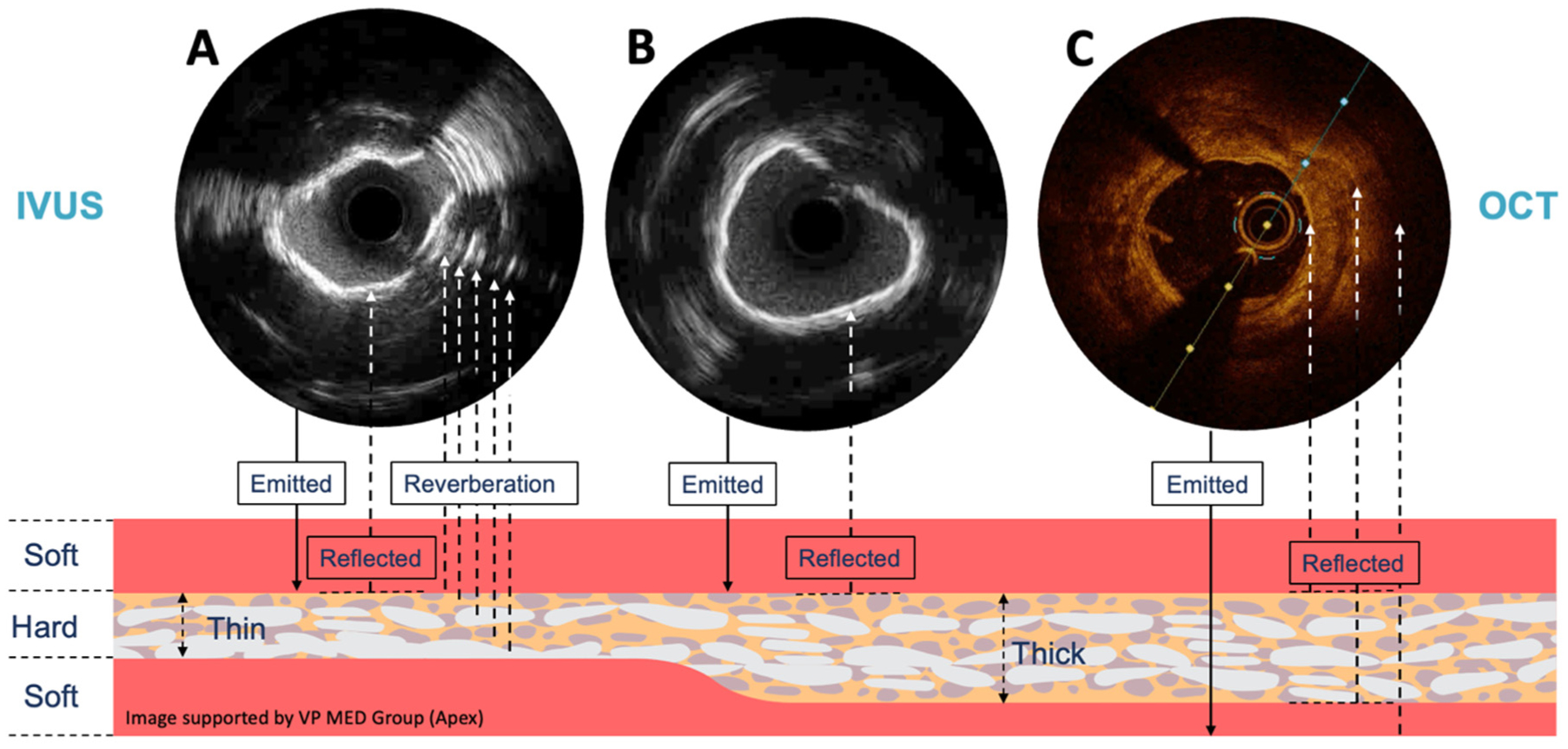
Coronary artery calcium (CAC) poses a major challenge during percutaneous coronary intervention (PCI). The prevalence of significant calcium is reported to be as high as 32%, with higher incidences in the male sex, alongside hypertension, an ST-segment elevation myocardial infarction (STEMI) presentation, and an advancing age. The identification and modification of calcium is important as an association between the presence of CAC and the observation of major adverse cardiovascular events, even in asymptomatic patients. Although angiographically-identified calcium likely reflects the highest burden disease, an inability to assess the calcium, both qualitatively and quantitatively, limits the interventionists in their strategy to achieve an optimal PCI result. Additionally, treating a stent underexpansion in a heavily calcified vessel is more difficult than preventing it. Intra-coronary imaging modalities such as optical coherence tomography (OCT) and intra-vascular ultrasound (IVUS) offer upfront valuable information that may guide the choice of calcium modification tools, evaluate the efficacy of modification/debulking, and permit an optimal stent expansion. Contemporary randomised studies of intra-coronary imaging- vs angiography-guided intervention have demonstrated a clear benefit in adopting an image-guided approach. This review article summarises the value of IVUS and OCT in patients who present with severe calcific diseases.
