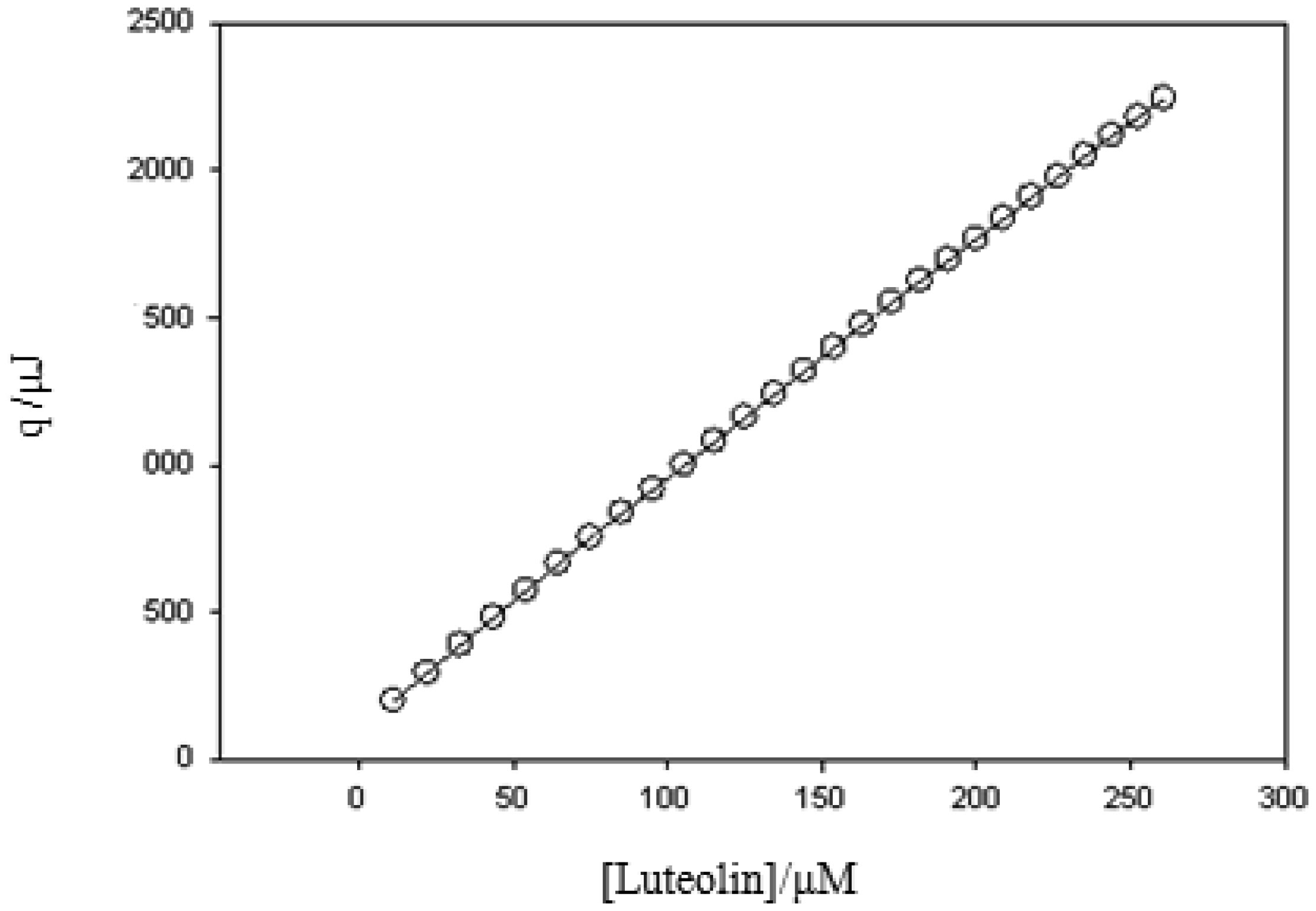
Citation: Maryam Mazinani, Gholamreza Rezaei Behbehani, Nematollah Gheibi, Alireza Farasat. Study of jack bean urease interaction with luteolin by the extended solvation model and docking simulation[J]. AIMS Biophysics, 2020, 7(4): 429-435. doi: 10.3934/biophy.2020029
[1] | Keleabetswe L. Mpye, Samantha Gildenhuys, Salerwe Mosebi . The effects of temperature on streptavidin-biotin binding using affinity isothermal titration calorimetry. AIMS Biophysics, 2020, 7(4): 236-247. doi: 10.3934/biophy.2020018 |
[2] | Auwal Muhammad, Kanikar Muangchoo, Ibrahim A. Muhammad, Ya'u S. Ajingi, Aliyu M. Bello, Ibrahim Y. Muhammad, Tasi'u A. Mika'il, Rakiya Aliyu . A molecular modeling study of novel aldose reductase (AR) inhibitors. AIMS Biophysics, 2020, 7(4): 380-392. doi: 10.3934/biophy.2020026 |
[3] | Tatyana Tretyakova, Maya Makharadze, Sopio Uchaneishvili, Mikhael Shushanyan, Dimitri Khoshtariya . Exploring the role of preferential solvation in the stability of globular proteins through the study of ovalbumin interaction with organic additives. AIMS Biophysics, 2023, 10(4): 440-452. doi: 10.3934/biophy.2023025 |
[4] | Arjun Acharya, Madan Khanal, Rajesh Maharjan, Kalpana Gyawali, Bhoj Raj Luitel, Rameshwar Adhikari, Deependra Das Mulmi, Tika Ram Lamichhane, Hari Prasad Lamichhane . Quantum chemical calculations on calcium oxalate and dolichin A and their binding efficacy to lactoferrin: An in silico study using DFT, molecular docking, and molecular dynamics simulations. AIMS Biophysics, 2024, 11(2): 142-165. doi: 10.3934/biophy.2024010 |
[5] | Morteza Vaezi, G. Rezaei Behbehani, Nematollah Gheibi, Alireza Farasat . Thermodynamic, kinetic and docking studies of some unsaturated fatty acids-quercetin derivatives as inhibitors of mushroom tyrosinase. AIMS Biophysics, 2020, 7(4): 393-410. doi: 10.3934/biophy.2020027 |
