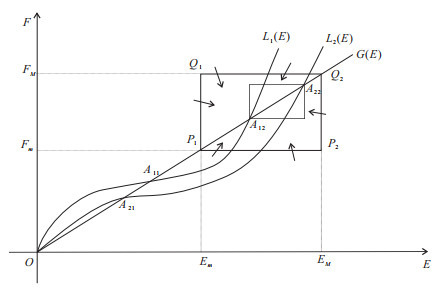
Using a quasi-natural experiment of the staggered construction of energy conservation and emission reduction (ECER) demonstration cities in China, this paper explores the impact of fiscal policy on green credit (GC) for listed enterprises. The main findings are the following: (1) The ECER demonstration cities policy significantly improves enterprise GC. This conclusion remains robust after considering the heterogeneous treatment effects. (2) By extending the estimation period beyond the policy withdrawal, we use the difference-in-differences designs with multiple groups and periods (DIDM) estimator to compute the average treatment effect of policy switches. The results show that the intervention effect still exists after the policy withdrawal. (3) Direct cash subsidies, corporate green spending, and external financing constraints are the main mechanisms through which the ECER demonstration cities policy works. (4) The effects of the ECER demonstration cities policy are heterogeneous at the batch, urban, industry, and corporate levels. This paper explores the role of fiscal policy on enterprise green financing. The findings provide theoretical and empirical insights for leveraging fiscal tools to enhance environmental governance.
Citation: Yangyang Cheng, Zhenhuan Xu. Fiscal policy promotes corporate green credit: Experience from the construction of energy conservation and emission reduction demonstration cities in China[J]. Green Finance, 2024, 6(1): 1-23. doi: 10.3934/GF.2024001
[1] | San-Xing Wu, Xin-You Meng . Hopf bifurcation analysis of a multiple delays stage-structure predator-prey model with refuge and cooperation. Electronic Research Archive, 2025, 33(2): 995-1036. doi: 10.3934/era.2025045 |
[2] | Bo Zheng, Lijie Chang, Jianshe Yu . A mosquito population replacement model consisting of two differential equations. Electronic Research Archive, 2022, 30(3): 978-994. doi: 10.3934/era.2022051 |
[3] | Xinrui Yan, Yuan Tian, Kaibiao Sun . Effects of additional food availability and pulse control on the dynamics of a Holling-(p+1) type pest-natural enemy model. Electronic Research Archive, 2023, 31(10): 6454-6480. doi: 10.3934/era.2023327 |
[4] | Mengting Sui, Yanfei Du . Bifurcations, stability switches and chaos in a diffusive predator-prey model with fear response delay. Electronic Research Archive, 2023, 31(9): 5124-5150. doi: 10.3934/era.2023262 |
[5] | Meng Wang, Naiwei Liu . Qualitative analysis and traveling wave solutions of a predator-prey model with time delay and stage structure. Electronic Research Archive, 2024, 32(4): 2665-2698. doi: 10.3934/era.2024121 |
[6] | Miao Peng, Rui Lin, Zhengdi Zhang, Lei Huang . The dynamics of a delayed predator-prey model with square root functional response and stage structure. Electronic Research Archive, 2024, 32(5): 3275-3298. doi: 10.3934/era.2024150 |
[7] | Gaohui Fan, Ning Li . Application and analysis of a model with environmental transmission in a periodic environment. Electronic Research Archive, 2023, 31(9): 5815-5844. doi: 10.3934/era.2023296 |
[8] | Zhili Zhang, Aying Wan, Hongyan Lin . Spatiotemporal patterns and multiple bifurcations of a reaction- diffusion model for hair follicle spacing. Electronic Research Archive, 2023, 31(4): 1922-1947. doi: 10.3934/era.2023099 |
[9] | Yujia Xiang, Yuqi Jiao, Xin Wang, Ruizhi Yang . Dynamics of a delayed diffusive predator-prey model with Allee effect and nonlocal competition in prey and hunting cooperation in predator. Electronic Research Archive, 2023, 31(4): 2120-2138. doi: 10.3934/era.2023109 |
[10] | Qiang Yu, Xiujuan Jiang . Stability analysis of discrete-time switched systems with bipartite PDT switching. Electronic Research Archive, 2024, 32(11): 6320-6337. doi: 10.3934/era.2024294 |
Using a quasi-natural experiment of the staggered construction of energy conservation and emission reduction (ECER) demonstration cities in China, this paper explores the impact of fiscal policy on green credit (GC) for listed enterprises. The main findings are the following: (1) The ECER demonstration cities policy significantly improves enterprise GC. This conclusion remains robust after considering the heterogeneous treatment effects. (2) By extending the estimation period beyond the policy withdrawal, we use the difference-in-differences designs with multiple groups and periods (DIDM) estimator to compute the average treatment effect of policy switches. The results show that the intervention effect still exists after the policy withdrawal. (3) Direct cash subsidies, corporate green spending, and external financing constraints are the main mechanisms through which the ECER demonstration cities policy works. (4) The effects of the ECER demonstration cities policy are heterogeneous at the batch, urban, industry, and corporate levels. This paper explores the role of fiscal policy on enterprise green financing. The findings provide theoretical and empirical insights for leveraging fiscal tools to enhance environmental governance.
Mosquito-borne infectious diseases are spread by mosquitoes and are prevalent worldwide, including malaria, dengue fever, filariasis, yellow fever, and other highly harmful infectious diseases. Research shows that there are more than 80 such diseases, many of which have caused significant losses to human lives and health in different countries and regions. In recent years, the risk of mosquito-borne infectious disease outbreaks has increased due to climate change, as well as the rise of commercial trade and personnel exchanges.
Due to the lack of an effective vaccine for mosquito-borne diseases, controlling the population of mosquitoes has become crucial in curbing the spread of such diseases. Traditional physicochemical control methods have significant disadvantages such as negative environmental effects and drug resistance. In recent years, new biological control approaches such as insect sterility technology (SIT) and its derivatives have been extensively studied and practiced. These approaches involve the release of sterile or Wolbachia-infected mosquitoes into the wild to disrupt or suppress the development of the wild mosquito population. Certain studies specifically explored the use of sterile insect release for targeted population control [1,2,3]. Other research considered how the endosymbiotic bacterium Wolbachia can induce resistance to the dengue virus in Aedes aegypti [4], and there has been research into the control of particular mosquito-borne diseases [5]. Furthermore, in [6], a combination of incompatible and sterile insect techniques has been implemented to eradicate wild mosquitoes. During the exploration of these technologies, detailed analyses have been conducted on specific population characteristics such as population diffusion, periodic changes, the Allee effect, and others [7,8,9,10,11]. To ensure successful suppression of mosquito populations, continuous release over a long period is often necessary. Researchers have studied release strategies for different situations through various methods. For instance, uninterrupted continuous release strategies, based on ODE or DDE models, have undergone extensive examination [12,13,14,15]. More recently, impulsive release strategies, which better align with practical operations, have received considerable attention. Switched systems have been widely employed in simulating these release strategies [16,17,18,19,20]. In addition, many researchers have shown a preference for impulsive differential dynamical systems [21,22,23,24]. Some have integrated the duration of sexual activity of sterile mosquitoes with impulsive release behavior to investigate release strategies that effectively suppress mosquito populations [25,26,27].
Typically, male sterile mosquitoes are released into the wild to mate with female wild mosquitoes, rendering them unable to lay or hatch eggs. In [18,26,27,28], the authors proposed a mathematical model that considers the sexual lifespan of sterile mosquitoes, including only those within their sexually active period. Due to the short sexual lifespan of sterile mosquitoes, natural deaths during the sexual activity period are often neglected. Instead of using a single equation to describe the change in the number of sterile mosquitoes, the number of sterile mosquitoes is used as a control function [15,18,26,27,28,29]. Based on these ideas, the authors provided a nearly complete characterization of the release strategy using the following model.
dωdt=aω2ω+g−μω−ξ(ω+g)ω | (1.1) |
where ω(t) and g(t) represent the number of wild mosquitoes and the number of sterile male mosquitoes in their sexually active period. The birth rate per wild mosquito, denoted by a, follows logistic growth. Additionally, ξ and μ represent the density-dependent and independent death rates, respectively.
We know that mosquito growth includes three aquatic stages, namely egg, larva, and pupa, as well as an adult stage. Intraspecific competition significantly affects the aquatic stages, while the adult stage is rarely affected. Building on model (1.1), the authors in [30] proposed and studied a stage-structured model for suppressing mosquito populations as follows:
{dJdt=βA2A+g−αJ−(μ0+ξJ)J,dAdt=αJ−μ1A, | (1.2) |
where J and A represent the number of wild mosquitoes in the aquatic and adult stages, respectively, while g represents the number of sterile males. The birth rate of adults is denoted by β. μi and ξ represent the natural mortality and intraspecific competition coefficient, respectively, where i=0,1. The parameter α represents the emergence rate from larvae to adults.
