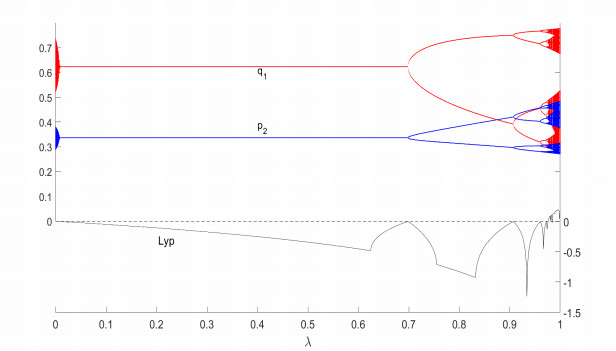
The Ornstein-Uhlenbeck (OU) process was used to simulate random perturbations in the environment. Considering the influence of telegraph noise and jump noise, a stochastic Gilpin-Ayala nonautonomous competition model driven by the mean-reverting OU process with finite Markov chain and Lévy jumps was established, and the asymptotic behaviors of the stochastic Gilpin-Ayala nonautonomous competition model were studied. First, the existence of the global solution of the stochastic Gilpin-Ayala nonautonomous competition model was proven by the appropriate Lyapunov function. Second, the moment boundedness of the solution of the stochastic Gilpin-Ayala nonautonomous competition model was discussed. Third, the existence of the stationary distribution of the solution of the stochastic Gilpin-Ayala nonautonomous competition model was obtained. Finally, the extinction of the stochastic Gilpin-Ayala nonautonomous competition model was proved. The theoretical results were verified by numerical simulations.
Citation: Meng Gao, Xiaohui Ai. A stochastic Gilpin-Ayala nonautonomous competition model driven by mean-reverting OU process with finite Markov chain and Lévy jumps[J]. Electronic Research Archive, 2024, 32(3): 1873-1900. doi: 10.3934/era.2024086
[1] | Wei Wu, Zhiyi Lin, Ming Wei . Research on a collaborative evolution model of multi-airport route network considering subsidy strategies. Mathematical Biosciences and Engineering, 2023, 20(11): 19808-19838. doi: 10.3934/mbe.2023877 |
[2] | Xiaochun Chen, Jie Zhao, Yingying Ma, Bo Lv, Xuanjin Du . Tripartite evolutionary game study on coordination information security in prescription circulation. Mathematical Biosciences and Engineering, 2023, 20(12): 21120-21146. doi: 10.3934/mbe.2023934 |
[3] | Pan Yang, Jianwen Feng, Xinchu Fu . Cluster collective behaviors via feedback pinning control induced by epidemic spread in a patchy population with dispersal. Mathematical Biosciences and Engineering, 2020, 17(5): 4718-4746. doi: 10.3934/mbe.2020259 |
[4] | A. Q. Khan, M. Tasneem, M. B. Almatrafi . Discrete-time COVID-19 epidemic model with bifurcation and control. Mathematical Biosciences and Engineering, 2022, 19(2): 1944-1969. doi: 10.3934/mbe.2022092 |
[5] | Xiaoling Han, Xiongxiong Du . Dynamics study of nonlinear discrete predator-prey system with Michaelis-Menten type harvesting. Mathematical Biosciences and Engineering, 2023, 20(9): 16939-16961. doi: 10.3934/mbe.2023755 |
[6] | Zhiyan Xing, Yanlong Yang, Zuopeng Hu . Nash equilibrium realization of population games based on social learning processes. Mathematical Biosciences and Engineering, 2023, 20(9): 17116-17137. doi: 10.3934/mbe.2023763 |
[7] | Mengshi Shu, Rui Fu, Wendi Wang . A bacteriophage model based on CRISPR/Cas immune system in a chemostat. Mathematical Biosciences and Engineering, 2017, 14(5&6): 1361-1377. doi: 10.3934/mbe.2017070 |
[8] | Ceyu Lei, Xiaoling Han, Weiming Wang . Bifurcation analysis and chaos control of a discrete-time prey-predator model with fear factor. Mathematical Biosciences and Engineering, 2022, 19(7): 6659-6679. doi: 10.3934/mbe.2022313 |
[9] | Qiulin Huang, Hengguo Yu, Chuanjun Dai, Zengling Ma, Qi Wang, Min Zhao . Dynamic analysis of a new aquatic ecological model based on physical and ecological integrated control. Mathematical Biosciences and Engineering, 2023, 20(1): 930-954. doi: 10.3934/mbe.2023043 |
[10] | Sonza Singh, Anne Marie France, Yao-Hsuan Chen, Paul G. Farnham, Alexandra M. Oster, Chaitra Gopalappa . Progression and transmission of HIV (PATH 4.0)-A new agent-based evolving network simulation for modeling HIV transmission clusters. Mathematical Biosciences and Engineering, 2021, 18(3): 2150-2181. doi: 10.3934/mbe.2021109 |
The Ornstein-Uhlenbeck (OU) process was used to simulate random perturbations in the environment. Considering the influence of telegraph noise and jump noise, a stochastic Gilpin-Ayala nonautonomous competition model driven by the mean-reverting OU process with finite Markov chain and Lévy jumps was established, and the asymptotic behaviors of the stochastic Gilpin-Ayala nonautonomous competition model were studied. First, the existence of the global solution of the stochastic Gilpin-Ayala nonautonomous competition model was proven by the appropriate Lyapunov function. Second, the moment boundedness of the solution of the stochastic Gilpin-Ayala nonautonomous competition model was discussed. Third, the existence of the stationary distribution of the solution of the stochastic Gilpin-Ayala nonautonomous competition model was obtained. Finally, the extinction of the stochastic Gilpin-Ayala nonautonomous competition model was proved. The theoretical results were verified by numerical simulations.
Oligopoly is a kind of market form dominated by a few sellers. It is a universal market structure that contains both monopoly and competition factors, and attracts the attention of mathematicians, economists and management scientists for its complexity and diversity. Two most notable models in oligopoly theory are Cournot model [1] and Bertrand model [2], in which duopoly firms compete respectively with respect to output and price. However, in actual economic activities, besides these two classic models, there are also Cournot- Bertrand hybrid models which take output and price as means of competition respectively. Unequal status between oligopolists is common in real life, oligopolists in the advantage position tend to use production competition to maintain their market position, while the disadvantaged ones tend to adopt the price competition to get more market share, such as the oil sales competition between Sinopec, PetroChina and some private oil companies. Therefore, it would be an interesting exploration to study the Cournot-Bertrand game, which exists in a large number of real economic systems.