[6] | Thuy Hien Nguyen, Catherine C. Moore, Preston B. Moore, Zhiwei Liu . Molecular dynamics study of homo-oligomeric ion channels: Structures of the surrounding lipids and dynamics of water movement. AIMS Biophysics, 2018, 5(1): 50-76. doi: 10.3934/biophy.2018.1.50 |
[7] | Iva Valkova, Petar Todorov, Victoria Vitkova . VV-hemorphin-5 association to lipid bilayers and alterations of membrane bending rigidity. AIMS Biophysics, 2022, 9(4): 294-307. doi: 10.3934/biophy.2022025 |
[8] | Raghvendra P Singh, Guillaume Brysbaert, Marc F Lensink, Fabrizio Cleri, Ralf Blossey . Kinetic proofreading of chromatin remodeling: from gene activation to gene repression and back. AIMS Biophysics, 2015, 2(4): 398-411. doi: 10.3934/biophy.2015.4.398 |
[9] | Abdulrahaman Mahmoud Dogara, Ateeq Ahmed Al-Zahrani, Sarwan W. Bradosty, Saber W. Hamad, Shorsh Hussein Bapir, Talar K. Anwar . Antioxidant, α-glucosidase, antimicrobial activities chemical composition and in silico analysis of eucalyptus camaldulensis dehnh. AIMS Biophysics, 2024, 11(3): 255-280. doi: 10.3934/biophy.2024015 |
[10] | Chia-Wen Wang, Oscar K. Lee, Wolfgang B. Fischer . Screening coronavirus and human proteins for sialic acid binding sites using a docking approach. AIMS Biophysics, 2021, 8(3): 248-263. doi: 10.3934/biophy.2021019 |
The question of finding effective and useful inhibitors of urease enzyme has been in the interest of researchers for many years. JBU is a urea amidohydrolase, this metalloenzyme contains two nickel ions per subunit and is widely found in soil, plants, and microscopic organisms like bacteria, algae, fungi, and invertebrates [1].
