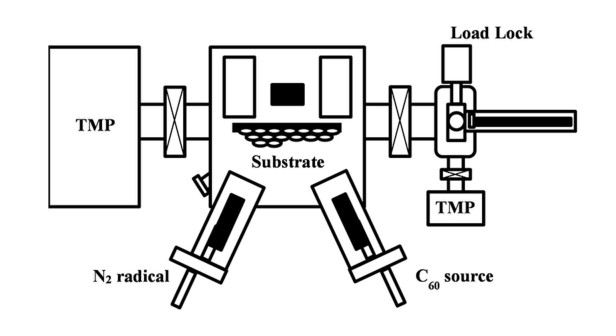
All-carbon photovoltaic devices have attracted attention in terms of resources and environment. However, the device application is very limited because of poor performance. In this work, we studied the solar cell characteristics of amorphous carbon (a–C)/fullerene (C60) junction when the thickness of the a–C layer was varied. When the thickness of the a–C layer was varied, the short-circuit current density and open-circuit voltage increased with increasing film thickness and then decreased after a certain value. Also, the spectral response measurement results suggest that most of the power generation is due to the light absorbed by the C60 layer, and that the light absorbed by the a–C layer may contribute little to power generation. This study suggests that the improvement in the electronic properties of a–C is necessary to make a photovoltaic device with high performance.
Citation: Takuto Eguchi, Shinya Kato, Naoki Kishi, Tetsuo Soga. Effect of thickness on photovoltaic properties of amorphous carbon/fullerene junction[J]. AIMS Materials Science, 2022, 9(3): 446-454. doi: 10.3934/matersci.2022026
[1] | Hmoud. Al-Dmour . Capacitance response of solar cells based on amorphous Titanium dioxide (A-TiO2) semiconducting heterojunctions. AIMS Materials Science, 2021, 8(2): 261-270. doi: 10.3934/matersci.2021017 |
[2] | Emily M. Barker, Ashli R. Toles, Kyle A. Guess, Janice Paige Buchanan . C60 and Sc3N@C80(TMB-PPO) derivatives as constituents of singlet oxygen generating, thiol-ene polymer nanocomposites. AIMS Materials Science, 2016, 3(3): 965-988. doi: 10.3934/matersci.2016.3.965 |
[3] | Wei-Chi Chen, Pin-Yao Chen, Sheng-Hsiung Yang . Solution-processed hybrid light emitting and photovoltaic devices comprising zinc oxide nanorod arrays and tungsten trioxide layers. AIMS Materials Science, 2017, 4(3): 551-560. doi: 10.3934/matersci.2017.3.551 |
[4] | M. F. Achoi, S. Kato, N. Kishi, T. Soga . Improved photovoltaic properties of ((CH3NH3)1-xCsx)3Bi2I9: (x = 0-1.0) hybrid perovskite solar cells via a hot immersion method. AIMS Materials Science, 2024, 11(4): 605-619. doi: 10.3934/matersci.2024031 |
[5] | Keith E. Whitener Jr . Rapid synthesis of thin amorphous carbon films by sugar dehydration and dispersion. AIMS Materials Science, 2016, 3(4): 1309-1320. doi: 10.3934/matersci.2016.4.1309 |
[6] | Wisatre Kongcharoensuntorn, Pornpen Atorngitjawat . Influence of chlorotrifluoroethylene on crystal structure and polymer dynamics of poly(vinylidenefluoride-co-chlorotrifluoroethylene) antibacterial copolymers. AIMS Materials Science, 2023, 10(1): 164-181. doi: 10.3934/matersci.2023009 |
[7] | Aruna P. Wanninayake, Benjamin C. Church, Nidal Abu-Zahra . Effect of ZnO nanoparticles on the power conversion efficiency of organic photovoltaic devices synthesized with CuO nanoparticles. AIMS Materials Science, 2016, 3(3): 927-937. doi: 10.3934/matersci.2016.3.927 |
[8] | Gennaro Gelao, Roberto Marani, Anna Gina Perri . Analysis and design of current mode logic based on CNTFET. AIMS Materials Science, 2023, 10(6): 965-980. doi: 10.3934/matersci.2023052 |