Citation: Nitin Chandra Mohan, Thomas W. Johnson. Role of intracoronary imaging in severely calcific disease[J]. AIMS Medical Science, 2024, 11(4): 388-402. doi: 10.3934/medsci.2024027
[1] | Teruo Sekimoto, Takamasa Tanaka, Tatsuya Shiraki, Renu Virmani, Aloke V. Finn . How does atherosclerotic plaque become calcified, and why?. AIMS Medical Science, 2024, 11(4): 421-438. doi: 10.3934/medsci.2024029 |
[2] | Ilana S. Golub, Angela Misic, Lucia P. Schroeder, Jairo Aldana-Bitar, Srikanth Krishnan, Sina Kianoush, Travis Benzing, Keishi Ichikawa, Matthew J. Budoff . Calcific coronary lesions: management, challenges, and a comprehensive review. AIMS Medical Science, 2024, 11(3): 292-317. doi: 10.3934/medsci.2024021 |
[3] | Todd T. Nowlen, Luis F. Goncalves, Susan S. Park, Arash A. Sabati . Adolescent Kawasaki Disease patient with coronary calcifications, severe 4-vessel stenosis, and a normal stress MRI. AIMS Medical Science, 2025, 12(1): 28-37. doi: 10.3934/medsci.2025003 |
[4] | Giulia Alagna, Alessia Cascone, Antonino Micari, Giancarlo Trimarchi, Francesca Campanella, Giovanni Taverna, Saro Pistorio, Giuseppe Andò . Type and duration of antithrombotic therapy after treatment of severely calcified lesions. AIMS Medical Science, 2025, 12(1): 38-62. doi: 10.3934/medsci.2025004 |
[5] | Sophia Khattak, Farhan Shahid, Sohail Q. Khan . Challenges and risks associated with coronary calcified lesions in cardiovascular interventions: What makes calcified lesions more challenging and dangerous?. AIMS Medical Science, 2025, 12(1): 69-89. doi: 10.3934/medsci.2025006 |
[6] | Anuj A. Shukla, Shreya Podder, Sana R. Chaudry, Bryan S. Benn, Jonathan S. Kurman . Non-small cell lung cancer: epidemiology, screening, diagnosis, and treatment. AIMS Medical Science, 2022, 9(2): 348-361. doi: 10.3934/medsci.2022016 |
[7] | Ekaterina Kldiashvili, Archil Burduli, Gocha Ghortlishvili . Application of Digital Imaging for Cytopathology under Conditions of Georgia. AIMS Medical Science, 2015, 2(3): 186-199. doi: 10.3934/medsci.2015.3.186 |
[8] | Yonggang Cui, Giuseppe S. Camarda, Anwar Hossain, Ge Yang, Utpal N. Roy, Terry Lall, Ralph B. James . Modeling an Interwoven Collimator for A 3D Endocavity Gamma Camera. AIMS Medical Science, 2016, 3(1): 114-125. doi: 10.3934/medsci.2016.1.114 |
[9] | Prarthana Shrestha, Rik Kneepkens, Gijs van Elswijk, Jeroen Vrijnsen, Roxana Ion, Dirk Verhagen, Esther Abels, Dirk Vossen, and Bas Hulsken . Objective and Subjective Assessment of Digital Pathology Image Quality. AIMS Medical Science, 2015, 2(1): 65-78. doi: 10.3934/medsci.2015.1.65 |
[10] | Gasim Dobie, Sarah Abutalib, Wafa Sadifi, Mada Jahfali, Bayan Alghamdi, Asmaa Khormi, Taibah Alharbi, Munyah Zaqan, Zahra M Baalous, Abdulrahim R Hakami, Mohammed H Nahari, Abdullah A Mobarki, Muhammad Saboor, Mohammad S Akhter, Abdullah Hamadi, Denise E Jackson, Hassan A Hamali . The correlation between severe complications and blood group types in COVID-19 patients; with possible role of T polyagglutination in promoting thrombotic tendencies. AIMS Medical Science, 2023, 10(1): 1-13. doi: 10.3934/medsci.2023001 |
Coronary artery calcium (CAC) poses a major challenge during percutaneous coronary intervention (PCI). The prevalence of significant calcium is reported to be as high as 32%, with higher incidences in the male sex, alongside hypertension, an ST-segment elevation myocardial infarction (STEMI) presentation, and an advancing age. The identification and modification of calcium is important as an association between the presence of CAC and the observation of major adverse cardiovascular events, even in asymptomatic patients. Although angiographically-identified calcium likely reflects the highest burden disease, an inability to assess the calcium, both qualitatively and quantitatively, limits the interventionists in their strategy to achieve an optimal PCI result. Additionally, treating a stent underexpansion in a heavily calcified vessel is more difficult than preventing it. Intra-coronary imaging modalities such as optical coherence tomography (OCT) and intra-vascular ultrasound (IVUS) offer upfront valuable information that may guide the choice of calcium modification tools, evaluate the efficacy of modification/debulking, and permit an optimal stent expansion. Contemporary randomised studies of intra-coronary imaging- vs angiography-guided intervention have demonstrated a clear benefit in adopting an image-guided approach. This review article summarises the value of IVUS and OCT in patients who present with severe calcific diseases.
Coronary artery calcium (CAC) poses a major challenge during percutaneous coronary intervention (PCI). A pooled analysis from large scale randomised trials of patients that presented with acute coronary syndrome (ACUITY and HORIZON-MI) has demonstrated that angiographically-defined moderate to severe target lesion calcium was present in 32% of the patients [1]. The incidence of significant calcium was more commonly associated with the male sex, alongside hypertension, an ST-segment elevation myocardial infarction (STEMI) presentation, and an advancing age. The identification and modification of calcium is important as an association between the presence of CAC and the observation of major adverse cardiovascular events, even in asymptomatic patients [2]. The mechanisms for poor outcomes include difficulties with the device delivery, an acute stent underexpansion, and an increased thrombogenicity [3].
