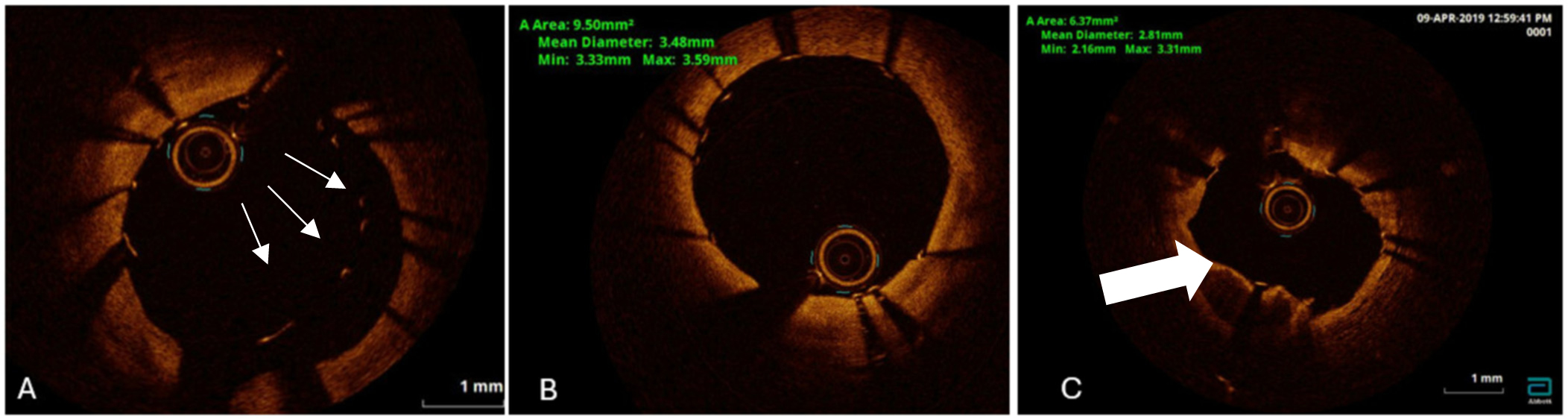
Calcified lesions pose significant challenges in cardiovascular interventions due to their complex nature and associated risks. These challenges range from accurate diagnosis to the modification of calcified areas using various techniques. Failing to adequately treat calcification can lead to suboptimal outcomes in coronary angioplasty, increasing the risk of complications such as stent thrombosis and in-stent restenosis. While various calcium modification techniques are available, they come with inherent risks, including vessel perforations and dissections. In this review article, we explored the difficulties associated with calcified lesions, categorizing them into access site issues, acute complications, and long-term complications. Understanding these challenges is important in improving patient outcomes.
Citation: Sophia Khattak, Farhan Shahid, Sohail Q. Khan. Challenges and risks associated with coronary calcified lesions in cardiovascular interventions: What makes calcified lesions more challenging and dangerous?[J]. AIMS Medical Science, 2025, 12(1): 69-89. doi: 10.3934/medsci.2025006
[1] | Ilana S. Golub, Angela Misic, Lucia P. Schroeder, Jairo Aldana-Bitar, Srikanth Krishnan, Sina Kianoush, Travis Benzing, Keishi Ichikawa, Matthew J. Budoff . Calcific coronary lesions: management, challenges, and a comprehensive review. AIMS Medical Science, 2024, 11(3): 292-317. doi: 10.3934/medsci.2024021 |
[2] | Nitin Chandra Mohan, Thomas W. Johnson . Role of intracoronary imaging in severely calcific disease. AIMS Medical Science, 2024, 11(4): 388-402. doi: 10.3934/medsci.2024027 |
[3] | Giulia Alagna, Alessia Cascone, Antonino Micari, Giancarlo Trimarchi, Francesca Campanella, Giovanni Taverna, Saro Pistorio, Giuseppe Andò . Type and duration of antithrombotic therapy after treatment of severely calcified lesions. AIMS Medical Science, 2025, 12(1): 38-62. doi: 10.3934/medsci.2025004 |
[4] | Bernardo Cortese, Davide Piraino, Eluisa La Franca, Dario Buccheri, Pedro Silva Orrego, Giuseppe Andolina, Romano Seregni . Coronary Stent Thrombosis in 2015: A Comprehensive and Uptodated Review. AIMS Medical Science, 2015, 2(3): 131-149. doi: 10.3934/medsci.2015.3.131 |
[5] | Marco Ferrone, Anna Franzone, Bruno Trimarco, Giovanni Esposito, Plinio Cirillo . Percutaneous Coronary Intervention in a Patient with Acute Thrombosis of Saphenous Vein Graft and Patent Native Coronary Artery: Which is the Vessel to Approach?. AIMS Medical Science, 2015, 2(4): 310-315. doi: 10.3934/medsci.2015.4.310 |
[6] | Todd T. Nowlen, Luis F. Goncalves, Susan S. Park, Arash A. Sabati . Adolescent Kawasaki Disease patient with coronary calcifications, severe 4-vessel stenosis, and a normal stress MRI. AIMS Medical Science, 2025, 12(1): 28-37. doi: 10.3934/medsci.2025003 |
[7] | Teruo Sekimoto, Takamasa Tanaka, Tatsuya Shiraki, Renu Virmani, Aloke V. Finn . How does atherosclerotic plaque become calcified, and why?. AIMS Medical Science, 2024, 11(4): 421-438. doi: 10.3934/medsci.2024029 |
[8] | Lorenzo Azzalini, Philippe L. L’Allier, Jean-François Tanguay . Bioresorbable Scaffolds: The Revolution in Coronary Stenting?. AIMS Medical Science, 2016, 3(1): 126-146. doi: 10.3934/medsci.2016.1.126 |
[9] | Margarida Pujol-López, Luis Ortega-Paz, Manel Garabito, Salvatore Brugaletta, Manel Sabaté, Ana Paula Dantas . miRNA Update: A Review Focus on Clinical Implications of miRNA in Vascular Remodeling. AIMS Medical Science, 2017, 4(1): 99-112. doi: 10.3934/medsci.2017.1.99 |
[10] | Valeria Paradies, Filippo Masi, Francesco Bartolomucci, Alfredo Marchese, Armando Liso, Fabrizio Resta, Stefano Favale, Martino Pepe . No Reflow-phenomenon: from Current State of the Art to Future Perspectives. AIMS Medical Science, 2015, 2(4): 374-395. doi: 10.3934/medsci.2015.4.374 |
Calcified lesions pose significant challenges in cardiovascular interventions due to their complex nature and associated risks. These challenges range from accurate diagnosis to the modification of calcified areas using various techniques. Failing to adequately treat calcification can lead to suboptimal outcomes in coronary angioplasty, increasing the risk of complications such as stent thrombosis and in-stent restenosis. While various calcium modification techniques are available, they come with inherent risks, including vessel perforations and dissections. In this review article, we explored the difficulties associated with calcified lesions, categorizing them into access site issues, acute complications, and long-term complications. Understanding these challenges is important in improving patient outcomes.
