic motion may allow reactivation of two opposite directed flows and therefore replication of double stranded DNA.
The revolutions of hydrogen rotors might result from the smallest possible size of hydrogen atom, which has empirical atomic radius of 25 (± 5) pm [14]. For comparison, the empirically established atomic radii of C, N and O are: 70 (± 5) pm, 65 (± 5) pm and 60 (± 5) pm respectively [14]. Thus, such small size of H atoms allow them to operate as active connectors being also electric switches, while small H rotors allow to conduct synchronized high frequency revolutions resulting in frequent subtractions of electrons form atoms located around. Then such electrons may be donated and accommodated by electronegative elements. Both hydrogen features should be considered as profound operational features allowing extremely efficient functionalities of RNA and DNA.
The only nitrogenous base without hydrogen rotors is uracil (C4H4N2O2) that is found in RNA. However, uracil is equipped with two N–H and two C–H groups that expose H atoms. Therefore, the H atoms in uracil may act as active switches allowing oscillatory transport of electrons between the (CNCNCC) ring and ribose.
Electrons, if generated by hydrogen rotors in nitrogen bases, may be continuously transferred to valence shell of electronegative atoms. Among them C, N and O have two valence shells, each of 8 electrons total capacity. Such configuration allows those atoms to share its covalent shells and form electric circuits enhancing capacity of electron flows. As a result, the shared shells conduct electric current that provide negative charge for each nucleotide. That system may cause induction of electric current, as illustrated in (Figures 4, 5, 6 and 7).
Finally, the generated electrons are transferred to deoxyribose or ribose (Figure 3) then to phosphate group (Figure 2) and finally energize H–H rotors exposed by tens of water molecules that are surrounding each cationic hydrate. Since, even billions of phosphate groups are accessible along each groove in RNA/DNA; the flow of electrons to cationic hydrates may be effectively energized. However, in order to initiate and maintain the attraction of cationic hydrates as well as conduct their rotational flow, steady access to water, cations and stable external source of energy is needed.
It has to be explained that the same rotational and electric processes are activated in bubble vortices, where the cationic hydrates may conduct even a few million revolutions per second [8]. Nevertheless, during the deceleration of cationic motion, the revolutions are gradually reduced and finally taken over by hydrogen rotors that are protruding in Cytosine (C), Guanine (G), Adenine (A) and Thymine (T) see Figures 4–7. These rotors “memorize” cationic flow and then may reproduce its electrical features. Thereafter, the reverse flow of electrons may generate flow of electrons allowing coherent replication of nucleotides and segments of RNA and DNA.
Considering the bubble mediated rotational-electric process we pose a hypothesis that the principles of storage, translation, transcription and replication of RNA/DNA is related to hydrogen atoms that may operate as a high frequency rotors. Thus, at this stage of research we state that C, G, A and T bases memorize (originally coded by rising bubbles) cationic rotational motion. Then, each base may reactivate backward flow of electrons through nucleotides, to cationic hydrates, thereby cause their self-replication. That may explain the principles of precise, i.e. rotational and electric replication of coherent polymeric RNA/DNA. In other words, the process of replication may rely on continuous generation of electrons by H rotors operating in the C, G, A bases as (N–H2) and in T base as (C–H3). Then, electrons may be transferred through the nitrogen bases and sugar-phosphate groups to cationic hydrates. Finally, if flow of cationic hydrates is energetic enough, it may be spirally projected out from grooves, polarize electronegative atoms, from which nucleotides may be coherently entwined. Such continuous rotational-cationic motion may likely read (stored in grooves) and copy (outside the grooves) sequences of cationic flow (previously coded by rising bubbles) that then may be translated to nucleotides. Thus, reverse rotational-electric flow of cationic hydrates may reproduce coherent nucleotides. In addition, one may infer that continuity in such flow may therefore also steadily repair faulty sequences, if they were inconsistently assembled. Therefore, we regard the electrically coded cationic rotational motion as responsible for rotational-electric replication of nitrogen bases, ribose or deoxyribose and phosphate group along with their integration into chains of nucleotides that may be arranged as single-helix RNAs or double-helix DNAs. However, replication may require renewable access to cationic/anionic hydrates and precisely balanced flux of energy.
