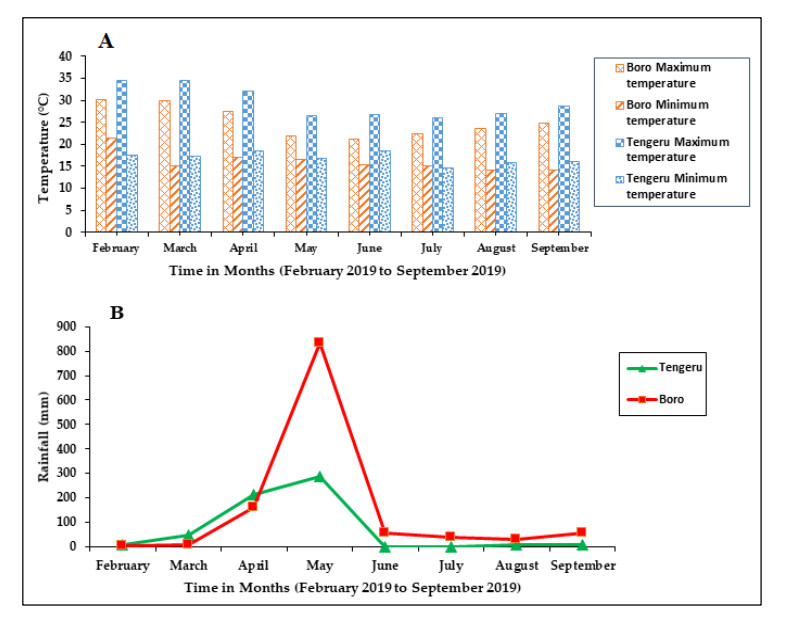
Citation: Nelson Mpumi, Revocatus L. Machunda, Kelvin M. Mtei, Patrick A. Ndakidemi. Insecticidal Efficacy of Syzygium aromaticum, Tephrosia vogelii and Croton dichogamus Extracts against Plutella xylostella and Trichoplusia ni on Brassica oleracea crop in Northern Tanzania[J]. AIMS Agriculture and Food, 2021, 6(1): 185-202. doi: 10.3934/agrfood.2021012
[1] | María Cámara-Ruiz, José María García Beltrán, Francisco Antonio Guardiola, María Ángeles Esteban . In vitro and in vivo effects of purslane (Portulaca oleracea L.) on gilthead seabream (Sparus aurata L.). AIMS Agriculture and Food, 2020, 5(4): 799-824. doi: 10.3934/agrfood.2020.4.799 |
[2] | Clarissa Trindade, Carla S.S. Gouveia, Maria Cristina O. Oliveira, Humberto Nóbrega, José G.R. de Freitas, Miguel A.A. Pinheiro de Carvalho . Inhibitory activity of the peel and seed of Annona cherimola Mill. against Fusarium and Aspergillus pathogens. AIMS Agriculture and Food, 2025, 10(1): 155-176. doi: 10.3934/agrfood.2025009 |
[3] | Tarik Ainane, Fatouma Mohamed Abdoul-Latif, Asmae Baghouz, Zineb El Montassir, Wissal Attahar, Ayoub Ainane, Angelo Maria Giuffrè . Correction: Essential oils rich in pulegone for insecticide purpose against legume bruchus species: Case of Ziziphora hispanica L. and Mentha pulegium L.. AIMS Agriculture and Food, 2023, 8(1): 236-238. doi: 10.3934/agrfood.2023013 |
[4] | Lenin O. Nevárez-Prado, Nubia Amaya-Olivas, Alejandro Sustaita-Rodriguez, Jesús Rodríguez-Zapién, Erick Zúñiga-Rodríguez, María Cordova-Lozoya, Antonio García-Triana, Fabiola Sandoval-Salas, León Hernández-Ochoa . Chemical composition and toxicity of extracts of Fouquieria splendens against Artemia salina. AIMS Agriculture and Food, 2022, 7(2): 357-369. doi: 10.3934/agrfood.2022023 |
[5] | Nicolas Nagahama, Bruno Gastaldi, Michael N. Clifford, María M. Manifesto, Renée H. Fortunato . The influence of environmental variations on the phenolic compound profiles and antioxidant activity of two medicinal Patagonian valerians (Valeriana carnosa Sm. and V. clarionifolia Phil.). AIMS Agriculture and Food, 2021, 6(1): 106-124. doi: 10.3934/agrfood.2021007 |
[6] | Talita Loureiro, Berta Gonçalves, Luís Serra, Ângela Martins, Isabel Cortez, Patrícia Poeta . Histological analysis of Xylella fastidiosa infection in Quercus pyrenaica in Northern Portugal. AIMS Agriculture and Food, 2024, 9(2): 607-627. doi: 10.3934/agrfood.2024033 |
[7] | Oksana B. Polivanova, Mikhail Yu. Cherednichenko, Elena A. Kalashnikova, Rima N. Kirakosyan . In vitro antibacterial effect of silver nanoparticles synthetized using Agastache foeniculum plant and callus extracts. AIMS Agriculture and Food, 2021, 6(2): 631-643. doi: 10.3934/agrfood.2021037 |
[8] | Fitri Damayanti, Salprima Yudha S, Aswin Falahudin . Oil palm leaf ash's effect on the growth and yield of Chinese cabbage (Brassica rapa L.). AIMS Agriculture and Food, 2023, 8(2): 553-565. doi: 10.3934/agrfood.2023030 |
[9] | The Han Nguyen, Patrick Achiever Blamo Jr, Xiaoyong Liu, Thi Van Anh Tran, Sang Moo Kim . β-Glucuronidase inhibitory activity of bromophenol isolated from red alga Grateloupia lancifolia. AIMS Agriculture and Food, 2021, 6(2): 551-559. doi: 10.3934/agrfood.2021032 |
[10] | Justyna Nasiłowska, Barbara Sokołowska, Monika Fonberg-Broczek . Escherichia coli and Listeria innocua stability in carrot juice preserved by high hydrostatic pressure. AIMS Agriculture and Food, 2022, 7(3): 623-636. doi: 10.3934/agrfood.2022039 |
Cabbage, Brassica oleracea var. capitata, is vital vegetable crop grown as an annual plant worldwide [1]. Cabbage is a leafy vegetable of Brassica family and is round, oblate and pointed shapes [2]. Cabbage is intensively grown by poor smallholder farmers of African countries for subsistence and a source of income [3]. The cabbage plant grows well on a well-drained soil, ranging from lighter sand and heavier clay preferring fertile soils and has soft, light green and whitish inner leaves covered with harder and dark green outer leaves [1]. Cabbage is the source of vitamins K and C, the dietary fibers and nutritional elements mainly potassium and Manganese [4].
Despite the nutritional and economical importance, the gardening of B. oleracea by smallholder farmers in Africa is constrained by several insect pest infestations. The high nutritional content contained in B. oleracea makes it attractive to various phytophagous insect pests which feed and cause severe damages and economic losses to it [5]. Specifically, Diamondback moth (Plutella xylostella L. Lepidoptera: Plutellidae) and the cabbage looper (Trichoplusia ni (Hübner) Lepidoptera: Noctuidae) infest severely the B. oleracea on the field and reduces the quality and marketability of the crop [1,6].
Diamondback moth (P. xylostella) is the most severely destructive insect pests of B. oleracea in many parts of Africa and are particularly damaging in the tropics and subtropics [7,8]. P. xylostella larvae feed on the leaves between the large veins and midribs of cruciferous crops and the plants which produce glucosinolates [2]. The P. xylostella larvae prefer to feed on the lower surface of the leaf which as a result leave a "window-paning" effect [9]. The severe feeding result into stunts and destroys the heads of B. oleracea and can cause abortion of the heads resulting into huge yield loss [10]. The T. ni larvae create irregular holes of different shapes when feeding on the leaves [11]. The created holes inhibit the growth and marketability of B. oleracea. T. ni is difficult to control and manage due to its broad distribution and resistance to many insecticides [11,12].
The management of P. xylostella and T. ni is inefficient in most African smallholder farmers [3]. Most of African B. oleracea gardeners in which the use of modern technology is limited prefer cultural practices such as site selection, crop rotation and seed selection, sowing date, row spacing, plant density and weed control to reduce insect infestation in B. oleracea [6,13]. For example, T. ni can be controlled by crop rotation when lettuce is introduced into the garden after B. oleracea [2]. Also, using clean planting materials and transplanting only healthy and vigorous insect-free seedlings, reduce the infestation of B. oleracea insect pests in the field [2]. However, those cultural practices are less effective to protect B. oleracea from P. xylostella and T. ni infestations although, they are cheap and safe to the environment. Natural enemies of insects like predators, parasitoids and pathogens support to reduce B. oleracea insect pest infestations [8]. But the natural enemies are also insufficiently effective to control P. xylostella and T. ni in the fields [2]. Therefore, the smallholder famers in African countries prefer intensively on the application of broad-spectrum synthetic pesticides to control the B. oleracea insect pest in the field [14]. The unselective and arduous use of broad-spectrum insecticides affect the ecosystems of useful natural enemies like bees, butterflies [1] and lady bird beetles [15,16]. Moreover, the massive application of synthetic pesticides contributes to a serious environmental pollution especially water and soil pollution [17]. The surface and ground water pollution due to synthetic pesticides, results into destruction of aquatic ecosystems [18].
However, botanical pesticides like T. vogelii and Azadirachta indica, Annona squamosa, Cupscum frutensces, Allium sativa are the encouraging alternatives and have been used to control insect pests in cereal crops successfully [16,19]. Botanical pesticides have been used broadly in the protection of crops like beans, cowpeas and maize from insect pest infestations in the field and during storage [13]. Botanical pesticides contain groups of active compounds of diverse chemical nature and have an average residual life of 2-5 days [3]. Botanical pesticides are affordable [20], easy to prepare and use [19], environmentally friendly [21], degraded rapidly in sunlight, air, and moisture and are readily broken down by detoxification enzymes and have reduced risks of toxicity to human and to non-target organisms [19,22].
