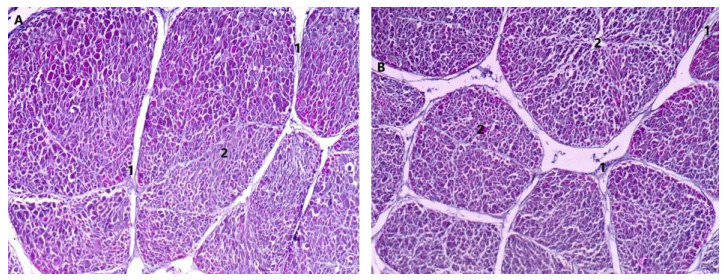
The objective of this study was to establish the morphological changes in the structure of Mediterranean mussel (Mytilus galloprovincialis) after frozen storage. Two hundred Mediterranean mussels (M. galloprovincialis) were collected from the Black Sea coastal waters. Forty mussels were subjected to histological analysis in fresh state. The remaining 160 mussels were divided into 4 groups and slowly frozen in a conventional freezer at −18 ℃ and subsequently stored at the same temperature for 3, 6, 9 and 12 months, respectively. The histological assessment of posterior adductor muscle and foot found a change in their morphological profile and overall structure. The fewest changes in the histostructure were recorded after a 3-month period and the most after a 12-month period of storage in frozen state. The results from that study can be used as an unambiguous marker in selecting optimum conditions for storage of mussels in frozen state.
Citation: Mariyana Strateva, Deyan Stratev, Georgi Zhelyazkov. Freezing influence on the histological structure of Mediterranean mussel (Mytilus galloprovincialis)[J]. AIMS Agriculture and Food, 2023, 8(2): 278-291. doi: 10.3934/agrfood.2023015
[1] | Talita Loureiro, Berta Gonçalves, Luís Serra, Ângela Martins, Isabel Cortez, Patrícia Poeta . Histological analysis of Xylella fastidiosa infection in Quercus pyrenaica in Northern Portugal. AIMS Agriculture and Food, 2024, 9(2): 607-627. doi: 10.3934/agrfood.2024033 |
[2] | María Quelal, Elena Villacrés, Karla Vizuete, Alexis Debut . Physicochemical characterization of sangorache natural colorant extracts (Amaranthus quitensis L.) prepared via spray- and freeze-drying. AIMS Agriculture and Food, 2023, 8(2): 343-358. doi: 10.3934/agrfood.2023019 |
[3] | Yenni, Mohd Hafiz Ibrahim, Rosimah Nulit, Siti Zaharah Sakimin . Influence of drought stress on growth, biochemical changes and leaf gas exchange of strawberry (Fragaria × ananassa Duch.) in Indonesia. AIMS Agriculture and Food, 2022, 7(1): 37-60. doi: 10.3934/agrfood.2022003 |
[4] | María Cámara-Ruiz, José María García Beltrán, Francisco Antonio Guardiola, María Ángeles Esteban . In vitro and in vivo effects of purslane (Portulaca oleracea L.) on gilthead seabream (Sparus aurata L.). AIMS Agriculture and Food, 2020, 5(4): 799-824. doi: 10.3934/agrfood.2020.4.799 |
[5] | Nelson Mpumi, Revocatus L. Machunda, Kelvin M. Mtei, Patrick A. Ndakidemi . Insecticidal Efficacy of Syzygium aromaticum, Tephrosia vogelii and Croton dichogamus Extracts against Plutella xylostella and Trichoplusia ni on Brassica oleracea crop in Northern Tanzania. AIMS Agriculture and Food, 2021, 6(1): 185-202. doi: 10.3934/agrfood.2021012 |
[6] | Tarik Ainane, Fatouma Mohamed Abdoul-Latif, Asmae Baghouz, Zineb El Montassir, Wissal Attahar, Ayoub Ainane, Angelo Maria Giuffrè . Correction: Essential oils rich in pulegone for insecticide purpose against legume bruchus species: Case of Ziziphora hispanica L. and Mentha pulegium L.. AIMS Agriculture and Food, 2023, 8(1): 236-238. doi: 10.3934/agrfood.2023013 |
[7] | Clarissa Trindade, Carla S.S. Gouveia, Maria Cristina O. Oliveira, Humberto Nóbrega, José G.R. de Freitas, Miguel A.A. Pinheiro de Carvalho . Inhibitory activity of the peel and seed of Annona cherimola Mill. against Fusarium and Aspergillus pathogens. AIMS Agriculture and Food, 2025, 10(1): 155-176. doi: 10.3934/agrfood.2025009 |
[8] | Nicolas Nagahama, Bruno Gastaldi, Michael N. Clifford, María M. Manifesto, Renée H. Fortunato . The influence of environmental variations on the phenolic compound profiles and antioxidant activity of two medicinal Patagonian valerians (Valeriana carnosa Sm. and V. clarionifolia Phil.). AIMS Agriculture and Food, 2021, 6(1): 106-124. doi: 10.3934/agrfood.2021007 |
[9] | Rossaporn Jiamjariyatam, Orachorn Mekkerdchoo, Pakkapong Phucharoenrak, Lu Zheng . Effects of freeze-drying and vacuum-drying on the quality, total phenolic contents, and antioxidant activities of bee honey in northern Thailand. AIMS Agriculture and Food, 2024, 9(2): 430-444. doi: 10.3934/agrfood.2024025 |
[10] | The Han Nguyen, Patrick Achiever Blamo Jr, Xiaoyong Liu, Thi Van Anh Tran, Sang Moo Kim . β-Glucuronidase inhibitory activity of bromophenol isolated from red alga Grateloupia lancifolia. AIMS Agriculture and Food, 2021, 6(2): 551-559. doi: 10.3934/agrfood.2021032 |