Only 40% of coronary calcium is seen on angiograms. Intra-coronary imaging (ICI) modalities such as intra-vascular ultrasound (IVUS) and optical coherence tomography (OCT) are more sensitive than angiography in being able to detect CAC, with IVUS detecting more calcium than OCT [4],[5]. Although angiographically-identified calcium likely reflects the highest burden disease, the inability to assess the calcium, both qualitatively and quantitatively, limits the interventionist in their strategy to achieve an optimal PCI result. Consequently, the interventionists will either select an adjunctive tool (debulking or fracturing devices) upfront, based upon the interpretation of the angiographic images +/− difficulty in the delivering equipment, or reactively select adjunctive devices when a failure to expand a lesion with standard angioplasty balloons is observed. If the vessel preparation is limited, then there is a further risk that high burden calcium is only detected following the deployment of the stent and a failure to achieve an acceptable acute angiographic result (<20% residual diameter stenosis). This is the worst-case scenario, as options to overcome an acute stent underexpansion are limited. Consequently, an upfront detailed assessment of CAC through either IVUS or OCT can offer valuable information that may guide the choice of calcium modification tools, evaluate the efficacy of modification/debulking, and permit an optimal stent expansion [6],[7]. Contemporary randomised studies of ICI- vs angiography-guided intervention have demonstrated a clear benefit in adopting an image-guided approach [8],[9]. This review article summarises the value of IVUS and OCT in patients who present with severe calcific diseases.
IVUS utilises ultrasound waves produced by the oscillatory movement of the transducer to render a spatial resolution of 100 µm and a tissue depth penetration of 4 to 8 mm. In post-mortem studies, IVUS was shown to have a high sensitivity and is specific to detect calcium (except for microcalcifications). Calcium reflects ultrasound waves, giving the appearance of a hyperechoic bright leading-edge of calcium with acoustic shadowing in the deeper vessel (Figures 1 and 2). The acoustic shadowing has been identified as a possible weakness of IVUS, which limits its ability to appreciate the calcium thickness/burden. Wang et al. have demonstrated that IVUS detects a smoother surface with a reverberation pattern in the presence of calcium thickness <0.5 mm: these appear as concentric arcs at duplicated distances that are generated by multiple reflections from the oscillation of the ultrasound between the transducer and calcium [6]. Moreover, the reverberation pattern can be present following treatment with either an orbital or rotational atherectomy. Interestingly, reverberation was less commonly observed when thick calcium was present (Figure 1) [6]. Although IVUS cannot measure thickness due to a signal drop out, a volumetric index of calcium can be calculated using the arc and length of calcium [6]. IVUS with virtual histology (IVUS-VH) permits a quantitative analysis of the plaque composition. It applies a mathematical model to create 4 plaque components with various color combinations—fibrous tissue (dark green), fibrofatty plaque (light green), necrotic core (red), and dense calcium (white). Whether or not IVUS-VH can accurately assess calcium thickness remains controversial. Furthermore, IVUS-VH has the potential to wrongly identify stents as calcium with or without a necrotic core [10].
Optical coherence tomography uses near-infrared light with a wavelength of 1.30 µm that is generated from a rotating fiber-optic system. Then, this confers high resolution axial images of the coronary artery with a resolution of 10–20 µm, which is 10-fold higher than IVUS. However, the depth of penetration in OCT is limited to 1–2 mm. Furthermore, red blood cells absorb the near-infrared light; therefore, OCT evaluation requires blood clearance, commonly using a contrast agent. Calcium appears as low attenuated areas with well delineated borders created through the refraction/reflection of the light at the interface between different tissue types (Figures 1 and 2). This allows the accurate measurement of the calcium area, thickness, and volume, in addition to its arc and length [11]. The latest available OCT software has the ability to automatically identify the calcific arc and thickness throughout the entire length of the target vessel (Figure 3) [12]. Additionally, the increased resolution from OCT permits the assessment of microcalcifications, which has been associated with peri-procedural enzyme elevation, especially when associated with a thin cap fibroatheroma [10],[13]. Although OCT offers a detailed assessment of calcium, there are certain limitations. Due to either a poor depth penetration or challenges with the contrast clearance, the assessment of calcium can be restricted in large vessels and aorto-ostial lesions [11]. Additionally, the interposition of large lipidic pools or a necrotic core can lead to inaccuracies in the calcium assessment through the attenuation of the light signal if the lipid/necrotic core is superficial to the calcium [10].
Studies have shown that there are certain metrics on ICI that can predict a stent under expansion. Fujino et al. described an OCT-based scoring system for calcific lesions, which was developed using a test cohort of 128 patients and was externally validated in 133 patients [5]. The score was defined as 2 points for a maximum calcium arc >180°, 1 point for a thickness >5 mm, and 1 point for a length >5 mm. A score of 4 was shown to independently predict a poor stent expansion (Figure 3). Similarly, an IVUS-derived scoring system was developed by Zhang et al. in a retrospective study of 328 lesions in 308 patients [4]. In any patient with a superficial calcific arc >270°, 1 point was given for each of the following: calcium >270° in ≥5 mm length, 360° of calcium, the presence of a calcific nodule, and a vessel diameter <3.5 mm (Figure 3). Either a rotational or orbital atherectomy was recommended in the presence of a score of ≥2. Interestingly, this study also reported that in the absence of angiographically visible calcium, even the presence of >270° arc of calcium IVUS was not associated with a stent under-expansion [4].
A facilitated calcium fracture has been shown as one of the key mechanisms that directly impacts stent expansion. An OCT study that consisted of 61 patients with heavy calcification reported that fractures caused by balloon dilatations led to a minimal residual diameter stenosis and a lower frequency of ischaemia-driven target lesion revascularisation during follow-ups [14].
Calcific nodules (CN) account for 30% of fibrocalcific lesions. Findings from the IVUS-VH PROSPECT study showed that bystander CN was identified in 314 of 1573 arteries in 623 patients. CN is most commonly observed at sites of hinge motions with calcific lesions (ostial/mid right coronary artery) or in areas with a high lipidic burden and a necrotic core (left main bifurcation/proximal coronary tree) [15]. CN can be classified as either eruptive or non-eruptive. Eruptive CN can be histologically identified by the presence of an overlying thrombus and a disrupted fibrous cap. On the other hand, non-eruptive nodules have an intact thick fibrous cap. Diagnoses through coronary angiographies can be challenging [15]. Although the presence of a radiolucent mass on an angiogram strongly predicts CN, its negative predictive value is only 65% [16]. In this setting, ICI may allow for the differentiation between these subtypes and guide treatments.