In the present study, the efficacy of phytochemicals from T. vogelii, C. dichogamus and S. aromaticum were assessed to control P. xylostella and T. ni in B. oleracea crop on the field. Normally, T. vogelii is known as the "fish bean", "fish-poison bean", or "vogel's tephrosia" [23]. Smallholder farmers in many countries in Africa use T. vogelii as an organic pesticide to control pests on livestock, in cultivated fields and as medicine for skin diseases [24]. Apart from that, S. aromaticum contains a chemical known as eugenol [25]. This chemical compound in S. aromaticum is used as an insect repellent. This property of insect repellent has been reported to be potential in agriculture to protect foods from micro-organisms during storage [26]. Moreover, S. aromaticum has a variety of pharmacological activities including antimicrobial, anti-inflammatory, analgesic, anti-oxidant and anticancer activities [27]. Also, globally, Croton species are usually used as folk medicines for the treatment of various health problems such as cancer, constipation and diabetes [28]. The phytochemical investigations of the Croton species revealed the presence of various secondary metabolites including alkaloids, phenolics and terpenoids in all plant's parts [29]. However, there is limited information on the applications of S. aromaticum, T. vogelii, C. dichogamus aqueous extracts to control P. xylostella and T. ni of B. oleracea crop in the field. Therefore, this study focused on assessment of insecticidal efficacy of phytochemicals extracted from T. vogelii, C. dichogamus and S. aromaticum to control P. xylostella and T. ni of B. oleracea in northern part of Tanzania.
The study was conducted in Northern part of Tanzania. The sites of the study were located in Arusha region and in Kilimanjaro region in Tanzania. In Kilimanjaro region, the experiment was set in Boro site located at Latitude 3°17'31.5"S and Longitude 37°17'49.1"E and an elevation of 1078 m above sea level. In Arusha region, the experiment was set in Tengeru site located at latitude 3°23'4.5"S and longitude 36°48'26.7"E at an elevation of 1262 m above sea level. The meteorological data of rainfall and temperature was also observed from the experimental locations.
The fresh plant materials were collected from different locations in Manyara, Arusha, Tanga and Kilimanjaro regions in Tanzania. Leaves of C. dichogamus and T. vogelii was collected from Manyara, Arusha and Kilimajaro regions of Tanzania. Flower buds was collected from S. aromaticum plant in Tanga Region. The leaves of plants were separately washed thoroughly with water and air dried under shade and at room temperature for seven days. The dried leaves of C. dichogamus and T. vogelii, and flower buds of S. aromaticum was pulverized into fine particles (powder) using electric blender. The fine powder was extracted separately using water and soap to get the aqueous plant extracts for field experiments. Soap was used during extraction experimental procedure because firstly, it helps to extract compounds which are not water soluble from plant materials and secondly, it helps to spread the extract onto the plant leaves more effectively during applications.
The land was cleared and prepared prior to transplanting of seedlings. Ploughing and harrowing was performed on the land before transplanting of the seedlings at both sites using a plough. The Cabbage (B. oleracea) seeds were sown near the experimental plots on March 2019, then after 5 weeks, were transferred and transplanted into the experimental plots from the mid of April to August 2019 at both study sites. The cabbage was planted at spacing of 50 cm between the rows and 50 cm in the rows in the plots that is measured 2.0 m × 2.5 m at both experimental sites. Watering was done twice a day in the morning and in the evening.
The experiment was designed in a Randomized Complete Block Design (RCBD) with 12 treatments replicated four times. The treatments consisted aqueous plant extracts of three pesticidal plants (T. vogelii, C. dichogamus and S. aromaticum), two negative control (water only and water plus soap) and one positive insecticide control (chlorpyrifos) was also included in the treatments. From each individual plant, three concentrations 1%, 5% and 10% w/v of aqueous plant extracts were prepared to spray on the B. oleracea crop field. The concentrations of aqueous plant extracts were prepared in water containing 0.1% soap from dry powder of T. vogelii, C. dichogamus and S. aromaticum. Thus, 1%, 5% and 10% of aqueous plant extracts was prepared by dissolving 10 g, 50 g and 100 g of powder into one litre of water respectively. The extraction experiment was left to stand for 24 hours at room temperature [15]. There were 12 treatments in each experimental site with 4 plot replicates making a total of 48 plots.
The treatments were sprayed into the B. oleracea crops in the field at the interval of 7 days [16,19] throughout the growing of the B. oleracea crop. The concentration of synthetic insecticide (Chlorpyrifos) was applied as per manufacturers' recommendations. The treatments were sprayed, on top and under the leaves of B. oleracea crop by using a 2 L sprayer in the evening during the growing of the crop. The spraying was done during the evening hours in order to avoid direct sun light which may cause the decomposition of bioactive compounds of the botanicals. Each plot required approximately 250 mL of the aqueous plant extracts at both sites [30]. The sprayer was thoroughly cleaned with water and soap before re-filling it again with another formulation for application.
Insect pests and damage evaluation was done one day before spraying of treatments by randomly selecting 5 inner B. oleracea crops inside the plots each week. The larval population of diamondback moth larvae (P. xylostella) and cabbage looper (T. ni) larvae were counted. Damage of the crops was done by counting the number of damaged leaves and head on weekly basis. Then, the damaged parts (leaves and heads) were differentiated into four scale; 0% damage, up to 25% damage, up to 50% damage, up to 75% damage and up to 100% depending onto the number of damaged leaves and heads [15].
The collected data were analyzed using Statistica 8.0 software package version 7 program. Two-way ANOVA statistical analyses were performed to split plots locations and the treatments. The Fisher's Least Significance Difference (LSD) was used to compare the treatment means at P = 0.05 level of significance.
Figure 1A and 1B shows the temperatures and precipitations of the two experimental study sites. It was observed that, the temperatures of Boro study site were lower than that of Tengeru study site (Figure 1A) during the experimental period (2019 season) (Figure 1B). Therefore, Boro study site was cooler than Tengeru study site. Figure 1B showed the rainfall precipitations of the two study sites. It was observed that, the rainfall precipitations of Boro study site were higher than that of Tengeru study site and the high difference in rain precipitations was observed during April-June at the two experimental study sites. Those weather conditions have affected either by lowering or by increasing the population abundance of P. xylostella and T. ni. The mean maximum and minimum temperatures of Boro experimental site were 25.16 and 16.11 ℃, respectively while the mean maximum and minimum temperatures of Tengeru experimental site were 29.54 and 16.91 ℃. The mean maximum rainfall precipitations of Boro and Tengeru experimental sites were 148.05 and 70.81 mm respectively.
The larval population of diamondback moth (P. xylostella) were observed on B. oleracea crop 4 weeks after transplanting and their larvae were recorded each week of the assessment in the field plots until the B. oleracea crops were harvested. The larvae were mostly found in the underside of the leaves of the B. oleracea crops. It was observed that, at 1st, 2nd, 3rd, 4th and 5th week after the application of treatments, the population of P. xylostella larvae were significantly (P ≤ 0.001) higher (0.41, 0.56, 0.87, 0.71 and 0.96) at Boro site than the population (0.09, 0.23, 0.30, 0.23 and 0.44) at Tengeru site (Table 1). A gradual increase in population of insect pests was noticed over weekly interval throughout the growing season at both experimental sites (Table 1).
Location and treatments | Week 1 before Treatment | Weeks after treatments | ||||
1 | 2 | 3 | 4 | 5 | ||
Location | ||||||
Tenger | 0.08 ± 0.02a | 0.09 ± 0.03b | 0.23 ± 0.08b | 0.30 ± 0.10b | 0.23 ± 0.08b | 0.44 ± 0.11b |
Boro | 0.07 ± 0.02a | 0.41 ± 0.11a | 0.56 ± 0.12a | 0.87 ± 0.16a | 0.71 ± 0.15a | 0.96 ± 0.24a |
Treatments | ||||||
Water (−ve control) | 0.13 ± 0.05a | 1.13 ± 0.53a | 1.68 ± 0.41a | 2.28 ± 0.44a | 2.20 ± 0.53a | 3.28 ± 0.60a |
Water + soap (−ve control) | 0.10 ± 0.04a | 0.75 ± 0.15ab | 1.60 ± 0.31a | 2.58 ± 0.36a | 1.83 ± 0.35a | 3.30 ± 0.49a |
Synthetic pesticide (+ve control) | 0.00 ± 0.00a | 0.05 ± 0.10c | 0.03 ± 0.03b | 0.28 ± 0.15b | 0.08 ± 0.04b | 0.08 ± 0.04b |
C. dichogamus (1%) | 0.13 ± 0.05a | 0.28 ± 0.12bc | 0.25 ± 0.09b | 0.30 ± 0.10b | 0.23 ± 0.10b | 0.33 ± 0.08b |
C. dichogamus (5%) | 0.08 ± 0.04a | 0.15 ± 0.10c | 0.33 ± 0.06b | 0.20 ± 0.08b | 0.23 ± 0.10b | 0.20 ± 0.07b |
C. dichogamus (10%) | 0.03 ± 0.03a | 0.08 ± 0.04c | 0.20 ± 0.08b | 0.15 ± 0.05b | 0.13 ± 0.08b | 0.18 ± 0.06b |
S. aromaticum (1%) | 0.18 ± 0.05a | 0.13 ± 0.05c | 0.18 ± 0.10b | 0.35 ± 0.12b | 0.23 ± 0.09b | 0.28 ± 0.04b |
S. aromaticum (5%) | 0.03 ± 0.03a | 0.15 ± 0.07c | 0.10 ± 0.05b | 0.23 ± 0.09b | 0.23 ± 0.08b | 0.18 ± 0.06b |
S. aromaticum (10%) | 0.03 ± 0.03a | 0.03 ± 0.03c | 0.05 ± 0.05b | 0.15 ± 0.08b | 0.18 ± 0.06b | 0.13 ± 0.04b |
T. vogelii (1%) | 0.00 ± 0.00a | 0.10 ± 0.05c | 0.15 ± 0.05b | 0.18 ± 0.07b | 0.20 ± 0.07b | 0.25 ± 0.07b |
T. vogelii (5%) | 0.08 ± 0.05a | 0.08 ± 0.05c | 0.18 ± 0.10b | 0.20 ± 0.08b | 0.13 ± 0.04b | 0.15 ± 0.03b |
T. vogelii (10%) | 0.05 ± 0.05a | 0.08 ± 0.04c | 0.15 ± 0.05b | 0.13 ± 0.08b | 0.05 ± 0.03b | 0.08 ± 0.04b |
2-way ANOVA | (F-Statistics) | |||||
Location | 0.42ns | 15.11*** | 19.55*** | 58.04*** | 38.06*** | 59.75*** |
Treatments | 2.04ns | 5.52*** | 20.22*** | 45.09*** | 28.14*** | 109.40*** |
Location*treatments | 1.95ns | 2.84*** | 3.00*** | 3.64*** | 5.63*** | 17.46*** |
Note: Each value is a mean ± standard error of eight replicates, *, **, and *** significant at P ≤ 0.05, P ≤ 0.01 and P ≤ 0.001 respectively and ns means not significant. Means within the same column followed by the same letter(s) are not significantly different at (P = 0.05) from each other using Fishers Least significant Difference (LSD) test. |
Three (1%, 5% and 10%) concentrations of T. vogelii, C. dichogamus and S. aromaticum aqueous plant extracts were used to control P. xylostella larvae in the B. oleracea crop field at both study sites (Table 1). The results showed that, the aqueous plant extracts and chlorpyrifos treated plots hosted fewer P. xylostella larvae (Table 1) when compared with negative controls (water and water plus soap) (P ≤ 0.001). In negative controls treated plots, the population of P. xylostella larvae were highest throughout the experimental duration as compared to aqueous plant extract treated plots with maximum population of 3.28 and 3.30 during the 5th week for water and water plus soap treated plots, respectively (Table 1). Statistically, aqueous plant extracts were significantly (P ≤ 0.001) as effective as chlorpyrifos in controlling the P. xylostella larvae (Table 1). It was observed that, P. xylostella persisted from week 1 before and from the 1st to the 5th week of the treatments (Table 1) in water and water plus soap and the population of larvae grew progressively in control plots. The interactions (Figure 2) of the weather conditions (rainfall and temperatures) of the study sites and treatments were observed from the 1st week of treatments to the 5th week (Table 1). It was observed that, the combination of rainfall and warm conditions (Figure 2) of the experimental sites and the treatments together significantly (P ≤ 0.01) inhibited the growth and development of P. xylostella in the field (Figure 2).