[9] | Navin Kafle, Alper Buldum . The interaction between fullerene-porphyrin dyad and graphene. AIMS Materials Science, 2017, 4(2): 505-514. doi: 10.3934/matersci.2017.2.505 |
[10] | Aruna P. Wanninayake, Shengyi Li, Benjamin C. Church, Nidal Abu-Zahra . Electrical and optical properties of hybrid polymer solar cells incorporating Au and CuO nanoparticles. AIMS Materials Science, 2016, 3(1): 35-50. doi: 10.3934/matersci.2016.1.35 |
All-carbon photovoltaic devices have attracted attention in terms of resources and environment. However, the device application is very limited because of poor performance. In this work, we studied the solar cell characteristics of amorphous carbon (a–C)/fullerene (C60) junction when the thickness of the a–C layer was varied. When the thickness of the a–C layer was varied, the short-circuit current density and open-circuit voltage increased with increasing film thickness and then decreased after a certain value. Also, the spectral response measurement results suggest that most of the power generation is due to the light absorbed by the C60 layer, and that the light absorbed by the a–C layer may contribute little to power generation. This study suggests that the improvement in the electronic properties of a–C is necessary to make a photovoltaic device with high performance.
Recently, global warming caused by greenhouse gases has become a worldwide problem. Solar cells have been attracting attention as a clean way to generate electricity to solve this problem. Currently, the mainstream solar cells use silicon, but the conversion efficiency has almost reached the theoretical value, and the high cost of high-purity silicon to produce high-efficiency silicon solar cells is a problem [1]. Therefore, next generation solar cells are being investigated, including compound solar cells such as GaAs [2], CdTe [3], organic thin-film [4], and perovskite materials [5].
As a next-generation solar cell, carbon-based materials are attractive in terms of resources and environment. Carbon is abundant element and can be synthesized from natural source. Moreover, it is possible to change the bandgap on demand by choosing the carbon allotrope, such as fullerene [6], nanotube [7] and graphene [8]. Furthermore, the band gap of amorphous carbon (a–C) can be changed from 0.5 eV to 3 eV with changing the sp2/sp3 ratio [9,10,11]. It means that amorphous carbon may convert the photons from UV-region to infrared region into electricity, resulting in the very high efficiency solar cell. In this context, we focus our attention on carbon materials for the next-generation solar cells using fullerene (C60) and a–C. C60 is known to be an excellent electron acceptor (n-type semiconductor) because it can stably accept six electrons due to the triple degeneracy of its lowest empty molecular orbital (LUMO) [12]. On the contrary, a–C is usually p-type semiconductor, and the optical gap of a–C can be changed by varying the deposition conditions [13,14].