In-vitro IVUS validation studies have demonstrated that non-eruptive CN can be visualised as convex formations protruding into the lumen of the coronary artery (Figure 2). An eccentric protrusion into the vessel lumen can potentially induce a turbulent flow and endothelial shear stress, which can result in platelet aggregation and thrombus formation [17]. However, studies have reported that the presence of non-eruptive CN in non-culprit lesions were benign and had a very low incidence of future cardiac events [18]. Additionally, Sato et al. showed that culprit lesions with non-eruptive CN had the lowest burden of calcium in comparison to eruptive CN and calcific sheets [16]. On OCT, non-eruptive CN had a high signal attenuation similar to that of lipid pools (Figure 2). With the knowledge that the necrotic core becomes calcified as part of the atherosclerotic process, non-eruptive CN may represent a previously ruptured necrotic core [19].
Eruptive CN is the least common aetiology of atherosclerotic acute coronary syndrome (ACS) [20]. OCT is superior to IVUS in identifying an overlying thrombus and an intimal disruption found in these lesions (Figure 2). However, an overlying thrombus can attenuate deeper structures, potentially leading to the misdiagnosis of the acute culprit event on OCT [21]. An OCT study by Jia et al. that involved an OCT analysis of 126 patients showed that a CN was the culprit in 7.9% of patients with ACS [22]. Eruptive nodules pose unique challenges during PCI and can impede the stent optimisation. An autopsy study of 26 consecutive lesions with CN in patients who had ACS showed that all cases had surface non-occlusive thrombus with evidence of endothelial loss [19]. Disruption of the fibrous caps in these patients was triggered by the fragmentation of necrotic-core calcifications, which is then encircled with collagen rich calcifications that are prone to shear mechanical stress [19]. Even though the eruptive nodules have shown to have better acute stent expansion results than the non-eruptive nodules, they were correlated with worse clinical outcomes in the longer term [16]. In a study of patients who underwent pre- and post-intervention OCT, Sato et al. demonstrated that eruptive CNs were associated with a great stent expansion (89.2% ± 18.7% vs. 81.5% ± 18.9%; P = 0.003) in comparison to non-eruptive CNs, after adjusting for procedural and morphological characteristics [16]. This supports the fact that eruptive CNs are more deformable due to the lack of a collagen matrix, a plaque haemorrhage, and small calcium fragments [16]. However, at the two-year follow-up, the presence of eruptive CN were shown to be independently associated with a target lesion failure (TLF) (HR: 2.07; P = 0.048) [16]. In this cohort, the in-stent protrusion of the calcific nodule was shown to be one of the major reasons for TLF [16]. These observations merit further studies, with consideration of a tailored interventional approach, either by selecting a stent with enhanced radial strength/reduced risk of fracture or perhaps considering a stent-free strategy with the use of drug-coated balloons.
Superficial calcific sheets are another subtype of fibrocalcific lesion, and their presence has been shown to predict negative remodelling, which could potentially limit the stretching capacity of the vessel (Figure 2). In the setting of ACS, culprit lesions associated with superficial calcium had smaller lumen areas compared to CN [23]. Luminal narrowing due to these superficial sheets could generate high endothelial shear stress that further provokes platelet aggregation. Furthermore, the stress at the junction of the calcific plate could lead to intimal disruption, which may subsequently thrombose [23]. Circumferential calcium poses the greatest challenge to an adequate vessel expansion; however, the advent of disruptive balloon-based modification tools (e.g., intravascular lithotripsy) has simplified the approach to disrupting and increasing the vessel compliance.
An ICI guided approach to PCI in calcific lesions should ideally begin with an imaging assessment; however, the nature of disease may prevent an upfront image-probe delivery. However, a failure to deliver the devices upfront, and the need for adjunctive calcium modification tools, should trigger the use of ICI “pre-stenting” to confirm an adequate vessel preparation.
Upfront imaging allows for the quantitative and qualitative assessments of calcium burden. We have already highlighted the benefit of quantitative assessments to identify lesions at risk of a subsequent stent underexpansion [4],[5]. Combining these validated methods of quantitation and a qualitative analysis of the calcium may aid the interventionist in selecting the most appropriate tool for modification. For example, IVL may be preferred where calcium is concentric and the lumen relatively preserved, whilst protrusive, high-volume calcium may be better approached with an upfront debulking strategy (rotational or orbital atherectomy). Treating a stent underexpansion in a heavily calcified vessel is more difficult than preventing it. Therefore, repeat pre-stenting imaging runs may be mandated to assess for an adequate calcium modification prior to the stent deployment. This is particularly beneficial when opting for a drug coated balloon strategy, where it essential to adequately prepare lesions prior to deployment [24]. Beyond calcium modifications, ICI helps with defining the appropriate landing zones with the sizing (diameter and length) of devices. Imaging using a motorised device with a continued control of the image quality is preferrable. The imaging run should begin at least 10mm distal to the target lesion and finish at the ostium of the vessel. Co-registration is a useful tool in establishing a correlation between the findings on angiograms and is available on both ICI modalities. This allows for an accurate assessment of the landing zones and can be particularly useful in the treatment of complex bifurcation disease where spatial orientation is challenging [25].