Urease is responsible for the hydrolysis of urea to carbon dioxide and ammonia. The mechanism of urea hydrolysis is widely proposed by many researchers. The metal cations interact with hydroxyl ions, and the three molecules of water immerse in the active site of the enzyme [1]. Since urea contains two hydrogen bonding sites, one carbonyl group, and the other amino acid group, hydrogen bond formation plays an important role in the binding of urea to the two nickel atoms present in the active site [2]–[3]. Because the binding affinity of urea is not so strong, the bridging of urea with metal ions is stabilized by three amino acid residues His a222, His a232, and Ala a366, found in the active site [1]. The carbonyl group of urea is susceptible to the nucleophilic attack, after the nucleophilic attack of hydroxide ion on the electrophilic center of urea, urea changes to ammonia gas and carbamate then carbamate is changed rapidly into carbon dioxide and a second molecule of ammonia. The pH increases from 6.5 to 9.0 so, this catalytic activity in the human body paves the way for the survival of Helicobacter pylori in the stomach and which can cause gastritis, gastric, duodenal ulcers, and even gastric cancer [1].
Inhibitors of Jack Bean Urease can block the hydrolysis of urea to ammonium and decrease ammonia. Moreover, they can play an effective role in the prevention of disorders related to the urinary tract and stomach, therefor finding novel urease inhibitors with better stability and fewer side effects can improve the level of human life [4]–[6].
Luteolin (3, 4, 5, 7-tetrahydroxy flavone) is an anticancer agent against different types of human cancers. This flavonoid found in different plants such as vegetables, medicinal herbs, and fruits, and it can block cancer development in vitro and in vivo [5].
The purpose of this study because of the crucial role of urease in the creation of various diseases is to evaluate the function of Luteolin as a non-toxic and stable urease inhibitor. In the present work, the interaction of Luteolin with Jack Bean Urease and the binding parameters of Luteolin was investigated by the extended solvation model and Molecular docking. Therefore, the results indicate that urease becomes stable in the low and high concentration regions of Luteolin, as indicated by positive values of δA and δB (Table 1).
Jack bean urease (JBU; MW = 545.34 kDa) and Luteolin were purchased from Sigma (Sigma-Aldrich Co). The buffer used in the assay was 40 mM, pH = 7.0, which was obtained from Sigma Chemical Co. The isothermal titration micro calorimetric experiments were performed with the four-channel commercial micro calorimetric system, Thermal Activity Monitor 2277, Thermometric, Sweden. The titration vessel was made from stainless steel. Luteolin (10 mM) was injected by the use of a Hamilton syringe into the calorimetric titration vessel, which contained 1.8 mL JBU (4 µM). Injection of Luteolin solution into the perfusion vessel was repeated 30 times, the first injection was 5 µL and the remaining ones were 10 µL. In all cases, each injection was done in 6 s at 3-min intervals. The measurements were performed under a constant temperature of 27.0 ± 0.02 ºC and the temperature was controlled using a Poly-Science water bath. The calorimetric signal was measured by a digital voltmeter that was part of a computerized recording system. The ‘Thermometric Digit am’3’ software program calculated the heat of each injection. The heat of dilution of the Luteolin solution was measured as described above except JBU was excluded. Moreover, the micro calorimeter was frequently calibrated electrically during the study.
To determine the interaction of Luteolin with JBU, a molecular docking process was performed. The geometries of Luteolin was obtained from PubChem (https://pubchem.ncbi.nlm.nih.gov/), and the crystal structure of Jack Bean Urease was downloaded from the PDB bank (http://www.rcsb.org) with PDB ID 4H9M. Luteolin docking to Jack Bean Urease was performed with the Auto Dock Vina program [6]. Afterward, the docking process was performed via a grid size of 94 × 96 × 118 along the X, Y, and Z axes with 1 Å spacing. The lowermost binding energy of Luteolin and JBU was created using Auto Dock Vina. After docking, the interaction of Luteolin as an inhibitor with JBU was examined using LigPlot software [7].