T. ni larvae were observed on the field 3 weeks after transplanting. Generally, the T. ni larvae were lower in population abundance at both study sites compared to P. xylostella larvae per B. oleracea crop. In the first week before spraying of the treatments, there were very few T. ni larvae in all plots and did not differed significantly (P > 0.05). The number of T. ni larvae per B. oleracea plant over five weeks of spray were shown in Table 2. It was observed that, the population of T. ni larvae were significantly (P ≤ 0.05) higher (0.18, 0.18, 0.23, 0.22) at Tengeru site (Table 3) than (0.09, 0.06, 0.06 and 0.10) at Boro site (Table 2) from the week before application of the treatments to the third week after application of the treatments. It was observed that, at the 1st, 2nd and the 3rd week of the treatments, the population of the T. ni larvae were significantly (P ≤ 0.001) lower at Boro study site compared with Tengeru site (Table 2).
Location and treatments | Week 1 before Treatment | Weeks after treatments | ||||
1 | 2 | 3 | 4 | 5 | ||
Location | ||||||
Tengeru | 0.18 ± 0.02a | 0.18 ± 0.03a | 0.23 ± 0.0a | 0.22 ± 0.07a | 0.17 ± 0.05a | 0.20 ± 0.05a |
Boro | 0.09 ± 0.02b | 0.06 ± 0.03b | 0.06 ± 0.02b | 0.10 ± 0.02b | 0.15 ± 0.04a | 0.14 ± 0.04a |
Treatments | ||||||
Water (−ve control) | 0.18 ± 0.07a | 0.50 ± 0.09a | 0.63 ± 0.30a | 0.60 ± 0.24a | 0.48 ± 0.15a | 0.78 ± 0.16a |
water + soap (−ve control) | 0.10 ± 0.05a | 0.30 ± 0.08b | 0.48 ± 0.20a | 0.68 ± 0.26a | 0.65 ± 0.20a | 0.80 ± 0.18a |
Synthetic pesticide (+ve control) | 0.13 ± 0.05a | 0.05 ± 0.03c | 0.03 ± 0.03b | 0.03 ± 0.03b | 0.03 ± 0.03bc | 0.00 ± 0.00b |
C. dichogamus (1%) | 0.15 ± 0.05a | 0.08 ± 0.04c | 0.05 ± 0.03b | 0.13 ± 0.05b | 0.23 ± 0.10b | 0.08 ± 0.04b |
C. dichogamus (5%) | 0.08 ± 0.04a | 0.10 ± 0.04c | 0.10 ± 0.05b | 0.05 ± 0.03b | 0.13 ± 0.04bc | 0.08 ± 0.04b |
C. dichogamus (10%) | 0.15 ± 0.05a | 0.03 ± 0.03c | 0.08 ± 0.04b | 0.05 ± 0.03b | 0.08 ± 0.05bc | 0.08 ± 0.04b |
S. aromaticum (1%) | 0.10 ± 0.04a | 0.13 ± 0.05c | 0.13 ± 0.08b | 0.05 ± 0.03b | 0.08 ± 0.04bc | 0.08 ± 0.04b |
S. aromaticum (5%) | 0.18 ± 0.03a | 0.05 ± 0.03c | 0.05 ± 0.03b | 0.05 ± 0.03b | 0.00 ± 0.00c | 0.03 ± 0.03b |
S. aromaticum (10%) | 0.10 ± 0.01a | 0.05 ± 0.03c | 0.03 ± 0.03b | 0.00 ± 0.00b | 0.05 ± 0.05bc | 0.03 ± 0.03b |
T. vogelii (1%) | 0.13 ± 0.05a | 0.10 ± 0.04c | 0.10 ± 0.05b | 0.18 ± 0.06b | 0.15 ± 0.03bc | 0.10 ± 0.04b |
T. vogelii (5%) | 0.20 ± 0.04a | 0.05 ± 0.03c | 0.05 ± 0.03b | 0.08 ± 0.04b | 0.03 ± 0.03bc | 0.00 ± 0.00b |
T. vogelii (10%) | 0.13 ± 0.04a | 0.00 ± 0.00c | 0.03 ± 0.03b | 0.05 ± 0.03b | 0.03 ± 0.03bc | 0.00 ± 0.00b |
2-way ANOVA | (F-Statistics) | |||||
Location | 12.10*** | 20.44*** | 8.80** | 4.66* | 0.24ns | 2.21ns |
Treatments | 0.67ns | 10.90*** | 3.83*** | 5.53*** | 5.42*** | 16.01*** |
Location*treatments | 0.26ns | 1.05ns | 1.96* | 2.46** | 0.37ns | 1.16ns |
Note: Each value is a mean ± standard error of eight replicates, *, **, and *** significant at P ≤ 0.05, P ≤ 0.01 and P ≤ 0.001 respectively and ns means not significant. Means within the same column followed by the same letter(s) are not significantly different at (P = 0.05) from each other using Fishers Least significant Difference (LSD) test. |
Location and treatments | Week 1 before Treatment | Weeks after treatments | ||||
1 | 2 | 3 | 4 | 5 | ||
Location | ||||||
Tengeru | 19.7 ± 1.1a | 19.1 ± 1.8a | 18.0 ± 2.0a | 16.3 ± 2.1a | 15.3 ± 1.9a | 19.9 ± 2.8a |
Boro | 10.1 ± 0.5b | 10.3 ± 1.0b | 11.8 ± 1.1b | 15.5 ± 1.4a | 18.3 ± 2.0a | 21.8 ± 3.2a |
Treatments | ||||||
Water (−ve control) | 18.8 ± 3.1a | 33.8 ± 5.6a | 33.1 ± 4.5a | 38.8 ± 2.3a | 45.0 ± 4.3a | 70.6 ± 3.1a |
water + soap (−ve control) | 15.6 ± 4.4a | 30.0 ± 5.7a | 31.9 ± 3.7a | 41.3 ± 3.1a | 41.3 ± 3.0a | 56.3 ± 2.3b |
Synthetic pesticide (+ve control) | 16.3 ± 2.6a | 8.1 ± 1.6c | 6.3 ± 1.6e | 6.9 ± 1.9e | 7.5 ± 1.6d | 5.0 ± 1.3g |
C. dichogamus (1%) | 16.9 ± 2.8a | 16.3 ± 3.2b | 17.5 ± 3.0b | 17.5 ± 1.9b | 18.1 ± 1.9b | 18.8 ± 1.8c |
C. dichogamus (5%) | 15.0 ± 2.7a | 10.0 ± 1.3bc | 13.8 ± 3.5bcde | 15.0 ± 2.7bc | 15.0 ± 2.1bc | 18.8 ± 1.8c |
C. dichogamus (10%) | 13.8 ± 1.8a | 11.3 ± 1.6bc | 11.3 ± 1.6bcde | 11.3 ± 0.8cde | 11.3 ± 2.1cd | 12.5 ± 2.8de |
S. aromaticum (1%) | 14.4 ± 2.4a | 13.8 ± 2.5bc | 15.6 ± 2.4bcd | 15.0 ± 1.3bc | 17.5 ± 1.9b | 18.1 ± 1.6c |
S. aromaticum (5%) | 12.5 ± 1.3a | 10.6 ± 1.1bc | 10.0 ± 0.0bcde | 8.1 ± 1.3e | 8.8 ± 1.3d | 10.6 ± 1.5ef |
S. aromaticum (10%) | 14.4 ± 2.2a | 8.8 ± 1.8bc | 8.1 ± 0.9de | 6.9 ± 1.3e | 8.1 ± 1.6d | 9.4 ± 1.5efg |
T. vogelii (1%) | 11.9 ± 3.3a | 12.5 ± 1.9bc | 16.3 ± 4.6bc | 13.8 ± 2.3bcd | 15.0 ± 0.9bc | 17.5 ± 1.3cd |
T. vogelii (5%) | 16.3 ± 3.0a | 11.3 ± 1.6bc | 8.8 ± 1.8cde | 8.8 ± 0.8de | 6.9 ± 1.6d | 6.9 ± 1.6fg |
T. vogelii (10%) | 11.9 ± 1.6a | 10.0 ± 1.9bc | 6.3 ± 1.6e | 7.5 ± 1.3e | 7.5 ± 1.3d | 5.6 ± 1.1fg |
2-way ANOVA | ||||||
Location | 77.06*** | 45.03*** | 18.56*** | 0.51ns | 6.05** | 2.89ns |
Treatments | 1.19ns | 13.70*** | 12.98*** | 44.96*** | 37.05*** | 117.55*** |
Location*treatments | 1.53ns | 1.94ns | 1.28ns | 1.90ns | 0.57ns | 0.77ns |
Note: Each value is a mean ± standard error of eight replicates, *, **, and *** significant at P ≤ 0.05, P ≤ 0.01 and P ≤ 0.001 respectively and ns means not significant. Means within the same column followed by the same letter(s) are not significantly different at (P = 0.05) from each other using Fishers Least significant Difference (LSD) test. |
The result showed that, the aqueous plant extracts and chlorpyrifos treated plots hosted fewer T. ni larvae compared with the negative controls (water and water plus soap) (Table 2). In the negative control plots, T. ni larvae were highest of all the treated plots (Table 2). The aqueous plant extracts from T. vogelii, C. dichogamus and S. aromaticum were significantly (P ≤ 0.05) effective as synthetic pesticide (Chlorpyrifos) in controlling the cabbage looper (T. ni) larvae (Table 2). Also, there was interactions of the weather conditions of the experimental sites and treatments which was observed at the 2nd and 3rd week of application of treatments (Figure 3). It was revealed that, the combination of the weather conditions of the experimental sites and the treatments together significantly (P ≤ 0.01) reduced the population of T. ni in the field.