Many papers are reported on C/Si junction solar cell until now [15,16,17,18,19]. But the merit of carbon is few if Si is used as a substrate. As all-carbon solar cell, several structures have been reported until now, such as C60/ITO [20], C60 homojunction [21], C60/single wall carbon nanotube (SWCNT)/graphene oxide (GO) [22], C60/diamond [23], etc. Chen et al reported the C60/ITO cell with short circuit current density (Jsc), open circuit voltage (Voc) and power conversion efficiency (PCE) of 1.98 mA/cm2, 0.52 V and 0.414%, respectively [20]. Kubo et al reported C60 homojunction solar cell with Jsc of 0.11 mA/cm2 [21]. Tung et al reported solution processed all-carbon solar cells from C60/SWCNT/GO with Jsc, Voc and PCE of 1.23 mA/cm2, 0.59 V and 0.21%, respectively [22]. Besides, C60/diamond cell with Jsc, Voc and PCE of 5.3 μA/cm2, 0.023 V and 4.3 × 10-5%, respectively, has been reported [23]. The authors reported a–C/C60 all-carbon photovoltaic devices with Jsc, Voc and PCE of 0.15 mA/cm2, 0.25 V and 0.01%, respectively, in 2013 by using the nature that a–C is p-type and C60 is n-type [24]. The a–C film was prepared by irradiating C60 in the process of sublimation with N2 radicals to break the bonds. Very recently, our group reported that a double junction carbon thin film cell using C60 and a–C (Al/C60/a–C/C60/a–C/ITO/glass) has twice the open circuit voltage compared to the single junction type, indicating tandem-like properties in this structure [25]. The thickness of each layer may affect the photovoltaic properties in two-junction carbon thin-film cells. Moreover, it was reported that nitrogen doping to a–C improved the short circuit current of a–C/C60 junction cell from 0.17 to 0.30 mA/cm2 [26]. However, so far, carbon thin-film solar cells have shown very low power conversion efficiency. We aim to fabricate carbon thin-film photovoltaic cells that show better power conversion efficiency by improving the device structure. In order to improve the performance, the thickness of each layer should be optimized. Although C60 film is commonly used as solar cell absorber, a–C is merely used because resistivity of a–C is high [9,26]. Therefore, it is expected that thickness of a–C layer greatly affects the properties of C60/a–C cell. In this study, the effect of the thickness of the a–C layer on the device characteristics was evaluated for single junction carbon thin film photovoltaic cells in order to improve the conversion efficiency of multi-junction carbon thin film solar cells.
In this study, p-type a–C and n-type C60 were deposited on ITO glass substrates or glass substrates using an ultra-high vacuum chamber (UHVC). The base pressure of the UHVC is 10-8 Torr. A schematic diagram of the deposition system is shown in Figure 1. The deposition system consists of a turbo molecular pump (TMP), a load-lock chamber, a Knudsen cell, a high frequency power supply for generating N2 radicals and a quartz discharge tube, and others. C60 was deposited by heating and sublimating 99% pure C60 in a Knudsen cell. Also, the a–C was deposited by irradiating C60 with N2 radicals while it was in the process of sublimation in UHVC.
The sublimation temperature and pressure during C60 deposition are 500 ℃ and 3.0–6.0 × 10-8 Torr, respectively. And the sublimation temperature during a–C deposition is also 500 ℃, pressure is 8.0–9.0 × 10-5 Torr, RF power is 300 W, RF frequency is 13.56 MHz, and N2 flow rate is 1.5 sccm.
In this study, photovoltaic cells were fabricated by depositing a–C and C60 on ITO glass substrates, followed by the deposition of Al electrodes using a separate deposition system. In order to reduce the influence of the substrate as much as possible when measuring the absorptivity, we also fabricated samples with a–C and C60 deposited on a glass substrate. The schematic diagram of the fabricated photovoltaic cell is shown in Figure 2. The thickness of each layer was fixed at 50 nm for the C60 layer, and the thickness of the a–C layer was varied from 25–125 nm.
The samples were evaluated by measuring the current density-voltage (J-V) characteristics under AM 1.5 standard light using a solar simulator, and the photovoltaic parameters such as Jsc, Voc, fill factor (FF), and PCE were calculated from the J-V curves. The spectral response was measured with Xenon lamp (model PS-X500) and single-phase lock-in amplifier (model 5600A). The cell area is 0.09 cm2. The absorption coefficient was calculated from the results of reflectance and transmittance measurements by Ultraviolet Visible (UV-Vis) Absorption Spectroscopy (JASCO 670UV) on samples of a–C and C60 deposited on glass substrates.
The optical absorption of a–C/C60 single junction carbon thin film cells was evaluated by UV-Vis absorption spectroscopy to confirm the change in absorption spectrum when the thickness of the a–C layer was changed. In addition, samples of a–C and C60 monolayers formed on glass substrates with a thickness of 50 nm each and glass substrates alone were also evaluated. The reflectance and transmittance of each sample were measured by UV-Vis absorption spectroscopy, and the absorption rate was calculated from the results. The calculation of the absorption is based on the assumption that all the light incident on the sample is reflected, transmitted, or absorbed. The calculation results include the effect of the glass substrate.