In order to discuss our preliminary research one should consider at list three particular lines of experimental investigations.
The Miller and Urey [15] experiment and similar later trials show that nucleotides and other organic building blocks may be formed under a relatively wide range of conditions that could have been present in the primordial so call “hot oceanic soup”. The current view is that individual nucleotides that bonded together to form RNA are very unstable and quickly broke down. However, some stable or somehow better protected RNAs may grow, finally forming self-copying RNA. It is expected that these RNAs were better at copying themselves than others, so they persisted longer and became more abundant, according to the rule of natural selection. Nevertheless, the Miller and Urey, didn't find any elongated complexes like RNA and DNA polymers. Therefore, we are confident that the processes conducted in a bubble vortices ensure the assemblage of elongated polymers.
According to the RNA world discontinuous synthesis model, segments of RNA may be spontaneously produced from formaldehyde (HCHO) and glycolaldehyde (HOCH2–CHO), especially in alkaline aquifers, if being generated by serpentinizing rocks. This substrate may have been produced by electrical discharge in the ancient atmosphere containing mainly CO2, H2O, and N2. However, many steps of this non-marine model have widely recognized fundamental problems. This causes the search for models useful in other environments, e.g., oceanic [16]. Our model, which is based on marine bubbles, seems not to suffer from that so call “asphalt syndrome”.
At the initial stage of life formation in the Earth's warm ocean at temperature range of about 50–60 °C, a single-cationic bubbles rotational motion could have prevailed, favoring formation of RNA. Thus, spiral molecules generated under such conditions could have become one of the most important sources of proto-tRNA and aminoacyl-tRNA in syntheses of protoribozyme. According to so called “The Smooth Evolution” hypothesis [17], these polymers, formed in the “Pre-Code Hot Era”, could have been the basic matrix in the early evolution of the genetic codes. These should be rich in poly-A and poly-U regions, but also contain some G and C nitrogen bases. However, this suggests that the first informative molecules may have been RNA polymers generated by bubbles forming only single-cationic rotational motions in a relatively warm ocean [18]. On the other hand, their instability in highly ionic seawater could have provided abundant stock of electronegative molecules, from which bubbles could selectively (electro-dynamically) assemble a self-replicating forms of RNA and DNA.
Considering the generation of electrons by hydrogen rotors operating in the nitrogen bases we may finally explain the mechanism of electrons supply to nucleotides and dynamical restoration of cationic atmosphere around RNA/DNA [19]. However, for polymerase continuous lamination by electroneutral matter [8] is needed to protect the assembled polymer from charge recombination. Thus only protected polymers may have the chance to preserve the ability of electron generation and provide operational source of so called “chemical energy” needed for life [20].
Similar mechanism is responsible for electric charge mediated scavenge and aerosolization of negatively charged bacteria cells [21]–[23], diatoms [11] and enveloped viruses [24],[25]. The same electric mechanism of charge mediated adhesion and then transmission of RNA via the positively charged spikes, which are exposed by coronaviruses, to negatively charged membranes of host's biological cells, was reported by Pawłowski [26].
At the molecular scale, the rotational interactions conducted within bubble vortex enforces at least two other processes: 1) increase in electrostriction as a result of vortex narrowing, and 2) induction of counter-currents according to the Lenz's rule. The related quenching of the vortex is the effect of energy dissipation due to friction and so called Larmor electromagnetic radiation [12]. A less significant factor may be the electromagnetic vortex effect, which is greatest at higher latitudes, where the Earth's magnetic field is perpendicular to the surface. In the southern hemisphere, the directions of rotation and induced currents will be opposite. For example, the average density of a charge eddy current (current/flow area) in the upper half of the bubble, estimated based on the formula (1) resulting from Faraday's law of electromagnetic induction:
where: the specific electrical conductivity of seawater, sigma = 4 [S/m]; magnetic induction near the earth's surface, B = 50·10−6 [T]; and bubble rising velocity, v = 2·10−2 [m/s], gives a j value of about 2·10−6 [A/m2]. It is much more than the mean atmospheric current density between the Earth's surface and the ionosphere, under fair weather conditions, which equals to c. 2·10−12 [A/m2].