As mentioned earlier, in order to suppress the wild mosquito population, male sterile mosquitoes are released in most scenarios. In addition, the role of female and male mosquitoes in interacting with sterile mosquitoes is different. Motivated by the previous research works, this paper aims to further investigate and develop a wild mosquito suppression model with both stage and sex structure.
The paper is organized as follows: In Section 2, we develop a mosquito population suppression model with both stage and sex structure. The model is a time-switched system with the number of sexually active sterile males serving as a control function. In Section 3, we first define two important thresholds for the release amount and then determine the conditions for the stability of the wild mosquito extinction equilibrium and the existence of positive periodic solutions. In Section 4, we provide numerical results to validate our theoretical findings. Finally, we conclude with a brief summary in Section 5.
L. Almeida et al. in [31] describes the dynamics of the mosquito population as follows
{dEdt=βEF(1−EK)−(νE+δE)E,dLdt=τEE−L(cL+τE+δL),dPdt=τLL−(τP+δP)P,dFdt=ντPP−δFF,dMdt=(1−ν)τPP−δMM, | (2.1) |
where E(t), L(t), P(t), F(t) and M(t) stands for the numbers of eggs, larvae, pupa, adult females and adult males at time t, respectively. The parameters βE, K, and τE represent the oviposition rate, environmental capacities and hatching rate for eggs, respectively. Pupas will develop into males or females, and ν∈(0,1) reflects the corresponding proportion. τL, τP are transition rates, c is the intraspecific competition of larvae, and δE, δL, δP, δF and δM are the corresponding death rates.
To simplify the mosquito population model, we make the following assumptions, similar to those in [31]: (i) the dynamics of larvae and pupae are fast, and intraspecific competition at the larvae stage is negligible (i.e.,c≪1); (ii) the probability of a pupa developing into a female is the same as that of a male (ν=12); (iii) male and female mosquitoes have the same death rate (δF=δM).
With these assumptions and the third and fourth functions of system (2.1), we obtain the equilibrium equations for larvae and pupae, which are:
P=τLτEE(τP+δP)(τL+δL),L=τEEτL+δL. | (2.2) |
After substituting Eq (2.2) into system (2.1) and introducing a release function u(⋅) for sterile male mosquitoes, we can derive the following interactive dynamical system describing the population dynamics of two kinds of mosquitoes
{dEdt=βEF(1−EK)FF+γMs−(νE+δE)E,dFdt=νβFE−δFF,dMsdt=u(⋅)−δMM, | (2.3) |
where Ms(t) is the number of sterile males in the field, γ measures the mating competitiveness of sterile males, and βF=τPτLτE(τP+δP)(τL+δL).
In this paper, we also only consider sexually active sterile mosquitoes in the dynamics. Let Ms(t) be the number of sexually active sterile males at time t and ignore their natural death as in previous works [15,18,26,27,28,29]. By taking Ms(t) as a control function, we convert the system (2.1) into
{dEdt=βEF(1−EK)FF+γMs−(νE+δE)E,dFdt=νβFE−δFF. | (2.4) |
Let T be the waiting time between two consecutive release, and sterile mosquitoes are released with an amount of m at time t=kT, k=0,1,2,⋯. We use ˉT to represent the sexual lifespan of sterile mosquitoes, ignoring their natural death as in [15,18,26,27,28,29]. There are three possible cases for T and ˉT: T=ˉT, T>ˉT, and T<ˉT. Previous works [30] studied the effect of releasing sterile mosquitoes on wild mosquito populations in the first two cases.
In this work, we mainly study the last case: T<ˉT. In fact, experimental studies have indicated that the lifespan of sterile mosquitoes can be as long as 51 days [32] such as the Wolbachia-infected mosquitoes, and their sexual lifespan can reach 14 days [33]. Nonetheless, the release of sterile mosquitoes is relatively frequent, such as in the experimental field of Shazai Island in Guangzhou, China, where they were released three times a week [32]. Hence, it is meaningful to consider the situation where the sexual lifespan of sterile mosquitoes exceeds the release period, that is T<ˉT.
Similar to the discussion in [17], when T<ˉT, there must exist a unique positive integer p and a non-negative number q that satisfy
ˉT=pT+q, | (2.5) |
where q∈[0,T) and p=[ˉT/T], which is the integer closest to ˉT/T and not greater than ˉT/T. Since pT≤ˉT, we can obtain the first p releases
Ms(t)=(k+1)m,t∈(kT,(k+1)T] |
where k=0,1,2,⋯,p−1. From the (p+1)th release, it is easy to get
Ms(t)={(p+1)mkT<t≤kT+q,pmkT+q<t≤(k+1)T,ifq≠0, |
where k=p,p+1,⋯. If q=0, then Ms(t)=pm, and the characteristics of the system are completely consistent with the simple system of constant value continuous release. In the present work, we assume that 0<q<T. As we are studying the asymptotic behavior of the system, for the sake of analysis convenience and without loss of generality, we can further assume that for k=0,1,2,⋯,
Ms(t)={(p+1)mkT<t≤kT+q,pmkT+q<t≤(k+1)T. | (2.6) |
Based on the above assumption, the model (2.4) is transformed as follows:
{dEdt=βEF(1−EK)FF+γ(p+1)m−(τE+δE)E,dFdt=νβFE−δFF,t∈(kT,kT+q], | (2.7) |
{dEdt=βEF(1−EK)FF+γpm−(τE+δE)E,dFdt=νβFE−δFF,t∈(kT+q,(k+1)T], | (2.8) |
where k=0,1,2,⋯.
Population dynamics of wild mosquitoes with sterile mosquito release then depend on the continuous switching between systems (2.7) and (2.8). In this paper, based on the switched systems (2.7) and (2.8), we mainly investigate the extinction and periodic change of wild mosquito population.
First, we consider the dynamic behavior of the wild mosquito population without the release of sterile mosquitoes. Let Ms(t)≡0, then the system (2.4) or (2.7) and (2.8) can be rewritten as follows:
dEdt=βEF(1−EK)−(τE+δE)E,dFdt=νβFE−δFF. | (3.1) |
Let Ω={(E,F)|0≤E≤K,0≤F}. Obviously, Ω is a positive invariant set of systems (3.1), (2.7) and (2.8), and every solution of systems (3.1), (2.7) and (2.8) with nonnegative initial values is positive and bounded. Therefore, in this work, we will investigate the dynamics of these systems in Ω. Additionally, it is easy to verify that system (3.1) is a monotonic system on Ω, so there are no closed orbits. By defining the basic offspring number of the wild mosquito as
R0:=νβEβFδF(τE+δE), |
we list the following conclusions about the system (3.1):
Lemma 1. (i) If R0≤1, then the extinction equilibrium A0(0,0) is the unique equilibrium of system (3.1) that is globally asymptotically stable in Ω.
(ii) If R0>1, then A0(0,0) is unstable, and the unique positive equilibrium A∗(E∗,F∗) of system (3.1) is globally asymptotically stable in Ω, where
E∗=(1−δF(τE+δE)νβFβE)K=(1−1R0)K,F∗=νβFδF(1−1R0)K. |
According to the necessity of releasing sterile mosquitoes, we will only consider the case R0>1 in this paper.
For the convenience of illustration, the following system is introduced to uniformly express the two subsystems (2.7) and (2.8)
{dEdt=βEF(1−EK)FF+γ˜pm−(τE+δE)E,dFdt=νβFE−δFF, | (3.2) |
where ˜p=p+1 or p.
To compute the jacobian matrix of the system (3.2), we obtain
J=(−βEF2(F+γ˜pm)K−(τE+δE)βE(1−EK)(Fγ˜pm+F+γ˜pmF(γ˜pm+F)2)νβF−δF). | (3.3) |
It has non-negative extra-diagonal coefficients on Ω={(E,F)|0≤E≤K,0≤F}, indicating that the system (3.2) is monotone on Ω.