Coronary artery disease (CAD) remains the leading cause of cardiovascular death worldwide. Despite advancements in pharmacological therapies and percutaneous treatment techniques, the outcomes for patients with calcified coronary arteries continue to be one of the most challenging aspects of coronary intervention [1]. Calcified lesions not only add procedural complexity but also increase the risk of adverse outcomes such as vessel perforation, arterial dissection, and stent fracture under deployed stent, resulting in restenosis or stent thrombosis [2].
Calcified coronary artery disease is an increasingly encountered challenge in percutaneous coronary interventions (PCI), with studies indicating that moderate to severe coronary calcification is present in approximately 20–30% of patients undergoing PCI. This prevalence increases with age and comorbidities such as diabetes, chronic kidney disease, and hypertension [3],[4]. Coronary artery calcification has been identified as an independent predictor of all-cause mortality and myocardial infarction and is a need for repeat revascularization. Patients with severe calcification have a poor prognosis both in the short term (within one year) and long term [2], with a 3–10-fold higher risk of events compared to the general population [5]. Coronary calcification is a marker of atherosclerosis and a predictor of its progression [6]. Coronary artery calcification reduces the success rate of PCI by increasing the likelihood of stent malapposition and under-expansion (Figure 1). Studies indicate that stent malapposition occurs in up to 20% of cases involving calcified arteries, while under-expansion is observed in about 30% of these cases [7]. This then increases the risk of in-stent restenosis (ISR) reported in approximately 10–20% of patients with calcified lesions [8] compared to 5–10% in non-calcified lesions. Thrombosis rates are also elevated, occurring in about 2–4% of cases with significant calcification [9]. Calcified lesions are also linked to a higher incidence of procedural complications such as coronary perforation and dissections [10],[11]. The risk of coronary perforation is approximately 0.5–1.0% in calcified arteries compared to less than 0.2% in non-calcified arteries. Dissections occur more frequently as well, with an incidence of about 1–3% in calcified lesions [12].
We aim to elucidate the challenges and risks associated with calcified lesions in cardiovascular interventions, highlighting the complexities they introduce and the strategies employed to mitigate these risks. These challenges and risks can be broadly categorized into access site issues, acute complications during the procedure, and long-term complications.
Coronary artery calcification is a complex and multi-step process which begins with endothelial injury. This enables low-density lipoprotein (LDL) cholesterol to infiltrate the arterial wall by compromising the endothelial barrier. The LDL in the subendothelial space triggers an inflammatory response and leads to monocyte recruitment from the bloodstream to the injury site. These monocytes become foam cells by engulfing LDL and form fatty streaks on the arterial walls. These fatty streaks evolve into more complex atherosclerotic plaques and are stabilised by vascular smooth muscle cells (VSMCs). The VSMCs also undergo osteogenic differentiation under the influence of inflammatory cytokines, oxidative stress and growth factors. These osteogenic VSMCs serve an important role in calcium phosphate crystal formation resulting in microcalcification within the plaques. Over time, microcalcification grows into larger calcium deposits, increasing arterial rigidity and decreasing vascular compliance, contributing to clinical complications associated with coronary calcification [13],[14].
Calcific plaque can be eccentric or concentric. Eccentric plaque is <270° of the vessel lumen circumference, whereas concentric lesion is cross-sectional >270° of the vessel circumference. Understanding this phenotype is crucial in dictating how lesions may respond to balloon inflation during percutaneous coronary interventions (PCI). For example, in eccentric calcification, balloon dilation results in some luminal gain by stretching and disrupting the non-calcified parts of the vessel wall. This process can sometimes cause dissections at the edges of the calcium wall. Conversely, in concentric calcification, balloon dilation fractures in the thinner and weaker areas of the calcium [15].
Coronary angiography on its own is not adequately sensitive in identifying the amount and depth of coronary calcium. Severe calcification appears radio-opaque without cardiac motion, double track visible on both sides of the cardiac lumen (Figure 2). The most accurate method to determine the extent of calcification is intravascular ultrasound (IVUS). It has a high sensitivity and specificity of 90% and 99%, respectively [16]. Coronary calcification on IVUS appears as a hyperechogenic mass with acoustic shadowing behind it [17]. Optical Coherence Tomography (OCT) has a higher resolution than IVUS but a lower penetration depth. On OCT, calcification appears to be well delineated, with low signal regions with sharp borders [18]. Both these parameters can differentiate calcification as concentric or eccentric and are used for procedure planning and help with selection of plaque modification strategies. Simplified scoring systems have been developed to help in assessing the need for advance lesion preparation (Table 1).
OCT based calcium score (0–4) [19] | IVUS based calcium score [20] |
Calcium arc: 1. 90–180° (1 point) 2. >180° (2 points) |
Presence of calcified nodule (1 point) |
Presence of 360° circumferential calcium (1 point) | |
Calcium length >5 mm (1 point) | Calcium 270° in >5 mm length (1 point) |
Calcium thickness of >0.5 mm (1 point) | Vessel diameter of <3.5 mm (1 point) |
Calcium nodules are one of the most difficult to manage in PCI. They can be categorized into two subtypes: Eruptive and non-eruptive, each with unique histopathological and prognostic characteristics [21]. Eruptive calcium nodules are associated with worse long-term outcomes possibly due to a high risk of re-protrusion [22]. Intravascular imaging can help distinguish these two subtypes, leading to better treatment strategies for improved patient outcomes [21]. IVUS images showing calcium nodules are shown in Figures 3 and 4.
Calcified lesions cause significant challenges in achieving optimal stent expansion, which is crucial in predicting adverse outcomes, such as restenosis and stent thrombosis. A direct association has been shown between high CAC scores and reduced spent expansion due to rigidity of calcified plaques impeding full stent deployment [23]. Achieving optimal stent expansion, quantified by minimum stent area (MSA), is essential as inadequate stent expansion and a significant predictor of restenosis and stent thrombosis. Suboptimal MSA, resulting from incomplete stent deployment, can lead to turbulent blood flow in the coronary arteries and, with increased sheer stress, promote thrombus formation and neointimal hyperplasia [24]. Higher levels of coronary calcification can lead to delayed of impaired healing due to rigidity and uneven structures of the calcified plaques. The suboptimal stent deployment can leave areas of the vessel exposed, increasing the risk of incomplete endothelization. Poor endothelial healing increases the risk of thrombosis and restenosis, leading to poor long-term outcomes [25],[26].
Semi-compliant or non-compliant balloons can be used to prepare calcified lesions for further modification. It creates dissections in the media, fracturing thin calcium to increase plaque elasticity, facilitating stent expansion. The pressure exerted by the balloon against the vessel wall is uneven along the length of the lesion due to the thickness, location and distribution of calcium, thus increasing the risk of perforation or balloon rupture due to dog-bone deformation exerting excessive pressure at the edges [16].
Cutting balloons are non-compliant balloons with surgical blades attached to the surface. They are used in a 1:1 ratio with the vessel diameter and act by creating small calcium fractures. They have a low nominal pressure that minimises intimal trauma. They are a valuable adjunct in modifying calcified lesions but have a higher perforation rate than conventional balloons [27].