The objective of this study was to establish the morphological changes in the structure of Mediterranean mussel (Mytilus galloprovincialis) after frozen storage. Two hundred Mediterranean mussels (M. galloprovincialis) were collected from the Black Sea coastal waters. Forty mussels were subjected to histological analysis in fresh state. The remaining 160 mussels were divided into 4 groups and slowly frozen in a conventional freezer at −18 ℃ and subsequently stored at the same temperature for 3, 6, 9 and 12 months, respectively. The histological assessment of posterior adductor muscle and foot found a change in their morphological profile and overall structure. The fewest changes in the histostructure were recorded after a 3-month period and the most after a 12-month period of storage in frozen state. The results from that study can be used as an unambiguous marker in selecting optimum conditions for storage of mussels in frozen state.
Mussel production worldwide has shown an exceptional growth [1], which is due to their high nutritional value and high biodiversity [2]. Mussel meat contains a small amount of saturated fatty acids and is a good source of omega-3 fatty acids and proteins, therefore it has beneficial effects on human health [3,4]. Mussels are a good source of vitamins C, B, A, as well as phosphorus, iron, magnesium, calcium, selenium [5].
The Mediterranean mussel (M. galloprovincialis) is one of the most threatened species due to marine pollution [6], but is nevertheless an important species consumed in Europe. In recent years, the growing commercial interest in this species has been accounted for by its high nutritional value. The meat of this species contains high-quality protein, polyunsaturated fatty acids and essential macro- and micronutrients [7]. M. galloprovincialis is widely distributed in the Adriatic, Mediterranean and Black Seas. It is obtained through traditional fishing and cultivation in coastal waters [7].
Consumers always prefer to consume fresh mussels, despite the great diversity of products offered on the market. Fresh mussels are valued for their nutritional value, but unfortunately their shelf life as such is extremely limited up to 3 days. This means that mussels are an ideal environment for the development of bacteria, which leads to spoilage and risk when consuming them after this period [5]. In order to store them for a longer time, mussels are subjected to low temperatures. Freezing is an economically advantageous method that minimizes microbial and enzymatic development and achieves a relatively long shelf life. Regardless of these possibilities, tissue structures always develop changes due to the aggressive action of the ice crystals. Ice is capable of causing damages that are often irreversible. To reduce the risk of significant damage to the raw material when frozen, it is of utmost importance to freeze only high quality raw materials [8,9]. Extreme care must be taken in choosing a method and a suitable low temperature for freezing. During freezing, recrystallization of ice and reduction of the quality of frozen foods should not be allowed [10]. In general, the changes occurring after freezing have an impact on the physicochemical and microbiological profile on the one hand, and on the other the physical state of the raw material is affected. Processes to be avoided or limited during freezing are lipid oxidation, protein denaturation, enzyme activity, microbial spoilage and excessive ice crystal formation [9,11,12,13].
To our knowledge, there is no scientific data on the changes in the histostructure of M. galloprovincialis during storage for different periods in frozen state. Therefore, we set out to study the morphological changes occurring in the structure of this mussel after storage for 3, 6, 9 and 12 months.
Two hundred Mediterranean mussels (M. galloprovincialis) were collected from the Black Sea coastal waters by fishing trawlers of a company possessing a permit for that activity in June 2021. Once the mussels were caught, they were immediately transported to the laboratory of the Department of Veterinary Anatomy, Histology and Embryology, where the experimental part was carried out. Forty of the fresh mussels were immediately subjected to histological analysis. From each fresh mussel, material for histological study was taken from the foot and posterior adductor muscle (PAM). The remaining 160 mussels were divided into 4 groups and slowly frozen in a conventional freezer at −18 ℃ and subsequently stored at the same temperature for 3, 6, 9 and 12 months, respectively. After each of these periods, the corresponding group of mussels was thawed at 4 ℃ for 24 hours. Material for histological examination was taken from all frozen and thawed mussels in the respective experimental groups, consisting of foot and РАМ.