Clearly, the wild mosquito extinction equilibrium A0(0,0) is an equilibrium of both systems (2.7) and (2.8). By using the general form (3.2) of systems (2.7) and (2.8), we can now calculate the positive equilibria by finding the positive roots of the following equations,
E=δFνβFF,βEF(1−EK)FF+γ˜pm−(τE+δE)E=0, |
which can be further equivalently transformed into
G(F)=−R0δFF2KνβF+(R0−1)F−γ˜pm=0. | (3.4) |
It is easy to know that the discriminant of the above equation is
Δ=(R0−1)2−4γ˜pmβEK(τE+δE), | (3.5) |
then we define the following release threshold for the system (3.2)
m∗(˜p)≡K(R0−1)2(τE+δE)4γβE˜p. | (3.6) |
Lemma 2. Let R0>1. The trivial equilibrium A0 of system (3.2) is always locally asymptotically stable. Moreover,
(i) There exists m∗(˜p)>0 such that the system (3.2) admits no positive equilibrium if m>m∗(˜p), one positive equilibrium if m=m∗(˜p), and two positive equilibria A⋅j(E⋅j(˜p),F⋅j(˜p)),j=1,2 if 0<m<m∗(˜p), where 5
F⋅j(˜p)=(R0−1)∓√(R0−1)2−4γ˜pmβEK(τE+δE)2βEK(τE+δE),E⋅j=δFF⋅j(˜p)νβF,j=1,2. |
(ii) If m>m∗(˜p), then the trivial equilibrium A0 is globally asymptotically stable in Ω. If 0<m<m∗(˜p), then A⋅1(E⋅1(˜p),F⋅1(˜p)) is unstable, while A⋅2(E⋅2(˜p),F⋅2(˜p)) is locally asymptotically stable. If m=m∗(˜p), then the unique positive equilibrium is a saddle node.
Proof. To calculate the Jacobian matrix of the system (3.2) at the trivial equilibrium A0(0,0), we have
JA0=(−(τE+δE)0νβF−δF). |
It has two negative eigenvalues, therefore the trivial equilibrium A0 is always locally asymptotically stable.
(i) By calculating the discriminant Δ in Eq (3.5), we can see that Δ=0 when m=m∗(˜p), Δ<0 when m>m∗(˜p), and Δ>0 when 0<m<m∗(˜p). Moreover, the root of the quadratic equation (3.4) must be positive roots if it exists. These observations lead to the conclusions in (i).
(ii) Since the system (3.2) is monotone on Ω, it has no closed orbits. If m>m∗(˜p), then the extinction equilibrium A0 is the unique equilibrium which is stable, so it is globally asymptotically stable in Ω.
If 0<m<m∗(˜p), we calculate Jacobian matrix of the system (3.2) at A⋅j,j=1,2 by using jacobian matrix (3.3) and get
JA⋅j=(−νβEβFF⋅jδF(γ˜pm+F⋅j)βE(1−δFF⋅jKνβF)(F⋅jγ˜pm+F⋅j+γ˜pmF⋅j(γ˜pm+F⋅j)2)νβF−δF). |
We can easily get trJA⋅j=−νβEβFF⋅jδF(m1+F⋅j)−δF<0 and
|JA⋅j|=νβEβFF⋅jγ˜pm+F⋅j−[νβEβFF⋅jγ˜pm+F⋅j+νβEβFγ˜pmF⋅j(γ˜pm+F⋅j)2−βEδFF2⋅jK(γ˜pm+F⋅j)−βEδFγ˜pmF2⋅jK(γ˜pm+F⋅j)2]=βEδFγ˜pmF2⋅jK(γ˜pm+F⋅j)2+βEδFF2⋅jK(γ˜pm+F⋅j)−νβEβFγ˜pmF⋅j(γ˜pm1+F⋅j)2=−νβEβFR0F⋅jΦ′(F⋅j), | (3.7) |
where Φ(F)=−G(F)γ˜pm+F.
Since −Φ′(F)=−G(F)(γ˜pm+F)2+G′(F)(γ˜pm+F), G(F⋅1)=G(F⋅2)=0 and G′(F⋅1)>0,G(F⋅2)<0, we can obtain |J(A⋅1)|<0 and |J(A⋅2)|>0. Therefore, A⋅1(E⋅1,F⋅1) is an unstable saddle, while A⋅2(E⋅2,F⋅2) is locally asymptotically stable.
If the release amount m increases to m=m∗(˜p), then the two positive equilibria A⋅1 and A⋅2 merge into a single semi-stable saddle node. The proof is completed.
Remark 1. According to Lemma 2, the constant continuous release system (3.2) has a threshold m(˜p) for the release amount m. When the release amount m>m(˜p), the wild mosquito population in the field will be successfully suppressed.
Referring to the two subsystems (2.7) and (2.8), we denote their corresponding release thresholds as m∗1 and m∗2, respectively, given by:
m∗1=m∗(p+1)<m∗2=m∗(p). | (3.8) |
When there are two positive equilibria, the positive equilibria corresponding to systems (2.7) and (2.8) are denoted by A1j(E1j,F1j) and A2j(E2j,F2j),j=1,2, respectively, where
E1j=E⋅j(p+1),F1j=F⋅j(p+1),E2j=E⋅j(p),F2j=F⋅j(p),j=1,2. |
Lemma 2 provides insights into the behavior of systems (2.7) and (2.8). We can now state the following result:
Lemma 3. Let R0>1. The trivial equilibrium A0 of system (2.7) (or (2.8)) is always locally asymptotically stable. In addition,
(i) If 0<m<m∗1, system (2.7) (or (2.8)) has two positive equilibria A11(E11,F11) and A12(E12,F12) (or A21(E21,F21) and A22(E22,F22)), where A11(E11,F11) (or A21(E21,F21)) is unstable, and A12(E12,F12) (or A22(E22,F22)) is locally asymptotically stable.
(ii) If m∗1<m<m∗2, system (2.8) has two positive equilibria A21(E21,F21) and A22(E22,F22), where A21(E21,F21) is unstable, and A22(E22,F22) is locally asymptotically stable. However, system (2.7) has no positive equilibrium.
(iii) If m>m∗2, both systems (2.7) and (2.8) have no positive equilibrium, and the unique equilibrium A0(0,0) is globally asymptotically stable in Ω.
When the release period of sterile mosquitoes is shorter than their sexual lifespan, that is, T<ˉT, the number of sterile mosquitoes that are sexual active will continually switch between two levels, as shown in function (2.6). The population dynamics of wild ones will then depend on the switched systems (2.7) and (2.8).
With the appearance of continual switching, the extinction equilibrium A0(0,0) becomes the unique equilibrium of the systems (2.7) and (2.8). We will first discuss its stability.
Since R0=νβEβFδF(τE+δE)>1, βF=τPτLτE(τP+δP)(τL+δL) and ν∈(0,1), we get νβEβF>δF(τE+δE) and βF<τE, and then τE+δE>νβF. Let
α:=min{(τE+δE)−νβF,δF}, |
then for any given 0<σ<1, denote
ℓ:=γ(p+1)mασβE,ℓ1:=γpmασβE. |
Theorem 1. Assuming R0>1 and ˉT=pT+q, for any solution (E(t),F(t)) of systems (2.7) and (2.8) with 0<E(0)+F(0)<ℓ1, the inequality
0≤E(t)+F(t)≤(E(0)+F(0))e−rt,t>0, | (3.9) |
holds, where r=(1−σ)α>0. Thus, the extinction equilibrium A0(0,0) of systems (2.7) and (2.8) is exponentially asymptotically stable.
Proof. Suppose (E(t),F(t)) is a solution of the systems (2.7) and (2.8) in Ω with 0<E(0)+F(0)<ℓ1 for fixed σ∈(0,1). Let P(t):=E(t)+F(t) for t≥0. According to the first subsystem (2.7), we have for t∈[kT,kT+q], k=0,1,2,⋯,
P′(t)=βEF(1−EK)FF+γ(p+1)m−(τE+δE)E+νβFE−δFF≤βEF2γ(p+1)m−[(τE+δE)−νβF]E−δFF≤βEγ(p+1)mP2(t)−αP(t)=[βEγ(p+1)mP(t)−α]P(t). | (3.10) |
We claim that if P(kT)<ℓ1<ℓ, then P(t)<ℓ1 for all t∈[kT,kT+q], k=0,1,2,⋯. Let's prove this by contradiction. Suppose it does not holds, then there must exist ˉt∈(kT,kT+q] such that P(t)<ℓ1<ℓ for t∈[kT,ˉt) and P(ˉt)=ℓ1, then P′(ˉt)≥0. However, from inequality (Eq 3.10), we deduce
P′(ˉt)≤(βEγ(p+1)mℓ1−α)ℓ1=−(1−pp+1σ)αℓ1<0, |
which leads a contradiction. Therefore, we have P(t)<ℓ1 for all t∈[kT,kT+q] and P′(t)≤(βEγ(p+1)mℓ1−α)P(t)=−(1−pp+1σ)αP(t)<0. Then we can further deduce
P(t)≤P(kT)e−(1−pp+1σ)α(t−kT)<P(kT)e−(1−pp+1σ)αq,t∈[kT,kT+q). | (3.11) |
Similarly, for the second subsystem (2.8), we can obtain
P′(t)≤[βEγpmP(t)−α]P(t),t∈[kT+q,(k+1)T). |
Then if P(kT+q)<ℓ1, then P(t)<ℓ1 for all t∈[kT+q,(k+1)T], k=0,1,2,⋯. In addition,
P(t)≤P(kT+q)e−(1−σ)α(t−kT)<P(kT+q)e−(1−σ)α(T−q),t∈[kT+q,(k+1)T). | (3.12) |
Combining with inequalities (Eq 3.11) and (Eq 3.12), we know that
P(t)≤P(kT)e−(1−pp+1σ)αqe−(1−σ)α(T−q)=ξP(kT),t∈[kT+q,(k+1)T), |
where ξ:=e−(1−σ)αT<1.