Traditional oligopoly game models are all based on perfect rationality and perfect information, but that's hard to achieve in the real world. As the important assumption and cornerstone of the traditional economics, perfect rationality needs to meet the requirements of perfection in many aspects, such as rational consciousness, analytical reasoning ability, identification and judgment ability, memory ability and accurate behavior ability. However, there are some realistic factors such as the non-uniqueness of decision-making goal, the limitation of decision maker's cognition, and the uncertainty of environment in economic society. Therefore perfect rationality has been criticized for its overstepping reality, and bounded rationality has attracted more and more attention. Based on the widespread existence of bounded rationality, enterprises can make their own production or sales plans by adopting different expectation adjustment strategies. According to extant works, naïve [3,4], boundedly rational [5,6,7], adaptive [8,9], local monopolistic approximation [10,11] expectations are widely applied in game models with bounded rationality. Scholars have applied bounded rationality and expectation rules to different industries, for example, Reference [12] analyzed the pricing strategy of automobile manufacturers with bounded rationality, References [13,14] studied complex dynamical behaviors in supply chains with bounded rational participants. there are also some works [15,16] extending the application scope, they discussed the generalization of duopoly models with adaptive expectations and bounded rationality, which are obtained by introducing spatial diffusion in the autonomous and non-autonomous equations.
Classic game models such as Cournot and Bertrand require oligopolists to have complete information, that is, market participants can know all the information of other competitors. So they can make their best response according to the strategies and expected rewards of other players, and finally achieve Nash equilibrium. However, due to the limitation of cognitive ability, incomplete information economy generally exists in the market, and it is even more realistic than complete information economy. At the same time, oligopolists have different abilities to obtain information under the same economic environment, such as their own research and development ability or professional information acquisition ability, hence asymmetric information also exists widely among oligopolists, such as the asymmetric information acquisition degree of market demand between a manufacturer and a retailer[17], the information asymmetry of production cost between a leading firm and a following firm [4], the asymmetric information on marginal costs in a Bertrand game[9].
An enterprise cluster refers to the geographical aggregation of some closely related enterprises and their supporting institutions in an industry with a leading industry as the core [18], it is an important carrier of regional economic development. Due to geographical proximity, organizational proximity and cognitive proximity, enterprises in the cluster also show stronger competitiveness than general enterprises [19], such as the world-famous high-tech enterprises in Silicon Valley and Zhongguancun. The professional division of labor, high social trust and free flow of knowledge and information within the cluster accelerate the transfer of intellectual resources between enterprises and reduce the labor conversion cost. In the process of personnel flow and communication, the spillover of knowledge and technology inevitably occurs [20], leading to the reduction of operating costs of enterprises to a certain extent. Many scholars have studied the effect of technology spillover on the competition and cooperation between oligopolists [21,22,23,24,25,26]. Our paper considers a Cournot-Bertrand game model in an enterprise cluster led by duopoly firms. Following the idea in the literature [20], we regard the spillover effect as an important feature that distinguishes spatial clustered enterprises from general enterprises, and the cluster spillover effect produces positive externalities due to the existence of cluster enterprises and their activities (technology research and development, talent flow, knowledge exchange, etc).
Reviewing the existing literature, we believe that the following issues deserve further attention:(ⅰ) A Cournot-Bertrand game with asymmetric information, bounded rationality and cluster spillovers needs further research. The Cournot-Bertrand game exists in a large number of actual economic systems and has some important characteristics different from Cournot model and Bertrand model, however, there are few researches on it, while a lot of literature have been studied on these two classic models. In addition, it is rare to find works that integrate bounded rationality, asymmetric information and spillover effects into Cournot-Bertrand games. (ⅱ) In a dynamic duopoly Cournot-Bertrand game with bounded rationality, what are the effects of asymmetric information and spillovers on the Nash equilibrium point? (ⅲ) Does Bayesian Nash equilibrium exist in the dynamic production-price system? If so, what are the conditions? To address these issues, our paper consider a duopoly Cournot-Bertrand game model with asymmetric information, where firm 1 with an information advantage knows the price of firm 2, while firm 2 with an information disadvantage only knows part of firm 1's output. We discuss the effects of cluster spillovers, asymmetric information and product substitution rate on the Nash equilibrium under bounded rationality, and provide the region where the stable Nash equilibrium exists.
Our paper expands the extant literature on dynamic Cournot-Bertrand games in terms of bounded rationality and asymmetric information. Reference [27] first proposed the concept of mixed competition with output and price in 1975. Reference [28] studied the difference between output competition and price competition, and proposed a cournot-Bertrand mixed model. Some scholars pointed out that Cournot-Bertrand competition is even more advantageous in some cases [29,30]. Tremblay and Tremblay [31] discussed the static properties of Cournot-Bertrand duopoly game and found that it survived with sufficient product differentiation. However, the limitation of the perfectly rational hypothesis has always hindered the characterization of actual economic phenomena, and a few literatures have gradually studied Cournot-Bertrand games under bounded rationality [32,33,34]. Naimzada and Tramontana [32] analyzed the role of product differentiation in a Cournot-Bertrand model, and highlighted the role of best response dynamics and of an adaptive adjustment mechanism for the stability of the equilibrium. Wang and Ma [33] studied the complex of a Cournot-Bertrand mixed discrete system with limited information. Also few works analyzed asymmetric market information in a Cournot-Bertrand game [34]. As can be seen, few literature discussed Cournot-Bertrand game with bounded rationality, especially with both asymmetric information and bounded rationality. By contrast, this paper introduces asymmetric information into a Cournot-Bertrand game with bounded rational duopoly, and studies the existence of Nash equilibrium and its local stability. Different from [34], we extend Cournot-Bertrand game to the field of enterprise cluster and explore the influence of cluster spillovers on dynamic complexity.