Generally, the damage to B. oleracea caused by P. xylostella and T. ni larvae were significant (P ≤ 0.001) at the two study sites (Boro and Tengeru) (Table 3). At the 1st week before application of the treatments and the 1st and the 2nd weeks after application of treatments the damage percentage of B. oleracea was significantly (P ≤ 0.001) lower (10.1, 10.3 and 11.8) at Boro site than (19.7, 19.1, 18.0) at Tengeru site respectively (Table 3). At the 3rd, 4th and the 5th weeks of the treatments, the percentages of B. oleracea damage was significantly (P ≤ 0.001) the same at both study sites.
Before treatments, there was non-significant different (P > 0.05) of B. oleracea percentage damage caused by P. xylostella and T. ni larvae in all treatments in different plots (Table 3). After the treatments, the aqueous plant extracts of S. aromaticum and T. vogelii at 10% concentration each, exhibited significantly (P ≤ 0.05) the lowest (8.8, 8.1, 6.9, 8.1 and 9.4) and (10.0, 6.3, 7.5, 7.5 and 5.6), percentage damage of B. oleracea at the 1st, 2nd, 3rd, 4th and the 5th of application of the treatments respectively. Moreover, the percentage of B. oleracea damage in the water (33.8, 33.1, 38.8, 45.0 and 70.6) and water plus soap (30.0, 31.9, 41.3, 41.3 and 56.3) was higher than in the T. vogelii, C. dichogamus and S. aromaticum aqueous plant extracts and chlorpyrifos pesticide treated plots (Table 3) from the first week to the 5th week of the treatments.
For the three concentrations (1%, 5% and 10%), the 10% of T. voelii and S. aromaticum aqueous plant extracts showed the least percentage damage (6.3%, 7.5%, 7.5% and 5.6%) and (8.1%, 6.9%, 8.1% and 9.4%) of the B. oleracea respectively compared with the 5 % and 1 % concentrations (Table 3). The 10% of the T. voelii and S. aromaticum aqueous plant extracts were as effective as synthetic pesticide (6.3, 6.9, 7.5 and 5.0) in reduction of the percentage damage of B. oleracea. The other concentrations (1% and 5%) of the aqueous plant extracts reduced the damage of B. oleracea compared with negative controls (Table 3).
Figure 4 indicates the relationship between the population of P. xlostella and T. ni and the percentage damage incurred in the negative controls and the treatments. It was revealed that, the percentage damage was highest in the negative controls (water and water plus soap) because the populations of P. xlostella and T. ni were higher compared with the pesticide and plant extract treatments. Also, it was found that, the percentage damage was lowest in the synthetic pesticide used (chlorpyrifos) followed by T. vogelli (10%) and S. aromaticum (10%). Moreover, C. dichogamus (10%), T. vogelii (5%), S. aromaticum (5%) and C. dichogamus (5%) aqueous plant extracts lowered the percentage damage of B. oleracea plant compared with 1% of extracts from each pesticidal plant (Figure 4).
Further correlation analysis (Table 4), clearly showed that, the population of P. xylostella and T. ni have positive and significantly (P < 0.001) very strong relationship with the damage score of the B. oleracea. From this study, the population of P. xylostella larvae has positive (R = 0.976) and significantly (P < 0.001) very strong correlation with the percentage damage of B. oleracea. Moreover, population of T. ni has positive (R = 0.990) and significantly (P < 0.001) very strong correlation with the percentage damage of B. oleracea crop. Therefore, due to strong infestation and damage caused by P. xylostella and T. ni, the smallholder farmers are convinced to increase the rate and the dose of synthetic pesticides to the field during the control and management of those insect pests.
P. xylostella | T. ni | Damage (%) | |
P. xylostella | 1.000 | ||
T. ni | 0.993 (<0.001) | 1.000 | |
Damage (%) | 0.976 (<0.001) | 0.99 (<0.001) | 1.000 |
Keys: In brackets are the P—values of significant correlations of measured variables. |
Generally, the population of P. xylostella and T. ni larvae from this study differed significantly from one study site to another. The population of T. ni larvae were significantly higher at Tengeru site than at Boro site. The population of P. xylostella larvae were significantly (P 0.01) higher at Boro site than at Tengeru site. These results could be contributed by variations of weather conditions of the two experimental sites and the building up of P. xylostella and T. ni larvae. Moreover, the building up of the two larvae could be contributed by the species diversity and present of natural enemies. For instance, the building up of P. xylostella at Boro site could have contributed the high damaging effect of the B. oleracea at Boro site at 3rd, 4th and 5th of the application of the treatments. Extreme weather conditions like heavy rainfall, either high or low temperatures and high humidity have strong influences on the population abundance of the insects in their ecosystem [31]. At Boro site the rainfall ranged from 5 mm to about 883 mm and the temperature ranged from 22 to 30.2 ℃ during the study period march to August 2019 which mighty have enhanced the growth and development of P. xylostella. However, at Tengeru experimental site, the rainfall precipitations and temperatures were lower which ranged from 0 mm to 287 mm and 25.9 to 34.5 ℃, respectively. These variations in rainfall and temperatures could have affected significantly (P ≤ 0.05) the population abundance of the P. xylostella and T. ni larvae in the two experimental sites. As results, the population abundance of the T. ni larvae were significantly (P ≤ 0.05) higher at Tengeru study site than at Boro site while, the population abundance of P. xylostella was higher at Boro study site than at Tengeru study site.
The higher rainfall precipitations and lower temperatures of the Boro site might have had contributed the higher populations of P. xylostella larvae compared with Tengeru study site whereby the population was low. The results concur with Tanyi et al. [32] and Patra [31] who reported that, P. xylostella is favoured by warm conditions and rain precipitations. Moreover, Ayalew et al. [33] showed that, rain precipitation and temperatures ranging from 25 to 33 ℃ have significant influences on the population of P. xylostella. According to them the increase of population of P. xylostella was reported to be positively correlated with high rain precipitations and the temperatures which ranges from 25 to 33 ℃. It was clear from this study that, the population abundance of P. xylostella and T. ni larvae varies from one location to another location due weather conditions variability, species diversity and distribution of natural enemies. Those results concur with Ayalew and Ogol [33] who indicated that, the importance of a particular insect pest varies from location to location due to differences in rainfall precipitations and temperatures.
The distribution of P. xylostella larvae and T. ni larvae in the 1st week before application of the aqueous plant extracts and the chlorpyrifos was low and was not significantly different and the infestation was less intense because the B. oleracea plants were still young. In the negative controls (water and water plus soap) plots the population of P. xylostella larvae persisted from week 1 up to week 6 and the infestation of the B. oleracea crops increased progressively week after week. After application of the treatments, it was observed that, the aqueous plant extracts from T. vogelii, C. dichogamus and S. aromaticum significantly lowered (P ≤ 0.05) the population of T. ni and P. xylostella larvae on B. oleracea crops and reduced the damage of B. oleracea. However, on the negative control plots, the infestations and damage increased progressively. It was found that, the aqueous plant extracts were as effective as synthetic pesticide (Chlorpyrifos) for controlling P. xylostella and T. ni larvae in the B. oleracea crop in the field. The effectiveness of botanical pesticides used for controlling P. xylostella larva and T. ni larvae in the field could be contributed by the presence of active chemical compounds in the studied pesticidal plants [34].
The 10% concentration of the aqueous plant extracts was more effective in reduction of the population of T. ni and P. xylostella larvae and the damage of B. oleracea compared with the other tested concentrations (1% and 5%). In general, the botanicals used were effective as chlorpyrifos in reducing the population of T. ni and P. xylostella larvae and the damage of B. oleracea crop in the field. The results obtained agree with the previous studies [16,35,36,37,38,39,40,41,42]. Kamanula et al. [40] and Grzywacz et al. [38] reported that, the control of insect pests using pesticidal plant extracts could be contributed by the presence of insecticidal bioactive compounds in those pesticidal plants. Belmain et al. [30] reported the present of rotenone in T. vogelii, which could be responsible for T. ni and P. xylostella larvae control efficacy. Khater [43] indicated that, rotenone is a contact and stomach poison which limits the electron transport chain in the Mitochondria.