Scoring balloons are semi-compliant balloons that have nitinol wires, exerting a scoring effect on the plaque [28]. They are mechanistically similar to cutting balloons with comparable angiographic results [29]. Scoring balloons are more deliverable and are associated with less risk of vessel injury and coronary dissections. Scoring balloons may not be sufficient in achieving adequate lesion dilation as cutting balloons, necessitating the use of additional intervention. Although the risk is lower than cutting balloons, in extremely calcified lesions, there remains a risk of vessel wall damage and perforation, distal embolization of plaque fragments and vessel dissection. After successful dilation, the vessel may experience elastic recoil requiring adjunctive stenting to maintain vessel patency [30].
Ultra-high pressure balloons can inflate 35–40 atm, providing the necessary force to adequately dilate severely calcified lesions that standard balloons cannot effectively treat [31]. It applies a more uniform radial force along the length of the lesion, thus reducing the risk due to uneven pressure distribution. However, high inflation pressures can lead to dissections, especially at lesion edges, and may damage adjacent calcified tissue if calcification is asymmetrical [32].
Lithotripsy (Shockwave medical) consists of a semi-compliant balloon with two emitters at the proximal and distal levels. When the balloon inflates at a nominal pressure of 4 atm, the system is activated, and the emitters vaporize the saline/contrast solution inside the balloon. This process creates microbubbles that expand and burst producing shockwaves that travel through the coronary wall and fracture calcium deposits in the artery with minimal effect on the adventitia [33]. Generally considered a safe and effective treatment for calcified lesions, the balloon can, however, rupture in heavily calcified vessels, potentially causing vessel perforation [34]. Additionally, vessel dissection may also occur, particularly at the fibrocalcific interface of the vessel where eccentric calcium is present, as the balloon dilation can bias away from the calcified areas, increasing the risk of tearing [35].
RA with a rotablator RA system (Boston Scientific) is used to treat heavily calcified lesions that are resistant to conventional balloon angioplasty (under-expansion of the balloon). RA functions on the principle of plaque modification via rotational ablation. It uses a diamond-coated elliptical burr rotating at a speed between 140,000 and 180,000 rpm, driven by a helical driveshaft. This burr is advanced across the calcified lesion on a specialized rota guidewire. Multiple runs with the burr are often needed to smooth the vessel lumen and break up the intravascular calcium rings, enabling the subsequent passage, dilation of balloons, and stent implantation [36],[37]. This mechanical grinding action of RA can tear the arterial wall resulting in coronary artery dissection, which is more common in non-uniform calcification [38]. Debris generated by RA, especially from calcific nodules, can embolize downstream increasing the risk of no reflow phenomenon and potentially leading to periprocedural myocardial infarction [39]. An analysis of the British Cardiovascular Intervention Society (BCIS) database from 2006 to 2016 revealed that rotational atherectomy is linked to a higher rate of in-hospital major adverse cardiac or cerebrovascular events. This underscores the challenges of treating calcified lesions and highlights the need for strategies to mitigate these risks [40].
OA uses an eccentrically mounted diamond-coated crown that orbits within the vessel, sanding down the calcium plaque but preserving the soft vessel wall. It has a dual mechanism of action (centrifugal and orbital motion) that enables a more uniform plaque modification. This is particularly useful in eccentric lesions [36]. Atherectomy devices (both RA and OA), due to their high rotational speed, can penetrate the vessel walls, causing vessel perforation.
Laser atherectomy, such as Excimer Laser Coronary Atherectomy (ELCA), uses high ultraviolet light to vaporize calcified and non-calcified plaque. It operates through three mechanisms: Molecular ablation, where ultraviolet light breaks molecular bonds within the plaque; photochemical/photothermal effects, which involve chemical bond disruption by laser energy; and photomechanical effects, where rapid vaporization creates gas bubbles that disrupt the plaque. ELCA's advantages include compatibility with any standard guidewire, minimal risk of entrapment, and the ability to protect bifurcations. Laser atherectomy does not rely on mechanical force, thus reducing the risk of vessel trauma and dissection [36],[41]. However, the high-energy laser pulses can weaken the vessel wall, particularly in areas of non-uniform calcification, increasing the risk of dissections. In calcified regions, the energy may disperse unevenly, generating heat that may lead to thermal energy. The heat can damage adjacent non-calcified segments, posing additional risk to vessel integrity. However, this risk has been mitigated with the use of pulsed UV laser catheters allowing more energy delivery [42],[43].
Despite the availability of various treatments, the adoption of atherectomy techniques remains limited. This is primarily due to restricted access, insufficient familiarity and inadequate training, all of which hinder the utilization of these technologies and contribute to inadequate treatment of coronary calcification [44].
Medical therapy, such as statins, plays an important role in stabilizing and reducing the progression of calcific plaques in coronary arteries [45]. Furthermore, aggressive lipid-lowering therapy has shown improved cardiovascular outcomes, particularly in high-risk populations [46]. A high coronary artery calcium score is strongly linked to an increased risk of myocardial infarction (MI), making risk stratification crucial. In patients with elevated CAC scores, the use of antiplatelet therapy, such as aspirin, may help reduce the incidence of MI [47]. Additionally, emerging evidence suggests that CAC scoring could guide personalised preventive strategies, including antihypertensive and lipid-lowering therapies, to optimize cardiovascular outcomes [45],[47]. Furthermore, better diabetic control is a crucial aspect of medical therapy for managing coronary calcification. Elevated leptin levels in diabetic patients drive atherosclerotic processes, including vascular wall calcification, oxidative stress, and smooth muscle proliferation. Improved diabetic control may reduce leptin-driven calcification, representing an important target in coronary calcification management [48].
Complications associated with coronary calcification are detailed below:
The treatment of complex calcified coronary lesions requires meticulous planning and anticipation of potential challenges. Several key aspects must be considered when selecting the guiding catheter and vascular access. These include system stability during the procedure, sufficient internal diameter for the chosen calcium modification technique, e.g., burr size in RA, the operator experience, the risk of bleeding from the access site, and vascular complications [49].
Radial artery calcification (RC) is frequently associated with calcified coronary plaques. In one study, researchers found a significant correlation between RC and coronary calcifications in adults with angina symptoms and associated risk factors, with patients requiring a higher rate of revascularization treatments. RC can make arterial puncture and catheter manipulation difficult. With increased rigidity due to calcium, the risk of trauma during access increases, thus leading to bleeding complications and haematoma formation [50].
Although radial access is preferable over femoral access due to reduced bleeding-associated complications, femoral access may be preferred in cases where guiding support is suboptimal, and there is a need to use a bigger-sized rota burr [51],[52]. Ultrasound-guided puncture offers several benefits such as anatomical visualization, identification and avoidance of calcification and minimized complications such as haematoma formation and pseudoaneurysms.
In cardiovascular interventions, vascular closure devices (VCD) are utilized to achieve haemostasis at the arterial access site. The presence of calcification can result in inadequate deployment of the Angio-Seal device (commonly used VCD in femoral access percutaneous intervention) and result in persistent bleeding, haematomas, or pseudoaneurysm formation [53].
Arteriovenous (AV) fistula formation occurs when there is an abnormal connection between an artery and a vein. In calcified vessels, the increased rigidity and decreased elasticity make the arterial walls more susceptible to injury during percutaneous interventions. The use of radial access (over femoral) and under ultrasound guidance reduces complication rates improves the security of vascular access and avoids AV fistula formation [54].