Thus,
P((k+1)T)≤ξP(kT)andP(kT)<ξkP(0),k=0,1,2,⋯. |
If t∈[kT,kT+q], then from inequality (Eq 3.11) we get
P(t)≤P(0)ξke−(1−pp+1σ)α(t−kT)≤P(0)e−((1−σ)α)t=P(0)e−rt,t∈[kT,kT+q]. | (3.13) |
If t∈[kT+ˉT,(k+1)T], then from inequality (Eq 3.12) we get
P(t)≤P(0)ξke−(1−pp+1σ)αqe−(1−σ)α(t−(kT+q))≤P(0)e−rt,t∈[kT+q,(k+1)T]. | (3.14) |
By combining inequalities (Eq 3.13) and (Eq 3.14), we can see that the inequality (Eq 3.9) holds and A0(0,0) is exponentially asymptotically stable. The proof is completed.
In Theorem 1, we show the exponential asymptotic stability of A0(0,0). However, this stability is only a local feature. Due to the periodic release of sterile mosquitoes, we are concerned about the possibility of a periodic change in the wild mosquito population, specifically, whether the systems (2.7) and (2.8) has a positive periodic solution. In the following discussion, we focus on the existence of positive periodic solutions.
Based on Lemma 3, when R0>1, T<ˉT, and 0<m<m∗1, both subsystems (2.7) and (2.8) have a pair of positive equilibria, namely A11(E11,F11), A12(E12,F12) and A21(E21,F21), A22(E22,F22). It is easy to verify that F22>F12>F11>F21>0 and K>E22>E12>E11>E21>0. Let Ω0:=[E12,E22]×[F12,F22] be a rectangle in the EF-phase plane. Then we can get the following result.
Theorem 2. If R0>1 and 0<m<m∗1, then the systems (2.7) and (2.8) has a T-periodic solution in Ω0. Suppose (E(t),F(t)) is a solution of the systems (2.7) and (2.8) with E(t0)≥E11 and F(t0)≥F11 for some t0≥0, then E(t)≥E11 and F(t)≥F11 for all t>t0, and dist((E(t),F(t)),Ω0)→0 as t→∞. Moreover, if such a solution is T-periodic, then (E(t),F(t))∈Ω0 for all t≥0.
Proof. For the conditions R0>1, T<ˉT, and 0<m<m∗1, we obtain the existence of Aij, i,j=1,2. Let η(E):=KE(τE+τE)K−E, E∈[0,K]. The vertical isoclines E′(t)=0 of systems (2.7) and (2.8) in the first quadrant of the EF-phase plane are given by
F=L1(E)=12βE[η(E)+√η2(E)+4γm(p+1)βEη(E)],F=L2(E)=12βE[η(E)+√η2(E)+4γmpβEη(E)], |
respectively (see Figure 1). Since L1(E)>L2(E), the curve of F=L1(E) is strictly above the curve of F=L2(E) for E>0. Additionally, F=G(E):=νβEEδF is the shared horizontal isocline of systems (2.7) and (2.8).
Select any two positive numbers Em and EM satisfying E11<Em<E12<E22<EM<K, then Fm=G(Em) and FM=G(EM). Due to the strict monotonic increase property with respect to E of G(E), it follows that Fm<FM. Let Ω1 be the closed rectangle with the vertices P1,P2,Q1 and Q2, where P1=(Em,Fm), P2=(EM,Fm), Q1=(Em,FM), and Q2=(EM,FM).
Clearly, F11<Fm<F12<F22<FM and Ω0⊂Ω1. Since G(E) is strictly monotone increasing with respect to E, the segment ¯P1P2 lies strictly below the isocline F=G(E) except for the point P1, while the segment ¯Q1Q2 lies strictly above the isocline F=G(E) except for the point Q2. According to the second equation of systems (2.7) and (2.8), we have
dFdt=δF(G(E)−F)>δF(G(Em)−Fm)=0,E∈[Em,EM],dFdt=δF(G(E)−F)<δF(G(EM)−FM)=0,E∈[Em,EM). |
Thus, the vector fields of (2.7) and (2.8) point into the closed rectangle Ω1 on ¯P1P2 and ¯Q1Q2.
In addition, since L1(E) and L2(E) are both strictly monotone increasing with respect to E on [0,K], we can see that the entire segment ¯P1Q1 lies strictly above the isocline F=L1(E), and the entire segment ¯P2Q2 lies strictly below the isocline F=L2(E). From system (2.7), we obtain
dEdt=βEF+γ(p+1)m(1−EK)(F2−γ(p+1)m+FβEη(E))=βEγ(p+1)m(1−EK)(F−L1(E))(F+L−1(E)), |
where L−1(E)=12βE[−η(E)+√η(E)2+4γ(p+1)mβEη(E)]>0.
From the system (2.8), we can obtain
dEdt=βEF+γpm(1−EK)(F2−γpm+FβEη(E))=βEγpm(1−EK)(F−L2(E))(F+L−2(E)) |
where L−2(E)=12βE[−η(E)+√η(E)2+4γpmβEη(E)]>0.
Clearly, on the segment ¯P1Q1, we have E≡Em, so F>L1(Em) and F>L2(Em). As a result, dEdt>0 holds for both systems (2.7) and (2.8) on ¯P1Q1. Similarly, on the segment ¯P2Q2, we have F<L1(EM) and F<L2(EM), leading to dEdt<0 for both systems (2.7) and (2.8) on this segment. Therefore, the vector fields of systems (2.7) and (2.8) point towards the inside of Ω1 on both ¯P1Q1 and ¯P2Q2.
In summary, Ω1 represents a positive invariant set of the switched systems (2.7) and (2.8), and any point A∈Ω1 will have the trajectory of the systems (2.7) and (2.8) starting from it stay within Ω1.
We define a map Φ:Ω1→Ω1 as given in [30]:
Φ(A):=(ψT−q∘ϕq)(A)=ψT−q(ϕq(A)) | (3.15) |
Here, ϕt(A) and ψt(A) are the solutions of systems (2.7) and (2.8) starting from the point A, respectively. Since Φ is continuous and maps Ω1 to Ω1, by Brouwer's fixed point theorem, we know that Φ has a fixed point ˉA∈Ω1. Then, the solution of systems (2.7) and (2.8) passing through the point ˉA is a continuous T-periodic solution whose trajectory lies entirely in Ω1.
It is worth noting the arbitrariness of Em and EM. If Em→E12 and EM→E22, then Ω1→Ω0, and the continuous T-periodic solution of systems (2.7) and (2.8) in Ω1 lies in Ω0. If Em→E11 and EM→∞, then Ω1→[E11,∞)×[F11,∞). Since Ω1 is an attraction domain, [E11,∞)×[F11,∞) is also a positively invariant set of systems (2.7) and (2.8). Then, for any solution of systems (2.7) and (2.8) with E(t0)≥E11 and F(t0)≥F11, it follows that E(t)≥E11 and F(t)≥F11 for all t>t0. Moreover, dist((E(t),F(t)),Ω0)→0 as t→∞. Furthermore, if this solution is periodic, then (E(t),F(t))∈Ω0. Thus, the proof is completed.
When we increase the release amount m to exceed the smaller threshold m∗1, that is, m∗1≤m<m∗2, we obtain the following result:
Theorem 3. Suppose R0>1 and m∗1<m<m∗2. Then there exist positive constants T0>0 and δ0>0 such that the systems (2.7) and (2.8) has a continuous and positive T-periodic solution when
q<T0,T−q<δ0. | (3.16) |
Proof. According to Lemma 3, when R0>1, T<ˉT and m∗1<m<m∗2, the subsystem (2.8) has two positive equilibria A21 and A22, while (2.7) has only the extinction equilibrium.
Similar to Theorem 2, we first select two arbitrary positive numbers Em and EM such that 0<Em<E22<EM and G(EM)<L1(Em) (G(E) and Li(E),i=1,2 are defined in the proof of Theorem 2). Let Fm=G(Em) and FM=G(EM). Then we construct a closed rectangle Ω1 with vertices at P1=(Em,Fm), P2=(EM,Fm), Q1=(Em,FM), and Q2=(EM,FM). In addition, let P3 be the intersection point of the segment P1P2 and the isocline F=L2(E), and for any E0∈(Em,E3), denote points P0:=(E0,Fm) and Q0:=(E0,FM) (see Figure 2). Then Ω1 can be regarded as a rectangle composed of two parts, namely, Ω1=Ω11⋃Ω21, where Ω11 is the rectangle with P1, P0, Q1, and Q0 as its vertices, and Ω21 is the rectangle with P0, P2, Q0, and Q2 as its vertices.