Both OCT and IVUS have workflows designed to help simplify and standardise their use in the catheterization laboratory. The algorithm, called MLD-MAX, was developed to help incorporate OCT into the workflow of imaging-guided intervention [26]. “MLD”, which stands for Morphology, lesion Length, and Diameter, forms the pre-stenting component of the algorithm, while “MAX” represents the post-stenting assessment of the Medial edge dissection, stent Apposition, and Expansion. The light lab (LL) initiative was setup to evaluate the value of the “MLD-MAX” algorithm in daily practice. The adoption of MLD-MAX resulted in a more aggressive vessel preparation in 85% of patients with significant calcium (n = 155), with an increase in modification tool selection to 55% (cutting/scoring balloon, rotational atherectomy, laser) [27]. With the LL OCT workflow, the overall radiation exposure was significantly lower compared to the angiogram guidance with no difference in the contrast use between the two groups [26]. Furthermore, the overall procedure time was only prolonged by 9 minutes with the LL OCT workflow. Similarly, the 123 workflow was developed for IVUS users. The principles of IVUS based on “123” are akin to the OCT based “MLD-MAX”. Although these algorithms are not dedicated for severe calcifications, they offer general guidance on how such complex lesions could be approached.
Decisions relating to the choice of calcium modification tool depends on several factors: the location of the lesion, the burden of disease, the lesion cross-ability, the concentricity, the operator experience, and the device availability. ICI plays a significant role and will reduce the uncertainty associated with the device selection. ICI allows for the precise assessment of the reference vessel diameter, which allows for an accurate sizing of the devices. According to the scoring systems, defining the calcium arc, length, and thickness may justify the adoption of adjunctive devices. The operator experience, procedural risk, and regulatory and economic restrictions all impact the decision making. Simplistically, devices can be considered to fracture, debulk, or have a combined effect on calcium. Many algorithms exist, but a consensus does not yet exist for which modality should be used for different types of calcium [28],[29]. As discussed earlier, concentric calcification poses a challenge to the vessel expansion; however, a balloon-assisted approach may be sufficient to overcome calcium. In a cost-restricted environment, an NC-balloon inflation followed by ICI to confirm a calcium fracture may be sufficient. In fact, data exists to support a balloon modification where calcium is non-concentric but the arc extends >227° with a thickness <0.67 mm through a canterlevering type effect on the calcium, with a fracture at the apex [30]. Although NC balloons can expand to high pressures, their single layer structure makes them prone to non-uniform expansion or even damage, particularly in the presence of spiculated calcium [31]. An alternative balloon-assisted modification may be considered, with scoring/cutting balloons being able to exert a greater stress on calcific lesions, thereby achieving fractures more consistently at lower pressures than the equivalent NC balloons [32]. In contrast, high-pressure, dual-layer OPN balloons could be considered. OPN balloons have a low profile and can uniformly exert pressure, thus demonstrating an equivalence when compared with the scoring balloons [33] Although effective, these balloons have the risk of inducing a local wall injury, which may be a substrate for future restenosis [28]. Intra-vascular lithotripsy (IVL) has been demonstrated to provide a safe and reliable approach to fracturing large-arc/concentric calcifications and achieves high rates of procedural success [34],[35]. IVL works by releasing energy, which then vaporises the saline/contrast mixture within the balloon and creates high amplitude ultrasonic pressure waves. It has been demonstrated as being safe for use in eccentric lesions, and calcium fractures were noted to be higher in the setting of an increasing calcium concentricity [35]. A recent pooled analysis of the DISRUPT CAD studies showed that IVL led to consistent improvements in the luminal gain and stent expansion. Interestingly, a contemporary registry of IVL use in France has highlighted the restricted use of lithotripsy (<80 pulses) related to higher rates of a target vessel failure [36]. However, this series was undertaken with a very low rate of ICI-use; therefore, it would be interesting to consider the impact ICI might have in confirming the efficacy of IVL and optimising an acute success with an impact on the subsequent TVF. Alternative strategies include the use of either cutting balloons (CB) or scoring balloons (SB).
In eccentric calcific plaques, calcium modification or fractures may not necessarily be observed following ballooning, as the force is biased towards the non-calcified part of the plaque. Therefore, this increases the risk of coronary perforations, particularly when the eccentric calcium is localised to the myocardial side, in which case the balloon inflation stretches the epicardial aspect of vessel [35]. In eccentric lesions, ICI can also be beneficial in the assessment of a wire bias and potentially predict the effect of plaque modifications. Specifically, if the imaging probe/interventional wire is in contact with regions of calcium, the likelihood of a device interaction with the lesion is enhanced [37].
Plaque modifications can be especially challenging in lesions with thick calcific plates (>0.5 mm); if an adequate balloon expansion cannot be achieved, an atherectomy device should be considered. Currently available atherectomy devices include rotational atherectomy, orbital atherectomy, and an excimer laser [28],[35],[38],[39].
Rotational atherectomy (RA) has a diamond encrusted burr at the tip, which rotates at speeds of 140000 to 220000 rpm and ablates non-elastic/fibro-calcified tissues while deflecting away from softer tissues. Studies have shown that RA offers the greatest benefit in smaller lumens (≤4 mm) [40]. Large retrospective studies have reported that complications associated with RA tends to be lower in high-operator volume centers than vice-versa [38]. Additionally, recent data suggests that larger burr sizes are associated with a greater risk of no-reflow, vessel dissection, perforation, bradycardia, and peri-procedural myocardial infarction (MI) [41]. The recent PREPARE-CALC COMBO [42] study compared the effects of using both RA and cutting/scoring balloons with the control arm (balloon-based strategy only) of the PREPARE-CALC trial [43]. The acute luminal gain and minimum stent area were significantly higher in the treatment arm and the rates of target vessel failures were comparable between both the groups at 9 months.
The ablative crown in orbital atherectomy (OA) is located 6.5mm proximal to the tip, which is different to RA, where the burr is located at the tip. The crowns orbital diameter radially expands with an increasing centrifugal force, giving the operator control over the depth of ablation by altering the speed of the burr [28]. Due to the elliptical nature of the burr, it is contraindicated in small vessels (<2.5 mm). The fact that the burr is proximal to the tip also makes it less suited for balloon uncrossable lesions. The current data suggest that OA is safe and effective with acceptable rates of MACE [39],[44].