To make permanent histological preparations, the material was fixed immediately in 10% buffered formalin. After the 48-hour fixation period, the samples were washed with water and processed according to a standard paraffin embedding procedure. Cuts of 6 µm thickness were obtained on a rotary microtome (YD-335A, China), stained with the hematoxylin/eosin method according to a standard protocol. Histological analysis and evaluation were made using an N-200 M microscope (Hangzhou Sumer Instrument Co. Ltd, China). Photo documenting was done via camera OptikamB5 Digital Camera (OPTIKA MICROSCOPES, Italy) and software PROVIEW (Optika Srl, Ponteranica, Italy).
Posterior adductor muscle was used whole and the pictures show its structure in cross-section. Histological analysis showed that the muscle fibres were grouped in the form of bundles of different size and shape. They are observed as compact structures. The muscle fibres were polygonal in shape and surrounded by a layer of connective tissue. The borders of the muscle bundles were clearly visible. They were visible due to the presence of delicate connective tissue surrounding the contours of these bundles. The source of this delicate connective tissue was the outermost connective tissue sheath of the entire muscle.
The histological finding showed a change related to muscle fibres and bundles. At certain places their shape was broken due to rupture of the connective tissue. Despite these breakings, after 3 months of storage in frozen state, areas with a preserved shape but with changed volume of the bundles were still found. Freezing had caused these structures to shrink, which in turn reflected a greater presence of optical gaps between the bundles. The void spaces between the bundles were increased. From Figure 2A it is clear that the outermost sheath retained its position.
The histological analysis revealed more pronounced changes associated with greater contraction of bundles, presence of more ruptured muscle fibres and connective tissue (endomysium) and their aggregation into a common eosinophilic mass. The increase in empty spaces between bundles was more pronounced. Sections were encountered in which it was not possible to determine the end borders of the muscle bundles. The tissue was damaged to a large extent since a change in the organization of the fibre bundles was recorded.
The histological picture revealed an extremely affected structure with irreversibly broken structures at a greater extent than the previous temperature regimes of freezing. It was clearly noted that the distance between muscle masses had increased due to their contraction.
The performed histological assessment of the posterior adductor muscle revealed the greatest level of damage of muscle components after one-year freezing. Muscle fibres were destructured and ruptured at the greatest extent and that resulted in difficulties in outlining the end borders of muscle bundles. The change in shape and proportions of muscle fibres was the most pronounced there. Void spaces between the formed total eosinophilic mass of ruptured fibres formed were significantly increased. It proved impossible to indicate the connective tissue sheaths again due to their damage and rupture. Histological analysis of the posterior adductor muscle showed that irreversible morphological changes occurred to the highest extent after freezing and storage for a period of one year.
The histological analysis of the foot revealed that it consisted of epithelial, connective, muscle and glandular tissue. The foot surface is highly folded (plied). Its end or border zone is formed by a single-layer prismatic ciliated epithelium with the presence of secretory cells among the main ones. Its end zone is presented in the form of folds of different sizes, which are more pronounced in its proximal part. Their size decreased reaching its middle part, being absent in the area of the top of the foot. Moreover, the cells had the tallest shape in the apical part. They were also the densest there with the most numerous cilia. In the interfold area they were shorter and with fewer cilia on their surface. A clear border was formed between the epithelial and the connective tissue, comprising a basal membrane of collagen fibres. The muscles were presented in the form of muscle fibre groups of different size made up of smooth muscles, i.e., the smooth muscle tissue was distributed in separate areas and was not represented as a complete layer. Regarding the quantitative distribution of the muscle groups, it was also different in the individual sections. Zoning was absent at the tip because no muscles were found there. This area was occupied by glandular and epithelial tissue. The smooth muscle tissue appeared connected to the connective tissue in the form of a net. The glandular part appeared to be represented by a set of glandular structures.
Disorganization of the structural components was observed. The epithelial cover was totally missing. There were void spaces among the components.
The histological analysis showed more pronounced damage and contraction of the components. Epithelial cover was missing and the muscle and glandular part were demarked by the presence of large optical void spaces that made the foot appear deformed.
Freezing had affected and damaged the foot at a greater extent compared to the previous temperature regimes. Extremely contracted and destructured muscles were observed. The presence of void spaces among the components was considerably greater than the previous regimes. The foot was ragged and torn in appearance due to fragmentation of its constituent structures.