Apart from that, this study revealed that, all concentrations of the aqueous extracts used from S. aromaticum significantly (P ≤ 0.05) reduced the population of T. ni and P. xylostella larvae when compared with negative controls (water and water plus soap). These results agree with Kamatou et al. [25], Araujo et al. [27] and Tian et al. [26]. Kamatou et al. [25] and Araujo et al. [27] reported that, S. aromaticum contains eugenol, β-caryophyllene, α-humulene and eugenol acetate and eugenol being the most active compound responsible for imparting the taste of the S. aromaticum (Figure 5). Because of that, eugenol is the bioactive chemical compound of S. aromaticum which can be used as repellent. Tian et al. [26] revealed that, S. aromaticum has a range of pharmacological activities which includes antimicrobial, anti-inflammatory, analgesic, anti-oxidant and anticancer activities, amongst others and therefore can be used in agricultural to protect crops and foods from micro-organisms during storage. In addition, S. aromaticum might have an effect on insect as a pesticide and fumigant [25,26]. Araujo et al. [27] showed that, the essential oils from S. aromaticum exhibited larvicidal activity against resistant populations of Aedes aegypti.
Also, this study reported that, all concentrations (1%, 5% and 10%) of the extracts used from C. dichogamus significant (P ≤ 0.05) reduced the T. ni and P. xylostella larvae when compared with negative controls. These results agree with Aldhaher et al. [29] and Silva et al. [28] who reported that, the Croton species possesse alkaloids, phenolics, terpenoids including monoterpenes, sesquiterpenes and diterpenes in all plant parts. Those compounds could be responsible for the insecticidal, repellents and deterrent effects to T. ni and P. xylostella larva in this study. In Africa, America and Asia croton species are commonly used as folk medicines in the treatment of cancer, constipation, diabetes, digestive problems, dysentery, external wounds, fever, hypercholesterolemia, hypertension, inflammation, intestinal worms, malaria, pain, ulcers and weight-loss [28] due to the present of those chemicals. These results agree with Aldhaher et al. [29] who reported that Croton species contain chemicals which are responsible for insecticidal activity.
The interactions of the site's weather condition (Temperatures and rainfall) and treatments was observed in some weeks during the application of aqueous plant extracts and organophosphate pesticide for reduction of the P. xylostella and T. ni larvae. The interactions of weather conditions (temperatures and rainfall precipitations) and treatments have significant (P 0.05) effect on the population abundance of P. xylostella and T. ni larvae. It was revealed that, the variations in weather conditions caused P. xylostella and T. ni larvae to be reduced more in the treatment plots compared with negative controls at both experimental sites. The vegetation density of a particular environment, predation exposure, and proliferation of natural enemies together with the treatments mighty have affected the reproduction capacity, growth and development of P. xylostella and T. ni larvae in the field. It is important to note that, the low and high temperatures have effect on the survival and development of P. xylostella larvae. High temperatures (above 30 ℃) for instance can reduce the reproduction capacity, growth and development of P. xylostella whereas low temperatures can favour the P. xylostella larvae survivorship [44]. P. xylostella larvae survive well in the regions in which the temperature is not more than 30 ℃ [44]. The combinations of fluctuations of rainfall precipitations and the treatments reduced the population of the P. xylostella at the experimental sites. Similarly, those results agree with Tanyi et al. [32], Patra [31], Kobori and Amano [45] who reported that, population abundance of P. xylostella is affected by rainfall precipitations. Moreover, most of T. ni larvae lay many eggs on the upper leaf surface of the host plants and few are laid on the lower surface. In heavy rainfall precipitations, the eggs of T. ni on the upper surface of the leaf are washed away and few eggs remain which hatch into few larvae and inhibit the reproduction capacity of T. ni larvae. Therefore, the combinations of temperatures and rainfall precipitations and treatments reduced the reproduction capacity of T. ni hence inhibited the population of the insect pest at both experimental sites. Therefore, smallholder farmers can manage the larvae of P. xylostella and T. ni by considering the weather conditions of a particular location and the botanicals in case synthetic pesticides are not affordable.
The three concentrations (1%, 5% and 10%) of the plant extracts used in this study were able to reduce the population of T. ni and P. xylostella larvae and the damage of B. oleracea crop in the field. Therefore, T. vogelii, C. dichogamus and S. aromaticum are at 10% w/v aqueous extracts is potential for African smallholder farmers for controlling T. ni, and P. xylostella larvae infestation and to increase their crop yield. Due to the efficacy of aqueous plant extracts against T. ni and P. xylostella larvae, these plants can be used as alternatives to synthetic pesticides.
This work was supported by African Development Bank (AfDB) through The Nelson Mandela African Institution of Science and Technology (NM-AIST), Arusha, Tanzania.
The authors are grateful to The Nelson Mandela African Institution of Science and Technology, Arusha (NM-AIST) Tanzania for providing institutional support for this study and African Development Bank (AfDB) for funding the study. Also, I thank the Tanzania Agricultural Research Institute (TARI), Tengeru center, Arusha, Tanzania and Wazazi Association in Boro Village in Kilimajaro region, Tanzania for providing land for establishing the study plots. We thank the technicians of the institutions for field assistance and data collection.
The authors declare no conflicts of interest regarding the publication of this paper.
[1] | Baidoo P, Mochiah M (2016) Comparing the effectiveness of garlic (Allium sativum L.) and hot pepper (Capsicum frutescens L.) in the management of the major pests of cabbage Brassica oleracea (L.). Sustainable Agric Res 5: 83-91. |
[2] | Mpumi N, Machunda RS, Mtei KM, et al. (2020) Selected insect pests of economic importance to Brassica oleracea, their control strategies and the potential threat to environmental pollution in Africa. Sustainability 12: 1-22. |
[3] | Mudzingwa S, Muzemu S, Chitamba J (2013) Pesticidal efficacy of crude aqueous extracts of Tephrosia vogelii L., Allium sativum L. and Solanum incanum L. in controlling aphids (Brevicoryne brassicae L.) in rape (Brassica napus L.). J Agric Res 2: 157-163. |
[4] | Norman C, Shealy M (2007) Illustrated Encyclopedia of Healing Remedies. Toledo, OH, USA: Elements Book Inc. |
[5] | Gibson A (2012) Colewart and the cole crops. Los Angeles: University of California Los Angeles. |
[6] | Baidoo P, Adam J (2012) The Effects of Extracts of Lantana camara (L.) and Azadirachta indica (A. Juss) on the Population Dynamics of Plutella xylostella, Brevicoryne brassicae and Hellula undalis on Cabbage. Sustainable Agric Res 1: 229-234. |
[7] | Furlong MJ, Wright DJ, Dosdall LM (2013) Diamondback moth ecology and management: problems, progress, and prospects. Annu Rev Entomol 58: 517-541. |