Our research also contributes to complement the works of cluster spillovers in nonlinear dynamic system. The spillover effect between enterprises based on perfect rationality [21,22] or bounded rationality has always been concerned [20,23,24,25,26]. Reference [20] introduced positive cost externalities into a duopoly Cournot game and found that the presence of spillovers was beneficial to stable outputs. References [23] and [35] discussed R & D externalities in a two-stage oligopoly game along networks. Reference [25] studied the complex behaviors of R & D input in a triopoly model with bounded rationality. Reference [26] analyzed R & D spillover effect in a Cournot duopoly game with product differentiation. Reference [9] studied asymmetric information and cluster spillovers in a dynamic duopoly Bertrand game. Most existing references focused on the study of R & D spillovers between enterprises, and ignored the more general spillover effects between cluster enterprises agglomerated in a local geographic space. Besides, prior works rarely studied the spillover effect under asymmetric information and imperfect rationality. To address these deficiencies, our paper differs from previous studies in three ways. First, in our paper, cluster spillovers refer to the positive externalities brought by activities of cluster enterprises, such as technology research and development, talent flow, knowledge exchange, rather than just R & D spillover or technology spillovers, which is similar to [20], but the difference between our paper and previous works [9,20,36] is that we study the dynamic Cournor-Bertrand game model with production-price competition, while they considered output competition in Cournot game. Second, most of the existing literature discussed spillover effects in Cournot game or Bertrand game alone, by contrast, we explore to analyze the Cournot-Bertrand game in which production competition and price competition coexist. Third, this paper focuses on enterprise cluster, an important economic carrier, and studies the influence of spillovers and information asymmetry between cluster enterprises on a Cournot-Bertrand game, which is an important extension of discrete system in the field of industrial organization.
Two important findings from our analysis are summarized as below. First, the equilibrium points and their existence condition are proposed in the dynamic duopoly Cournot-Bertrand game with bounded rationality and asymmetric information, the critical boundary of Neimark-Sacker bifurcation and Flip bifurcation are also given. Second, in a dynamic duopoly Cournot-Bertrand game with heterogenous expectation and asymmetric information, where two firms use boundedly rational expectation and naïve expectation respectively, the Nash equilibrium is locally stable only if certain conditions are satisfied. Specifically theoretical research indicates that, when the output adjustment speed of enterprise in dominant position or the product substitution is larger, too low or too high effective information may lead to market disorder. When the product differentiation between duopoly enterprises is small, moderate cluster spillovers is conducive to the stability of product market, while excessive spillovers may lead to the fluctuation or even chaos. When the product differentiation is large, the higher the cluster spillovers is, the more stable the product market is.
The remainder of this article is shown as follows. A duopoly Cournot-Bertrand game model with asymmetric information, cluster spillovers and bounded rationality is considered in Section 2. The existence of Nash equilibrium and its stability region are discussed in Section 3. Various numerical simulation tools, including bifurcation diagrams, largest Lyapunov exponents, strange attractors, attraction basins, sensitive dependence on initial conditions, fractal dimension are presented in Section 4 to verify our theoretical analysis, we also give the practical significance of management. Finally, Section 5 gives a summary of our research.
We consider a duopoly market with differentiated products supplied by firms 1 and 2 in an enterprise cluster. Because of its advantages in market size, core competence and information mastery, firm 1 chooses output as the decision variable and influences market supply by controlling output. Firm 2, an oligopolist in a weak position, chooses price as the decision variable and influences market demand by controlling price. We further assume there exists asymmetric information between them, that is, firm 1 knows the current price of firm 2, while firm 2 only knows the current output of firm 1 to a certain extent. We let qi(t), pi(t) respectively represent firm i's (i=1,2) output and price at period t(t=0,1,2...). Therefore the inverse demand functions are given as follows:
p1(t)=1−q1(t)−dq2(t)p2(t)=1−q2(t)−dq1(t) | (2.1) |
where d∈[0,1] denotes the rate of product substitution, when d=0, products, produced by the two firms, are independent with no complementarity and substitution, while d=1 means that products are homogeneous. In this paper, the value range of d is (0,1), that is, we assume products of firms 1 and 2 can be replaced by each other.
It is assumed that firms have no fixed costs and firm i's cost function has the linear form: Ci(qi(t))=ciqi(t)−βQ(t). ciqi(t) represents firm i's cost in the absence of cluster spillover, where ci∈(0,1) is firm i's marginal cost. Q(t)=q1(t)+q2(t) is the total output of these two firms. β represents the spillover coefficient due to the geographical proximity, free flow of talents and good information sharing atmosphere among cluster enterprises, the cluster spillover can reduce product costs to a certain extent. According to the similar hypothesis of [20], where the cost reduction is positively correlated with outputs, we assume that βQ(t) represents the cost reduction. To ensure firms' cost positive, β lies in the interval (0,max{c1q1(t),c2q2(t)}Q(t)).
As firms choose different decision variables, we can convert Eq (2.1) into the following form:
p1(t)=1−d−(1−d2)q1(t)+dp2(t)q2(t)=1−p2(t)−dq1(t) | (2.2) |
As we know πi(t)=pi(t)qi(t)−Ci(qi(t)), then the firms' profits are given by:
π1(t)=(1−c1+β−βd−d)q1(t)+(d2−1)q21(t)+dq1(t)p2(t)−βp2(t)+βπ2(t)=(1+c2−β)p2(t)−p22(t)−dp2(t)q1(t)+(β−βd+c2d)q1(t)+β−c2 | (2.3) |
In the economic market, firms are faced with a complex and uncertain world, and their computing and cognitive abilities to the environment are limited, so both firms gradually adjust their output (or prices) with bounded rationality. We assume that firm 1 adopts a boundedly rational expectation, which means he determines his output on the basis of expected marginal profit ∂π1∂q1. So his adjustment mechanism is:
q1(t+1)=q1(t)+αq1(t)∂π1(t)∂q1(t) |
where α>0 represents the speed of output adjustment.
