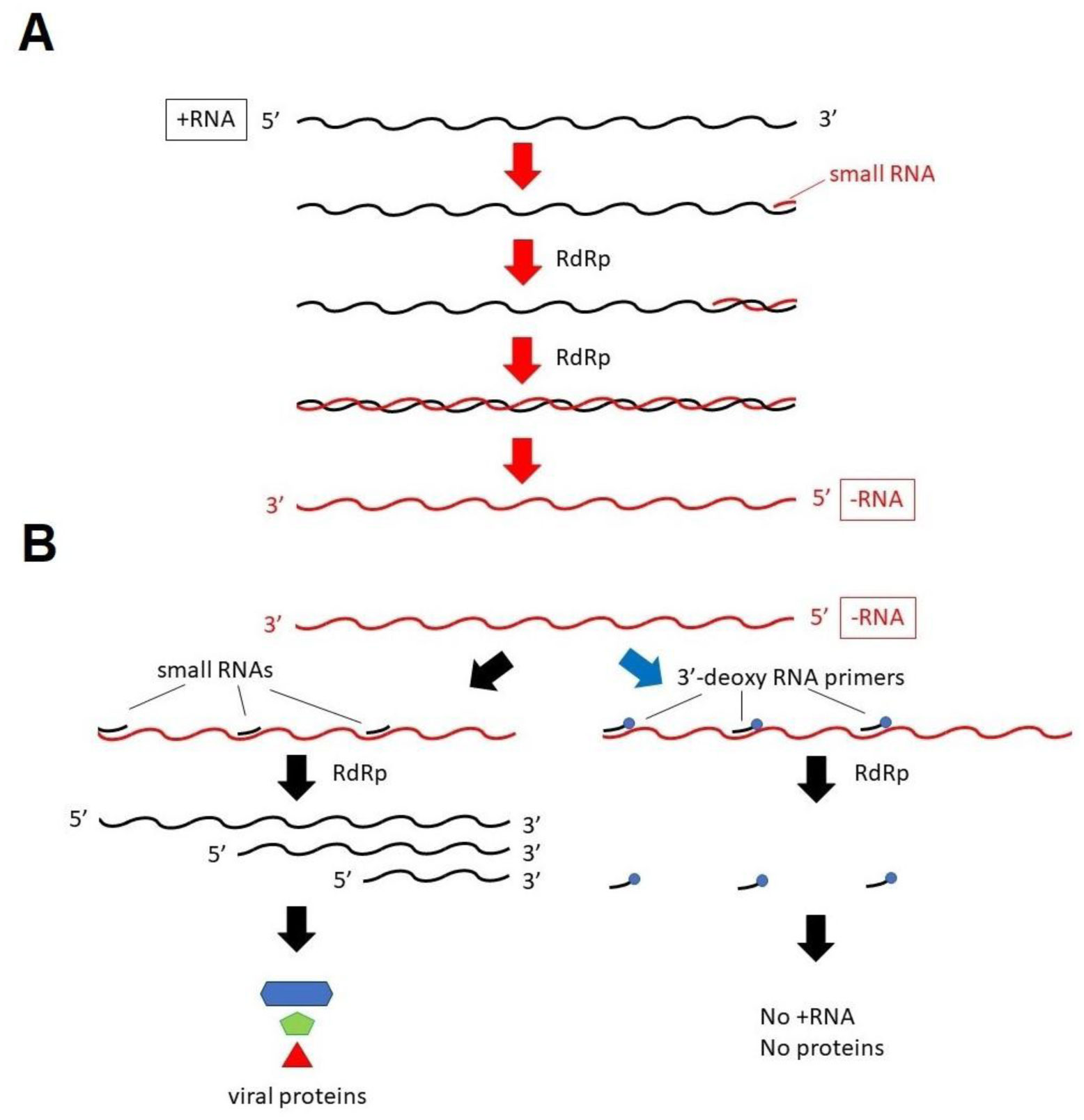
The life cycle of severe acute respiratory syndrome coronavirus 2 (SARS-CoV-2), which is the etiologic agent for coronavirus disease-19 (COVID-19), has been revealed, and the molecular mechanism of how this virus replicates has been studied. Effective drugs could be designed to target one of the replication processes. This virus, which contains plus-strand RNA as a genome, is characteristic because it synthesizes its genome and other transcripts with minus-strand template RNA. These viral RNAs, including its genome, are transcribed by an RNA-dependent RNA polymerase (RdRp). In this article, we discuss whether novel materials from RNA derivatives could be applied to COVID-19 patients to prevent or disturb viral transcription.