The rigidity and irregular structure of the calcified lesions make stent delivery challenging, particularly in tortuous and severely calcified vessels. Inadequate vessel preparation prior to stent delivery can prevent the stent from advancing the lesion, causing deformation. Forcefully retracting the stent into the guiding catheter in such cases may lead to stent dislodgment, an uncommon yet life-threatening PCI complication [55],[56]. Stent loss is managed non-surgically by either retrieval using a small balloon technique and equipment such as a goose neck snare or crushing the deployed stent [57].
Coronary perforation occurs due to the calcified segments rupturing the vessel wall. In coronary calcification, the vessel walls lose their elasticity and flexibility. Modification of calcified plaque with high pressure balloon or atherectomy devices can create hard or sharp edges of the calcified plaque that can then puncture the vessel wall. This can lead to life-threatening complications such as cardiac tamponade. Management strategies include prolonged balloon inflation to seal the perforation, deployment of covered stents and pericardiocentesis (Figure 2).
There are three major coronary artery perforations depending on the location within the vessel. These include (a) large vessel perforation, (b) distal vessel perforation, and (c) collateral perforation. The management strategy for each type depends on multiple factors, including the size of the vessel, the anatomical location of the perforation, and the clinical scenario.
Large vessel perforations are more likely to occur in calcified vessels, especially when oversized balloons and stents are used in inadequately modified calcific lesions. They can also be caused using atherectomy devices, including rotational, orbital, and laser atherectomy [58]. Large vessel perforations are usually managed with prolonged balloon inflation and conventional/covered stents. Distal vessel perforation is usually caused by distal wire migration, especially with hydrophilic wires. Calcified lesions are more challenging to cross with standard workhorse wires, leading to greater reliance on hydrophilic options [58],[59]. Epicardial vessel perforation usually occurs in retrograde CTO PCI [58]. Distal and collateral vessel perforations, depending on the vessel size and location, may be treated using the embolization technique utilizing micro-coils, fat, collagen, and microspheres. If embolization is not feasible, a covered stent may be used to manage distal perforation by blocking the origin of the perforated side branch [58],[60],[61].
Coronary artery perforation can be classified using five types according to its severity [12]. The classification helps guide the management of the complication (Table 2).
Description | Management | |
Type I | A perforation with extraluminal crater without extravasation | This is the least severe type and typically does not cause significant bleeding. It can be managed conservatively but may require balloon tamponade or covered stents. |
Type II | A perforation with pericardial or myocardial blushing but no jet of contrast extravasation | Balloon tamponade, covered stents/coil embolization. The patient may require pericardiocentesis |
Type III | A perforation with extravasation through frank (>1 mm) perforation | These are severe types with substantial bleeding into the pericardial space and surrounding pericardium. It requires immediate pericardiocentesis and treatment with covered stents/coil embolization. |
Type IV (Cavity spilling) | Perforation into an anatomical cavity, chamber, or a vessel. | Because the blood leaks into another cardiovascular cavity, symptoms are usually of a new shunt. Type IV tends to be better tolerated than Type III. Management is similar to type III. |
Type V | Perforation of the distal segment | May require embolization of the distal vessel with thrombin, coils or fat. |
Stent thrombosis causes abrupt closure of the stented coronary artery and, therefore, carries a high risk of myocardial infarction and death. Stent thrombosis is influenced by various factors related to patients, procedural techniques, and stent characteristics. Another key factor influencing stent thrombosis is the degree of calcium in the coronary artery. Specifically, the arc, length, and thickness of calcification can impact the deployment and expansion of the stent. Suboptimal stent expansion, leading to under-deployment, is a significant predictor of stent thrombosis due to inadequate stent apposition and coverage of the diseased vessel wall [63].
Stent thrombosis is classified based on the level of certainty and timings of the event after the index procedure with stent implantation (Table 3).
Definition | |
Timing | Acute stent thrombosis—occurs within 24 hours of stent implantation |
Subacute stent thrombosis—occurs between 24 hours and 30 days of stent implantation | |
Late stent thrombosis—occurs between 30 days and 1 year post stent implantation | |
Very late stent thrombosis—occurs more than one year after stent implantation | |
Level of certainty | Definite—angiographic or pathological confirmation of stent thrombosis |
Probable—unexplained death within 30 days or target vessel myocardial infarction without angiographic confirmation of stent thrombosis | |
Possible—unexplained death after 30 days |
Coronary artery disease with severe calcified lesions is more likely to receive suboptimal revascularisation without the use of intracoronary imaging. Unfortunately, the utilization of intracoronary imaging is often limited, and visualization of coronary calcium is not as sensitive on angiography alone. Incomplete lesion preparation, improper stent sizing, and stent under expansion can all contribute to stent thrombosis or restenosis (Figure 5) [2],[66]. Minimum stent area (MSA) is a critical predictor of stent failure and can be adversely affected by under-expansion in calcified vessels [67].
Emergency PCI is indicated in acute presentation and is treated with balloon inflation, aspiration thrombectomy and pharmacological treatment with antiplatelets and anticoagulants. Further treatment with drug-eluting stent implantation may be required if it is due to edge dissection, which is more common in calcified lesions [68].
Various large trials and registries have identified several factors associated with stent thrombosis. For instance, the Champion-Phoenix trial highlighted that factors like presenting with non-ST-elevation myocardial infarction (NSTEMI) or ST-elevation myocardial infarction (STEMI), high angiographic thrombus burden, and longer stent length independently predict acute stent thrombosis [69]. Additionally, an IVUS study from the Horizon AMI trial revealed that factors such as stent area less than 5 mm2, improper positioning of stent struts, plaque protrusion or prolapse, edge dissection, and residual narrowing significantly contribute to early stent thrombosis [70]. Moreover, bifurcation stenting was also linked to increased stent thrombosis risk. Other researchers found a higher risk of stent thrombosis in patients presenting with STEMI, irrespective of the stent type. The use of more potent antiplatelet drugs, such as Prasugrel, has shown to halve this risk [71]. Importantly, coronary calcification can act as a central contributor to these risks by creating a challenging environment for stent deployment, leading to incomplete stent expansion, improper apposition, and edge dissection, which are critical drivers of stent thrombosis.
Stent malapposition refers to the separation of any stent strut from the intimal surface of the arterial wall in the absence of bifurcation. On IVUS, its prevalence is reported to be approximately 13% with an OCT around 51%. One cause of acute stent malapposition is when the stent is deployed in the region with a shift from non-calcified to calcified plaque, especially with the presence of a calcified nodule. The expanded stent has difficulty adapting to sudden changes in vessel lumen geometry [72]. Severely calcified lesions can prevent stents from expanding uniformly. As a result, areas with calcification may cause the stent to expand unevenly and not fully adhere to the vessel wall nearby. This can happen because the balloon used during the procedure may not apply enough pressure or the stiffness of the stent itself makes it difficult to conform perfectly to the vessel's contours in those areas.