The measurement results are shown in Figure 3. First, from the measurement results of C60 and a–C monolayers, it was confirmed that C60 has peaks at around 380 nm and 450 nm and absorbs light of wavelengths below 550 nm, which is a typical absorption spectrum of C60 film [27]. On the other hand, a–C has a broad absorption spectrum from the ultraviolet to around 800 nm as reported previously. From the Tauc plot the optical bandgap of a–C films is around 1.2 eV [20]. And the absorption spectrum for the sample of solar cell structure was confirmed to have the characteristics of both C60 and a–C monolayer. It was also confirmed that the absorption rate increased as the thickness of the a–C layer increased.
The spectral response characteristics of a–C/C60 single junction carbon thin film solar cells were evaluated when the thickness of the a–C layer was varied. Figure 4a, b show the measurement results of the spectral response characteristics for different current range.
From this figure, it was found that the fabricated photovoltaic cells generated electricity at wavelengths below 550 nm and the broad hump is observed at around 500–650 nm as shown in Figure 4b. This result and the measurement of the absorption suggest that most of the power generation in a–C/C60 single junction carbon thin film cells are due to the light absorbed in the C60 layer, and that the light absorbed in the a–C layer may have little role in the power generation. Also, as the film thickness of the a–C layer increases, the sensitivity decreases at wavelengths below 400 nm, and the peak around 400–500 nm moves to the longer wavelength side. This is thought to be due to the fact that light is absorbed by the a–C layer, since light passes through the a–C layer first due to the structure of the photovoltaic cell.
Our results suggest that the photons created in C60 contribute to current, whereas the contribution of a–C is very small. It is due to that the diffusion length of a–C is much shorter than that of C60. Based on these results, improving the minority carrier properties such as lifetime and mobility of the a–C layer is particularly important to increase the power conversion efficiency in a–C/C60 carbon thin film solar cells with highly-nitrogen doping [26], a-carbon nitride film [28], C3N4 [29], etc.
Photovoltaic properties of a–C/C60 single junction carbon thin film photovoltaic cells were evaluated when the thickness of the a–C layer was varied. The J-V characteristics of each sample under AM 1.5 are shown in Figure 5, and the variation of Jsc, Voc, FF, and PCE with the change of a–C layer thickness is shown in Figure 6. The measured photoelectric conversion parameters are shown in Table 1.
a–C layer thickness (nm) | Jsc (mA/cm2) | Voc (V) | FF | PCE (%) |
25 | 0.145 | 0.236 | 0.296 | 0.0102 |
75 | 0.168 | 0.416 | 0.322 | 0.0225 |
100 | 0.188 | 0.314 | 0.324 | 0.0215 |
125 | 0.164 | 0.307 | 0.366 | 0.0184 |
From Figures 5 and 6, it was found that there was a change in the photoelectric conversion parameters as the thickness of the a–C layer changed. The Jsc increased as the thickness of the a–C layer increased, reaching a maximum at 100 nm, and tended to decrease thereafter. The reason why the Jsc became smaller when the a–C layer was thin is thought to be that the a–C layer was not uniform, and current flowing in the opposite direction occurred in areas with a thin film thickness, which results in the decrease of spectral response at wavelength region of 400 nm to 500 nm as shown in Figure 4. Jsc decreases when the thickness of the a–C layer became thicker than 100 nm because of the increase in the amount of light absorbed by the a–C layer and the decrease in the amount of light reaching the C60 layer as the a–C layer became thicker, as well as the increase in series resistance due to the increase in film thickness. As in Jsc, Voc increased with increasing thickness of the a–C layer, reaching a maximum at 75 nm, and showed a decreasing trend thereafter. The reason why the Voc became small when the a–C layer thickness was thin is thought to be that the a–C layer was not a uniform film as in Jsc, and a current flowing in the reverse direction occurred via pin-hole or defects. The reason why Voc tended to decrease as the thickness of the a–C layer became thicker than 75 nm could be due to an increase of series resistance due to high defect density of a–C, which reduces Jsc. Fill factor is gradually increased with a–C thickness since the properties of a–C such as pin-hole or defect density is improved with thickness, resulting in the decrease of saturation current. From the variation of Jsc and Voc with the thickness of the a–C layer, PCE was maximum when the a–C layer was 75 nm.