It is considered that firm 2 acts as a naïve player, he adjusts his price of next period with the reaction function. Because of the weak position in information acquisition, it is hard for firm 2 to master complete information of firm 1's output decision at current period, we use λ∈[0,1] to denote the effective information, which indicates how well firm 2 knows about firm 1's current exact production decision. A bigger value of λ means more accurate estimation. So firm 2's estimation of firm 1's output at period t+1 is qe1(t+1)=(1−λ)q1(t)+λq1(t+1), and firm 2's price adjustment expectation takes the following form:
p2(t+1)=1+c2−β−dqe1(t+1)2 |
Combining the above equations, then the Cournot-Bertrand discrete dynamic system, with bounded rationality and asymmetric information, can be described by the nonlinear difference equations:
{q1(t+1)=q1(t)+αq1(t)[1−c1+β−βd−d+2(d2−1)q1(t)+dp2(t)]p2(t+1)=1+c2−β−d[(1−λ)q1(t)+λq1(t+1)]2 | (2.4) |
This section is devoted to the study of the qualitative behavior of system (2.4). To this end, setting q1(t+1)=q1(t) and p2(t+1)=p1(t), the equilibrium points are obtained. Apart the boundary equilibrium E0≡(0,1+c2−β2),E1(q∗1,p∗2), system (2.4) admits the positive interior equilibrium E1≡(q∗1,p∗2) where
q∗1=2(1−c1+β)−(1−c2+3β)d4−3d2, |
p∗2=2(1+c2−β)−(1−c1+β)d−(1+2c2−3β)d24−3d2 |
and
{2(1−c1+β)−(1−c2+3β)d>02(1+c2−β)−(1−c1+β)d−(1+2c2−3β)d2>0 | (3.1) |
The condition (3.1) ensures the positivity of E1 since d∈[0,1].
To investigate the local stability of the equilibrium points E0 and E1, the Jacobian matrix J of system (2.4) is worked out in the following form:
J=[J11αdq1−d(1−λ+λJ11)2−αλd2q12] |
where J11=1+α[1−c1+β−βd−d+4(d2−1)q1+dp2].
Proposition 1. The boundary equilibrium point E0 of system (2.4) is a saddle point.
Proof. the Jacobian matrix at boundary equilibrium E0 is
J(E0)=[J110−d(1−λ+λJ11)20] |
The eigenvalues are given by the diagonal entries, i.e., φ1=1+α[1−c1+β−βd−d+d1+c2−β2], φ2=0, distinctly we have φ1>1 under condition (3.1), thereby proposition 1 is verified.
Proposition 2. On the hypothesis of that firm 1 chooses a boundedly rational expectation and firm 2 uses a naive expectation, with α<8(4−3d2)q∗1 given, E1 is locally asymptotically stable if λ∈(max{0,λ∗1},min{1,λ∗2}), λ∗1=1−2αd2q∗1,λ∗2=52−2d2+2αd2q∗1. System (2.4) undergoes a Neimark-Sacker bifurcation when λ≤λ∗1, stay stable when λ∈(max{0,λ∗1},min{1,λ∗2}), and experiences a flip bifurcation at E1 when λ=λ∗2. Moreover, period-2 points bifurcate from E1 and even chaos would occur when λ>λ∗2.
Proof. the Jacobian matrix at Nash equilibrium point E1 is
J(E1)=[1+2α(d2−1)q∗1αdq∗1−d[(1+2αλ(d2−1)q∗1)]2−αλd2q∗12] |
the characteristic polynomial of matrix J(E1) is
P(φ)=φ2−Trφ+Det, |
where Tr, Det are the trace and the determinant of J(E1) respectively, hence
Tr(J(E1))=1+2α(d2−1)q∗1−αλd2q∗12, |
Det(J(E1))=α(1−λ)d2q∗12. |
According to the stability theory, the necessary and sufficient conditions guaranteeing eigenvalues of Jacobian matrix J(E1) are less than one in modulus are [37]:
{(i):1+Tr(J(E1))+Det(J(E1))>0(ii):1−Tr(J(E1))+Det(J(E1))>0(iii):1−Det(J(E1))>0 | (3.2) |
The above inequalities of (i), (ii) and (iii) define a region where the Nash equilibrium point E1 is locally stable. The violation of any single inequality in (i), (ii) and (iii), while the other two inequalities are true, leads to: (1) a flip bifurcation (real eigenvalue that passes through −1) when 1+Tr(J(E1))+Det(J(E1))=0; (2) a fold or transcritical bifurcation (a real eigenvalue that passes through 1) when 1−Tr(J(E1))+Det(J(E1))=0; (3) a Neimark-Sacker bifurcation (i.e., the modulus of a complex eigenvalue pair that passes through 1) when 1−Det(J(E1))=0 and |Tr(J(E1))|<2.
Substitute Tr(J(E1)) and Det(J(E1)) into the inequalities (i)(ii)(iii), then the stability conditions in (3.2) can be written as follows:
{(i):1+Tr(J)+Det(J)=2+(5−2λ)d2−42αq∗1>0(ii):1−Tr(J)+Det(J)=4+(α−3)d22q∗1>0(iii):1−Det(J)=1−α(1−λ)d22q∗1>0 | (3.3) |
Obviously, the inequality (ii) is always satisfied with d,q∗1∈(0,1) and λ∈[0,1]. Then the condition (3.3) becomes
{2+(5−2λ)d2−42αq∗1>01−α(1−λ)d22q∗1>0 | (3.4) |
From the stable region (3.4), it's easy to know that the Jury condition is established when λ is in the interval (λ∗1,λ∗2), here we assume α<8(4−3d2)q∗1 to ensure the accuracy of the interval. As λ∈[0,1], then the stable interval of λ is (max{0,λ∗1},min{1,λ∗2}). In the case where λ∗1 and λ∗2 are positive, combined with chaos theory, we can derive that, as λ increases, system (2.4) may undergo chaos, Neimark-Sacker bifurcation when λ<λ∗1, becomes stable when λ∗1<λ<λ∗2, and experiences period-doubling bifurcation or even falls in chaos when λ>λ∗2, therefore proposition 2 is proved.
From the inequalities (3.4) we can also know that the equilibrium point E1 is stable when q∗1<min{2(1−λ)αd2,44α−(5−2λ)αd2}. By expanding the specific value of q∗1, proposition 3 which shows the relationship between β and the stability of system (2.4) can be obtained through the same analysis as proposition 2.