The electric, i.e. circuit like charge transfer in DNA was already proposed by Xu [27]. Author assumed that DNA can be treated as an oscillating electrical circuit in which each pair of nitrogenous bases acts as a capacitor; deoxyribose acts as a charge router, while each of the sugar-phosphate bridge acts as a charge inducer. In general, the Xu model is consistent with model presented in this paper, but our concept, in addition explains the mechanism of electron supply to nucleotides as well as to cationic hydrates. Both features are needed to ensure dynamics of ionic atmosphere and related induction of cationic motion generated in RNA/DNA grooves.
Investigations of nucleic acids optical properties have shown that all nitrogen bases absorb ultraviolet light in the wavelength range from 249 nm to 276 nm. However, bi-ringed guanine and adenine absorb a bit more light within a slightly shorter wavelength in ultraviolet range: guanine has an absorbance maximum at 249 nm and adenine at 265.5 nm. Whereas, single ringed bases as uracil show absorbance at 260 nm, thymine at 265 nm, and cytosine at 276 nm. In addition, each of the nitrogen bases also differs in the intensity of light absorption at its maxima [28],[29]. Our research suggests that the process of light absorption may result from interactions between light and hydrogen rotors, since these may swirl with a frequency allowing interception of photons. Although, a lack of such activity is expected for uracil. This however suggest, that the N–H and C–H switches that are exposed in uracil as well as in all other nitrogen bases may interact with photons and absorb light.
In highly ionic seawater, the negatively charged phosphate acid residues in nucleotides do not favor the interconnection to polynucleide strands found in the ancient warm ocean of the RNA world [18]. The electrostatic repulsion is further supported by the Debye screening (persistence of electrostatic effects induced by ions) within the surrounding ion cloud chains. Under the conditions analyzed in this work, the formation of bubble vortex may significantly reorganize the ion cloud and catalyze elongated electronegative RNAs or DNAs. As a result, the hydrophilic negatively charged phosphate residues of nucleotides can orient themselves towards the positively charged stream that is entangling the vortex axis in its end part; thus exposing nitrogen bases in the peri-axial zone, which should enable hydrophobic stabilization of elongated structures. Although, this mechanism in the case of interacting strands does not ensure flawless in complementarity of A–U and G–C, possible linkages, non-specifically loosely entangled strands may facilitate the formation of “correctly” bound bases. In addition, positively charged amino acids (lysine, arginine or histidine) can attach to negative skeletons. The resulting assemblage of molecular complexes of this kind may reveal catalytic properties or be the matrix forming ribosomes. However, the binding of double helix structure of DNA is possible only when ribose is replaced with deoxyribose, and uracil with thymine.
Since, hydrogen is the most pervasive element in the hydrosphere as well as in the lithosphere; it might have been played a dominating role in synthesis of RNA/DNA and evolution of life. Considering that fact, we suggest that hydrogen rotors with ability to generate electrons may also sense and adjust to physical and chemical fields operating on the Earth. Especially, long term interactions with solar, thermal, gravitational, electric, magnetic and acoustic fields that have significant energies might have been shaping the course of evolution. Thus, we state that RNA and DNA, beside the ability of self-replication, possess also ability of self-adjustment to physical and chemical fields. The principle role in those abilities is played by hydrogen rotors. These may have allowed RNA/DNA to transform into self-replicating lifeforms, which firstly emerged in the ocean, then colonized coastal areas and lands.
Intriguing is also a fact that rotational processes are important for warming climate, since all of the gases absorbing thermal energy have rotational configuration. In particular the H–H rotors that are whirling around oxygen atoms in water vapor molecules as well as a pair of such rotors operating in methane (CH4) may effectively store energy in the troposphere. These rotors can intercept upward directed thermal radiation, which is trapped, causing warming climate on the Earth [9].
It is worth to underline that the importance of rotational motion was for the first time correctly described by Nicolaus Copernicus in his hand written manuscript entitled “Commentariolus” over 500 years ago (the original manuscript is presently a museum material in possession of the Center of History of Science of the Swedish Royal Academy of Sciences in Stockholm). We are confident that rotational motion plays also a basic role in all electrostatic and electrodynamic activities in RNA/DNA, as for example studied in molecular scales by means of experiments and mathematical modelling e.g. [30]–[35]. The same rotational principles impose all rotational-electric interactions that are responsible for efficient transcription and replication of RNA/DNA, transport of molecules in biological cells as well as internal and external communication that is conducted in all lifeforms.