In this experiment, the thickness of C60 was kept constant to 50 nm. When the C60 thickness is changed, the optimum thickness of a–C may be slightly changed. But these present results will be useful to understand the photovoltaic properties of a–C/C60 junction with different thickness.
In this study, the photoelectric and optical properties of carbon thin film photovoltaic cells using C60 and a–C were evaluated when the thickness of the a–C layer was varied. As the thickness of the a–C layer was increased, the photoelectric properties improved up to a certain value, but deteriorated as the thickness increased. When the a–C layer is thin, it is not a uniform film, which is thought to be the cause of poor photoelectric properties, and each layer needs to have a certain thickness. In addition, the possibility that the light absorbed by the a–C layer hardly contributes to power generation is mentioned. Therefore, we believe that it is important to improve the minority carrier properties of the a–C layer by reducing defects, in order to improve the photoelectric properties.
All authors declare no conflicts of interest in this paper.
[1] |
Lamnatou C, Chemisana D (2017) Photovoltaic/thermal (PVT) systems: A review with emphasis on environmental issues. Renew Energ 105: 270-287. https://doi.org/10.1016/j.renene.2016.12.009 doi: 10.1016/j.renene.2016.12.009
![]() |
[2] | Kayes BM, Nie H, Twist R, et al. (2011) 27.6% conversion efficiency, a new record for single‐junction solar cells under 1 sun illumination, 37th IEEE Photovoltaic Specialists Conference, Seattle: IEEE, 000004-000008. https://doi.org/10.1109/PVSC.2011.6185831 |
[3] |
Britt J, Ferekides C (1993) Thin-film CdS/CdTe solar cell with 15.8% efficiency. Appl Phys Lett 62: 2851-2852. https://doi.org/10.1063/1.109629 doi: 10.1063/1.109629
![]() |
[4] |
He Z, Xiao B, Liu F, et al. (2015) Single-junction polymer solar cells with high efficiency and photovoltage. Nat Photon 5: 174179. https://doi.org/10.1038/nphoton.2015.6 doi: 10.1038/nphoton.2015.6
![]() |
[5] |
Park NG, Grätzel M, Miyasaka T, et al. (2016) Towards stable and commercially available perovskite solar cells. Nat Energy 1: 16152. https://doi.org/10.1038/nenergy.2016.152 doi: 10.1038/nenergy.2016.152
![]() |
[6] |
Zhang F, Inganäs O, Zhou Y, et al. (2016) Development of polymer-fullerene solar cells. Natl Sci Rev 3: 222-239. https://doi.org/10.1093/nsr/nww020 doi: 10.1093/nsr/nww020
![]() |
[7] |
Tune DD, Flavel BS, Krupke R, et al. (2012) Carbon nanotube-silicon solar cells. Adv Energy Mater 2: 1043-1055. https://doi.org/10.1002/aenm.201200249 doi: 10.1002/aenm.201200249
![]() |
[8] |
Zhang Y, Tang TT, Girit C, et al. (2009) Direct observation of a widely tunable bandgap in bilayer graphene. Nature 459: 820-823. https://doi.org/10.1038/nature08105 doi: 10.1038/nature08105
![]() |
[9] |