The calorimetry results have been analyzed using Gholamreza Rezaei Behbehani's solvation model. The extended solvation model reported in the previously published articles shown that the heats of the biomolecules and ligands interactions in the aqueous solvent systems can be described by eq (1) [8]–[9].
Where the heats of Luteolin+urease interactions are q, and qmax shows the heat value upon saturation of all JBU. The δA and δB parameters reflect the net effect of Luteolin on the JBU structural changes in the low and high inhibitor concentrations, respectively. The positive values for δA and δB indicate that JBU stabilization by the ligands, while negative values of δA and δB show that Luteolin stabilized the JBU structure as a result of its interaction.
Cooperativity, in enzymology, a phenomenon in which the shape of one subunit of an enzyme is altered by the substrate or some other molecule. So cooperative binding requires macromolecule with more than one binding site
Fitting of the heats of JUB+Luteolin interactions was performed across the entire Luteolin. In the fitting procedure, p was changed until the best agreement between the experimental and calculated data was approached; a comparison between the experimental heats has been shown graphically in Figure 1. The binding parameters for JUB+Luteolin were reported in Table 1. The agreement between the experimental and theoretical calculation results proves the eq (1).
parameters | p | n | Ka/L.mol−1 | ΔH/kJ mol−1 | ΔG/kJ mol−1 | TΔS/kJ mol−1 | δA | δB |
1.16 | 16 | 139410 | 4.93 | -29.54 | 34.48 | 0.3 | 0.9 |
Indicated by the binding parameters, the interaction is entropy-driven indicating that the hydrophobic forces are dominant. δA and δB are very closed together, indicating so little changes in JBU structure as a result of its interaction with Luteolin. Small changes in δA and δB values is the characteristic of specific interaction and it is possible to conclude that the most of JBU is in its native state. Also, we can say that this interaction is a specific one because the affinity of the ligand for binding with JBU is low as indicated by the associated binding constant (Ka in Table 2). P > 1 as well as positive δA and δB, indicates that Luteolin causes a little stabilization of the JBU structure.
Consider a biomolecule, with n binding sites for ligands. The binding of the ligands to the biomolecule can be represented by the chemical equilibrium expression:
Where Ka (forward rate, or the rate of association of the protein-ligand complex). Assuming that
The number of Luteolin around urease, n, and association constant, Ka, were determined graphically based on eq (4).
Ka is the association equilibrium constant for Luteolin+urease binding and n values obtained from the eq 1, reported in Table 1. The Gibbs free energies of the interaction can be obtained as follows:
The low Ka values in the low Luteolin concentrations reflect the lower affinity of urease for biding to Luteolin in this domain as illustrated in Table 2. The n value increases in the high Luteolin indicate that the increase of the hydrophobic forces in the interaction, leading to the enhancement of water structure. The greater the extent of this enhancement, the greater the stabilization of the JBU structure (Figue 2 and 3).
n | KA/M−1 | △H/kJmol−1 | △G/kJmol−1 | T△S/kJmol−1 | |
Low concentration | 1.35 | 6.42 × 107 | 29.82 | -44.94 | 74.76 |
High concentration | 1.91 | 2.01 × 108 | 12.97 | -47.77 | 60.77 |
After docking, the interaction of Luteolin as an inhibitor with JBU was examined using LigPlot software. The energy binding of the interactions of Luteolin and JBU was −7.5 kJ/mol. The molecular docking study has shown that two residues: Glu718 and Lys716 were involved in a hydrogen bond with a length of 2.74 and 2.94 Å, respectively. Also, eight residues (Asp730, Val744, Thr33, Ala37, Val36, Phe712, Tyr32, and Lys709) were involved in the hydrophobic interaction Figure 4 shows the best-docked conformations of JBU and Luteolin. Comparing the results of molecular docking and the extended solvation model shows that the hydrophobic forces are the main force on the interactions of Luteolin and JBU.