Considering that here outlined research base only on observations dedicated to rotational motion of rising bubbles, we call for more extended investigations exploring rotational and electric processes at molecular and atomic scales including even a sub-atomic processes. In particular, the generation of electrons by hydrogen rotors operating in methyl and amine groups and related flow of electrons through the hydrogen switches needs to be investigated.
The authors declare they have not used Artificial Intelligence (AI) tools in the creation of this article.
[1] | Blanchard DC, Woodcock AH (1957) Bubble formation and modification in the sea and its meteorological significance. Tellus 9: 145-158. https://doi.org/10.3402/tellusa.v9i2.9094 |
[2] |
Monahan EC (1971) Oceanic whitecaps. J Phys Oceanogr 1: 139-144. https://doi.org/10.1175/1520-0485(1971)001%3C0139:OW%3E2.0.CO;2 ![]() |
[3] |
Liss PS (1983) Gas transfer: experiments and geochemical implications. Air-Sea Exchange of Gases and Particles. Dordrecht: Springer Netherlands 241-298. https://doi.org/10.1007/978-94-009-7169-1_5 ![]() |
[4] | Garbalewski C, Marks R (1987) Latitudinal characteristics of aerosol distribution in the near surface air over the Atlantic. Acta Geophys Pol 35: 77-86. |
[5] |
Stramska M, Marks R, Monahan EC (1990) Bubble-mediated aerosol production as a consequence of wave breaking in supersaturated (hyperoxic) seawater. J Geophys Res Oceans 95: 18281-18288. https://doi.org/10.1029/JC095iC10p18281 ![]() |
[6] |
Woolf DK, Thorpe SA (1991) Bubbles and the air-sea exchange of gases in near saturation conditions. J Mar Res 49: 435-466. ![]() |
[7] | Marks R (2015) Sub-bubble bi-pirouette splicing of cationic and anionic bases as a process of RNA/DNA creation. Oceanography 2: 128. https://www.researchgate.net/publication/284766375 |
[8] |
Marks R (2022) Bubble mediated polymerization of RNA and DNA. AIMS Biophys 9: 96-107. https://doi.org/10.3934/biophy.2022009 ![]() |
[9] |
Marks R (2019) Water vpor iduced arborne rtational fatures. Meteorol Hydrol Water Manage 7: 39-47. https://doi.org/10.26491/mhwm/104634 ![]() |
[10] | Marks R (2014) Bubble rotational features-preliminary investigations. Oceanography 2: 128. https://doi.org/10.4172/2332-2632.1000128 |
[11] |
Marks R, Górecka E, McCartney K, et al. (2019) Rising bubbles as mechanism for scavenging and aerosolization of diatoms. J Aerosol Sci 128: 79-88. https://doi.org/10.1016/j.jaerosci.2018.12.003 ![]() |
[12] | Larmor J (1987) On the theory of the magnetic influence on spectra; and on the radiation from moving ions. Philosoph Magazine 44: 503-512. https://doi:10.1080/14786449708621095 |
[13] |
Lipfert J, Doniach S, Das R, et al. (2014) Understanding of nucleic acid-ion interactions. Annu Rev Biochem 83: 813-841. https://doi.org/10.1146/annurev-biochem-060409-092720 ![]() |
[14] |
Slater JC (1964) Atomic Radii in crystals. J Chem Phys 41: 3199-3204. https://doi.org/10.1063/1.1725697 ![]() |
[15] |
Miller SL, Urey HC (1959) Organic compound synthesis on the primitive earth. Science 130: 245-251. https://doi.org/10.1126/science.130.3370.245 ![]() |
[16] |
Benner SA, Kim HJ, Carrigan MA (2012) Asphalt, water, and the prebiotic synthesis of ribose, ribonucleosides, and RNA. Acc Chem Res 45: 2025-2034. https://doi.org/10.1021/ar200332w ![]() |
[17] | Pawłowski PH (2019) The smooth evolution of the universal genetic code. Main episodes. Inter J Sci 8: 28-51. https://doi.org/10.18483/ijSci.218019 |