Krishna KM, Ebisu H, Hagimoto K, et al. (2000) Low density of defect states in hydrogenated amorphous carbon thin films grown by plasma-enhanced chemical vapor deposition. Appl Phys Lett 78: 294-296. https://doi.org/10.1063/1.1335548 doi: 10.1063/1.1335548
![]() |
[10] |
Zkria A, Abdel-Wahab F, Katamune Y, et al. (2018) Optical and structural characterization of ultrananocrystalline diamond/hydrogenated amorphous carbon composite films deposited via coaxial arc plasma. Curr Appl Phys 19: 143-148. https://doi.org/10.1016/j.cap.2018.11.012 doi: 10.1016/j.cap.2018.11.012
![]() |
[11] |
Zarei Moghadam R, Rezagholipour Dizaji H, Ehsani MH (2019) Modification of optical and mechanical properties of nitrogen doped diamond-like carbon layers. J Mater Sci-mater El 30: 19770-19781. https://doi.org/10.1007/s10854-019-02343-4 doi: 10.1007/s10854-019-02343-4
![]() |
[12] |
Wojciechowski K, Leijtens T, Siprova S, et al. (2015) C60 as an efficient n-type compact layer in perovskite solar cells. J Phys Chem Lett 6: 2399-2405. https://doi.org/10.1021/acs.jpclett.5b00902 doi: 10.1021/acs.jpclett.5b00902
![]() |
[13] |
Chhowalla M, Robertson J, Chen CW (1997) Influence of ion energy and substrate temperature on the optical and electronic properties of tetrahedral amorphous carbon (ta-C) films. J Appl Phys 81: 139-145. https://doi.org/10.1063/1.364000 doi: 10.1063/1.364000
![]() |
[14] |
Alves MAR, Rossetto JF, Balachova O, et al. (2001) Some optical properties of amorphous hydrogenated carbon thin films prepared by rf plasma deposition using methane. Microelectronics J 32: 783-786. https://doi.org/10.1016/S0026-2692(01)00046-5 doi: 10.1016/S0026-2692(01)00046-5
![]() |
[15] |
Yu FA, Kaneko T, Yoshimura S, et al. (1996) The spectro‐photovoltaic characteristics of a carbonaceous film/n‐type silicon (C/n-Si) photovoltaic cell. Appl Phys Lett 69: 4078-4080. https://doi.org/10.1063/1.117825 doi: 10.1063/1.117825
![]() |
[16] |
Ma M, Xue Q, Chen H, et al. (2010) Photovoltaic characteristics of Pd doped amorphous carbon film/SiO2/Si. Appl Phys Lett 97: 061902. https://doi.org/10.1063/1.3478230 doi: 10.1063/1.3478230
![]() |
[17] |
Soga T, Kokubu T, Hayashi Y, et al. (2005) Effect of rf power on the photovoltaic properties of boron-doped amorphous carbon/n-type silicon junction fabricated by plasma enhanced chemical vapor deposition. Thin Solid Films 482: 86-89. https://doi.org/10.1016/j.tsf.2004.11.123 doi: 10.1016/j.tsf.2004.11.123
![]() |
[18] |
Ma ZQ, Liu BX (2001) Boron-doped diamond-like amorphous carbon as photovoltaic films in solar cell. Sol Energy Mater Sol Cells 69: 339-344. https://doi.org/10.1016/S0927-0248(00)00400-1 doi: 10.1016/S0927-0248(00)00400-1
![]() |
[19] |
Zhu H, Wei J, Wang K, et al. (2009) Applications of carbon materials in photovoltaic solar cells. Sol Energy Mater Sol Cells 93: 1461-1470. https://doi.org/10.1016/j.solmat.2009.04.006 doi: 10.1016/j.solmat.2009.04.006
![]() |
[20] |