Proposition 3. On the hypothesis of that firm 1 chooses a boundedly rational expectation and firm 2 uses a naive expectation, the relationship between system stability and β can be divided into three cases: (i) In the case of d<23, the Nash equilibrium point E1 is asymptotically stable if β<β∗1=4(4−3d2)α(2−3d)[4−(5−2λ)d2]−2(1−c1)−(1−c2)d2−3d, system (2.4) would undergo a flip bifurcation at E1 when β=β∗1, and passes through period-doubling bifurcation until chaos when β>β∗1. (ii) In the case of d>23, the Nash equilibrium point E1 is asymptotically stable if β>β∗2=4−3d22−3dmin{2(1−λ)αd2,4α[4−(5−2λ)d2]}−2(1−c1)−(1−c2)d2−3d, system (2.4) may experience chaos, Neimark-Sacker bifurcations when β≤β∗2, and maintains stable when β>β∗2. (iii) In the case of d=23, the stability of system (2.4) is independent of β.
From Proposition 3 we can infer the following relations easily. (Ⅰ) In the case of d<23, β∗1 is negatively correlated with α and λ. The higher the output adjustment speed or effective information, the more likely high cluster spillover will cause the system to fall into bifurcation or chaos. (Ⅱ) In the case of d>23, β∗2 is positively correlated with α. The higher the output adjustment speed, the more likely low cluster spillover will make the system unstable. The relationship between β∗2 and λ depends on min{2(1−λ)αd2,4α[4−(5−2λ)d2]}. Further calculation shows that, when 4−(7−4λ)d2≥0, β∗2 is positively correlated with λ, while 4−(7−4λ)d2<0, β∗2 is negatively correlated with λ.
This section theoretically studies the stability and existence condition of Nash equilibrium when duopoly firms adopt heterogenous expectation rules, some interesting findings about the relationship between system stability and effective information λ, cluster spillovers β are showed in propositions 1–3. Next section we will use numerical simulation to verify our theoretical analysis and give the corresponding significance of management.
The main purpose of this section is to exhibit how the system (2.4) evolves with different values of model parameters. To provide numerical evidences for the existence of bifurcate and chaotic motions, various numerical simulation tools, including bifurcation diagram, 2D bifurcation diagram, the largest Lyapunov exponent diagram, strange attractors, basin of attraction, diagram of sensitive dependence on initial conditions and fractal dimension, are presented.
Figure 1 is a bifurcation diagram of system (2.4) with respect to λ, where other parameters setting is (α,c1,c2,β,d)=(4.52,0.1,0.3,0.1,0.846). As λ increases, system (2.4) first breaks away from chaos, then gradually stabilizes from Neimark-Sacker bifurcation when λ>0.0074, and remains stable at Nash equilibrium point (0.6228,0.3365) until λ reaches 0.6982. After that, the system undergoes flip bifurcation and finally falls into chaos. We also figure out the largest Lyapunov exponents to analyze the properties of system (2.4) quantitatively, where positive values imply that the outputs (prices) are in chaos and zero means that they are at bifurcation points.
Figure 2 shows the 2D bifurcation diagrams in the (λ,α) plane by setting (c1,c2,β,d)=(0.1,0.3,0.1,0.846). Different colors represent different cycles, the stable state is marked in brown, the green, orange, yellow, dark green, red, blue and purple regions represent the stable cycles of periods from 2 to 8 respectively, black regions means chaos. It can be seen from Figure 2(a) that when the output adjustment speed becomes large enough, no matter what value λ takes, the system remains stable. But when α lies in a certain range, the value of λ will deeply affect the stability of system (2.4), depicted in Figure 2(b). As λ goes from 0 to 1, system (2.4) becomes stable through Neimark-Sacker bifurcation, loses its stability through flip bifurcation, then goes into the stable cycles of periods 2, 4, 8..., and even fall into chaos state, as shown in Figure 1.
Figures 1 and 2 simulate Proposition 2. In some cases, either too low or too high effective information may cause market disorder. If too little information is obtained, the firm in a weak position would make decision based on the output of his rival in the last period, and disrupt the market because he doesn't know his rival's current output. If it is close to complete information, the firms in a strong position would constantly adjust their output, which affects the opponent's price decision, and eventually leads to the turbulence of the product market. Further analysis from Proposition 2 shows that, the larger the product substitution is, the more likely low efficient information is to promote market stability. In other words, when the product similarity between firms is high, the less information the firm in a weak position has, the more likely it is to form a stable market. Therefore, in the actual decision-making, maintaining a proper grasp of information is conducive to stabilizing the market.
Figure 3 shows the evolution trajectory of system (2.4) with respect to β under different conditions. In the case of d<23, shown in Figure 3(a), the dynamic system presents an equilibrium state when β<0.2918, and undergoes a flip bifurcation at E1(0.5042,0.5692) when β=0.2918, then it fluctuates until falling into chaos when β>0.2918. In the case of d>23, shown in Figure 3(b), we get an opposite trajectory of the system. However, the difference is that system (2.4) passes through a Neimark-Sacker bifurcation at E1(0.5580,0.2293) when β=0.0428, and then becomes stable when β>0.0428. In the case of d=23, there is no correlation between β and the stability of system (2.4), which is described in Figure 3(c). Other parameters (α,c1,c2,d,λ) in Figure 3(a)–(c) are respectively set as (2.532,0.16,0.65,0.436,0.22), (4.15,0.1405,0.0225,0.934,0.01) and (2.8,0.1,0.3,0.6667,0.4).
The numerical simulation in Figure 3 further supports the conclusions of proposition 3. When the product substitution between duopoly enterprises is small, that is, there is a certain difference between products, moderate cluster spillovers is conducive to the stability of product market, while excessive spillovers may lead to the fluctuation or even market disorders. When the product substitution is large, the higher the cluster spillovers is, the more stable the product market is. This is because the existence of spillover effect enables the firm in a weak position to occupy a place in the market by lowering the price. If there is no cluster spillovers, the random pricing of oligopolists with asymmetric information can lead to market chaos.
The strange attractor is a standard tool to characterize the chaos of a dynamic system, and it reflects the complexity and inherent regularity of chaotic behaviors. With its help, prices in a short term can be forecasted. In Figure 1, when λ takes a small or big value, the system has a positive Lyapunov exponent, which means that system (2.4) falls into chaos. Figure 4 shows the map of strange attractors of system (2.4) with different values of λ(0.002,0.2,0.97,0.99), where other parameters are the same as in Figure 1.