We define ω1 and ω2 as follows:
ω1:=min{G1(E,F):(E,F)∈Ω11}<0,ω2:=min{G2(E,F):(E,F)∈Ω11}>0, | (3.17) |
where G1(E,F) and G2(E,F) are the right-hand side functions of the first equations of systems (2.7) and (2.8), respectively.
We assume that
q<T0,T−q>δ0, | (3.18) |
where T0:=E0−Em−ω1, δ0:=E0−Emω2.
In the following, we will prove that the Poincare map Φ defined in Theorem 2 is continuous and maps Ω21 to itself. Then, similarly to the proof of Theorem 2, we can deduce that the systems (2.7) and (2.8) has a continuous and positive T-periodic solution in Ω21.
The vector fields of system (2.7) point towards the interior of Ω1 along the segments ¯P1P2, ¯Q1Q2, and ¯P2Q2. Therefore, for any solution of system (2.7) with initial value (E(0),F(0))∈Ω21, it can only cross the segment ¯P0Q0 if it leaves Ω1. We will now show that this is impossible by contradiction.
Suppose there exists a time t0∈[0,q) such that (E(t0),F(t0))∈¯P0Q0 and (E(t),F(t))∈Ω21 for t∈[0,t0). Then, we have E′(t0)<0, which means that the solution immediately enters Ω11 after t0. When the solution leaves Ω11, it can only go through the segment ¯P1Q1.
Now, assume there exists a time ˜t∈(t0,q] such that (E(˜t),F(˜t))∈¯P1Q1 and (E(t),F(t))∈Ω11 for t∈[t0,˜t). Then, we have E′(t)=G1(E(t),F(t))≥ω1 for t∈[t0,˜t], and E(˜t)=Em≥E(t0)+ω1(˜t−t0)≥E0+ω1q. Since ω1<0 and q<T0, we have Em<E0+ω1T0, which is a contradiction. Therefore, any solution (E(t),F(t)) of system (2.7) with (E(0),F(0))∈Ω21 will remain in Ω1 for t∈[0,q].
Next, we show that for any solution of system (2.8) with (E(q),F(q))∈Ω1, the solution will stay in Ω1 for t∈[q,T] with (E(T),F(T))∈Ω21. Noting that the vector fields of system (2.8) point to the inside of both Ω1 and Ω21, we see that both the rectangles Ω1 and Ω21 are positive invariant sets of system (2.8). Therefore, (E(q),F(q))∈Ω1 implies (E(t),F(t))∈Ω1 for all t∈[q,T]. Furthermore, we can claim that (E(T),F(T))∈Ω21. If this does not hold, then (E(t),F(t))∈Ω11 for all t∈[q,T] due to the properties of the invariant set Ω21. This implies that E′(t)≥ω2 for t∈[q,T] and E(T)≥E(q)+ω2(T−q). We further deduce that E0−Em≥E(T)−E(q)≥ω2(T−q), which contradicts the inequality (3.18).
In summary, we have shown that for any solution (E(t),F(t)) of systems (2.7) and (2.8) starting from the initial point (E(0),F(0))∈Ω21, the solution will stay in Ω1 for t∈[0,T] and (E(T),F(T))∈Ω21. Thus, the Poincare map Φ:Ω21→Ω21 is well-defined and has at least one fixed point A∗ in Ω21. Then the solution of systems (2.7) and (2.8) through the point A∗ is a continuous and positive T-periodic solution. The proof is completed.
Remark 2. If m=m∗1, then the two equilibria A11(E11,F11) and A12(E12,F12) of (2.7) coincide into one, denoted by A∗1c(E∗1c,F∗1c). By slightly modifying the proof of Theorem 3.3 and selecting values of Em and EM such that E∗1c<Em<E22<EM and G(EM)<L1(Em), we can similarly prove that the systems (2.7) and (2.8) has a positive T-periodic solution.
Next, we consider the case where the release amount m is further increased until m≥m∗2, and we present the following result.
Theorem 4. If R0>1 and m>m∗2, then the unique extinction equilibrium A0 of systems (2.7) and (2.8) is globally asymptotically stable.
Proof. According to Theorem 1, if R0>1 and m>m∗2, the extinction equilibrium A0(0,0) of systems (2.7) and (2.8) is locally asymptotically stable. We will now prove that it is also globally attractive.
To this end, we construct the following system:
{d˜Edt=βE˜F(1−˜EK)˜F˜F+γpm−(τE+δE)˜E,d˜Fdt=νβF˜E−δF˜F,t≥0. | (3.19) |
Similar to the discussion about system (3.1), we can show that system (3.19) is monotone on Ω. Furthermore, we have
dEdt≤d˜Edt,dFdt≤d˜Fdt,t∈(kT,(k+1)T],k=0,1,2,⋯. |
Thus, system (3.19) can be regarded as a comparison system for systems (2.7) and (2.8). With the same initial values ˜E(0)=E(0) and ˜F(0)=F(0), we obtain
0≤E(t)≤˜E(t),0≤F(t)≤˜F(t),t≥0. |
From Lemma 2, we know that if R0>1 and m>m∗2, the trivial equilibrium (0,0) of system (3.19) is globally asymptotically stable. That is, for any non-negative initial value (˜E(0),˜F(0)), the solution of system (3.19) from it satisfies
limt→∞˜E(t)=0,limt→∞˜F(t)=0. |
By the comparison theorem, we can easily see that for any non-negative initial value (E(0),F(0)), the solution of systems (2.7) and (2.8) from it satisfies
limt→∞E(t)=0,limt→∞F(t)=0. |
Thus, the extinction equilibrium A0(0,0) of systems (2.7) and (2.8) is also globally attractive. The proof is completed.
Remark 3. If m=m∗2, then the extinction equilibrium A0(0,0) of system (2.7) is globally asymptotically stable, meaning that every solution of system (2.7) will tend to the extinction equilibrium A0(0,0). Moreover, the two equilibria A21(E21,F21) and A22(E22,F22) of system (2.8) coincide into one, denoted by A∗2c(E∗2c,F∗2c). According to Lemma 2, this case presents a possible semi-stability, where the basin of attraction of A0(0,0) contains the interval [0,A∗2c), denoted as {(E,F)∈R+2:0≤E<E∗2c,0≤F<F∗2c}, and the basin of attraction of A∗2c(E∗2c,F∗2c) contains the interval (A∗2c,∞), denoted as {(E,F)∈R+2:E>E∗2c,F>F∗2c}. Due to the continual switching between two subsystems (2.7) and (2.8), any solution of systems (2.7) and (2.8) must enter [0,A∗2c) after some time and will eventually tend to the extinction equilibrium A0(0,0). Therefore, the extinction equilibrium A0(0,0) of systems (2.7) and (2.8) is globally asymptotically stable.
Remark 4. According to Theorems 1–4 and Remark 3, the periodic and impulsive release systems (2.7) and (2.8) has a threshold m∗2 for the release amount m, and only when the release amount m≥m∗2, can the wild mosquito population in the field be successfully suppressed.
This section aims to illustrate the theoretical results developed in the previous section through several numerical examples. We use the values of most model parameters from [31,34], which are summarized in Table 1.
Parameters | Value | Unit | Parameters | Value | Unit |
βE | 10 | day−1 | γ | 1 | - |
τE | 0.05 | - | δE | 0.03 | day−1 |
δF | 0.04 | day−1 | βF | 0.01 | day−1 |
ν | 0.5 | - |
Another important parameter to be determined is the carrying capacity, denoted by K. We consider an island with an area of 74 hectares, as in [31,34], with an estimated male population of about 69 males per hectare. If there are no sterile mosquitoes in the field and the wild population reaches a stable state, the number of adult wild males is assumed to be M∗=F∗=69×74=5106. Then, the number of eggs at the stable state is estimated as E∗=δFF∗νβF=40848, and the carrying capacity can be calculated as
K=E∗1−(τE+δE)δFνβEβF≈43641. |
For the sexual lifespan of sterile mosquitoes, we take the value from [17], and set ˉT=14. Through direct calculation, we can get
R0:=νβEβFδF(τE+δE)=15.625>1,m∗(˜p)=K(1−ℵ0)2(τE+δE)4βEγ˜p≈18669˜p. |
When no sterile ones are released, the unique positive equilibrium A∗(E∗,F∗) is globally and uniformly asymptotically stable (see Figure 3(a)), which is consistent with the result in Lemma 1.