Malapposition can disrupt the endothelialization process of the vessel wall and promote the proliferation of smooth muscle cells around the stent struts. This can contribute to in-stent restenosis described below. It can also compromise drug penetration and distribution from the drug-eluting stents, reducing the efficacy of the anti-proliferative agents and increasing the risk of restenosis. Malapposition creates gaps between the stent struts and the vessel wall, which poses a potential risk of guidewires going behind the stent during the same or subsequent procedures. If the malapposed stent is placed ostially, this can lead to stent thrombosis. Stent malapposition is difficult to detect on standalone angiography, and intracoronary imaging is often required for acute diagnosis and optimizing outcomes in patients undergoing coronary intervention [70].
Heavily calcified lesions have been associated with an increased risk of long-term complications such as in-stent restenosis and late target vessel revascularisation (TLR). The presence of an in-stent calcific nodule is a primary cause for TLR following PCI in patients with coronary calcification.
In-stent restenosis (ISR) is characterized by >50% narrowing within the stent on repeat coronary angiography [73]. ISR is primarily caused by neointimal hyperplasia, where smooth muscle cells proliferate within the stent, resulting in the re-narrowing of the treated artery. This process is a critical factor contributing to the recurrence of symptoms and the need for additional interventions [74].
Several risk factors influence the development of restenosis. These include underlying comorbidities such as diabetes mellitus and smoking, which exacerbate the vascular response to injury. Procedural factors also play a significant role, including the type of stent used and the technique employed during deployment [74].
Clinical manifestations of restenosis often present as recurrent angina or myocardial infarction, necessitating further revascularisation procedures. Treatment strategies typically involve interventions like balloon angioplasty or the use of drug-eluting stents, aimed at improving luminal patency and relieving symptoms [74].
In calcified coronary arteries, ISR results from the stent's inability to fully expand during stent deployment due to underlying calcification. Furthermore, neoatherosclerosis within the stent further contributes to the stenosis [73]. ISRs are associated with a high risk of recurrent target lesion revascularization (Figures 6 and 7).
When a calcified coronary artery is not well prepared prior to stent deployment, it significantly increases the risk of stent fracture. The stent may not expand uniformly, exerting excessive pressure on the calcified areas. This uneven pressure can lead to mechanical fatigue and stent fracture. Stent fracture can result in in-stent restenosis and stent thrombosis [75].
To address the challenges associated with calcified coronary lesions, future research and clinical guidelines should include developing and evaluating forward-facing devices, like the shockwave IVL system. Evaluating these devices in trials will help determine their safety and utility in high-risk cases.
Expanding research on combination therapy such as conducting head-to-head trials comparing IVL and orbital atherectomy in patients, especially with calcified nodules would provide insights into the efficacy and safety profiles of combining these modalities and guide optimal treatment strategy.
Investigating the role of medications to reduce coronary artery calcification progression, such as anti-inflammatory agents or novel lipid-lowering agents as adjunctive therapy, may improve overall outcomes in these patients.
The management of calcified lesions in cardiovascular interventions remains complex with significant challenges and risks leading to procedural complications such as perforation, dissection, stent thrombosis, and suboptimal stent expansion and apposition. Intracoronary imaging is important in procedure planning as it enables the assessment of calcification and guide selection of devices and techniques. Lesion preparation with balloon-based or atherectomy techniques enhances the success of PCI in patients with severe coronary calcification. However, this remains a challenging aspect of interventional cardiology with further work and innovative techniques required to optimize long-term patient outcomes.
Sophia Khattak: drafted the original manuscript; Farhan Shahid and Sohail Q. Khan: reviewed and revised the manuscript.
The authors declare they have not used Artificial Intelligence (AI) tools in the creation of this article.
[1] |
Caiazzo G, Di Mario C, Kedhi E, et al. (2023) Current management of highly calcified coronary lesions: an overview of the current status. J Clin Med 12: 4844. https://doi.org/10.3390/jcm12144844 ![]() |
[2] |
Bourantas CV, Zhang YJ, Garg S, et al. (2014) Prognostic implications of coronary calcification in patients with obstructive coronary artery disease treated by percutaneous coronary intervention: a patient-level pooled analysis of 7 contemporary stent trials. Heart 100: 1158-1164. https://doi.org/10.1136/heartjnl-2013-305180 ![]() |
[3] |
Kedhi E, Berta B, Roleder T, et al. (2021) Thin-cap fibroatheroma predicts clinical events in diabetic patients with normal fractional flow reserve: the COMBINE OCT-FFR trial. Eur Heart J 42: 4671-4679. https://doi.org/10.1093/eurheartj/ehab433 ![]() |
[4] |
Protty M, Sharp ASP, Gallagher S, et al. (2022) Defining percutaneous coronary intervention complexity and risk: an analysis of the United Kingdom BCIS database 2006–2016. JACC Cardiovasc Interv 15: 39-49. https://doi.org/10.1016/j.jcin.2021.09.039 ![]() |
[5] |
Budoff MJ, Shaw LJ, Liu ST, et al. (2007) Long-term prognosis associated with coronary calcification. Observations from a registry of 25253 patients. J Am Coll Cardiol 49: 1860-1870. https://doi.org/10.1016/j.jacc.2006.10.079 ![]() |
[6] |
Folsom AR, Kronmal RA, Detrano RC, et al. (2008) Coronary artery calcification compared with carotid intima-media thickness in the prediction of cardiovascular disease incidence: the Multi-Ethnic Study of Atherosclerosis (MESA). Arch Intern Med 168: 1333-1339. https://doi.org/10.1001/archinte.168.12.1333 ![]() |
[7] |
Moussa I, Di Mario C, Moses J, et al. (1997) Coronary stenting after rotational atherectomy in calcified and complex lesions. Angiographic and clinical follow-up results. Circulation 96: 128-136. https://doi.org/10.1161/01.cir.96.1.128 ![]() |
[8] |
Alfonso F, Kastrati A (2021) Clinical burden and implications of coronary interventions for in-stent restenosis. EuroIntervention 17: e355-e357. https://doi.org/10.4244/EIJV17I5A60 ![]() |