[8] | Labou B, Brévault T, Sylla S, et al. (2017) Spatial and temporal incidence of insect pests in farmers' cabbage fields in Senegal. Int J Trop Insect Sci 37: 225-233. |
[9] | Begna F, Damtew T (2015) Evaluation of four botanical insecticides against Diamondback Moth, Plutella Xylostella L.(Lepidoptera: Plutellidae) on head cabbage in the central rift valley of Ethiopia. Sky J Agric Res 4: 97-105. |
[10] | Timbilla J, Nyarko K (2004) A survey of cabbage production and constraints in Ghana. Ghana J Agric Sci 37: 93-101. |
[11] | Fening K, Amoabeng B, Adama I, et al. (2013) Sustainable management of two key pests of cabbage, Brassica oleracea var. capitata L.(Brassicaceae), using homemade extracts from garlic and hot pepper. Org Agric 3: 163-173. |
[12] | Capinera J (2001) Handbook of vegetable pests. Eds, editor: Cambridge, MA, USA: Academic Press. |
[13] | Mpumi N, Mtei K, Machunda R, et al. (2016) The toxicity, persistence and mode of actions of selected botanical pesticides in Africa against insect pests in common beans, P. vulgaris: a review. Am J Plant Sci 7: 138-151. |
[14] | Labou B, Brévault T, Bordat D, et al. (2016) Determinants of parasitoid assemblages of the diamondback moth, Plutella xylostella, in cabbage farmer fields in Senegal. Crop Prot 89: 6-11. |
[15] | Mkenda P, Mwanauta R, Stevenson PC, et al. (2015) Extracts from field margin weeds provide economically viable and environmentally benign pest control compared to synthetic pesticides. PLoS ONE 10: 1-14. |
[16] | Mkindi A, Mpumi N, Tembo Y, et al. (2017) Invasive weeds with pesticidal properties as potential new crops. Ind Crops Prod 110: 113-122. |
[17] | Ondieki JJ (1996) The current state of pesticide management in Sub-Saharan Africa. Sci Total Environ 188: 30-34. |
[18] | Weinberger K, Srinivasan R (2009) Farmers' management of cabbage and cauliflower pests in India and their approaches to crop protection. J Asia-Pac Entomol 12: 253-259. |
[19] | Amoabeng BW, Gurr GM, Gitau CW, et al. (2014) Cost: Benefit analysis of botanical insecticide use in cabbage: Implications for smallholder farmers in developing countries. Crop Prot 57: 71-76. |
[20] | de Cássia Seffrin R, Shikano I, Akhtar Y, et al. (2010) Effects of crude seed extracts of Annona atemoya and Annona squamosa L. against the cabbage looper, Trichoplusia ni in the laboratory and greenhouse. Crop Prot 29: 20-24. |
[21] | Mochiah M, Banful B, Fening K, et al. (2011) Botanicals for the management of insect pests in organic vegetable production. J Entomol Nematol 3: 85-97. |
[22] | Isman MB (2006) Botanical insecticides, deterrents, and repellents in modern agriculture and an increasingly regulated world. Annu Rev Entomol 51: 45-66. |
[23] | Orwa C, Mutua A, Kindt R, et al. (2009) Agroforestree database: a tree species reference and selection guide version 4.0. World Agroforestry Centre ICRAF, Nairobi, KE. |
[24] | Munthali MG, Gachene CK, Sileshi GW, et al. (2014) Amendment of Tephrosia Improved Fallows with Inorganic Fertilizers Improves Soil Chemical Properties, N Uptake, and Maize Yield in Malawi. Int J Agron 2014: 1-9. |
[25] | Kamatou GP, Vermaak I, Viljoen AM (2012) Eugenol from the remote Maluku Islands to the international market place: A review of a remarkable and versatile molecule. Molecules 17: 6953-6981. |
[26] | Tian BL, Liu QZ, Liu ZL, et al. (2015) Insecticidal potential of clove essential oil and its constituents on Cacopsylla chinensis (Hemiptera: Psyllidae) in laboratory and field. J Econ Entomol 108: 957-961. |
[27] | Araujo AFdO, Ribeiro-Paes JT, Deus JTd, et al. (2016) Larvicidal activity of Syzygium aromaticum (L.) Merr and Citrus sinensis (L.) Osbeck essential oils and their antagonistic effects with temephos in resistant populations of Aedes aegypti. Memórias do Instituto Oswaldo Cruz 111: 443-449. |
[28] | Silva CG, Oliveira J, da Camara CA (2018) Insecticidal activity of the ethanolic extract from Croton species against Plutella xylostella L.(Lepidoptera: Plutellidae). Revista Facultad Nacional de Agronomía Medellín 71: 8543-8551. |
[29] | Aldhaher A, Langat M, Ndunda B, et al. (2017) Diterpenoids from the roots of Croton dichogamus Pax. Phytochemistry 144: 1-8. |
[30] | Belmain SR, Amoah BA, Nyirenda SP, et al. (2012) Highly variable insect control efficacy of Tephrosia vogelii chemotypes. J Agric Food Chem 60: 10055-10063. |
[31] | Patra S, Dhote V, Alam S, et al. (2013) Population dynamics of major insect pests and their natural enemies on cabbage under new alluvial zone of West Bengal. J Plant Prot Sci 5: 42-49. |
[32] | Tanyi CB, Ngosong C, Ntonifor NN (2018) Effects of climate variability on insect pests of cabbage: adapting alternative planting dates and cropping pattern as control measures. Chem Biol Technol Agric 5: 1-11. |
[33] | Ayalew G, Ogol C (2006) Occurrence of the diamondback moth (Plutella xylostella L.) and its parasitoids in Ethiopia: influence of geographical region and agronomic traits. J Appl Entomol 130: 343-348. |
[34] | Grzywacz D, Rossbach A, Rauf A, et al. (2010) Current control methods for diamondback moth and other brassica insect pests and the prospects for improved management with lepidopteran-resistant Bt vegetable brassicas in Asia and Africa. Crop Prot 29: 68-79. |
[35] | Belmain S, Haggar J, Holt J, et al. (2013) Managing legume pests in sub-Saharan Africa: Challenges and prospects for improving food security and nutrition through agro-ecological intensification. Chatham Maritime (United Kingdom): Natural Resources Institute, University of Greenwich, 34. |
[36] | Belmain S, Stevenson P (2001) Ethnobotanicals in Ghana: Reviving and modernising age-old farmer practice. Pestic Outlook 12: 233-238. |
[37] | Do Ngoc Dai TDT, Ogunmoye A, Eresanya OI, et al. (2015) Chemical constituents of essential oils from the leaves of Tithonia diversifolia, Houttuynia cordata and Asarum glabrum grown in Vietnam. Am J Essent Oils Nat Prod 2: 17-21. |
[38] | Grzywacz D, Stevenson P, Mushobozi WL, et al. (2014) The use of indigenous ecological resources for pest control in Africa. Food Secur 6: 71-86. |
[39] | Ileke K, Oni M (2011) Toxicity of some plant powders to maize weevil, Sitophilus zeamais (motschulsky)[Coleoptera: Curculiondae] on stored wheat grains (Triticum aestivum). Afr J Biotechnol 6: 3043-3048. |
[40] | Kamanula J, Sileshi GW, Belmain SR, et al. (2010) Farmers' insect pest management practices and pesticidal plant use in the protection of stored maize and beans in Southern Africa. Int J Pest Manag 57: 41-49. |
[41] | Mkenda PA, Mtei K, Ndakidemi PA (2014) Pesticidal efficacy of Tephrosia vogelii and Tithonia diversifolia against field insect pests of common beans[Phaseolus vulgaris L.]. within African farming communities. Afr J Appl Agric Sci Technol 2: 9-26. |
[42] | Mwanauta RW, Mtei K, Ndakidemi PA (2014) Prospective Bioactive Compounds from Vernonia amygdalina, Lippia javanica, Dysphania ambrosioides and Tithonia diversifolia in Controlling Legume Insect Pests. Agric Sci 5: 1129-1139. |
[43] | Khater HF (2012) Prospects of botanical biopesticides in insect pest management. Pharmacologia 3: 641-656. |
[44] | Marchioro C, Foerster L (2011) Development and survival of the diamondback moth, Plutella xylostella (L.)(Lepidoptera: Yponomeutidae) as a function of temperature: effect on the number of generations in tropical and subtropical regions. Neotrop Entomol 40: 533-541. |
[45] | Kobori Y, Amano H (2003) Effect of rainfall on a population of the diamondback moth, Plutella xylostella (Lepidoptera: Plutellidae). Appl Entomol Zool 38: 249-253. |