For the constant release system (3.2), we can take ˜p=4 without loss of generality, yielding m∗(4)≈4667. When 0<m<m∗(4), the system (3.2) displays bistability, as shown in Figure 3(b), where the extinction equilibrium A0 and a positive equilibrium are locally stable. Increasing the release amount to m=m∗(4) results in a unique positive equilibrium that is semi-stable (see Figure 3(c)). However, if m>m∗(4), then the unique equilibrium A0 becomes globally stable (see Figure 3(d)).
For the switched systems (2.7) and (2.8), textcolor{red}{we choose the release period T=3 }and set ˉT=pT+q with p=4 and q=2. After a simple calculation, we obtain m∗1=m∗(p+1)≈3735 and m∗2=m∗(p)≈4667.
Firstly, let m=3250<m∗1 and we note that the systems (2.7) and (2.8) displays bistability. In addition to the locally stable extinction equilibrium, there also exists a stable positive periodic solution (see Figure 4 (a)). This is consistent with the conclusions of Theorems 1 and 2. As shown in Figures 4(b, c), when the release amount increases to m∗1<m<m∗2, the systems (2.7) and (2.8) continues to exhibit bistability, which is consistent with the conclusions of Theorems 1 and 3. Furthermore, we can see that the attraction region of the extinction equilibrium increases with the release amount m until the release amount is not less than m∗2. If m<m∗2, the extinction equilibrium becomes globally attractive (see Figure 4(d)).
In this work, we constructed and analyzed a two-dimensional switched system of the wild mosquito population with stage structure and periodic releases of sterile mosquitoes. To consider the role of sterile mosquitoes in the interaction between the two mosquito populations, we referred to some previous studies and only included sexually active sterile males in the system. Existing research data suggests that the sexual lifespan ˉT of sterile mosquitoes can be shorter or longer than their release period T, and in [30], the authors studied the case ˉT≤T. Therefore, we focused on investigating the case ˉT>T based on the switched systems (2.7) and (2.8).
We first investigated the non-release system (3.1) and the general form (3.2) of the two subsystems. We gave conditions for the existence and stability of their equilibria (Lemmas 2 and 3), which prepared for the later study of the switched system. Two important release amount thresholds (m∗1 and m∗2) were determined. Then, we proved that the extinction equilibrium A0(0,0) of systems (2.7) and (2.8) must be exponentially asymptotically stable (Theorem 1). This differs from the conclusion in [30] when ˉT<T since, in the latter case, the extinction equilibrium may be unstable. As systems (2.7) and (2.8) has no positive equilibrium, we further discussed the existence of positive periodic solutions. In Theorems 2 and 3, through the analysis of vector fields and using the fixed-point theorem, we show that systems (2.7) and (2.8) has a positive periodic solution when 0<m<m∗1 and m∗1≤m<m∗2. Further increasing the release of sterile mosquitoes, and when m≥m∗2, we confirmed that the extinction equilibrium A0(0,0) of systems (2.7) and (2.8) becomes globally asymptotically stable. This is a previously unconfirmed dynamic phenomenon when studying the case of ˉT<T.
Regarding the periodic switched systems (2.7) and (2.8), due to the complexity of its model construction, we only prove the existence of positive periodic solutions using the fixed-point theorem. However, neither the number nor the stability of the positive periodic solutions are determined. In future research, we will try more methods to solve these problems, such as constructing Lyapunov functions to study the stability of periodic solutions [35,36,37]. In addition, we mainly focus on the theoretical study and the validation of the related results in this work. For the numerical analysis, we used data from a mosquito population on a 74-hectare island. Due to the relatively small number of wild mosquitoes on the island, the amplitude of the periodic fluctuation in the mosquito population is indeed small. In future research, we will collect and organize relevant data from other scenarios with large mosquito populations, and apply our research results to the development of management strategies for wild mosquito populations.
This work is supported by the National Natural Science Foundation of China (11901502), Program for Science & Technology Innovation Talents in Universities of Henan Province (21HASTIT026), Program for Innovative Research Team (in Science and Technology) in University of Henan Province (21IRTSTHN014) and Graduate Research Innovation Fundation of XYNU(2022KYJJ055).
The authors declare no conflicts of interest in this paper.
[1] |
Agyeman SD, Lin BQ (2023) The influence of natural gas (De)regulation on innovation for climate change mitigation: Evidence from OECD countries. Environ Impact Asses Rev 98: 106961. https://doi.org/10.1016/j.eiar.2022.106961 doi: 10.1016/j.eiar.2022.106961
![]() |
[2] |
Aizawa M, Yang CF (2010) Green Credit, Green Stimulus, Green Revolution? China's Mobilization of Banks for Environmental Cleanup. J Environ Dev 19: 119–144. https://doi.org/10.1177/1070496510371192 doi: 10.1177/1070496510371192
![]() |
[3] |
Athey S, Imbens GW (2022) Design-based analysis in Difference-In-Differences settings with staggered adoption. J Econometrics 226: 62–79. https://doi.org/10.1016/j.jeconom.2020.10.012 doi: 10.1016/j.jeconom.2020.10.012
![]() |
[4] |
Bao T, Dai Y, Feng YX, et al. (2023) Trade liberalisation and trade and capital flows: Evidence from China pilot free trade zones. World Econ 46: 1408–1422 https://doi.org/10.1111/twec.13387 doi: 10.1111/twec.13387
![]() |
[5] |
Barney JB, Harrison JS (2020) Stakeholder Theory at the Crossroads. Bus Soc 59: 203–212. https://doi.org/10.1177/0007650318796792 doi: 10.1177/0007650318796792
![]() |
[6] |
Beck T, Levine R, Levkov A (2010) Big Bad Banks? The Winners and Losers from Bank Deregulation in the United States. J Financ 65: 1637–1667. https://doi.org/10.1111/j.1540-6261.2010.01589.x doi: 10.1111/j.1540-6261.2010.01589.x
![]() |
[7] |
Bi KX, Huang P, Wang XX (2016) Innovation performance and influencing factors of low-carbon technological innovation under the global value chain: A case of Chinese manufacturing industry. Technol Forecast Soc 111: 275–284. https://doi.org/10.1016/j.techfore.2016.07.024 doi: 10.1016/j.techfore.2016.07.024
![]() |
[8] | Borusyak K, Jaravel X, Spiess J (2021) Revisiting Event Study Designs: Robust and Efficient Estimation. Unpublished. https://doi.org/10.48550/arXiv.2108.12419 |
[9] |
Braghieri L, Levy R, Makarin A (2022) Social Media and Mental Health. Am Econ Rev 112: 3660–3693. https://doi.org/10.1257/aer.20211218 doi: 10.1257/aer.20211218
![]() |
[10] |
Callaway B, Sant'Anna PHC (2021) Difference-in-Differences with multiple time periods. J Econometrics 225: 200–230. https://doi.org/10.1016/j.jeconom.2020.12.001 doi: 10.1016/j.jeconom.2020.12.001
![]() |
[11] |
Carlson D, Robinson SA, Blair C, et al. (2021) China's climate ambition: Revisiting its First Nationally Determined Contribution and centering a just transition to clean energy. Energ Policy 155: 112350. https://doi.org/10.1016/j.enpol.2021.112350 doi: 10.1016/j.enpol.2021.112350
![]() |
[12] |
Chen ZF, Zhang X, Chen FL (2021) Do carbon emission trading schemes stimulate green innovation in enterprises? Evidence from China. Technol Forecast Soc 168: 120744. https://doi.org/10.1016/j.techfore.2021.120744 doi: 10.1016/j.techfore.2021.120744
![]() |
[13] |
Cheng YY, Xu ZH (2023) Fiscal centralization and urban industrial pollution emissions reduction: Evidence from the vertical reform of environmental administrations in China. J Environ Manage 347: 119212. https://doi.org/10.1016/j.jenvman.2023.119212 doi: 10.1016/j.jenvman.2023.119212