Chen J, Song QL, Xiong ZH, et al. (2011) Environment-friendly energy from all-carbon solar cells based on fullerene-C60. Sol Energy Mater Sol Cells 95: 1138-1140. https://doi.org/10.1016/j.solmat.2010.12.037 doi: 10.1016/j.solmat.2010.12.037
![]() |
[21] |
Kubo M, Kaji T, Hiramoto M (2011) pn-homojunction formation in single fullerene films. AIP Adv 1: 032177. https://doi.org/10.1063/1.3647994 doi: 10.1063/1.3647994
![]() |
[22] |
Tung VC, Huang JH, Kim J, et al. (2012) Towards solution processed all-carbon solar cells: a perspective. Energy Environ Sci 5: 7810-7818. https://doi.org/10.1039/C2EE21587J doi: 10.1039/C2EE21587J
![]() |
[23] |
Nagata A, Oku T, Suzuki A, et al. (2010) Fabrication and photovoltaic property of diamond: fullerene nanocomposite thin films. J Ceram Soc Jpn 118: 1006-1008. https://doi.org/10.2109/jcersj2.118.1006 doi: 10.2109/jcersj2.118.1006
![]() |
[24] |
Soga T, Kondoh T, Kishi N, et al. (2013) Photovoltaic properties of an amorphous carbon/fullerene junction. Carbon 60: 1-4. https://doi.org/10.1016/j.carbon.2013.02.050 doi: 10.1016/j.carbon.2013.02.050
![]() |
[25] |
Kato H, Yamamoto Y, Kato S, et al. (2018) Fabrication and evaluation of two-junction carbon thin film photovoltaic devices. J Solar Eneg Res Updat 5: 1-7. http://dx.doi.org/10.15377/2410-2199.2018.05.1 doi: 10.15377/2410-2199.2018.05.1
![]() |
[26] |
Soga T, Kishi N (2019) Improved photovoltaic properties of amorphous carbon/fullerene junction by nitrogen doping. J Mater Sci-mater El 30: 6628-6632. https://doi.org/10.1007/s10854-019-00970-5 doi: 10.1007/s10854-019-00970-5
![]() |
[27] |
Bogdanov AA (2018) Absorption spectra of C60 fullerene monomolecular films. Tech Phy 63: 1006-1009. https://doi.org/10.1134/S1063784218070083 doi: 10.1134/S1063784218070083
![]() |
[28] |
Katsuno T, Nitta S, Habuchi H, et al. (2004) Highly photoconductive amorphous carbon nitride films prepared by cyclic nitrogen radical sputtering. Appl Phys Lett 85: 2803-2805. https://doi.org/10.1063/1.1792384 doi: 10.1063/1.1792384
![]() |
[29] |
Yang X, Zhao L, Wang S, et al. (2021) Recent progress of g-C3N4 applied in solar cells. J Materiomics 7: 728-741. https://doi.org/10.1016/j.jmat.2021.01.004 doi: 10.1016/j.jmat.2021.01.004
![]() |
1. | Kulandai Velu Ramanathan, Vishnu Vardhana Chary, Shantikumar V. Nair, Dhamodaran Santhanagopalan, 2024, Chapter 8, 978-981-99-9934-7, 139, 10.1007/978-981-99-9935-4_8 | |
2. | Budhi Priyanto, Imam Khambali, Irma Septi Ardiani, Khoirotun Nadhiyah, Anna Zakiyatul Laila, M. Chasrun Hasani, Bima Romadhon, Retno Asih, Yoyok Cahyono, Nitrogen-Doped Amorphous Carbon Homojunction from Palmyra Sugar as a Renewable Solar Cell, 2024, 12, 2164-6341, 57, 10.32604/jrm.2023.028619 |
a–C layer thickness (nm) | Jsc (mA/cm2) | Voc (V) | FF | PCE (%) |
25 | 0.145 | 0.236 | 0.296 | 0.0102 |
75 | 0.168 | 0.416 | 0.322 | 0.0225 |
100 | 0.188 | 0.314 | 0.324 | 0.0215 |
125 | 0.164 | 0.307 | 0.366 | 0.0184 |