1. | Fabrizia Ratto, Toby Bruce, Gilson Chipabika, Sithembile Mwamakamba, Rachel Mkandawire, Zeyaur Khan, Angela Mkindi, Jimmy Pittchar, Susannah M. Sallu, Stephen Whitfield, Kenneth Wilson, Steven M. Sait, Biological control interventions reduce pest abundance and crop damage while maintaining natural enemies in sub-Saharan Africa: a meta-analysis, 2022, 289, 0962-8452, 10.1098/rspb.2022.1695 | |
2. | Nelson Mpumi, Kelvin M. Mtei, Revocatus L. Machunda, Patrick A. Ndakidemi, Nguya K. Maniania, Efficacy of Aqueous Extracts from Syzygium aromaticum, Tephrosia vogelii, and Croton dichogamus against Myzus persicae on Brassica oleracea in Northern Tanzania, 2021, 2021, 1687-7438, 1, 10.1155/2021/2525328 | |
3. | Gianluca Paventi, Giuseppe Rotundo, Marco Pistillo, Ilaria D’Isita, Giacinto Salvatore Germinara, Bioactivity of Wild Hop Extracts against the Granary Weevil, Sitophilus granarius (L.), 2021, 12, 2075-4450, 564, 10.3390/insects12060564 |
Location and treatments | Week 1 before Treatment | Weeks after treatments | ||||
1 | 2 | 3 | 4 | 5 | ||
Location | ||||||
Tenger | 0.08 ± 0.02a | 0.09 ± 0.03b | 0.23 ± 0.08b | 0.30 ± 0.10b | 0.23 ± 0.08b | 0.44 ± 0.11b |
Boro | 0.07 ± 0.02a | 0.41 ± 0.11a | 0.56 ± 0.12a | 0.87 ± 0.16a | 0.71 ± 0.15a | 0.96 ± 0.24a |
Treatments | ||||||
Water (−ve control) | 0.13 ± 0.05a | 1.13 ± 0.53a | 1.68 ± 0.41a | 2.28 ± 0.44a | 2.20 ± 0.53a | 3.28 ± 0.60a |
Water + soap (−ve control) | 0.10 ± 0.04a | 0.75 ± 0.15ab | 1.60 ± 0.31a | 2.58 ± 0.36a | 1.83 ± 0.35a | 3.30 ± 0.49a |
Synthetic pesticide (+ve control) | 0.00 ± 0.00a | 0.05 ± 0.10c | 0.03 ± 0.03b | 0.28 ± 0.15b | 0.08 ± 0.04b | 0.08 ± 0.04b |
C. dichogamus (1%) | 0.13 ± 0.05a | 0.28 ± 0.12bc | 0.25 ± 0.09b | 0.30 ± 0.10b | 0.23 ± 0.10b | 0.33 ± 0.08b |
C. dichogamus (5%) | 0.08 ± 0.04a | 0.15 ± 0.10c | 0.33 ± 0.06b | 0.20 ± 0.08b | 0.23 ± 0.10b | 0.20 ± 0.07b |
C. dichogamus (10%) | 0.03 ± 0.03a | 0.08 ± 0.04c | 0.20 ± 0.08b | 0.15 ± 0.05b | 0.13 ± 0.08b | 0.18 ± 0.06b |
S. aromaticum (1%) | 0.18 ± 0.05a | 0.13 ± 0.05c | 0.18 ± 0.10b | 0.35 ± 0.12b | 0.23 ± 0.09b | 0.28 ± 0.04b |
S. aromaticum (5%) | 0.03 ± 0.03a | 0.15 ± 0.07c | 0.10 ± 0.05b | 0.23 ± 0.09b | 0.23 ± 0.08b | 0.18 ± 0.06b |
S. aromaticum (10%) | 0.03 ± 0.03a | 0.03 ± 0.03c | 0.05 ± 0.05b | 0.15 ± 0.08b | 0.18 ± 0.06b | 0.13 ± 0.04b |
T. vogelii (1%) | 0.00 ± 0.00a | 0.10 ± 0.05c | 0.15 ± 0.05b | 0.18 ± 0.07b | 0.20 ± 0.07b | 0.25 ± 0.07b |
T. vogelii (5%) | 0.08 ± 0.05a | 0.08 ± 0.05c | 0.18 ± 0.10b | 0.20 ± 0.08b | 0.13 ± 0.04b | 0.15 ± 0.03b |
T. vogelii (10%) | 0.05 ± 0.05a | 0.08 ± 0.04c | 0.15 ± 0.05b | 0.13 ± 0.08b | 0.05 ± 0.03b | 0.08 ± 0.04b |
2-way ANOVA | (F-Statistics) | |||||
Location | 0.42ns | 15.11*** | 19.55*** | 58.04*** | 38.06*** | 59.75*** |
Treatments | 2.04ns | 5.52*** | 20.22*** | 45.09*** | 28.14*** | 109.40*** |
Location*treatments | 1.95ns | 2.84*** | 3.00*** | 3.64*** | 5.63*** | 17.46*** |
Note: Each value is a mean ± standard error of eight replicates, *, **, and *** significant at P ≤ 0.05, P ≤ 0.01 and P ≤ 0.001 respectively and ns means not significant. Means within the same column followed by the same letter(s) are not significantly different at (P = 0.05) from each other using Fishers Least significant Difference (LSD) test. |
Location and treatments | Week 1 before Treatment | Weeks after treatments | ||||
1 | 2 | 3 | 4 | 5 | ||
Location | ||||||
Tengeru | 0.18 ± 0.02a | 0.18 ± 0.03a | 0.23 ± 0.0a | 0.22 ± 0.07a | 0.17 ± 0.05a | 0.20 ± 0.05a |
Boro | 0.09 ± 0.02b | 0.06 ± 0.03b | 0.06 ± 0.02b | 0.10 ± 0.02b | 0.15 ± 0.04a | 0.14 ± 0.04a |
Treatments | ||||||
Water (−ve control) | 0.18 ± 0.07a | 0.50 ± 0.09a | 0.63 ± 0.30a | 0.60 ± 0.24a | 0.48 ± 0.15a | 0.78 ± 0.16a |
water + soap (−ve control) | 0.10 ± 0.05a | 0.30 ± 0.08b | 0.48 ± 0.20a | 0.68 ± 0.26a | 0.65 ± 0.20a | 0.80 ± 0.18a |
Synthetic pesticide (+ve control) | 0.13 ± 0.05a | 0.05 ± 0.03c | 0.03 ± 0.03b | 0.03 ± 0.03b | 0.03 ± 0.03bc | 0.00 ± 0.00b |
C. dichogamus (1%) | 0.15 ± 0.05a | 0.08 ± 0.04c | 0.05 ± 0.03b | 0.13 ± 0.05b | 0.23 ± 0.10b | 0.08 ± 0.04b |
C. dichogamus (5%) | 0.08 ± 0.04a | 0.10 ± 0.04c | 0.10 ± 0.05b | 0.05 ± 0.03b | 0.13 ± 0.04bc | 0.08 ± 0.04b |
C. dichogamus (10%) | 0.15 ± 0.05a | 0.03 ± 0.03c | 0.08 ± 0.04b | 0.05 ± 0.03b | 0.08 ± 0.05bc | 0.08 ± 0.04b |
S. aromaticum (1%) | 0.10 ± 0.04a | 0.13 ± 0.05c | 0.13 ± 0.08b | 0.05 ± 0.03b | 0.08 ± 0.04bc | 0.08 ± 0.04b |
S. aromaticum (5%) | 0.18 ± 0.03a | 0.05 ± 0.03c | 0.05 ± 0.03b | 0.05 ± 0.03b | 0.00 ± 0.00c | 0.03 ± 0.03b |
S. aromaticum (10%) | 0.10 ± 0.01a | 0.05 ± 0.03c | 0.03 ± 0.03b | 0.00 ± 0.00b | 0.05 ± 0.05bc | 0.03 ± 0.03b |
T. vogelii (1%) | 0.13 ± 0.05a | 0.10 ± 0.04c | 0.10 ± 0.05b | 0.18 ± 0.06b | 0.15 ± 0.03bc | 0.10 ± 0.04b |
T. vogelii (5%) | 0.20 ± 0.04a | 0.05 ± 0.03c | 0.05 ± 0.03b | 0.08 ± 0.04b | 0.03 ± 0.03bc | 0.00 ± 0.00b |
T. vogelii (10%) | 0.13 ± 0.04a | 0.00 ± 0.00c | 0.03 ± 0.03b | 0.05 ± 0.03b | 0.03 ± 0.03bc | 0.00 ± 0.00b |
2-way ANOVA | (F-Statistics) | |||||
Location | 12.10*** | 20.44*** | 8.80** | 4.66* | 0.24ns | 2.21ns |
Treatments | 0.67ns | 10.90*** | 3.83*** | 5.53*** | 5.42*** | 16.01*** |
Location*treatments | 0.26ns | 1.05ns | 1.96* | 2.46** | 0.37ns | 1.16ns |
Note: Each value is a mean ± standard error of eight replicates, *, **, and *** significant at P ≤ 0.05, P ≤ 0.01 and P ≤ 0.001 respectively and ns means not significant. Means within the same column followed by the same letter(s) are not significantly different at (P = 0.05) from each other using Fishers Least significant Difference (LSD) test. |
Location and treatments | Week 1 before Treatment | Weeks after treatments | ||||
1 | 2 | 3 | 4 | 5 | ||
Location | ||||||
Tengeru | 19.7 ± 1.1a | 19.1 ± 1.8a | 18.0 ± 2.0a | 16.3 ± 2.1a | 15.3 ± 1.9a | 19.9 ± 2.8a |
Boro | 10.1 ± 0.5b | 10.3 ± 1.0b | 11.8 ± 1.1b | 15.5 ± 1.4a | 18.3 ± 2.0a | 21.8 ± 3.2a |
Treatments | ||||||
Water (−ve control) | 18.8 ± 3.1a | 33.8 ± 5.6a | 33.1 ± 4.5a | 38.8 ± 2.3a | 45.0 ± 4.3a | 70.6 ± 3.1a |
water + soap (−ve control) | 15.6 ± 4.4a | 30.0 ± 5.7a | 31.9 ± 3.7a | 41.3 ± 3.1a | 41.3 ± 3.0a | 56.3 ± 2.3b |
Synthetic pesticide (+ve control) | 16.3 ± 2.6a | 8.1 ± 1.6c | 6.3 ± 1.6e | 6.9 ± 1.9e | 7.5 ± 1.6d | 5.0 ± 1.3g |
C. dichogamus (1%) | 16.9 ± 2.8a | 16.3 ± 3.2b | 17.5 ± 3.0b | 17.5 ± 1.9b | 18.1 ± 1.9b | 18.8 ± 1.8c |
C. dichogamus (5%) | 15.0 ± 2.7a | 10.0 ± 1.3bc | 13.8 ± 3.5bcde | 15.0 ± 2.7bc | 15.0 ± 2.1bc | 18.8 ± 1.8c |
C. dichogamus (10%) | 13.8 ± 1.8a | 11.3 ± 1.6bc | 11.3 ± 1.6bcde | 11.3 ± 0.8cde | 11.3 ± 2.1cd | 12.5 ± 2.8de |
S. aromaticum (1%) | 14.4 ± 2.4a | 13.8 ± 2.5bc | 15.6 ± 2.4bcd | 15.0 ± 1.3bc | 17.5 ± 1.9b | 18.1 ± 1.6c |
S. aromaticum (5%) | 12.5 ± 1.3a | 10.6 ± 1.1bc | 10.0 ± 0.0bcde | 8.1 ± 1.3e | 8.8 ± 1.3d | 10.6 ± 1.5ef |
S. aromaticum (10%) | 14.4 ± 2.2a | 8.8 ± 1.8bc | 8.1 ± 0.9de | 6.9 ± 1.3e | 8.1 ± 1.6d | 9.4 ± 1.5efg |
T. vogelii (1%) | 11.9 ± 3.3a | 12.5 ± 1.9bc | 16.3 ± 4.6bc | 13.8 ± 2.3bcd | 15.0 ± 0.9bc | 17.5 ± 1.3cd |
T. vogelii (5%) | 16.3 ± 3.0a | 11.3 ± 1.6bc | 8.8 ± 1.8cde | 8.8 ± 0.8de | 6.9 ± 1.6d | 6.9 ± 1.6fg |
T. vogelii (10%) | 11.9 ± 1.6a | 10.0 ± 1.9bc | 6.3 ± 1.6e | 7.5 ± 1.3e | 7.5 ± 1.3d | 5.6 ± 1.1fg |
2-way ANOVA | ||||||
Location | 77.06*** | 45.03*** | 18.56*** | 0.51ns | 6.05** | 2.89ns |
Treatments | 1.19ns | 13.70*** | 12.98*** | 44.96*** | 37.05*** | 117.55*** |
Location*treatments | 1.53ns | 1.94ns | 1.28ns | 1.90ns | 0.57ns | 0.77ns |