The interaction of JBU with Luteolin was studied by the Isothermal Titration Calorimetry method and molecular docking. The results recovered from the extended solvation model and the agreement between the calculated and experimental results (Figure 1) is striking, and gives considerable support to the use of Equation 1. δA and δB values for Luteolin and JBU interactions are positive indicating that in the high concentration of the Luteolin, the JBU structure is stabilized also the positive values show that the JBU structure is stabilized by the addition of Luteolin. P > 1, as well as positive δA and δB, indicates that Luteolin causes a little stabilization of the JBU structure and the affinity of the binding sites for a ligand is increased upon the binding of a ligand to a binding site. Moreover, the molecular docking results indicate that Luteolin has binding potency and JBU structure is stabilized by Luteolin.
[1] | Kafarski P, Talma M (2018) Recent advances in design of new urease inhibitors: A review. J Adv Res 13: 101-112. |
[2] | Behbehani GR, Saboury AA, Taleshi E (2018) Determination of partial unfolding enthalpy for lysozyme upon interaction with dodecyltrimethylammonium bromide using an extended solvation model. J Mol Recogn: Interdisciplinary J 21: 132-135. |
[3] | Shalbafan M, Behbehani GR, Divsalar A (2018) The effect of methotrexate on the structure changes of human serum. Int J 74: 60-67. |
[4] | Jiang R, Poschet G, Owen R, et al. (2019) Serum concentration of genistein, luteolin and colorectal cancer prognosis. Nutrients 11: 600. |
[5] | Behbehani GR, Divsalar A, Saboury AA, et al. (2009) A thermodynamic study on the binding of PEG-stearic acid copolymer with lysozyme. J Solution Chem 38: 219. |
[6] | Trott O, Olson AJ (2010) AutoDock Vina: improving the speed and accuracy of docking with a new scoring function, efficient optimization, and multithreading. J Comput Chem 31: 455-461. |
[7] | Wallace AC, Laskowski RA, Thornton JM (1995) LIGPLOT: a program to generate schematic diagrams of protein-ligand interactions. Protein Eng, Des Sel 8: 127-134. |
[8] | Zolghadr L, Rezaei Behbehani G (2019) Thermodynamic study of comfarol binding to urease. Orbital: Electron J Chem 11: 151-154. |
[9] | Mohammadian M, Rezaei Behbehani G, Ghalami-Choobar B, et al. (2019) A thermodynamic study on the binding of polyethyleneglycol 1500 stearic acid with lysozyme. Orbital: Electron J Chem 11: 422-426. |
[10] | Payeghadr M, Rezaei Behbehani G, Shalbafan M (2017) Lanthanide chlorides decrease the antioxidant property of human serum albumin. Orbital: Electron J Chem 9: 72-75. |
[11] | Lemeski ET, Behbehani GR, Saboury AA, et al. (2011) Thermodynamic study of the binding of mercury ion to human growth hormone at different temperatures. J Solution Chem 40: 575-586. |
[12] | Poursoleiman A, Karimi-Jafari MH, Zolmajd-Haghighi Z, et al. (2019) Polymyxins interaction to the human serum albumin: A thermodynamic and computational study. Spectrochim Acta A 217: 155-163. |
[13] | Jamali Z, Rezaei Behbehani G, Zare K, et al. (2019) Effect of chrysin omega-3 and 6 fatty acid esters on mushroom tyrosinase activity, stability, and structure. J Food Biochem 43: e12728. |
1. | Leila Zolghadr, Gholamreza Rezaei Behbehani, Babak PakBin, Seied Ali Hosseini, Adeleh Divsalar, Nematollah Gheibi, Molecular dynamics simulations, molecular docking, and kinetics study of kaempferol interaction on Jack bean urease: Comparison of extended solvation model, 2022, 10, 2048-7177, 3585, 10.1002/fsn3.2956 |
parameters | p | n | Ka/L.mol−1 | ΔH/kJ mol−1 | ΔG/kJ mol−1 | TΔS/kJ mol−1 | δA | δB |
1.16 | 16 | 139410 | 4.93 | -29.54 | 34.48 | 0.3 | 0.9 |
n | KA/M−1 | △H/kJmol−1 | △G/kJmol−1 | T△S/kJmol−1 | |
Low concentration | 1.35 | 6.42 × 107 | 29.82 | -44.94 | 74.76 |
High concentration | 1.91 | 2.01 × 108 | 12.97 | -47.77 | 60.77 |