Citation: Fumiaki Uchiumi, Yoko Ogino, Akira Sato, Sei-ichi Tanuma. Insights into the development of effective materials to suppress replication of severe acute respiratory syndrome coronavirus 2 (SARS-CoV-2)[J]. AIMS Bioengineering, 2020, 7(3): 124-129. doi: 10.3934/bioeng.2020012
[1] | Júlia Novaes Matias, Gyovanna Sorrentino dos Santos Campanari, Gabriela Achete de Souza, Vinícius Marinho Lima, Ricardo José Tofano, Claudia Rucco Penteado Detregiachi, Sandra M. Barbalho . Metabolic syndrome and COVID-19. AIMS Bioengineering, 2020, 7(4): 242-253. doi: 10.3934/bioeng.2020021 |
[2] | Kranthi Kumar Lella, Alphonse PJA . A literature review on COVID-19 disease diagnosis from respiratory sound data. AIMS Bioengineering, 2021, 8(2): 140-153. doi: 10.3934/bioeng.2021013 |
[3] | Uri Adrian Prync Flato, Karina Vilariço Ferreira, Piero Biteli, Daniela Ortega Balbo Rodrigues Reina, Fábio Tadeu Rodrigues Reina, Fausto Tucunduva Vernaschi, Gabriela Achete de Souza, Gyovanna Sorrentino dos Santos Campanari, Júlia Novaes Matias, Vinícius Marinho Lima, Tereza Lais Menegucci Zutin, Rogério Leone Buchaim, Daniela Vieira Buchaim, Sandra Maria Barbalho . Rabdomyolysis as a serious complication of COVID-19. AIMS Bioengineering, 2021, 8(2): 165-172. doi: 10.3934/bioeng.2021015 |
[4] | Eduardo Federighi Baisi Chagas, Piero Biteli, Bruno Moreira Candeloro, Miguel Angelo Rodrigues, Pedro Henrique Rodrigues . Physical exercise and COVID-19: a summary of the recommendations. AIMS Bioengineering, 2020, 7(4): 236-241. doi: 10.3934/bioeng.2020020 |
[5] | Cong Toai Truong, Kim Hieu Huynh, Van Tu Duong, Huy Hung Nguyen, Le An Pham, Tan Tien Nguyen . Model-free volume and pressure cycled control of automatic bag valve mask ventilator. AIMS Bioengineering, 2021, 8(3): 192-207. doi: 10.3934/bioeng.2021017 |
[6] | Sarbaz H. A. Khoshnaw, Azhi Sabir Mohammed . Computational simulations of the effects of social distancing interventions on the COVID-19 pandemic. AIMS Bioengineering, 2022, 9(3): 239-251. doi: 10.3934/bioeng.2022016 |
[7] | Karthikeyan D. Ramachandriya, Dimple K. Kundiyana, Ashokkumar M. Sharma, Ajay Kumar, Hasan K. Atiyeh, Raymond L. Huhnke, Mark R. Wilkins . Critical factors affecting the integration of biomass gasification and syngas fermentation technology. AIMS Bioengineering, 2016, 3(2): 188-210. doi: 10.3934/bioeng.2016.2.188 |
[8] | Kuna Dhananjay Rao, Mudunuru Satya Dev Kumar, Paidi Pavani, Darapureddy Akshitha, Kagitha Nagamaleswara Rao, Hafiz Tayyab Rauf, Mohamed Sharaf . Cardiovascular disease prediction using hyperparameters-tuned LSTM considering COVID-19 with experimental validation. AIMS Bioengineering, 2023, 10(3): 265-282. doi: 10.3934/bioeng.2023017 |
[9] | Caroline Rhim, William E. Kraus, George A. Truskey . Biomechanical effects on microRNA expression in skeletal muscle differentiation. AIMS Bioengineering, 2020, 7(3): 147-164. doi: 10.3934/bioeng.2020014 |
[10] | Ayub Ahmed, Bashdar Salam, Mahmud Mohammad, Ali Akgül, Sarbaz H. A. Khoshnaw . Analysis coronavirus disease (COVID-19) model using numerical approaches and logistic model. AIMS Bioengineering, 2020, 7(3): 130-146. doi: 10.3934/bioeng.2020013 |
The life cycle of severe acute respiratory syndrome coronavirus 2 (SARS-CoV-2), which is the etiologic agent for coronavirus disease-19 (COVID-19), has been revealed, and the molecular mechanism of how this virus replicates has been studied. Effective drugs could be designed to target one of the replication processes. This virus, which contains plus-strand RNA as a genome, is characteristic because it synthesizes its genome and other transcripts with minus-strand template RNA. These viral RNAs, including its genome, are transcribed by an RNA-dependent RNA polymerase (RdRp). In this article, we discuss whether novel materials from RNA derivatives could be applied to COVID-19 patients to prevent or disturb viral transcription.