[9] |
Giustino G, Mastoris I, Baber U, et al. (2016) Correlates and impact of coronary artery calcifications in women undergoing percutaneous coronary intervention with drug-eluting stents: from the Women in Innovation and Drug-Eluting Stents (WIN-DES) collaboration. JACC Cardiovasc Interv 9: 1890-1901. https://doi.org/10.1016/j.jcin.2016.06.022 ![]() |
[10] |
Cialdella P, Sergi SC, Zimbardo G, et al. (2023) Calcified coronary lesions. Eur Heart J Suppl 25: C68-C73. https://doi.org/10.1093/eurheartjsupp/suad009 ![]() |
[11] |
Guedeney P, Claessen BE, Mehran R, et al. (2020) Coronary calcification and long-term outcomes according to drug-eluting stent generation. JACC Cardiovasc Interv 13: 1417-1428. https://doi.org/10.1016/j.jcin.2020.03.053 ![]() |
[12] |
Ellis SG, Ajluni S, Arnold AZ, et al. (1994) Increased coronary perforation in the new device era: incidence, classification, management, and outcome. Circulation 90: 2725-2730. https://doi.org/10.1161/01.cir.90.6.2725 ![]() |
[13] |
Virmani R, Kolodgie FD, Burke AP, et al. (2005) Atherosclerotic plaque progression and vulnerability to rupture: angiogenesis as a source of intraplaque hemorrhage. Arterioscler Thromb Vasc Biol 25: 2054-2061. https://doi.org/10.1161/01.ATV.0000178991.71605.18 ![]() |
[14] |
Aikawa E, Nahrendorf M, Figueiredo JL, et al. (2007) Osteogenesis associates with inflammation in early-stage atherosclerosis evaluated by molecular imaging in vivo. Circulation 116: 2841-2850. https://doi.org/10.1161/CIRCULATIONAHA.107.732867 ![]() |
[15] |
Jadhav KP, Kavalipatu KNR, Kuchulakanti PK, et al. (2021) Coronary artery calcification: from cell to stent—a review. Indian J Clin Cardiol 2: 97-109. https://doi.org/10.1177/26324636211013156 ![]() |
[16] |
Zaidan M, Alkhalil M, Alaswad K (2022) Calcium modification therapies in contemporary percutaneous coronary intervention. Curr Cardiol Rev 18: e281221199533. https://doi.org/10.2174/1573403X18666211228095457 ![]() |
[17] |
Mintz GS, Popma JJ, Pichard AD, et al. (1995) Patterns of calcification in coronary artery disease: a statistical analysis of intravascular ultrasound and coronary angiography in 1155 lesions. Circulation 91: 1959-1965. https://doi.org/10.1161/01.cir.91.7.1959 ![]() |
[18] |
Terashima M, Kaneda H, Suzuki T (2012) The role of optical coherence tomography in coronary intervention. Korean J Intern Med 27: 1-12. https://doi.org/10.3904/kjim.2012.27.1.1 ![]() |
[19] |
Fujino A, Mintz GS, Matsumura M, et al. (2018) A new optical coherence tomography-based calcium scoring system to predict stent underexpansion. EuroIntervention 13: e2182-e2189. https://doi.org/10.4244/EIJ-D-17-00962 ![]() |
[20] |
Zhang M, Matsumura M, Usui E, et al. (2021) Intravascular ultrasound-derived calcium score to predict stent expansion in severely calcified lesions. Circ Cardiovasc Interv 14: e010296. https://doi.org/10.1161/CIRCINTERVENTIONS.120.010296 ![]() |
[21] |
Watanabe Y, Sakakura K, Taniguchi Y, et al. (2020) Comparison of clinical outcomes of intravascular ultrasound-calcified nodule between percutaneous coronary intervention with versus without rotational atherectomy in a propensity-score matched analysis. PLoS One 15: e0241836. https://doi.org/10.1371/journal.pone.0241836 ![]() |
[22] |
Sato T, Matsumura M, Yamamoto K, et al. (2023) Impact of eruptive vs noneruptive calcified nodule morphology on acute and long-term outcomes after stenting. JACC Cardiovasc Interv 16: 1024-1035. https://doi.org/10.1016/j.jcin.2023.03.009 ![]() |
[23] |
Komaki S, Ishii M, Ikebe S, et al. (2021) Association between coronary artery calcium score and stent expansion in percutaneous coronary intervention. Int J Cardiol 334: 31-36. https://doi.org/10.1016/j.ijcard.2021.04.021 ![]() |
[24] | Kang SJ (2021) Intravascular ultrasound-derived criteria for optimal stent expansion and preprocedural prediction of stent underexpansion. Circ Cardiovasc Interv 14: e011374. https://doi.org/10.1161/CIRCINTERVENTIONS.121.011374 |
[25] |
Torngren K, Rylance R, Björk J, et al. (2020) Association of coronary calcium score with endothelial dysfunction and arterial stiffness. Atherosclerosis 313: 70-75. https://doi.org/10.1016/j.atherosclerosis.2020.09.022 ![]() |
[26] |
Byrne RA, Joner M, Kastrati A (2015) Stent thrombosis and restenosis: What have we learned and where are we going? the Andreas Grüntzig Lecture ESC 2014. Eur Heart J 36: 3320-3331. https://doi.org/10.1093/eurheartj/ehv511 ![]() |
[27] |
Mauri L, Bonan R, Weiner BH, et al. (2002) Cutting balloon angioplasty for the prevention of restenosis: results of the cutting balloon global randomized trial. Am J Cardiol 90: 1079-1083. https://doi.org/10.1016/s0002-9149(02)02773-x ![]() |
[28] |
Bonaventura K, Schwefer M, Yusof AKM, et al. (2020) Systematic scoring balloon lesion preparation for drug-coated balloon angioplasty in clinical routine: results of the PASSWORD observational study. Adv Ther 37: 2210-2223. https://doi.org/10.1007/s12325-020-01320-2 ![]() |
[29] | Leick J, Rheude T, Cassese S, et al. (2024) Comparison of angiographic result and long-term outcome in patients with in-stent restenosis treated with cutting balloon or with scoring balloon angioplasty. J Invasive Cardiol 36. https://doi.org/10.25270/jic/24.00070 |
[30] |
Angsubhakorn N, Kang N, Fearon C, et al. (2022) Contemporary management of severely calcified coronary lesions. J Pers Med 12: 1638. https://doi.org/10.3390/jpm12101638 ![]() |
[31] |
Secco GG, Ghione M, Mattesini A, et al. (2016) Very high-pressure dilatation for undilatable coronary lesions: indications and results with a new dedicated balloon. EuroIntervention 12: 359-365. https://doi.org/10.4244/EIJY15M06_04 ![]() |
[32] |
Secco GG, Buettner A, Parisi R, et al. (2019) Clinical experience with very high-pressure dilatation for resistant coronary lesions. Cardiovasc Revasc Med 20: 1083-1087. https://doi.org/10.1016/j.carrev.2019.02.026 ![]() |
[33] |
Hill JM, Kereiakes DJ, Shlofmitz RA, et al. (2020) Intravascular lithotripsy for treatment of severely calcified coronary artery disease. J Am Coll Cardiol 76: 2635-2646. https://doi.org/10.1016/j.jacc.2020.09.603 ![]() |
[34] |
Mastrangelo A, Monizzi G, Galli S, et al. (2022) Intravascular lithotripsy in calcified coronary lesions: a single-center experience in “real-world” patients. Front Cardiovasc Med 9: 829117. https://doi.org/10.3389/fcvm.2022.829117 ![]() |
[35] |
Ali ZA, Nef H, Escaned J, et al. (2019) Safety and effectiveness of coronary intravascular lithotripsy for treatment of severely calcified coronary stenoses: the disrupt CAD II study. Circ Cardiovasc Interv 12: e008434. https://doi.org/10.1161/CIRCINTERVENTIONS.119.008434 ![]() |