Figures 5–7 simulate the attraction basin of system (2.4) with different values of λ and β, where yellow, blue, red represent the attraction domain, the escape domain and the attractor. Other parameter settings are the same as in Figures 1, 3(a), (b) respectively. Figure 5 once again confirms that appropriate effective information is conducive to market stability under certain circumstances. Figures 6 and 7 show that high spillovers does not necessarily destroy market stability, system stability is also related to the substitutability between products. As can be seen from Figures 5(b), 6(a) and 7(b), when the initial decision output and price are in the domain of attraction, the system will eventually converge to the Nash equilibrium point after several games. However, in the periodic bifurcation and chaotic state, the firms' output will fluctuate periodically and even become unpredictable. In addition, from theses figures, we can see that the attraction domain of Nash equilibrium point is larger than that of the attractors in unstable state. Therefore, maintaining market stability is more conducive to reducing the interference of firms' decision-making errors.
One of the most important characteristics of discrete system in chaos is its sensitivity to initial conditions. This behavior is simulated in Figures 8 and 9. As we can see, when the dynamic system is chaotic, although there is a tiny change 0.001 of the initial values, the difference between them builds up rapidly after a number of iterations. Other parameters are given as: (α,c1,c2,β,d,λ)=(4.52,0.1,0.3,0.1,0.846,0.999) and the initial value is (q1(0),p2(0)=(0.3,0.2)).
Fractal dimension is a measure of the self-similarity and scale invariance of strange attractors, which is an important feature of chaotic dynamical systems. The Lyapunov dimension see [38] is defined as follows:
dL=j+∑ji=1li|lj+1| |
where j is the largest integer satisfying ∑ji=1li≥0 and ∑j+1i=1li<0, li is the Lyapunov exponents series, arranged in descending order. With the parameters set as (α,c1,c2,β,d,λ)=(4.52,0.1,0.3,0.1,0.846,0.99), the same setting as Figure 4(d), system (2.4) has two Lyapunov exponents l1=0.1582,l2=−4.9332, so the fractal dimension of the chaos attractor is dL=1+0.1582/4.9332≈1.0321. The result verifies that the performance of attractor in system (2.4) is consistent with the fractal theory, and it shows that the economic system exhibits a fractal structure at this time, and the evolution of system becomes more complex.
Based on the above theoretical analysis and numerical simulation, the following measures can be taken to ensure the stability of the product market in the Cournot-Bertrand game with asymmetric information and bounded rationality. First, for oligopoly products with certain differences, cluster managers and oligopoly managers should advocate a cluster environment with moderate spillovers, such as the mobility and communication among employees. Cluster oligopolists, especially those in a weak position, can enhance their competitiveness through spillover effect. However, cluster spillovers should not be excessive, because it would lead to bad free-riding behaviors, discouraging the enthusiasm of production research and development, which is the source of cluster spillover. Second, for oligopoly products with high substituability or similarity, a good cluster spillover atmosphere should be advocated. When there is information asymmetry, oligopolists in a weak position cannot know the exact output of other participants. Therefore, the benefits brought by low cluster spillover effects cannot make up for the losses caused by market uncertainty. In this case, market pricing is more random, which is easy to lead to market chaos. If the cluster spillover increases, the oligopolists in a weak position will choose to lower the product price because of the benefits brought by the high cluster spillover, which is conducive to the stability of the market. Third, disadvantaged oligopolists should keep an appropriate degree of effective information acquisition. Too little information may cause the oligopolist not be able to make the optimal decision for too much dependence on the opponent's previous production. However, too much information will lead to constant adjustment of oligopolists' decision-making, resulting in market fluctuations and even the exit of disadvantaged oligopolists from the market.
A dynamic Cournot-Bertrand duopoly model with imperfect rationality and incomplete information is considered in this paper, we analyzed the stability of Nash equilibrium where firms adopted heterogenous expectations, and presented the region where Nash equilibrium existed. Theoretical analysis shows that, in the case of that firm 1 with an information advantage uses boundedly rational expectation and firm 2 with an information disadvantage adopts naïve expectation, if the effective information of enterprise 2's output is too low or too high, the product market may fall into chaos. The correlation between cluster spillovers and system stability depends on the substitutability of oligopoly products, when the substitutability is larger, the probability of positive correlation is higher; otherwise, the probability of negative correlation is higher. Finally we verify the theoretical analysis with numerical simulation, and presents the corresponding management interpretation.
This research was supported by the General Project of Chongqing Natural Science Foundation (Grant No. cstc2020jcyj-msxmX0026), Science and Technology Research Project of Chongqing Municipal Education Commission (Grant No. KJQN202000832), High-level Talents Program of Chongqing Technology and Business University (Grant No. 1955046), On-campus Scientific Research Project of Chongqing Technology and Business University (Grant No. 2151018). and Youth Science Foundation of Hunan Agricultural University (Grant No. 18QN31).
The authors declare that there is no conflict of interest.