The histological analysis of frozen foot for a period of one year revealed a different picture compared to the previous temperature regimes. Structural components were damaged to the highest extent. The foot appeared to be dehydrated and destructured. Conformational changes such as contracted structures in the muscles and glandular part were noted. They predominated in the monitored areas and resulted in an increase of void spaces (in white colour) among them. The changes that occurred were serious and irreversible to the highest extent here.
The main muscles of M. galloprovincialis are posterior adductor muscle and byssus retractor muscle. Muscles are represented by smooth muscle tissue and they are characterized as specialized [14,15]. According to Castro-Claros et al. [16] adductor muscles of the mussels are connected to the valves and their main role is to control the opening and closing of these valves. Thus, they are vitally important for their survival in the aquatic environment. Mediodia [17] believes that the size of the anterior and posterior adductor muscles is not the same, with the posterior adductor muscle being larger. Cappello et al. [15] found that the main muscle system in mussels are actually the adductor muscles. Some studies indicate that there is a difference in the morphological structure of the adductor muscles of bivalve molluscs and they may be species specific [16,18]. In mussels Ostrea stentina and Anomia ephippium, adductor muscles are composed of both smooth and striated fibres. McElwain and Bullard [19] describe the connective tissue sheaths of the adductor muscles as perimysium and endomysium, and the outermost is epimysium. According to Simone [20], adductor muscles are strategically located at the ends of the shells and this allows them to react and close quickly. It turns out that mussels do not have the so-called abductors and their function is performed by a ligament.
The morphological features of the Mediterranean mussel (M. galloprovincialis) have been described by Vitellaro-Zuccarello et al. [21]. The foot is a set of muscle and glandular tissue. Its dorsal part consists of muscles, while the ventral part is byssus glands. The primary role of the muscle-glandular foot is to form the byssus for the formation of the byssus threads. McElwain and Bullard [19] reckon that varied muscle fibres form the muscles of the foot. They define the outer part of the foot as striated, which is also clearly visible from results in the present study. Balamurugan and Subramanian [22] describe the foot in bivalves as an organ with a motor function that allows them to burrow. They also found that its outer part was composed of fold-forming epithelium. The results of our study coincide with those of Balamurugan and Subramanian [22] since we also found the presence of differently sized folds formed with the participation of epithelial tissue from the foot structure in mussels. We are also in agreement with the statements that epithelial cells have different sizes in the individual parts of these folds. Larger sized and clearly visible oval and basally located nuclei are found in the apical parts. Lee et al. [23] support the statement that foot muscles are smooth muscle tissue. Smooth muscle myocytes are composed of thick and thin myofilaments. Radwan et al. [24] studied the foot of the Pinctata radiata mussel and found that it was built in a similar way to other mussels, having an epithelial part, a connective tissue zone and muscles of smooth muscle fibres. Our results coincide with what was found by Radwan et al. [24] regarding the morphological characteristic of the foot. We also confirm the presence of the same tissues in its histological structure.
Foot structure in fresh Greenshell™ mussel (Perna canaliculus) was studied by Angane et al. [25]. The presence of a membrane surrounding the shape of the foot, as well as well-preserved and recognizable muscles have been found. After freezing, damage and rupture of the muscles due to the aggressive action of the ice crystals have been observed. The position of the so-called outer membrane, broken in places, has been changed. To a certain extent, we find similar changes, and in our study epithelial tissue detachment has been observed due to foot detachment in mussels that had been frozen. This means that its border zone has been damaged and we thus confirm the affected surrounding membrane described by Angane et al. [25], since our results made it clear that in fact the epithelial covering of the foot is its outermost part or end border. Park et al. [26] also studied and revealed the histological structure of the foot in Gomphina veneriformis, which has epithelial, connective and muscle tissue. The typical shape of the foot is maintained by the epithelial layer, which is a very well-recognizable borderline here as well. In the other case, when distinguishing the muscle part from the connective tissue one, no clearcut border has been observed. The surface is repeatedly folded. As a type of epithelial tissue, a prismatic ciliated epithelium with the presence of secretory cells has been established. The section covered by the connective tissue is presented in the form of a strip of small dimensions, composed mainly of fibres of collagen origin. The muscle part is formed by a compilation of collagen fibres and muscle fibres in separate groups. This descriptive feature fully coincides with our results, and this structure is also confirmed in the present study. Mohamed et al. [27] also found that the structure of the mussel foot is related to the presence of an outer plicated epithelial part, connective and muscle tissue. The authors again share the view that a sharp boundary exists between connective and epithelial tissue, whereas no such boundary is found between connective and muscle tissue.