Balance must be struck between attempts at minimising malapposition with larger balloons versus increasing the risk of vessel perforations. Clinicians need to be aware that malapposition is a common finding in severely calcific lesions as these plaque types have poor conformability. Previous OCT and IVUS studies have demonstrated that the presence of severe calcifications is more commonly associated with acute malapposition, and an calcific arc, rather than the thickness, has shown to have a greater impact on malapposition [10],[25].
The incidence of a stent fracture was also higher in calcific lesions; however, this did not result in a greater amount of intimal hyperplasia during follow-ups, particularly when drug-eluting stents were used [45]. With respect to edge dissections, IVUS studies have illustrated that a balloon related injury was likely to occur at the transition point from a calcific to a non-calcific plaque [46]. The severity of the dissection was related to the extent of calcium [47]. However, the presence of calcium appears to prevent an extension of the intramural haematoma and commonly occurs at the non-diseased stent edge segments [47].
One of the main determinants for target lesion failures is stent underexpansion [8],[9]. The metrics used to measure a stent expansion include the minimum stent area (MSA) and the relative expansion compared with the reference area. According to the European Society of Cardiology consensus document on intracoronary imaging, an optimal expansion is said to be achieved when the absolute MSA is >5.5 mm2 in IVUS or >4.5 mm2 in OCT/relative stent expansion is >80% [21],[25]. The use of absolute values of MSA should be cautioned, as they must be considered in the context of the treated vessel. For example, in a vessel with a reference diameter of 2.5 mm, an OCT MSA of 4.5 mm2 equates to an area stenosis of 8% (or stent expansion 92%—4.5/4.9 mm2 × 100), whereas in a 4.0 mm vessel, an OCT MSA of 4.5 mm2 equates to a stent expansion of 36%. Consequently, we can advocate for using a stent expansion as the preferred method of assessing the stent optimisation, and realistically achieving 80% expansion in complex disease is appropriate.
Managing severely calcified lesion is challenging and operators need to recognise the inherent limitations of solely utilizing angiograms as guidance. In this cohort, PCI was associated with high rates of peri-procedural complications and longer-term MACE, which was largely driven by stent underexpansion. ICI allows for the assessment of calcium with accurate quantitative and qualitative analyses of the calcium distribution and may provide guidance on the appropriate modification device selection. Most importantly, ICI facilitates the assessment of adequate calcium modifications, an appropriate stent selection, and the ultimate optimisation of the acute result. More studies are needed to identify and validate a dedicated algorithm to manage severely calcific lesions; however, it is clear that ICI should play a central role with the management of coronary calcifications.
Nitin Chandra Mohan and Thomas W. Johnson were equally involved in literature review and drafting of the manuscript.
The authors declare they have not used Artificial Intelligence (AI) tools in the creation of this article.
[1] |
Généreux P, Madhavan MV, Mintz GS, et al. (2014) Ischemic outcomes after coronary intervention of calcified vessels in acute coronary syndromes. Pooled analysis from the HORIZONS-AMI (Harmonizing Outcomes With Revascularization and Stents in Acute Myocardial Infarction) and ACUITY (Acute Catheterization and Urgent Intervention Triage Strategy) trials. J Am Coll Cardiol 63: 1845-1854. https://doi.org/10.1016/j.jacc.2014.01.034 ![]() |
[2] |
Petousis S, Skalidis E, Zacharis E, et al. (2023) The role of intracoronary imaging for the management of calcified lesions. J Clin Med 12: 4622. https://doi.org/10.3390/jcm12144622 ![]() |
[3] |
O'Donnell CJ, Kavousi M, Smith AV, et al. (2011) Genome-wide association study for coronary artery calcification with follow-up in myocardial infarction. Circulation 124: 2855-2864. https://doi.org/10.1161/CIRCULATIONAHA.110.974899 ![]() |
[4] |
Zhang M, Matsumura M, Usui E, et al. (2021) Intravascular ultrasound-derived calcium score to predict stent expansion in severely calcified lesions. Circ Cardiovasc Interv 14: e010296. https://doi.org/10.1161/CIRCINTERVENTIONS.120.010296 ![]() |
[5] |
Fujino A, Mintz GS, Matsumura M, et al. (2018) A new optical coherence tomography-based calcium scoring system to predict stent underexpansion. EuroIntervention 13: e2182-e2189. https://doi.org/10.4244/EIJ-D-17-00962 ![]() |
[6] |
Wang X, Matsumura M, Mintz GS, et al. (2017) In vivo calcium detection by comparing optical coherence tomography, intravascular ultrasound, and angiography. JACC Cardiovasc Imaging 10: 869-879. https://doi.org/10.1016/j.jcmg.2017.05.014 ![]() |
[7] |
Mintz GS, Painter JA, Pichard AD, et al. (1995) Atherosclerosis in angiographically “normal” coronary artery reference segments: an intravascular ultrasound study with clinical correlations. J Am Coll Cardiol 25: 1479-1485. https://doi.org/10.1016/0735-1097(95)00088-l ![]() |
[8] |