![]() |
[14] |
Cheng ZH, Yu XJ, Zhang Y (2023) Is the construction of new energy demonstration cities conducive to improvements in energy efficiency? Energy 263: 125517. https://doi.org/10.1016/j.energy.2022.125517 doi: 10.1016/j.energy.2022.125517
![]() |
[15] |
Coase RH (1981) The Coase Theorem and the Empty Core - a Comment. J Law Econ 24: 183–187. https://doi.org/10.1086/466980 doi: 10.1086/466980
![]() |
[16] |
Cui L, Wu KJ, Tseng ML (2017) Selecting a remanufacturing quality strategy based on consumer preferences. J Clean Prod 161: 1308–1316. https://doi.org/10.1016/j.jclepro.2017.03.056 doi: 10.1016/j.jclepro.2017.03.056
![]() |
[17] |
De Chaisemartin C, D'Haultfoeuille X (2020) Two-Way Fixed Effects Estimators with Heterogeneous Treatment Effects. Am Econ Rev 110: 2964–2996. https://doi.org/10.1257/aer.20181169 doi: 10.1257/aer.20181169
![]() |
[18] |
Decramer S, Vanormelingen S (2016) The effectiveness of investment subsidies: evidence from a regression discontinuity design. Small Bus Econ 47: 1007–1032. https://doi.org/10.1007/s11187-016-9749-2 doi: 10.1007/s11187-016-9749-2
![]() |
[19] |
Fare R, Grosskopf S, Lovell CAK, et al. (1989) Multilateral Productivity Comparisons When Some Outputs Are Undesirable - a Nonparametric Approach. Rev Econ Stat 71: 90–98. https://doi.org/10.2307/1928055 doi: 10.2307/1928055
![]() |
[20] |
Fazzari SM, Hubbard RG, Petersen BC (1988) Financing Constraints and Corporate-Investment. Brookings Pap Eco Ac 1988: 141–195. https://doi.org/10.2307/2534426 doi: 10.2307/2534426
![]() |
[21] |
Fee CE, Hadlock CJ, Pierce JR (2009) Investment, Financing Constraints, and Internal Capital Markets: Evidence from the Advertising Expenditures of Multinational Firms. Rev Financ Stud 22: 2361–2392. https://doi.org/10.1093/rfs/hhn059 doi: 10.1093/rfs/hhn059
![]() |
[22] |
Forcadell FJ, Aracil E, Ubeda F (2020) Using reputation for corporate sustainability to tackle banks digitalization challenges. Bus Strateg Environ 29: 2181–2193. https://doi.org/10.1002/bse.2494 doi: 10.1002/bse.2494
![]() |
[23] |
Freudenreich B, Luedeke-Freund F, Schaltegger S (2020) A Stakeholder Theory Perspective on Business Models: Value Creation for Sustainability. J Bus Ethics 166: 3–18. https://doi.org/10.1007/s10551-019-04112-z doi: 10.1007/s10551-019-04112-z
![]() |
[24] |
Friedman AL, Miles S (2002) Developing stakeholder theory. J Manage Stud 39: 1–21. https://doi.org/10.1111/1467-6486.00280 doi: 10.1111/1467-6486.00280
![]() |
[25] |
Ge T, Hao XL, Li JY (2021) Effects of public participation on environmental governance in China: A spatial Durbin econometric analysis. J Clean Prod 321: 129042. https://doi.org/10.1016/j.jclepro.2021.129042 doi: 10.1016/j.jclepro.2021.129042
![]() |
[26] |
Gollop FM, Roberts MJ (1983) Environmental-Regulations and Productivity Growth - the Case of Fossil-Fueled Electric-Power Generation. J Polit Econ 91: 654–674. https://doi.org/10.1086/261170 doi: 10.1086/261170
![]() |
[27] |
González X, Pazó C (2008) Do public subsidies stimulate private R & D spending? Res Policy 37: 371–389. https://doi.org/10.1016/j.respol.2007.10.009 doi: 10.1016/j.respol.2007.10.009
![]() |
[28] |
Goodman-Bacon A (2021) Difference-in-differences with variation in treatment timing. J Econometrics 225: 254–277. https://doi.org/10.1016/j.jeconom.2021.03.014 doi: 10.1016/j.jeconom.2021.03.014
![]() |
[29] |
Heckman JJ, Robb R (1985) Alternative Methods for Evaluating the Impact of Interventions - an Overview. J Econometrics 30: 239–267. https://doi.org/10.1016/0304-4076(85)90139-3 doi: 10.1016/0304-4076(85)90139-3
![]() |
[30] |
Hong M, Drakeford B, Zhang KX (2020) The impact of mandatory CSR disclosure on green innovation: evidence from China. Green Financ 2: 302–322. https://doi.org/10.3934/Gf.2020017 doi: 10.3934/Gf.2020017
![]() |
[31] |
Hu GQ, Wang XQ, Wang Y (2021) Can the green credit policy stimulate green innovation in heavily polluting enterprises? Evidence from a quasi-natural experiment in China. Energ Econ 98: 105134. https://doi.org/10.1016/j.eneco.2021.105134 doi: 10.1016/j.eneco.2021.105134
![]() |
[32] |
Huang ZH, Liao GK, Li ZH (2019) Loaning scale and government subsidy for promoting green innovation. Technol Forecast Soc 144: 148–156. https://doi.org/10.1016/j.techfore.2019.04.023 doi: 10.1016/j.techfore.2019.04.023
![]() |
[33] |
Jorgenson DW, Wilcoxen PJ (1990) Environmental-Regulation and United-States Economic-Growth. Rand J Econ 21: 314–340. https://doi.org/10.2307/2555426 doi: 10.2307/2555426
![]() |
[34] |
Kaplan SN, Zingales L (1997) Do investment-cash flow sensitivities provide useful measures of financing constraints? Q J Econ 112: 169–215. https://doi.org/10.1162/003355397555163 doi: 10.1162/003355397555163
![]() |
[35] |
Kouvelis P, Wu XL, Xiao YX (2019) Cash Hedging in a Supply Chain. Manage Sci 65: 3928–3947. https://doi.org/10.1287/mnsc.2017.2997 doi: 10.1287/mnsc.2017.2997
![]() |
[36] |
Lee CC, Nie CF (2023) Place-based policy and green innovation: Evidence from the national pilot zone for ecological conservation in China. Sustain Cities Soc 97: 104730. https://doi.org/10.1016/j.scs.2023.104730 doi: 10.1016/j.scs.2023.104730
![]() |
[37] |
Lee CC, Wang FH, Lou RC, et al. (2023) How does green finance drive the decarbonization of the economy? Empirical evidence from China. Renew Energ 204: 671–684. https://doi.org/10.1016/j.renene.2023.01.058 doi: 10.1016/j.renene.2023.01.058
![]() |
[38] |
Liu J, Xia SM, Wang ZX, et al. (2023) How to balance economic profits and environmental protection: The impacts of cash hedging on remanufacturing firms. Int J Prod Econ 258: 108783. https://doi.org/10.1016/j.ijpe.2023.108783 doi: 10.1016/j.ijpe.2023.108783
![]() |
[39] |
Liu MC, Rao DD, Yang L, et al. (2021) Subsidy, training or material supply? The impact path of eco-compensation method on farmers' livelihood assets. J Environ Manage 287: 112339. https://doi.org/10.1016/j.jenvman.2021.112339 doi: 10.1016/j.jenvman.2021.112339
![]() |
[40] |
Liu XH, Wang EX, Cai DT (2019) Green credit policy, property rights and debt financing: Quasi-natural experimental evidence from China. Financ Res Lett 29: 129–135. https://doi.org/10.1016/j.frl.2019.03.014 doi: 10.1016/j.frl.2019.03.014
![]() |
[41] |
Liu YY, Liu GC, Zhang CS (2021) Local land supply and fiscal incentives for R & D: Evidence from a quasi-natural experiment in China. China Econ Rev 68: 101630. https://doi.org/10.1016/j.chieco.2021.101630 doi: 10.1016/j.chieco.2021.101630
![]() |
[42] |
Ma YC, Sha YZ, Wang ZL, et al. (2023) The effect of the policy mix of green credit and government subsidy on environmental innovation. Energ Econ 118: 106512. https://doi.org/10.1016/j.eneco.2023.106512 doi: 10.1016/j.eneco.2023.106512
![]() |
[43] |
Myers SC, Majluf NS (1984) Corporate Financing and Investment Decisions When Firms Have Information That Investors Do Not Have. J Financ Econ 13: 187–221. https://doi.org/10.1016/0304-405x(84)90023-0 doi: 10.1016/0304-405x(84)90023-0
![]() |
[44] |
Nandy M, Lodh S (2012) Do banks value the eco-friendliness of firms in their corporate lending decision? Some empirical evidence. Int Rev Financ Anal 25: 83–93. https://doi.org/10.1016/j.irfa.2012.06.008 doi: 10.1016/j.irfa.2012.06.008
![]() |
[45] |
Niesten E, Jolink A, Chappin M (2018) Investments in the Dutch onshore wind energy industry: A review of investor profiles and the impact of renewable energy subsidies. Renew Sust Energ Rev 81: 2519–2525. https://doi.org/10.1016/j.rser.2017.06.056 doi: 10.1016/j.rser.2017.06.056
![]() |
[46] |