[1] |
M. Vogels, R. Zoeckler, D. M. Stasiw, L. C. Cerny, Notice sur la loi que la populations suit dans son accroissement, J. Biol. Phys., 3 (1975), 183–192. https://doi.org/10.1007/BF02309004 doi: 10.1007/BF02309004
![]() |
[2] |
M. Gilpin, F. J. Ayala, Global models of growth and competition, Proc. Natl. Acad. Sci. U. S. A., 70 (1973), 3590–3593. https://doi.org/10.1073/PNAS.70.12.3590 doi: 10.1073/PNAS.70.12.3590
![]() |
[3] | X. H. Zhang, Analysis on Dynamics of Some Stochastic Population Systems with Lévy Jumps, Ph.D thesis, Harbin Institute of Technology in China, 2014. https://doi.org/10.7666/d.D751846 |
[4] | R. M. May, Stability and Complexity in Model Ecosystems, Princeton: Princeton University Press, 2001. https://doi.org/10.1515/9780691206912 |
[5] |
X. Y. Li, X. R. Mao, Population dynamical behavior of non-autonomous Lotka-Volterra competitive system with random perturbation, Discrete Contin. Dyn. Syst., 24 (2009), 523–545. https://doi.org/10.3934/dcds.2009.24.523 doi: 10.3934/dcds.2009.24.523
![]() |
[6] |
M. Liu, K. Wang, Population dynamical behavior of Lotka-Volterra cooperative systems with random perturbations, Discrete Contin. Dyn. Syst., 33 (2013), 2495–2522. https://doi.org/10.3934/dcds.2013.33.2495 doi: 10.3934/dcds.2013.33.2495
![]() |
[7] |
M. Liu, P. Mandal, Dynamical behavior of a one-prey two-predator model with random perturbations, Commun. Nonlinear Sci. Numer. Simul., 28 (2015), 123–137. https://doi.org/10.1016/j.cnsns.2015.04.010 doi: 10.1016/j.cnsns.2015.04.010
![]() |
[8] |
W. R. Li, Q. M. Zhang, M. Anke, M. B. Ye, Y. Li, Taylor approximation of the solution of age-dependent stochastic delay population equations with Ornstein-Uhlenbeck process and Poisson jumps, Math. Biosci. Eng., 17 (2020), 2650–2675. https://doi.org/10.3934/mbe.2020145 doi: 10.3934/mbe.2020145
![]() |
[9] |
Y. L. Cai, J. J. Jiao, Z. J. Gui, Y. T. Liu, W. M. Wang, Environmental variability in a stochastic epidemic model, Appl. Math. Comput., 329 (2018), 210–226. https://doi.org/10.1016/j.amc.2018.02.009 doi: 10.1016/j.amc.2018.02.009
![]() |
[10] |
E. Allen, Environmental variability and mean-reverting processes, Discrete Contin. Dyn. Syst., 21 (2017), 2073–2089. https://doi.org/10.3934/dcdsb.2016037 doi: 10.3934/dcdsb.2016037
![]() |
[11] |
X. Mao, T. Aubrey, C. Yuan, Euler-Maruyama approximations in mean-reverting stochastic volatility model under regime switching, J. Appl. Math. Stochastic Anal., 2006 (2014), 5–12. https://doi.org/10.1155/JAMSA/2006/80967 doi: 10.1155/JAMSA/2006/80967
![]() |
[12] |
J. H. Bao, Z. T. Hou, An analytic approximation of solutions of stochastic differential delay equations with Markovian switching, Math. Comput. Modell., 50 (2009), 1379–1384. tps://doi.org/10.1016/j.mcm.2009.07.006 doi: 10.1016/j.mcm.2009.07.006
![]() |
[13] |
Q. Luo, X. R. Mao, Stochastic population dynamics under regime switching, J. Math. Anal. Appl., 334 (2007), 69–84. https://doi.org/10.1016/j.jmaa.2006.12.032 doi: 10.1016/j.jmaa.2006.12.032
![]() |
[14] |
T. Bui, G. Yin, Hybrid competitive Lotka–Volterra ecosystems: Countable switching states and two-time-scale models, Stochastic Anal. Appl., 37 (2019), 219–242. https://doi.org/10.1080/07362994.2018.1551141 doi: 10.1080/07362994.2018.1551141
![]() |
[15] |
S. J. Gao, D. M. Zhong, Y. Zhang, A remark on stochastic stability of a novel hybrid system, Appl. Math. Lett., 83 (2018), 145–150. https://doi.org/10.1016/j.aml.2018.03.028 doi: 10.1016/j.aml.2018.03.028
![]() |
[16] |
X. W. Yu, S. L. Yuan, T. H. Zhang, Persistence and ergodicity of a stochastic single species model with Allee effect under regime switching, Commun. Nonlinear Sci. Numer. Simul., 59 (2018), 359–374. https://doi.org/10.1016/j.cnsns.2017.11.028 doi: 10.1016/j.cnsns.2017.11.028
![]() |
[17] |
A. Settati, A. Lahrouz, On stochastic Gilpin–Ayala population model with Markovian switching, Biosystems, 130 (2015), 17–27. https://doi.org/10.1016/j.biosystems.2015.01.004 doi: 10.1016/j.biosystems.2015.01.004
![]() |
[18] | Y. Jiang, Z. J. Liu, J. Yang, Y. S. Tan, Dynamics of a stochastic Gilpin–Ayala population model with Markovian switching and impulsive perturbations, Adv. Differ. Equations, 2020 (2020). https://doi.org/10.1186/s13662-020-02900-w |
[19] |
J. H. Bao, C. G. Yuan, Stochastic population dynamics driven by Lévy noise, J. Math. Anal. Appl., 391 (2012), 363–375. https://doi.org/10.1016/j.jmaa.2012.02.043 doi: 10.1016/j.jmaa.2012.02.043
![]() |
[20] |
X. H. Zhang, K. Wang, Stability analysis of a stochastic Gilpin-Ayala model driven by Lévy noise, Commun. Nonlinear Sci. Numer. Simul., 19 (2014), 1391–1399. https://doi.org/10.1016/j.cnsns.2013.09.013 doi: 10.1016/j.cnsns.2013.09.013
![]() |
[21] |
M. Liu, K. Wang, Stochastic Lotka-Volterra systems with Lévy noise, J. Math. Anal. Appl., 410 (2014), 750–763. https://doi.org/10.1016/j.jmaa.2013.07.078 doi: 10.1016/j.jmaa.2013.07.078
![]() |
[22] |
Y. L. Zhu, K. Wang, Asymptotic properties of a stochastic Gilpin-Ayala model under regime switching, Nonlinear Anal. Hybrid Syst., 32 (2019), 79–90. https://doi.org/10.1016/j.nahs.2018.10.011 doi: 10.1016/j.nahs.2018.10.011
![]() |
[23] |