Parisi et al. [28] described posterior adductor muscle of M. galloprovincialis as proper organization of muscle fibres and connective tissue. Our research is in agreement with Parisi et al. [28] concerning the proper distribution of muscle fibres and connective tissue of posterior adductor muscle in fresh M. galloprovincialis. In another study Parisi et al. [29] found severe changes in posterior adductor muscle after freezing M. galloprovincialis. Loss of muscle volume has been found which is a cause for the appearance of void spaces among fibres and the bundles. In our study contacted muscle bundles and presence of large void spaces among them were also available after freezing. Sheir et al. [30] and Al-Subiai et al. [31] proved that posterior adductor muscle of the Mytilus edulis mussel was made of muscle bundles surrounded by connective tissue coat and the muscle fibres were polygonal in shape. Our results coincide with the characteristics pointed out by Parisi et al. [29], Sheir et al. [30] and Al-Subiai et al. [31].
Freezing is widely used in the trade of sea species because they are products of short shelf life [32,33]. The recommended temperature for freezing molluscan shellfish is −18 ℃ and this slow freezing method takes between 3 and 72 hours depending on the amount of product to be frozen [34]. It is a well-known fact that freezing affects the composition of meat, while the loss of integrity of cell membranes is initiated by the formed ice crystals [35]. Tissue damage is the essential image most clearly recognized in mussels subjected to freezing. During slow freezing, the occurrence of osmotic phenomena is the main cause of change in the shape of fibres and their bundles. The observed changes are visible and show contracted fibres and bundles. As a result, the tissue appears dehydrated. The fact is that the slow freezing method results in the formation of extracellular and large ice crystals. This, in turn, is an exceptional prerequisite and a real reason for the disruption of the cell membranes of the fibres [36]. Void spaces localized among bundles of grouped muscle fibres resulted from the freezing and ice mass formation in these places [37]. Furnesvik et al. [38] claim that freezing resulted in the formation of ice crystals in the spaces among fibres, which is the cause for the occurrence of void spaces among them. In this way, they look separated and distant from each other, and some are of broken integrity. Disruption and damage to cellular components and structures are inevitable after freezing. Its consequence is the deterioration of the quality of the raw material due to lipid oxidation, protein denaturation and water loss after thawing. As a result, these processes will have a negative impact on the taste of the respective product [33]. After freezing, the changes that are visualized are related to disruption of cellular structures and the appearance of biochemical components in the intercellular spaces [39]. According to Shafieipour and Sami [40], tissue damage during freezing comes from the pressure of ice crystals, which results in the release of cellular contents into the spaces around fibres and bundles. The use of the histological method provides reliable information about the type of fibres and muscle bundles. With its help, the overall picture in the studied tissues is unambiguously interpreted [41]. As already stated, slow rate freezing is responsible for the formation of the large and extracellular ice crystals. It is in this type of freezing that the water found in the intercellular spaces is first affected and the ice masses are formed there. These masses result in tissue damage that is irreversible. After thawing such a product, its quality is questionable because water and all components dissolved in it are lost. Freezing rate is the parameter that shapes the type and size of ice crystals [42]. Our study fully supports these views and confirms the disruptive role of extracellular ice crystals.
The study elucidates the histological changes occurring during different freezing storage periods of Mediterranean mussels (M. galloprovincialis). The histological assessment of the posterior adductor muscle and foot after different freezing storage revealed a change in their morphological profile and overall structure. The fewest changes in histostructure were reported after a 3-month period, and the greatest number after a 12-month period of frozen storage. The results of this study can be used as an unambiguous marker in the selection of optimal conditions for storage of mussels in the frozen state.
The study was funded under Project No. 8/2021 of the Faculty of Agriculture at Trakia University–Stara Zagora, Bulgaria.
The authors declare no conflict of interest.