[36] | Riley RF, Patel MP, Abbott JD, et al. (2024) SCAI expert consensus statement on the management of calcified coronary lesions. J Soc Cardiovasc Angiogr Interv 3: 101259. https://doi.org/10.1016/j.jscai.2023.101259 |
[37] |
Khattak S, Sharma H, Khan SQ (2024) Atherectomy techniques: rotablation, orbital and laser. Interv Cardiol 19: e21. https://doi.org/10.15420/icr.2024.16 ![]() |
[38] |
Tomey MI, Kini AS, Sharma SK (2014) Current status of rotational atherectomy. JACC Cardiovasc Interv 7: 345-353. https://doi.org/10.1016/j.jcin.2013.12.196 ![]() |
[39] | Kobayashi N, Ito Y, Yamawaki M, et al. (2018) Distal embolization of coronary calcified nodule after rotational atherectomy. SAGE Open Med Case Rep 6. https://doi.org/10.1177/2050313X18799243 |
[40] |
Kinnaird T, Gallagher S, Sharp A, et al. (2021) Operator volumes and in-hospital outcomes: an analysis of 7740 rotational atherectomy procedures from the BCIS national database. JACC Cardiovasc Interv 14: 1423-1430. https://doi.org/10.1016/j.jcin.2021.04.034 ![]() |
[41] |
Jawad-Ul-Qamar M, Sharma H, Vetrugno V, et al. (2021) Contemporary use of excimer laser in percutaneous coronary intervention with indications, procedural characteristics, complications and outcomes in a university teaching hospital. Open Heart 8: e001522. https://doi.org/10.1136/openhrt-2020-001522 ![]() |
[42] |
Tsutsui RS, Sammour Y, Kalra A, et al. (2021) Excimer laser atherectomy in percutaneous coronary intervention: a contemporary review. Cardiovasc Revasc Med 25: 75-85. https://doi.org/10.1016/j.carrev.2020.10.016 ![]() |
[43] |
Egred M, Brilakis ES (2020) Excimer Laser Coronary Angioplasty (ELCA): fundamentals, mechanism of action, and clinical applications. J Invasive Cardiol 32: E27-E35. https://doi.org/10.25270/jic/19.00325 ![]() |
[44] | Ali ZA, Shin D, Barbato E (2024) Between a rock and a hard place: a consensus statement on the management of calcified coronary lesions. J Soc Cardiovasc Angiogr Interv 3: 101265. https://doi.org/10.1016/j.jscai.2023.101265 |
[45] |
Achenbach S, Ropers D, Pohle K, et al. (2002) Influence of lipid-lowering therapy on the progression of coronary artery calcification: a prospective evaluation. Circulation 106: 1077-1082. https://doi.org/10.1161/01.cir.0000027567.49283.ff ![]() |
[46] |
Schwartz GG, Steg PG, Szarek M, et al. (2018) Alirocumab and cardiovascular outcomes after acute coronary syndrome. N Engl J Med 379: 2097-2107. https://doi.org/10.1056/NEJMoa1801174 ![]() |
[47] |
Limpijankit T, Jongjirasiri S, Unwanatham N, et al. (2022) Causal relationship of coronary artery calcium on myocardial infarction and preventive effect of antiplatelet therapy. Front Cardiovasc Med 9: 871267. https://doi.org/10.3389/fcvm.2022.871267 ![]() |
[48] | Matheus ASDM, Tannus LRM, Cobas RA, et al. (2013) Impact of diabetes on cardiovascular disease: an update. Int J Hypertens 2013: 653789. https://doi.org/10.1155/2013/653789 |
[49] |
Allali A, Abdel-Wahab M, Elbasha K, et al. (2023) Rotational atherectomy of calcified coronary lesions: current practice and insights from two randomized trials. Clin Res Cardiol 112: 1143-1163. https://doi.org/10.1007/s00392-022-02013-2 ![]() |
[50] |
Achim A, Kákonyi K, Nagy F, et al. (2022) Radial artery calcification in predicting coronary calcification and atherosclerosis burden. Cardiol Res Pract 2022: 5108389. https://doi.org/10.1155/2022/5108389 ![]() |
[51] | Yin WH, Tseng CK, Tsao TP, et al. (2015) Transradial versus transfemoral rotablation for heavily calcified coronary lesions in contemporary drug-eluting stent era. J Geriatr Cardiol 12: 489-496. https://doi.org/10.11909/j.issn.1671-5411.2015.05.004 |
[52] |
Khan AA, Panchal HB, Zaidi SIM, et al. (2019) Safety and efficacy of radial versus femoral access for rotational atherectomy: a systematic review and meta-analysis. Cardiovasc Revasc Med 20: 241-247. https://doi.org/10.1016/j.carrev.2018.06.006 ![]() |
[53] | Saleem T, Baril DT (2023) Vascular Access Closure Devices. StatPearls Publishing. Available from: https://www.ncbi.nlm.nih.gov/books/NBK470233/?report=reader. [cited January 16, 2025]. |
[54] |
Mehta A, Bath A, Kalavakunta J (2020) Arteriovenous fistula: rare complication of radial artery access. BMJ Case Rep 13: e237278. https://doi.org/10.1136/bcr-2020-237278 ![]() |
[55] | Alomar ME, Michael TT, Patel VG, et al. (2013) Stent loss and retrieval during percutaneous coronary interventions: a systematic review and meta-analysis. J Invasive Cardiol 25: 637-641. |
[56] |
Khattak S, Upadhyaya S, Nolan J, et al. (2021) Manual extraction of a dislodged and migrated DES from radial artery puncture site. Eur Heart J Case Rep 5: ytab468. https://doi.org/10.1093/ehjcr/ytab468 ![]() |
[57] |
Eggebrecht H, Haude M, Von Birgelen C, et al. (2000) Nonsurgical retrieval of embolized coronary stents. Catheter Cardiovasc Interv 51: 432-440. https://doi.org/10.1002/1522-726x(200012)51:4<432::aid-ccd12>3.0.co;2-1 ![]() |
[58] |
Abdalwahab A, Farag M, Brilakis ES, et al. (2021) Management of coronary artery perforation. Cardiovasc Revasc Med 26: 55-60. https://doi.org/10.1016/j.carrev.2020.11.013 ![]() |
[59] |
Umar H, Sharma H, Osheiba M, et al. (2022) Changing trends in the incidence, management and outcomes of coronary artery perforation over an 11-year period: single-centre experience. Open Heart 9: e001916. https://doi.org/10.1136/openhrt-2021-001916 ![]() |
[60] |
Giannini F, Candilio L, Mitomo S, et al. (2018) A practical approach to the management of complications during percutaneous coronary intervention. JACC Cardiovasc Interv 11: 1797-1810. https://doi.org/10.1016/j.jcin.2018.05.052 ![]() |
[61] |
Xenogiannis I, Brilakis ES (2019) Advances in the treatment of coronary perforations. Catheter Cardiovasc Interv 93: 921-922. https://doi.org/10.1002/ccd.28205 ![]() |
[62] |
Muller O, Windecker S, Cuisset T, et al. (2008) Management of two major complications in the cardiac catheterisation laboratory: the no-reflow phenomenon and coronary perforations. EuroIntervention 4: 181-183. https://doi.org/10.4244/eijv4i2a32 ![]() |
[63] |
Anghel L, Tudurachi BS, Tudurachi A, et al. (2023) Patient-related factors predicting stent thrombosis in percutaneous coronary interventions. J Clin Med 12: 7367. https://doi.org/10.3390/jcm12237367 ![]() |
[64] | Grove ECL, Kristensen SD (2007) Stent thrombosis: definitions, mechanisms and prevention. E J Cardiol Practice 5. |
[65] |
Cutlip DE, Windecker S, Mehran R, et al. (2007) Clinical end points in coronary stent trials: a case for standardized definitions. Circulation 115: 2344-2351. https://doi.org/10.1161/CIRCULATIONAHA.106.685313 ![]() |
[66] |