C. Y. Ji, D. Q. Jiang, N. Z. Zhong, Analysis of a predator–prey model with modified Leslie-Gower and Holling-type II schemes with stochastic perturbation, J. Math. Anal. Appl., 359 (2009), 482–498. https://doi.org/10.1016/j.jmaa.2009.05.039 doi: 10.1016/j.jmaa.2009.05.039
![]() |
[24] |
Y. Q. Li, H. L. Gao, Existence, uniqueness and global asymptotic stability of positive solutions of a predator-prey system with Holling II functional response with random perturbation, Nonlinear Anal. Theory Methods Appl., 68 (2008), 1694–1705. https://doi.org/10.1016/j.na.2007.01.008 doi: 10.1016/j.na.2007.01.008
![]() |
[25] |
H. Wei, W. Li, Dynamical behaviors of a Lotka-Volterra competition system with the Ornstein-Uhlenbeck process, Math. Biosci. Eng., 20 (2023), 7882–7904. https://doi.org/10.3934/mbe.2023341 doi: 10.3934/mbe.2023341
![]() |
[26] |
T. Ayoubi, H. Bao, Persistence and extinction in stochastic delay Logistic equation by incorporating Ornstein-Uhlenbeck process, Appl. Math. Comput., 386 (2020), 125465. https://doi.org/10.1016/j.amc.2020.125465 doi: 10.1016/j.amc.2020.125465
![]() |
[27] |
B. Q. Zhou, D. Q. Jiang, T. Hayat, Analysis of a stochastic population model with mean-reverting Ornstein-Uhlenbeck process and Allee effects, Commun. Nonlinear Sci. Numer. Simul., 111 (2022), 106450–106468. https://doi.org/10.1016/j.cnsns.2022.106450 doi: 10.1016/j.cnsns.2022.106450
![]() |
[28] |
Y. Zhou, D. Jiang, Dynamical behavior of a stochastic SIQR epidemic model with Ornstein-Uhlenbeck process and standard incidence rate after dimensionality reduction, Commun. Nonlinear Sci. Numer. Simul., 116 (2022), 106878. https://doi.org/10.1016/j.cnsns.2022.106878 doi: 10.1016/j.cnsns.2022.106878
![]() |
[29] |
X. Zou, K. Wang, Quasi-morphisms and thepoisson bracketnumerical simulations and modeling for stochastic biological systems with jumps, Commun. Nonlinear Sci. Numer. Simul., 19 (2014), 1557–1568. https://doi.org/10.48550/arXiv.math/0605406 doi: 10.48550/arXiv.math/0605406
![]() |
[30] |
Q. Luo, X. Mao, Stochastic population dynamics under regime switching, J. Math. Anal. Appl., 334 (2007), 69–84. https://doi.org/10.1016/j.jmaa.2006.12.032 doi: 10.1016/j.jmaa.2006.12.032
![]() |
[31] |
R. A. Lipster, Stochastic stability of differential equations, Stochastics, 3 (1980), 217–228. https://doi.org/10.1080/17442508008833146 doi: 10.1080/17442508008833146
![]() |
[32] | N. Ikeda, S. Watanabe, Stochastic Differential Equations and Diffusion Processes, North-Holland Publishing Company, Japan, 1981. |
[33] | G. D. Prato, J. Zabczyk, Ergodicity for Infinite Dimensional Systems, Cambridge University Press, 1996. |
[34] |
D. J. Higham, An algorithmic introduction to numerical simulation of stochastic differential equations, SIAM Rev., 43 (2001), 525–546. https://doi.org/10.1137/S0036144500378302 doi: 10.1137/S0036144500378302
![]() |
[35] |
X. F. Zhang, R. Yuan, A stochastic chemostat model with mean-reverting Ornstein–Uhlenbeck process and Monod-Haldane response function, Appl. Math. Comput., 394 (2021), 125833. https://doi.org/10.1016/j.amc.2020.125833 doi: 10.1016/j.amc.2020.125833
![]() |
[36] |
Y. L. Cai, J. J. Jiao, Z. J. Gui, Y. T. Liu, W. M. Wang, Environmental variability in a stochastic epidemic model, Appl. Math. Comput., 329 (2018), 210–226. https://doi.org/10.1016/j.amc.2018.02.009 doi: 10.1016/j.amc.2018.02.009
![]() |
[37] |
Y. Zhao, S. L. Yuan, J. L. Ma, Survival and stationary distribution analysis of a stochastic competitive model of three species in a polluted environment, Bull. Math. Biol., 77 (2015), 1285–1326. http://dx.doi.org/10.1007/s11538-015-0086-4 doi: 10.1007/s11538-015-0086-4
![]() |
[38] |
I. R. Geijzendorffer, W. van der Werf, F. J. J. A. Bianchi, R. P. O. Schulte, Sustained dynamic transience in a Lotka-Volterra competition model system for grassland species, Ecol. Modell., 222 (2011), 2817–2824. https://doi.org/10.1016/j.ecolmodel.2011.05.029 doi: 10.1016/j.ecolmodel.2011.05.029
![]() |
1. | Jing Yuan, Jianjun Zhu, Analysis of heterogeneous duopoly game with information asymmetry based on extrapolative mechanism, 2023, 0, 1081-1826, 10.1515/snde-2022-0052 | |
2. | Ivan Ivanov, Ivelin Ivanov, Anton Purnama, Burhanuddin Arafah, 2023, A fast algorithm to compute the Nash equilibrium for a two player positive game, 9781510671768, 31, 10.1117/12.3011689 | |
3. | Jianjun Long, Fenglian Wang, Complexity of a two-stage R&D game within a cluster supply chain considering vertical R&D spillovers, effective information, and government subsidies, 2024, 221, 03784754, 606, 10.1016/j.matcom.2024.03.027 | |
4. | Jianjun Long, Fenglian Wang, Equilibrium stability of dynamic duopoly Cournot game under heterogeneous strategies, asymmetric information, and one-way R&D spillovers, 2023, 12, 2192-8029, 10.1515/nleng-2022-0313 | |
5. | Rizwan Ahmed, M. Zubair Akbar Qureshi, Muhammad Abbas, Nida Mumtaz, Bifurcation and chaos control in a heterogeneous Cournot-Bertrand duopoly game model, 2025, 190, 09600779, 115757, 10.1016/j.chaos.2024.115757 | |
6. | Hanyu Yang, Mengru Ding, Muyao Li, Shilei Wu, Ye Zhang, Xun Dou, Optimal Operation of Generation Company’s Participating in Multiple Markets with Allocation and Exchange of Energy-Consuming Rights and Carbon Credits, 2024, 17, 1996-1073, 5884, 10.3390/en17235884 |