Oliner SD, Rudebusch GD (1992) Sources of the Financing Hierarchy for Business Investment. Rev Econ Stat 74: 643–654. https://doi.org/10.2307/2109378 doi: 10.2307/2109378
![]() |
[47] |
Pawlina G, Renneboog L (2005) Is investment-cash flow sensitivity caused by agency costs or asymmetric information? Evidence from the UK. Eur Financ Manag 11: 483–513. https://doi.org/10.1111/j.1354-7798.2005.00294.x doi: 10.1111/j.1354-7798.2005.00294.x
![]() |
[48] |
Qin M, Zhang XJ, Li YM, et al. (2023) Blockchain market and green finance: The enablers of carbon neutrality in China. Energ Econ 118: 106501. https://doi.org/10.1016/j.eneco.2022.106501 doi: 10.1016/j.eneco.2022.106501
![]() |
[49] |
Shi XW, Ma JT, Jiang AX, et al. (2023) Green bonds: Green investments or greenwashing? Int Rev Financ Anal 90: 102850. https://doi.org/10.1016/j.irfa.2023.102850 doi: 10.1016/j.irfa.2023.102850
![]() |
[50] |
Sim J (2018) The economic and environmental values of the R & D investment in a renewable energy sector in South Korea. J Clean Prod 189: 297–306. https://doi.org/10.1016/j.jclepro.2018.04.074 doi: 10.1016/j.jclepro.2018.04.074
![]() |
[51] |
Sloczynski T, Wooldridge JM (2018) A General Double Robustness Result for Estimating Average Treatment Effects. Economet Theor 34: 112–133. https://doi.org/10.1017/S0266466617000056 doi: 10.1017/S0266466617000056
![]() |
[52] |
Songailiene E, Winklhofer H, McKechnie S (2011) A conceptualisation of supplier-perceived value. Eur J Marketing 45: 383–418. https://doi.org/10.1108/03090561111107249 doi: 10.1108/03090561111107249
![]() |
[53] |
Su CW, Liu FY, Stefea P, et al. (2023) Does technology innovation help to achieve carbon neutrality? Econ Anal Policy 78: 1–14. https://doi.org/10.1016/j.eap.2023.01.010 doi: 10.1016/j.eap.2023.01.010
![]() |
[54] |
Sueyoshi T, Goto M (2009) Can environmental investment and expenditure enhance financial performance of US electric utility firms under the clean air act amendment of 1990? Energ Policy 37: 4819–4826. https://doi.org/10.1016/j.enpol.2009.06.038 doi: 10.1016/j.enpol.2009.06.038
![]() |
[55] |
Sun LY, Abraham S (2021) Estimating dynamic treatment effects in event studies with heterogeneous treatment effects. J Econometrics 225: 175–199. https://doi.org/10.1016/j.jeconom.2020.09.006 doi: 10.1016/j.jeconom.2020.09.006
![]() |
[56] |
Tan XJ, Dong HM, Liu YS, et al. (2022) Green bonds and corporate performance: A potential way to achieve green recovery. Renew Energ 200: 59–68. https://doi.org/10.1016/j.renene.2022.09.109 doi: 10.1016/j.renene.2022.09.109
![]() |
[57] |
Tatomir M, Dreyer JK, Sund KJ, et al. (2023) Warm-Glow Investing and the Greenwashing Hypothesis. J Clean Prod 419: 138229. https://doi.org/10.1016/j.jclepro.2023.138229 doi: 10.1016/j.jclepro.2023.138229
![]() |
[58] |
Wagner M (2007) On the relationship between environmental management, environmental innovation and patenting: Evidence from German manufacturing firms. Res Policy 36: 1587–1602. https://doi.org/10.1016/j.respol.2007.08.004 doi: 10.1016/j.respol.2007.08.004
![]() |
[59] |
Wang Q, Yi HT (2021) New energy demonstration program and China's urban green economic growth: Do regional characteristics make a difference? Energ Policy 151: 112161. https://doi.org/10.1016/j.enpol.2021.112161 doi: 10.1016/j.enpol.2021.112161
![]() |
[60] |
Wang ZL, Qiu SL (2021) Can "energy saving and emission reduction" demonstration city selection actually contribute to pollution abatement in China? Sustain Prod Consump 27: 1882–1902. https://doi.org/10.1016/j.spc.2021.04.030 doi: 10.1016/j.spc.2021.04.030
![]() |
[61] |
Wen HW, Lee CC, Zhou FX (2021) Green credit policy, credit allocation efficiency and upgrade of energy-intensive enterprises. Energ Econ 94: 105099. https://doi.org/10.1016/j.eneco.2021.105099 doi: 10.1016/j.eneco.2021.105099
![]() |
[62] |
Wu AH (2017) The signal effect of Government R & D Subsidies in China: Does ownership matter? Technol Forecast Soc 117: 339–345. https://doi.org/10.1016/j.techfore.2016.08.033 doi: 10.1016/j.techfore.2016.08.033
![]() |
[63] |
Wu WF, Wu CF, Zhou CY, et al. (2012) Political connections, tax benefits and firm performance: Evidence from China. J Account Public Pol 31: 277–300. https://doi.org/10.1016/j.jaccpubpol.2011.10.005 doi: 10.1016/j.jaccpubpol.2011.10.005
![]() |
[64] |
Xie XM, Huo JG, Zou HL (2019) Green process innovation, green product innovation, and corporate financial performance: A content analysis method. J Bus Res 101: 697–706. https://doi.org/10.1016/j.jbusres.2019.01.010 doi: 10.1016/j.jbusres.2019.01.010
![]() |
[65] |
Xu QP, Kim T (2022) Financial Constraints and Corporate Environmental Policies. Rev Financ Stud 35: 576–635. https://doi.org/10.1093/rfs/hhab056 doi: 10.1093/rfs/hhab056
![]() |
[66] |
Xu S (2020) International comparison of green credit and its enlightenment to China. Green Financ 2: 75–99. https://doi.org/10.3934/Gf.2020005 doi: 10.3934/Gf.2020005
![]() |
[67] |
Xu XK, Li JS (2020) Asymmetric impacts of the policy and development of green credit on the debt financing cost and maturity of different types of enterprises in China. J Clean Prod 264: 121574. https://doi.org/10.1016/j.jclepro.2020.121574 doi: 10.1016/j.jclepro.2020.121574
![]() |
[68] |
Xu XY, Hou P, Liu Y (2022) The impact of heterogeneous environmental regulations on the technology innovation of urban green energy: a study based on the panel threshold model. Green Financ 4: 115–136. https://doi.org/10.3934/Gf.2022006 doi: 10.3934/Gf.2022006
![]() |
[69] |
Yi YY, Li JX (2018) The effect of governmental policies of carbon taxes and energy-saving subsidies on enterprise decisions in a two-echelon Supply chain. J Clean Prod 181: 675–691. https://doi.org/10.1016/j.jclepro.2018.01.188 doi: 10.1016/j.jclepro.2018.01.188
![]() |
[70] |
Yu BJ, Li CM, Mirza N, et al. (2022) Forecasting credit ratings of decarbonized firms: Comparative assessment of machine learning models. Technol Forecast Soc 174: 121255. https://doi.org/10.1016/j.techfore.2021.121255 doi: 10.1016/j.techfore.2021.121255
![]() |
[71] |
Yu FF, Guo Y, Le-Nguyen K, et al. (2016) The impact of government subsidies and enterprises' R & D investment: A panel data study from renewable energy in China. Energ Policy 89: 106–113. https://doi.org/10.1016/j.enpol.2015.11.009 doi: 10.1016/j.enpol.2015.11.009
![]() |
[72] |
Zhang B, Yang Y, Bi J (2011) Tracking the implementation of green credit policy in China: Top-down perspective and bottom-up reform. J Environ Manage 92: 1321–1327. https://doi.org/10.1016/j.jenvman.2010.12.019 doi: 10.1016/j.jenvman.2010.12.019
![]() |
[73] |
Zhang LL, Long RY, Huang Z, et al. (2020) Evolutionary game analysis on the implementation of subsidy policy for sustainable transportation development. J Clean Prod 267: 122159. https://doi.org/10.1016/j.jclepro.2020.122159 doi: 10.1016/j.jclepro.2020.122159
![]() |
[74] |
Zhang X, Yang YL, Li Y (2023) Does Public Participation Reduce Regional Carbon Emission? Atmosphere-Basel 14: 165. https://doi.org/10.3390/atmos14010165 doi: 10.3390/atmos14010165
![]() |
[75] |
Zhang ZH, Wang J, Feng C, et al. (2023) Do pilot zones for green finance reform and innovation promote energy savings? Evidence from China. Energ Econ 124: 106763. https://doi.org/10.1016/j.eneco.2023.106763 doi: 10.1016/j.eneco.2023.106763
![]() |
[76] | Zhao X, Ma XW, Chen BY, et al. (2022) Challenges toward carbon neutrality in China: Strategies and countermeasures. Resour Conserv Recy 176: 105959. https://doi.org/10.1016/j.resconrec.2021.105959 |
1. | Xiaohuan Yu, Mingzhan Huang, Dynamics of a Gilpin-Ayala predator-prey system with state feedback weighted harvest strategy, 2023, 8, 2473-6988, 26968, 10.3934/math.20231380 |