Note: Each value is a mean ± standard error of eight replicates, *, **, and *** significant at P ≤ 0.05, P ≤ 0.01 and P ≤ 0.001 respectively and ns means not significant. Means within the same column followed by the same letter(s) are not significantly different at (P = 0.05) from each other using Fishers Least significant Difference (LSD) test. |
P. xylostella | T. ni | Damage (%) | |
P. xylostella | 1.000 | ||
T. ni | 0.993 (<0.001) | 1.000 | |
Damage (%) | 0.976 (<0.001) | 0.99 (<0.001) | 1.000 |
Keys: In brackets are the P—values of significant correlations of measured variables. |
Location and treatments | Week 1 before Treatment | Weeks after treatments | ||||
1 | 2 | 3 | 4 | 5 | ||
Location | ||||||
Tenger | 0.08 ± 0.02a | 0.09 ± 0.03b | 0.23 ± 0.08b | 0.30 ± 0.10b | 0.23 ± 0.08b | 0.44 ± 0.11b |
Boro | 0.07 ± 0.02a | 0.41 ± 0.11a | 0.56 ± 0.12a | 0.87 ± 0.16a | 0.71 ± 0.15a | 0.96 ± 0.24a |
Treatments | ||||||
Water (−ve control) | 0.13 ± 0.05a | 1.13 ± 0.53a | 1.68 ± 0.41a | 2.28 ± 0.44a | 2.20 ± 0.53a | 3.28 ± 0.60a |
Water + soap (−ve control) | 0.10 ± 0.04a | 0.75 ± 0.15ab | 1.60 ± 0.31a | 2.58 ± 0.36a | 1.83 ± 0.35a | 3.30 ± 0.49a |
Synthetic pesticide (+ve control) | 0.00 ± 0.00a | 0.05 ± 0.10c | 0.03 ± 0.03b | 0.28 ± 0.15b | 0.08 ± 0.04b | 0.08 ± 0.04b |
C. dichogamus (1%) | 0.13 ± 0.05a | 0.28 ± 0.12bc | 0.25 ± 0.09b | 0.30 ± 0.10b | 0.23 ± 0.10b | 0.33 ± 0.08b |
C. dichogamus (5%) | 0.08 ± 0.04a | 0.15 ± 0.10c | 0.33 ± 0.06b | 0.20 ± 0.08b | 0.23 ± 0.10b | 0.20 ± 0.07b |
C. dichogamus (10%) | 0.03 ± 0.03a | 0.08 ± 0.04c | 0.20 ± 0.08b | 0.15 ± 0.05b | 0.13 ± 0.08b | 0.18 ± 0.06b |
S. aromaticum (1%) | 0.18 ± 0.05a | 0.13 ± 0.05c | 0.18 ± 0.10b | 0.35 ± 0.12b | 0.23 ± 0.09b | 0.28 ± 0.04b |
S. aromaticum (5%) | 0.03 ± 0.03a | 0.15 ± 0.07c | 0.10 ± 0.05b | 0.23 ± 0.09b | 0.23 ± 0.08b | 0.18 ± 0.06b |
S. aromaticum (10%) | 0.03 ± 0.03a | 0.03 ± 0.03c | 0.05 ± 0.05b | 0.15 ± 0.08b | 0.18 ± 0.06b | 0.13 ± 0.04b |
T. vogelii (1%) | 0.00 ± 0.00a | 0.10 ± 0.05c | 0.15 ± 0.05b | 0.18 ± 0.07b | 0.20 ± 0.07b | 0.25 ± 0.07b |
T. vogelii (5%) | 0.08 ± 0.05a | 0.08 ± 0.05c | 0.18 ± 0.10b | 0.20 ± 0.08b | 0.13 ± 0.04b | 0.15 ± 0.03b |
T. vogelii (10%) | 0.05 ± 0.05a | 0.08 ± 0.04c | 0.15 ± 0.05b | 0.13 ± 0.08b | 0.05 ± 0.03b | 0.08 ± 0.04b |
2-way ANOVA | (F-Statistics) | |||||
Location | 0.42ns | 15.11*** | 19.55*** | 58.04*** | 38.06*** | 59.75*** |
Treatments | 2.04ns | 5.52*** | 20.22*** | 45.09*** | 28.14*** | 109.40*** |
Location*treatments | 1.95ns | 2.84*** | 3.00*** | 3.64*** | 5.63*** | 17.46*** |
Note: Each value is a mean ± standard error of eight replicates, *, **, and *** significant at P ≤ 0.05, P ≤ 0.01 and P ≤ 0.001 respectively and ns means not significant. Means within the same column followed by the same letter(s) are not significantly different at (P = 0.05) from each other using Fishers Least significant Difference (LSD) test. |
Location and treatments | Week 1 before Treatment | Weeks after treatments | ||||
1 | 2 | 3 | 4 | 5 | ||
Location | ||||||
Tengeru | 0.18 ± 0.02a | 0.18 ± 0.03a | 0.23 ± 0.0a | 0.22 ± 0.07a | 0.17 ± 0.05a | 0.20 ± 0.05a |
Boro | 0.09 ± 0.02b | 0.06 ± 0.03b | 0.06 ± 0.02b | 0.10 ± 0.02b | 0.15 ± 0.04a | 0.14 ± 0.04a |
Treatments | ||||||
Water (−ve control) | 0.18 ± 0.07a | 0.50 ± 0.09a | 0.63 ± 0.30a | 0.60 ± 0.24a | 0.48 ± 0.15a | 0.78 ± 0.16a |
water + soap (−ve control) | 0.10 ± 0.05a | 0.30 ± 0.08b | 0.48 ± 0.20a | 0.68 ± 0.26a | 0.65 ± 0.20a | 0.80 ± 0.18a |
Synthetic pesticide (+ve control) | 0.13 ± 0.05a | 0.05 ± 0.03c | 0.03 ± 0.03b | 0.03 ± 0.03b | 0.03 ± 0.03bc | 0.00 ± 0.00b |
C. dichogamus (1%) | 0.15 ± 0.05a | 0.08 ± 0.04c | 0.05 ± 0.03b | 0.13 ± 0.05b | 0.23 ± 0.10b | 0.08 ± 0.04b |
C. dichogamus (5%) | 0.08 ± 0.04a | 0.10 ± 0.04c | 0.10 ± 0.05b | 0.05 ± 0.03b | 0.13 ± 0.04bc | 0.08 ± 0.04b |
C. dichogamus (10%) | 0.15 ± 0.05a | 0.03 ± 0.03c | 0.08 ± 0.04b | 0.05 ± 0.03b | 0.08 ± 0.05bc | 0.08 ± 0.04b |
S. aromaticum (1%) | 0.10 ± 0.04a | 0.13 ± 0.05c | 0.13 ± 0.08b | 0.05 ± 0.03b | 0.08 ± 0.04bc | 0.08 ± 0.04b |
S. aromaticum (5%) | 0.18 ± 0.03a | 0.05 ± 0.03c | 0.05 ± 0.03b | 0.05 ± 0.03b | 0.00 ± 0.00c | 0.03 ± 0.03b |
S. aromaticum (10%) | 0.10 ± 0.01a | 0.05 ± 0.03c | 0.03 ± 0.03b | 0.00 ± 0.00b | 0.05 ± 0.05bc | 0.03 ± 0.03b |
T. vogelii (1%) | 0.13 ± 0.05a | 0.10 ± 0.04c | 0.10 ± 0.05b | 0.18 ± 0.06b | 0.15 ± 0.03bc | 0.10 ± 0.04b |
T. vogelii (5%) | 0.20 ± 0.04a | 0.05 ± 0.03c | 0.05 ± 0.03b | 0.08 ± 0.04b | 0.03 ± 0.03bc | 0.00 ± 0.00b |
T. vogelii (10%) | 0.13 ± 0.04a | 0.00 ± 0.00c | 0.03 ± 0.03b | 0.05 ± 0.03b | 0.03 ± 0.03bc | 0.00 ± 0.00b |
2-way ANOVA | (F-Statistics) | |||||
Location | 12.10*** | 20.44*** | 8.80** | 4.66* | 0.24ns | 2.21ns |
Treatments | 0.67ns | 10.90*** | 3.83*** | 5.53*** | 5.42*** | 16.01*** |
Location*treatments | 0.26ns | 1.05ns | 1.96* | 2.46** | 0.37ns | 1.16ns |
Note: Each value is a mean ± standard error of eight replicates, *, **, and *** significant at P ≤ 0.05, P ≤ 0.01 and P ≤ 0.001 respectively and ns means not significant. Means within the same column followed by the same letter(s) are not significantly different at (P = 0.05) from each other using Fishers Least significant Difference (LSD) test. |
Location and treatments | Week 1 before Treatment | Weeks after treatments | ||||
1 | 2 | 3 | 4 | 5 | ||
Location | ||||||
Tengeru | 19.7 ± 1.1a | 19.1 ± 1.8a | 18.0 ± 2.0a | 16.3 ± 2.1a | 15.3 ± 1.9a | 19.9 ± 2.8a |
Boro | 10.1 ± 0.5b | 10.3 ± 1.0b | 11.8 ± 1.1b | 15.5 ± 1.4a | 18.3 ± 2.0a | 21.8 ± 3.2a |
Treatments | ||||||
Water (−ve control) | 18.8 ± 3.1a | 33.8 ± 5.6a | 33.1 ± 4.5a | 38.8 ± 2.3a | 45.0 ± 4.3a | 70.6 ± 3.1a |
water + soap (−ve control) | 15.6 ± 4.4a | 30.0 ± 5.7a | 31.9 ± 3.7a | 41.3 ± 3.1a | 41.3 ± 3.0a | 56.3 ± 2.3b |
Synthetic pesticide (+ve control) | 16.3 ± 2.6a | 8.1 ± 1.6c | 6.3 ± 1.6e | 6.9 ± 1.9e | 7.5 ± 1.6d | 5.0 ± 1.3g |
C. dichogamus (1%) | 16.9 ± 2.8a | 16.3 ± 3.2b | 17.5 ± 3.0b | 17.5 ± 1.9b | 18.1 ± 1.9b | 18.8 ± 1.8c |
C. dichogamus (5%) | 15.0 ± 2.7a | 10.0 ± 1.3bc | 13.8 ± 3.5bcde | 15.0 ± 2.7bc | 15.0 ± 2.1bc | 18.8 ± 1.8c |
C. dichogamus (10%) | 13.8 ± 1.8a | 11.3 ± 1.6bc | 11.3 ± 1.6bcde | 11.3 ± 0.8cde | 11.3 ± 2.1cd | 12.5 ± 2.8de |
S. aromaticum (1%) | 14.4 ± 2.4a | 13.8 ± 2.5bc | 15.6 ± 2.4bcd | 15.0 ± 1.3bc | 17.5 ± 1.9b | 18.1 ± 1.6c |
S. aromaticum (5%) | 12.5 ± 1.3a | 10.6 ± 1.1bc | 10.0 ± 0.0bcde | 8.1 ± 1.3e | 8.8 ± 1.3d | 10.6 ± 1.5ef |
S. aromaticum (10%) | 14.4 ± 2.2a | 8.8 ± 1.8bc | 8.1 ± 0.9de | 6.9 ± 1.3e | 8.1 ± 1.6d | 9.4 ± 1.5efg |
T. vogelii (1%) | 11.9 ± 3.3a | 12.5 ± 1.9bc | 16.3 ± 4.6bc | 13.8 ± 2.3bcd | 15.0 ± 0.9bc | 17.5 ± 1.3cd |
T. vogelii (5%) | 16.3 ± 3.0a | 11.3 ± 1.6bc | 8.8 ± 1.8cde | 8.8 ± 0.8de | 6.9 ± 1.6d | 6.9 ± 1.6fg |
T. vogelii (10%) | 11.9 ± 1.6a | 10.0 ± 1.9bc | 6.3 ± 1.6e | 7.5 ± 1.3e | 7.5 ± 1.3d | 5.6 ± 1.1fg |
2-way ANOVA | ||||||
Location | 77.06*** | 45.03*** | 18.56*** | 0.51ns | 6.05** | 2.89ns |
Treatments | 1.19ns | 13.70*** | 12.98*** | 44.96*** | 37.05*** | 117.55*** |
Location*treatments | 1.53ns | 1.94ns | 1.28ns | 1.90ns | 0.57ns | 0.77ns |
Note: Each value is a mean ± standard error of eight replicates, *, **, and *** significant at P ≤ 0.05, P ≤ 0.01 and P ≤ 0.001 respectively and ns means not significant. Means within the same column followed by the same letter(s) are not significantly different at (P = 0.05) from each other using Fishers Least significant Difference (LSD) test. |
P. xylostella | T. ni | Damage (%) | |
P. xylostella | 1.000 | ||
T. ni | 0.993 (<0.001) | 1.000 | |
Damage (%) | 0.976 (<0.001) | 0.99 (<0.001) | 1.000 |
Keys: In brackets are the P—values of significant correlations of measured variables. |