Généreux P, Madhavan MV, Mintz GS, et al. (2014) Ischemic outcomes after coronary intervention of calcified vessels in acute coronary syndromes: pooled analysis from the HORIZONS-AMI (Harmonizing Outcomes with Revascularization and Stents in Acute Myocardial Infarction) and ACUITY (Acute Catheterization and Urgent Intervention Triage Strategy) trials. J Am Coll Cardiol 63: 1845-1854. https://doi.org/10.1016/j.jacc.2014.01.034 ![]() |
[67] |
Abouelnour A, Gori T (2022) Intravascular imaging in coronary stent restenosis: prevention, characterization, and management. Front Cardiovasc Med 9: 843734. https://doi.org/10.3389/fcvm.2022.843734 ![]() |
[68] | Klein LW, Nathan S, Maehara A, et al. (2023) SCAI expert consensus statement on management of in-stent restenosis and stent thrombosis. J Soc Cardiovasc Angiogr Interv 2: 100971. https://doi.org/10.1016/j.jscai.2023.100971 |
[69] |
Généreux P, Stone GW, Harrington RA, et al. (2014) Impact of intraprocedural stent thrombosis during percutaneous coronary intervention: insights from the champion phoenix trial (clinical trial comparing Cangrelor to Clopidogrel standard of care therapy in subjects who require Percutaneous coronary intervention). J Am Coll Cardiol 63: 619-629. https://doi.org/10.1016/j.jacc.2013.10.022 ![]() |
[70] |
Dangas GD, Claessen BE, Mehran R, et al. (2013) Stent thrombosis after primary angioplasty for STEMI in relation to non-adherence to dual antiplatelet therapy over time: results of the HORIZONS-AMI trial. EuroIntervention 8: 1033-1039. https://doi.org/10.4244/EIJV8I9A159 ![]() |
[71] |
Husted S, Boersma E (2016) Case study: ticagrelor in PLATO and Prasugrel in TRITON-TIMI 38 and TRILOGY-ACS trials in patients with acute coronary syndromes. Am J Ther 23: e1876-e1889. https://doi.org/10.1097/MJT.0000000000000237 ![]() |
[72] |
Lee SY, Mintz GS, Kim JS, et al. (2020) Long-term clinical outcomes of drug-eluting stent malapposition. Korean Circ J 50: 880-889. https://doi.org/10.4070/kcj.2020.0198 ![]() |
[73] |
Alfonso F, Coughlan JJ, Giacoppo D, et al. (2022) Management of in-stent restenosis. EuroIntervention 18: e103-e123. https://doi.org/10.4244/EIJ-D-21-01034 ![]() |
[74] | Omeh DJ, Shlofmitz E (2023) Restenosis of Stented Coronary Arteries. Available from: https://www.ncbi.nlm.nih.gov/books/NBK545139/. (Updated Aug 8, 2023). |
[75] |
Halwani DO, Anderson PG, Brott BC, et al. (2012) The role of vascular calcification in inducing fatigue and fracture of coronary stents. J Biomed Mater Res B Appl Biomater 100: 292-304. https://doi.org/10.1002/jbm.b.31911 ![]() |
OCT based calcium score (0–4) [19] | IVUS based calcium score [20] |
Calcium arc: 1. 90–180° (1 point) 2. >180° (2 points) |
Presence of calcified nodule (1 point) |
Presence of 360° circumferential calcium (1 point) | |
Calcium length >5 mm (1 point) | Calcium 270° in >5 mm length (1 point) |
Calcium thickness of >0.5 mm (1 point) | Vessel diameter of <3.5 mm (1 point) |
Description | Management | |
Type I | A perforation with extraluminal crater without extravasation | This is the least severe type and typically does not cause significant bleeding. It can be managed conservatively but may require balloon tamponade or covered stents. |
Type II | A perforation with pericardial or myocardial blushing but no jet of contrast extravasation | Balloon tamponade, covered stents/coil embolization. The patient may require pericardiocentesis |
Type III | A perforation with extravasation through frank (>1 mm) perforation | These are severe types with substantial bleeding into the pericardial space and surrounding pericardium. It requires immediate pericardiocentesis and treatment with covered stents/coil embolization. |
Type IV (Cavity spilling) | Perforation into an anatomical cavity, chamber, or a vessel. | Because the blood leaks into another cardiovascular cavity, symptoms are usually of a new shunt. Type IV tends to be better tolerated than Type III. Management is similar to type III. |
Type V | Perforation of the distal segment | May require embolization of the distal vessel with thrombin, coils or fat. |
Definition | |
Timing | Acute stent thrombosis—occurs within 24 hours of stent implantation |
Subacute stent thrombosis—occurs between 24 hours and 30 days of stent implantation | |
Late stent thrombosis—occurs between 30 days and 1 year post stent implantation | |
Very late stent thrombosis—occurs more than one year after stent implantation | |
Level of certainty | Definite—angiographic or pathological confirmation of stent thrombosis |
Probable—unexplained death within 30 days or target vessel myocardial infarction without angiographic confirmation of stent thrombosis | |
Possible—unexplained death after 30 days |
OCT based calcium score (0–4) [19] | IVUS based calcium score [20] |
Calcium arc: 1. 90–180° (1 point) 2. >180° (2 points) |
Presence of calcified nodule (1 point) |
Presence of 360° circumferential calcium (1 point) | |
Calcium length >5 mm (1 point) | Calcium 270° in >5 mm length (1 point) |
Calcium thickness of >0.5 mm (1 point) | Vessel diameter of <3.5 mm (1 point) |
Description | Management | |
Type I | A perforation with extraluminal crater without extravasation | This is the least severe type and typically does not cause significant bleeding. It can be managed conservatively but may require balloon tamponade or covered stents. |
Type II | A perforation with pericardial or myocardial blushing but no jet of contrast extravasation | Balloon tamponade, covered stents/coil embolization. The patient may require pericardiocentesis |
Type III | A perforation with extravasation through frank (>1 mm) perforation | These are severe types with substantial bleeding into the pericardial space and surrounding pericardium. It requires immediate pericardiocentesis and treatment with covered stents/coil embolization. |
Type IV (Cavity spilling) | Perforation into an anatomical cavity, chamber, or a vessel. | Because the blood leaks into another cardiovascular cavity, symptoms are usually of a new shunt. Type IV tends to be better tolerated than Type III. Management is similar to type III. |
Type V | Perforation of the distal segment | May require embolization of the distal vessel with thrombin, coils or fat. |
Definition | |
Timing | Acute stent thrombosis—occurs within 24 hours of stent implantation |
Subacute stent thrombosis—occurs between 24 hours and 30 days of stent implantation | |
Late stent thrombosis—occurs between 30 days and 1 year post stent implantation | |
Very late stent thrombosis—occurs more than one year after stent implantation | |
Level of certainty | Definite—angiographic or pathological confirmation of stent thrombosis |
Probable—unexplained death within 30 days or target vessel myocardial infarction without angiographic confirmation of stent thrombosis | |
Possible—unexplained death after 30 days |