[1] |
Subramaniam T, Lee HJ, Jeung HD, et al. (2021) Report on the annual gametogenesis and tissue biochemical composition in the Gray mussel, Crenomytilus grayanus (Dunker 1853) in the subtidal rocky bottom on the east coast of Korea. Ocean Sci J 56: 424–433. https://doi.org/10.1007/s12601-021-00042-y doi: 10.1007/s12601-021-00042-y
![]() |
[2] |
Fitori A, Ishag IA, Masoud AN, et al. (2021) Assessment of some heavy metals using sediments and bivalvia (Mytilus galloprovincialis) samples collected from Tobruk coast. Sci J Fac Sci-Sirte Univ 1: 25–31. https://doi.org/10.37375/sjfssu.v1i2.79 doi: 10.37375/sjfssu.v1i2.79
![]() |
[3] |
Czech A, Grela ER, Ognik K (2015) Effect of frying on nutrients content and fatty acid composition of muscles of selected freezing seafoods. J Food Nutr Res 3: 9–14. https://doi.org/10.12691/jfnr-3-1-2 doi: 10.12691/jfnr-3-1-2
![]() |
[4] |
Tan K, Zhang HK, Li SK, et al. (2022) Lipid nutritional quality of marine and freshwater bivalves and their aquaculture potential. Crit Rev Food Sci Nutr 62: 6990–7014. https://doi.org/10.1080/10408398.2021.1909531 doi: 10.1080/10408398.2021.1909531
![]() |
[5] | Gurdal AA, Caglak E (2021) Investigation of nutritional, some quality changes of mussels covered with edible films prepared using extracts of persimmon, Cherry Laurel and Likapa. Fresenius Environ Bull 30: 1823–1836. |
[6] |
Afsa S, De Marco G, Giannetto A, et al. (2022) Histological endpoints and oxidative stress transcriptional responses in the Mediterranean mussel Mytilus galloprovincialis exposed to realistic doses of salicylic acid. Environ Toxicol Pharmacol 92: 103855. https://doi.org/10.1016/j.etap.2022.103855 doi: 10.1016/j.etap.2022.103855
![]() |
[7] |
Peycheva K, Panayotova V, Stancheva R, et al. (2022) Effect of steaming on chemical composition of Mediterranean mussel (Mytilus galloprovincialis): Evaluation of potential risk associated with human consumption. Food Sci Nutr 10: 3052–3061. https://doi.org/10.1002/fsn3.2903 doi: 10.1002/fsn3.2903
![]() |
[8] |
Tan MT, Ye JX, Chu YM, et al. (2021) The effects of ice crystal on water properties and protein stability of large yellow croaker (Pseudosciaena crocea). Int J Refrig 130: 242–252. https://doi.org/10.1016/j.ijrefrig.2021.05.040 doi: 10.1016/j.ijrefrig.2021.05.040
![]() |
[9] |
Zhu SC, Yu HJ, Chen X, et al. (2021) Dual cryoprotective strategies for ice-binding and stabilizing of frozen seafood: A review. Trends Food Sci Technol 111: 223–232. https://doi.org/10.1016/j.tifs.2021.02.069 doi: 10.1016/j.tifs.2021.02.069
![]() |
[10] |
Lee S, Jo K, Jeong HG, et al. (2022) Freezing-induced denaturation of myofibrillar proteins in frozen meat. Crit Rev Food Sci Nutr, 1–18. https://doi.org/10.1080/10408398.2022.2116557 doi: 10.1080/10408398.2022.2116557
![]() |
[11] |
Al-Jeddawi W, Dawson P (2022) The effect of frozen storage on the quality of Atlantic Salmon. J Food Sci Nutr Res 5: 552–569. https://doi.org/10.26502/jfsnr.2642-11000098 doi: 10.26502/jfsnr.2642-11000098
![]() |
[12] |
Gao YP, Jiang HL, Lv DD, et al. (2021) Shelf-life of half-shell mussel (Mytilus edulis) as affected by pullulan, acidic electrolyzed water, and stable chlorine dioxide combined ice-glazing during frozen storage. Foods 10: 1896. https://doi.org/10.3390/foods10081896 doi: 10.3390/foods10081896
![]() |
[13] |
Tian J, Walayat N, Ding YT, et al. (2022) The role of trifunctional cryoprotectants in the frozen storage of aquatic foods: Recent developments and future recommendations. Compr Rev Food Sci Food Saf 21: 321–339. https://doi.org/10.1111/1541-4337.12865 doi: 10.1111/1541-4337.12865
![]() |
[14] |
Bardales JR, Cascallana JL, Villamarín A (2011) Differential distribution of cAMP-dependent protein kinase isoforms in various tissues of the bivalve mollusc Mytilus galloprovincialis. Acta Histochem 113: 743–748. https://doi.org/10.1016/j.acthis.2010.11.002 doi: 10.1016/j.acthis.2010.11.002
![]() |
[15] |