Lee JM, Choi KH, Song YB, et al. (2023) Intravascular imaging-guided or angiography-guided complex PCI. N Engl J Med 388: 1668-1679. https://doi.org/10.1056/NEJMoa2216607 ![]() |
[9] |
Zhang J, Gao X, Kan J, et al. (2018) Intravascular ultrasound versus angiography-guided drug-eluting stent implantation: the ULTIMATE trial. J Am Coll Cardiol 72: 3126-3137. https://doi.org/10.1016/j.jacc.2018.09.013 ![]() |
[10] |
Mintz GS (2015) Intravascular imaging of coronary calcification and its clinical implications. JACC Cardiovasc Imaging 8: 461-471. https://doi.org/10.1016/j.jcmg.2015.02.003 ![]() |
[11] |
Mohan NC, Johnson TW (2022) Intracoronary optical coherence tomography—an introduction. Catheter Cardio Inte 100: S57-S65. https://doi.org/10.1002/ccd.30583 ![]() |
[12] |
Chandramohan N, Hinton J, O'Kane P, et al. (2024) Artificial intelligence for the interventional cardiologist: powering and enabling OCT image interpretation. Interv Cardiol 19: e03. https://doi.org/10.15420/icr.2023.13 ![]() |
[13] |
Tearney GJ, Regar E, Akasaka T, et al. (2012) Consensus standards for acquisition, measurement, and reporting of intravascular optical coherence tomography studies: a report from the international working group for intravascular optical coherence tomography standardization and validation. J Am Coll Cardiol 59: 1058-1072. https://doi.org/10.1016/j.jacc.2011.09.079 ![]() |
[14] |
Kubo T, Shimamura K, Ino Y, et al. (2015) Superficial calcium fracture after PCI as assessed by OCT. JACC Cardiovasc Imaging 8: 1228-1229. https://doi.org/10.1016/j.jcmg.2014.11.012 ![]() |
[15] |
Shin D, Karimi Galougahi K, Spratt JC, et al. (2024) Calcified nodule in percutaneous coronary intervention: therapeutic challenges. JACC Cardiovasc Interv 17: 1187-1199. https://doi.org/10.1016/j.jcin.2024.03.032 ![]() |
[16] |
Sato T, Matsumura M, Yamamoto K, et al. (2023) Impact of eruptive vs noneruptive calcified nodule morphology on acute and long-term outcomes after stenting. JACC Cardiovasc Interv 16: 1024-1035. https://doi.org/10.1016/j.jcin.2023.03.009 ![]() |
[17] |
Malek AM, Alper SL, Izumo S (1999) Hemodynamic shear stress and its role in atherosclerosis. JAMA 282: 2035-2042. https://doi.org/10.1001/jama.282.21.2035 ![]() |
[18] |
Xu Y, Mintz GS, Tam A, et al. (2012) Prevalence, distribution, predictors, and outcomes of patients with calcified nodules in native coronary arteries: a 3-vessel intravascular ultrasound analysis from Providing Regional Observations to Study Predictors of Events in the Coronary Tree (PROSPECT). Circulation 126: 537-545. https://doi.org/10.1161/CIRCULATIONAHA.111.055004 ![]() |
[19] |
Torii S, Sato Y, Otsuka F, et al. (2021) Eruptive calcified nodules as a potential mechanism of acute coronary thrombosis and sudden death. J Am Coll Cardiol 77: 1599-1611. https://doi.org/10.1016/j.jacc.2021.02.016 ![]() |
[20] |
Virmani R, Kolodgie FD, Burke AP, et al. (2000) Lessons from sudden coronary death: a comprehensive morphological classification scheme for atherosclerotic lesions. Arterioscler Thromb Vasc Biol 20: 1262-1275. https://doi.org/10.1161/01.atv.20.5.1262 ![]() |
[21] |
Johnson TW, Räber L, Di Mario C, et al. (2019) Clinical use of intracoronary imaging. Part 2: acute coronary syndromes, ambiguous coronary angiography findings, and guiding interventional decision-making: an expert consensus document of the European Association of Percutaneous Cardiovascular Interventions: endorsed by the Chinese Society of Cardiology, the Hong Kong Society of Transcatheter Endocardiovascular Therapeutics (HKSTENT) and the Cardiac Society of Australia and New Zealand. Eur Heart J 40: 2566-2584. https://doi.org/10.1093/eurheartj/ehz332 ![]() |
[22] |
Jia H, Abtahian F, Aguirre AD, et al. (2013) In vivo diagnosis of plaque erosion and calcified nodule in patients with acute coronary syndrome by intravascular optical coherence tomography. J Am Coll Cardiol 62: 1748-1758. https://doi.org/10.1016/j.jacc.2013.05.071 ![]() |
[23] |
Sugiyama T, Yamamoto E, Fracassi F, et al. (2019) Calcified plaques in patients with acute coronary syndromes. JACC Cardiovasc Interv 12: 531-540. https://doi.org/10.1016/j.jcin.2018.12.013 ![]() |
[24] |
Jeger RV, Eccleshall S, Wan Ahmad WA, et al. (2020) Drug-coated balloons for coronary artery disease: third report of the international DCB consensus group. JACC Cardiovasc Interv 13: 1391-1402. https://doi.org/10.1016/j.jcin.2020.02.043 ![]() |
[25] |
Räber L, Mintz GS, Koskinas K C, et al. (2018) Clinical use of intracoronary imaging. Part 1: guidance and optimization of coronary interventions. An expert consensus document of the European Association of Percutaneous Cardiovascular Interventions. Eur Heart J 39: 3281-3300. https://doi.org/10.1093/eurheartj/ehy285 ![]() |
[26] |
Osborn EA, Johnson M, Maksoud A, et al. (2023) Safety and efficiency of percutaneous coronary intervention using a standardised optical coherence tomography workflow. EuroIntervention 18: 1178-1187. https://doi.org/10.4244/EIJ-D-22-00512 ![]() |
[27] |
Bergmark B, Dallan LAP, Pereira GTR, et al. (2022) Decision-making during percutaneous coronary intervention guided by optical coherence tomography: insights from the LightLab initiative. Circ Cardiovasc Interv 15: 872-881. https://doi.org/10.1161/CIRCINTERVENTIONS.122.011851 ![]() |