[18] |
de Farias ST, dos Santos Junior AP, Rêgo TG, et al. (2017) Origin and evolution of RNA-dependent RNA polymerase. Front Genet 8: 125. https://doi.org/10.3389/fgene.2017.00125 ![]() |
[19] |
Draper DE (2008) RNA folding: Thermodynamic and molecular descriptions of the role of ions. Biophys J 95: 5489-5495. https://doi.org/10.1529/biophysj.108.131813 ![]() |
[20] | Eisenberg R (2023) Kirchhoff coupling generates ATP, the chemical energy of life. Qeios . https://doi.org/10.32388/C8DYYK |
[21] |
Blanchard DC, Syzdek LD (1970) Mechanism for the water-to-air transfer and concentration of bacteria. Science 170: 626-628. ![]() |
[22] |
Marks R, Kruczalak K, Jankowska K, et al. (2001) Bacteria and Fungi in air over the Gulf of Gdańsk and Baltic Sea. J Aerosol Sci 32: 43-56. https://doi.org/10.1016/S0021-8502(00)00064-1 ![]() |
[23] |
Aller JY, Kuznetsova MR, Jahns C, et al. (2005) The sea surface microlayer as a source of viral and bacterial enrichment in marine aerosol. J Aerosol Sci 36: 801-812. https://doi/10.1016/j.jaerosci.2004.10.012 ![]() |
[24] |
Michaud JM, Thompson LR, Kaul D, et al. (2018) Taxon-specific aerosolization of bacteria and viruses in an experimental ocean-atmosphere mesocosm. Nat Commun 9: 2017. https://doi.org/10.1038/s41467-018-04409-z ![]() |
[25] |
Reche I. D'Orta G, D'Orta G, Mladenov N, et al. (2018) Deposition rates of viruses and bacteria above the atmospheric boundary layer. ISME J 12: 1154-1162. https://doi.org/10.1038/s41396-017-0042-4 ![]() |
[26] |
Pawłowski PH (2021) Charged amino acids may promote coronavirus SARS-CoV-2 fusion with the host cell. AIMS Biophys 8: 111-120. https://doi.org/10.3934/biophys.2021008 ![]() |
[27] | Xu K (2013) DNA circuit system and charge transfer mechanism. Engineering 5: 382-385. https://doi.org/10.4236/eng.2013.510B077 |
[28] | Roberts GCK (2013) UV absorbance spectroscopy of biological macromolecules. Encyclopedia of Biophysics : 1-5. https://doi.org/10.1007/978-3-642-16712-6_780 |
[29] |
Chou PJ, Johnson WC (1993) Base inclinations in natural and synthetic DNAs. J Am Chem Soc 115: 1205-1214. https://doi.org/10.1021/ja00057a001 ![]() |
[30] |
Cherstvy AG, Kornyshev AA (2005) DNA melting in aggregates: Impeded or facilitated?. J Phys Chem B 109: 13024-13029. https://doi.org/10.1021/jp051117i ![]() |
[31] |
Cherstvy AG (2005) Structure of DNA toroids and electrostatic attraction of DNA duplexes. J Phys Condens Matter 17: 1363. https://doi.org/10.1088/0953-8984/17/8/015 ![]() |
[32] |
Baldwin GS, Brooks NJ, Robson RE, et al. (2008) DNA double helices recognize mutual sequence homology in a protein free environment. J Phys Chem B 112: 1060-1064. https://doi.org/10.1021/jp7112297 ![]() |
[33] |
Kornyshev AA, Lee DJ, Wynveen A (2017) Theory of phase segregation in DNA assemblies containing two different base-pair sequence types. New J Phys 19: 015014. https://doi.org/10.1088/1367-2630/aa5482 ![]() |
[34] |
Dominic J (2018) Introducing a model of pairing based on base pair specific interactions between identical DNA sequences. J Phys Condens Matter 30: 075102. https://doi.org/10.1088/1361-648X/aaa043 ![]() |
[35] |
Gladyshev E, Kleckner N (2018) Recombination-independent recognition of DNA homology for repeat-induced point mutation (RIP) is modulated by the underlying nucleotide sequence. PLoS Genet 12: e1006015. https://doi.org/10.1371/journal.pgen.1006015 ![]() |