Cappello T, Maisano M, Mauceri A, et al. (2017) 1 H NMR-based metabolomics investigation on the effects of petrochemical contamination in posterior adductor muscles of caged mussel Mytilus galloprovincialis. Ecotoxicol Environ Saf 142: 417–422. https://doi.org/10.1016/j.ecoenv.2017.04.040 doi: 10.1016/j.ecoenv.2017.04.040
![]() |
[16] |
Castro-Claros JD, Checa A, Lucena C, et al. (2021) Shell-adductor muscle attachment and Ca2+ transport in the bivalves Ostrea stentina and Anomia ephippium. Acta Biomater 120: 249–262. https://doi.org/10.1016/j.actbio.2020.09.053 doi: 10.1016/j.actbio.2020.09.053
![]() |
[17] |
Mediodia DP, Santander-de Leon SMS, Añasco N, et al. (2017) Shell Morphology and Anatomy of the Philippine Charru Mussel Mytella charruana (d'Orbigny 1842). Asian Fish Sci 30: 185–194. https://doi.org/10.33997/j.afs.2017.30.3.004 doi: 10.33997/j.afs.2017.30.3.004
![]() |
[18] |
Yamada A, Yoshio M, Nakamura A, et al. (2004) Protein phosphatase 2B dephosphorylates twitchin, initiating the catch state of invertebrate smooth muscle. J Biol Chem 279: 40762–40768. https://doi.org/10.1074/jbc.M405191200 doi: 10.1074/jbc.M405191200
![]() |
[19] |
McElwain A, Bullard SA (2014) Histological atlas of freshwater mussels (Bivalvia, Unionidae): Villosa nebulosa (Ambleminae: Lampsilini), Fusconaia cerina (Ambleminae: Pleurobemini) and Strophitus connasaugaensis (Unioninae: Anodontini). Malacologia 57: 99–239. https://doi.org/10.4002/040.057.0104 doi: 10.4002/040.057.0104
![]() |
[20] | Simone LRL (2019) Modifications in adductor muscles in bivalves. Malacopedia 2: 1–12. |
[21] |
Vitellaro-Zuccarello L, De Biasi S, Amadeo A (1990) Immunocytochemical demonstration of neurotransmitters in the nerve plexuses of the foot and the anterior byssus retractor muscle of the mussel, Mytilus galloprovincialis. Cell Tissue Res 261: 467–476. https://doi.org/10.1007/BF00313525 doi: 10.1007/BF00313525
![]() |
[22] |
Balamurugan S, Subramanian P (2021) Histopathology of the foot, gill and digestive gland tissues of freshwater mussel, Lamellidens marginalis exposed to oil effluent. Austin J Environ Toxicol 7: 1033. https://doi.org/10.26420/austinjenvirontoxicol.2021.1033 doi: 10.26420/austinjenvirontoxicol.2021.1033
![]() |
[23] |
Lee JS, Lee YG, Park JJ, et al. (2012) Microanatomy and ultrastructure of the foot of the infaunal bivalve Tegillarca granosa (Bivalvia: Arcidae). Tissue Cell 44: 316–324. https://doi.org/10.1016/j.tice.2012.04.010 doi: 10.1016/j.tice.2012.04.010
![]() |
[24] |
Radwan EH, Saad GA, Hamed SSh (2016) Ultrastructural study on the foot and the shell of the oyster Pinctada radiata (leach, 1814), (Bivalvia Petridae). J Biosci Appl Res 2: 274–282. doi: 10.21608/jbaar.2016.107548
![]() |
[25] |
Angane M, Gupta S, Fletcher GC, et al. (2020) Effect of air blast freezing and frozen storage on Escherichia coli survival, n-3 polyunsaturated fatty acid concentration and microstructure of GreenshellTM mussels. Food Control 115: 107284. https://doi.org/10.1016/j.foodcont.2020.107284 doi: 10.1016/j.foodcont.2020.107284
![]() |
[26] |
Park JJ, Lee JS, Lee YG, et al. (2012) Micromorphology and Ultrastructure of the Foot of the Equilateral Venus Gomphina veneriformis (Bivalvia: Veneridae). CellBio 1: 11–16. https://doi.org/10.4236/cellbio.2012.11002 doi: 10.4236/cellbio.2012.11002
![]() |
[27] |
Mohamed AS, Bin Dajem S, Al-Kahtani M, et al. (2021) Silver/chitosan nanocomposites induce physiological and histological changes in freshwater bivalve. J Trace Elem Med Biol 65: 126719. https://doi.org/10.1016/j.jtemb.2021.126719 doi: 10.1016/j.jtemb.2021.126719
![]() |
[28] |
Parisi MG, Baranzini N, Dara M, et al. (2022) AIF-1 and RNASET2 are involved in the inflammatory response in the Mediterranean mussel Mytilus galloprovincialis following Vibrio infection. Fish Shellfish Immun 127: 109–118. https://doi.org/10.1016/j.fsi.2022.06.010 doi: 10.1016/j.fsi.2022.06.010
![]() |
[29] |