[28] |
Shah M, Najam O, Bhindi R, et al. (2021) Calcium modification techniques in complex percutaneous coronary intervention. Circ Cardiovasc Interv 14: e009870. https://doi.org/10.1161/CIRCINTERVENTIONS.120.009870 ![]() |
[29] |
De Maria GL, Scarsini R, Banning AP (2019) Management of calcific coronary artery lesions: Is it time to change our interventional therapeutic approach?. JACC Cardiovasc Interv 12: 1465-1478. https://doi.org/10.1016/j.jcin.2019.03.038 ![]() |
[30] |
Maejima N, Hibi K, Saka K, et al. (2016) Relationship between thickness of calcium on optical coherence tomography and crack formation after balloon dilatation in calcified plaque requiring rotational atherectomy. Circ J 80: 1413-1419. https://doi.org/10.1253/circj.CJ-15-1059 ![]() |
[31] |
Hoffmann R, Mintz GS, Popma JJ, et al. (1998) Treatment of calcified coronary lesions with Palmaz-Schatz stents. An intravascular ultrasound study. Eur Heart J 19: 1224-1231. https://doi.org/10.1053/euhj.1998.1028 ![]() |
[32] |
Song J, Ascherman B, Eudailey KW, et al. (2017) Long-term failure of Amplatzer plugs in the treatment of aortic pathology. J Card Surg 32: 426-429. https://doi.org/10.1111/jocs.13166 ![]() |
[33] |
Rheude T, Rai H, Richardt G, et al. (2021) Super high-pressure balloon versus scoring balloon to prepare severely calcified coronary lesions: the ISAR-CALC randomised trial. EuroIntervention 17: 481-488. https://doi.org/10.4244/EIJ-D-20-01000 ![]() |
[34] |
Hill JM, Kereiakes DJ, Shlofmitz RA, et al. (2020) Intravascular lithotripsy for treatment of severely calcified coronary artery disease. J Am Coll Cardiol 76: 2635-2646. https://doi.org/10.1016/j.jacc.2020.09.603 ![]() |
[35] | Ali ZA, Kereiakes DJ, Hill JM, et al. (2023) Impact of calcium eccentricity on the safety and effectiveness of coronary intravascular lithotripsy: pooled analysis from the disrupt CAD studies. Circ Cardiovasc Interv 16: e012898. https://doi.org/10.1161/CIRCINTERVENTIONS.123.012898 |
[36] |
Honton B, Cayla G, Lhermusier T, et al. (2024) National france LILI registry on intravascular lithotripsy: 30 days follow up. Arch Cardiovasc Dis 117: S15-S16. https://doi.org/10.1016/j.acvd.2023.10.028 ![]() |
[37] |
Hamana T, Kawamori H, Toba T, et al. (2023) Prediction of the debulking effect of rotational atherectomy using optical frequency domain imaging: a prospective study. Cardiovasc Interv Ther 38: 316-326. https://doi.org/10.1007/s12928-023-00928-9 ![]() |
[38] |
Isogai T, Yasunaga H, Matsui H, et al. (2016) Relationship between hospital volume and major cardiac complications of rotational atherectomy: a nationwide retrospective cohort study in Japan. J Cardiol 67: 442-448. https://doi.org/10.1016/j.jjcc.2015.07.008 ![]() |
[39] |
Meraj PM, Shlofmitz E, Kaplan B, et al. (2018) Clinical outcomes of atherectomy prior to percutaneous coronary intervention: a comparison of outcomes following rotational versus orbital atherectomy (COAP-PCI study). J Interv Cardiol 31: 478-485. https://doi.org/10.1111/joic.12511 ![]() |
[40] |
Yamamoto MH, Maehara A, Karimi Galougahi K, et al. (2017) Mechanisms of orbital versus rotational atherectomy plaque modification in severely calcified lesions assessed by optical coherence tomography. JACC Cardiovasc Interv 10: 2584-2586. https://doi.org/10.1016/j.jcin.2017.09.031 ![]() |
[41] |
Barbato E, Carrié D, Dardas P, et al. (2015) European expert consensus on rotational atherectomy. EuroIntervention 11: 30-36. https://doi.org/10.4244/EIJV11I1A6 ![]() |
[42] |
Allali A, Toelg R, Abdel-Wahab M, et al. (2022) Combined rotational atherectomy and cutting balloon angioplasty prior to drug-eluting stent implantation in severely calcified coronary lesions: the PREPARE-CALC-COMBO study. Catheter Cardiovasc Interv 100: 979-989. https://doi.org/10.1002/ccd.30423 ![]() |
[43] |
Abdel-Wahab M, Toelg R, Byrne RA, et al. (2018) High-speed rotational atherectomy versus modified balloons prior to drug-eluting stent implantation in severely calcified coronary lesions. Circ Cardiovasc Interv 11: e007415. https://doi.org/10.1161/CIRCINTERVENTIONS.118.007415 ![]() |
[44] |
Généreux P, Bettinger N, Redfors B, et al. (2016) Two-year outcomes after treatment of severely calcified coronary lesions with the orbital atherectomy system and the impact of stent types: Insight from the ORBIT II trial. Catheter Cardiovasc Interv 88: 369-377. https://doi.org/10.1002/ccd.26554 ![]() |
[45] |
Morlacchi S, Pennati G, Petrini L, et al. (2014) Influence of plaque calcifications on coronary stent fracture: a numerical fatigue life analysis including cardiac wall movement. J Biomech 47: 899-907. https://doi.org/10.1016/j.jbiomech.2014.01.007 ![]() |
[46] |
Maehara A, Mintz GS, Bui AB, et al. (2002) Incidence, morphology, angiographic findings, and outcomes of intramural hematomas after percutaneous coronary interventions: an intravascular ultrasound study. Circulation 105: 2037-2042. https://doi.org/10.1161/01.cir.0000015503.04751.bd ![]() |
[47] |
Liu X, Tsujita K, Maehara A, et al. (2009) Intravascular ultrasound assessment of the incidence and predictors of edge dissections after drug-eluting stent implantation. JACC Cardiovasc Interv 2: 997-1004. https://doi.org/10.1016/j.jcin.2009.07.012 ![]() |