Parisi MG, Maisano M, Cappello T, et al. (2019) Responses of marine mussel Mytilus galloprovincialis (Bivalvia: Mytilidae) after infection with the pathogen Vibrio splendidus. Comp Biochem Phys C 221: 1–9. https://doi.org/10.1016/j.cbpc.2019.03.005 doi: 10.1016/j.cbpc.2019.03.005
![]() |
[30] |
Sheir SK, Handy RD, Galloway TS (2010) Tissue injury and cellular immune responses to mercuric chloride exposure in the common mussel Mytilus edulis: Modulation by lipopolysaccharide. Ecotoxicol Environ Saf 73: 1338–1344. https://doi.org/10.1016/j.ecoenv.2010.01.014 doi: 10.1016/j.ecoenv.2010.01.014
![]() |
[31] |
Al-Subiai SN, Moody AJ, Mustafa SA, et al. (2011) A multiple biomarker approach to investigate the effects of copper on the marine bivalve mollusc, Mytilus edulis. Ecotoxicol Environ Saf 74: 1913–1920. https://doi.org/10.1016/j.ecoenv.2011.07.012 doi: 10.1016/j.ecoenv.2011.07.012
![]() |
[32] |
Nieto-Ortega S, Melado-Herreros Á, Foti G, et al. (2021) Rapid differentiation of unfrozen and frozen-thawed tuna with non-destructive methods and classification models: Bioelectrical impedance analysis (BIA), Near-infrared spectroscopy (NIR) and Time domain reflectometry (TDR). Foods 11: 55. https://doi.org/10.3390/foods11010055 doi: 10.3390/foods11010055
![]() |
[33] |
Yang SB, Hu YQ, Takaki K, et al. (2021) Effect of water ice-glazing on the quality of frozen swimming crab (Portunus trituberculatus) by liquid nitrogen spray freezing during frozen storage. Int J Refrig 131: 1010–1015. https://doi.org/10.1016/j.ijrefrig.2021.06.035 doi: 10.1016/j.ijrefrig.2021.06.035
![]() |
[34] | Noomhorm A, Vongsawasdi P (2004) Freezing shellfish, In: Hui YH, Legarretta IG, Lim MH, et al. (Eds.), Handbook of frozen foods, New York: Marcel Dekker, 309–324. |
[35] |
Stella R, Mastrorilli E, Pretto T, et al. (2022) New strategies for the differentiation of fresh and frozen/thawed fish: Non-targeted metabolomics by LC-HRMS (part B). Food Control 132: 108461. https://doi.org/10.1016/j.foodcont.2021.108461 doi: 10.1016/j.foodcont.2021.108461
![]() |
[36] |
Tinacci L, Armani A, Guidi A, et al. (2018) Histological discrimination of fresh and frozen/thawed fish meat: European hake (Merluccius merluccius) as a possible model for white meat fish species. Food Control 92: 154–161. https://doi.org/10.1016/j.foodcont.2018.04.056 doi: 10.1016/j.foodcont.2018.04.056
![]() |
[37] |
Tinacci L, Armani A, Scardino G, et al. (2020) Selection of histological parameters for the development of an analytical method for discriminating fresh and frozen/thawed common octopus (Octopus vulgaris) and preventing frauds along the seafood chain. Food Anal Methods 13: 2111–2127. https://doi.org/10.1007/s12161-020-01825-0 doi: 10.1007/s12161-020-01825-0
![]() |
[38] |
Furnesvik L, Erkinharju T, Hansen M, et al. (2022) Evaluation of histological post-mortem changes in farmed Atlantic salmon (Salmo salar L.) at different time intervals and storage temperatures. J Fish Dis 45: 1571–1580. https://doi.org/10.1111/jfd.13681 doi: 10.1111/jfd.13681
![]() |
[39] |
Bouchendhomme T, Soret M, Devin A, et al. (2022) Differentiating between fresh and frozen-thawed fish fillets by mitochondrial permeability measurement. Food Control 141: 109197. https://doi.org/10.1016/j.foodcont.2022.109197 doi: 10.1016/j.foodcont.2022.109197
![]() |
[40] |
Shafieipour A, Sami M (2015) The effect of different thawing methods on chemical properties of frozen pink shrimp (Penaeus duorarum). Iran J Vet Med 9: 1–6. https://doi.org/10.22059/ijvm.2015.53226 doi: 10.22059/ijvm.2015.53226
![]() |
[41] |
Anderssen KE, Kranz M, Syed S, et al. (2022) Diffusion tensor imaging for spatially-resolved characterization of muscle fiber structure in seafood. Food Chem 380: 132099. https://doi.org/10.1016/j.foodchem.2022.132099 doi: 10.1016/j.foodchem.2022.132099
![]() |
[42] |
Zhang LH, Zhang M, Mujumdar AS (2021) Technological innovations or advancement in detecting frozen and thawed meat quality: A review. Crit Rev Food Sci Nutr, 1–17. https://doi.org/10.1080/10408398.2021.1964434 doi: 10.1080/10408398.2021.1964434
![]() |