Molecular biologists have learned that the transcription of genes is executed by proteins or protein complexes, including TBP, TFIID, and an RNA polymerase II [1]. Elongation of the RNA chain requires ribonucleotide triphosphates as substrates, and an RNA primer is essentially required in in vitro reactions [1]. However, how the primers for the transcription of genes are provided in the cells remains unclear because it is quite obvious that they are present in every cell from its beginning as an egg.
Coronavirus consists of several proteins and a positive-strand RNA (+RNA) as a genome [2]. The complete sequence of the severe acute respiratory syndrome coronavirus 2 (SARS-CoV-2), which causes COVID-19, has been revealed (https://www.ncbi.nlm.nih.gov/nuccore/1798174254). Whole genome sequencing of SARS-CoV-2 indicated that it is most closely related to the bat coronavirus SL-CoVZC45 [3]. It contains several open reading frames that encode viral proteins [2],[3]. To replicate the viral genome, the +RNA chain needs to be transcribed first by an RNA-dependent RNA polymerase (RdRp), which is a non-structural protein 12 (nsp12) encoded on the viral genome [4]–[6]. The transcripts, including minus-strand RNA (-RNA) and templates for translation of the viral proteins, are thought to be produced by the RNA polymerizing enzyme RdRp.
The RdRp enzyme needs a primer to start transcription [4]. Although it has been speculated that the nsp7-nsp8 complex confers RNA-primer synthesis [7], the kind of primers required in the first step to polymerize ribonucleotides has not been precisely indicated or described. We, as molecular biologists, have learned that life has been evolved through the RNA world [8],[9]. Cells must contain short RNAs from the beginning. Presumably, the nucleotide sequences of the viral genome will give us a hint in developing a new strategy to suppress or disturb its replication system. Previously, based on the surveillance of the SARS-coronavirus genome, it was reported that a specific nucleotide sequence 5′-ACGAAC-3′ is present near the translation start sites or first AUG of the open reading frames [10].
To predict possible competitors that could affect replication of the +RNA strand, the 5′-UTR sequence (1 to 265) or the most 5′-upstream of the +RNA was converted to the complimentary -RNA strand, and it was surveyed by the miRBase program (miRbase.org/index.shtml). A few miRNAs were retrieved from this surveillance; they are hairpin RNAs, including hsa-mir-2053 and hsa-mir-668. Of them, hsa-mir-2053 has a sequence in the loop that may hybridize with the most 5′-upstream of the SARS-CoV-2 +RNA. Interestingly, hsa-mir-2053 has recently been reported to be a tumor suppressor miRNA [11]. The hairpin RNA hsa-mir-668 plays a role in the suppression of mitochondrial fragmentation by suppressing MTP18 gene expression to protect from acute kidney injury [12],[13]. We should also remember that some of the miRNAs have antiviral functions [14]. These observations have inspired us to hypothesize that miRNAs may prevent or hinder the initiation or elongation of the +RNA strand on the template -RNA. The clinical data show that worsening of the COVID-19 viral infectious disease appears to occur in older people [15]. This might be due to lower amounts of transcription suppressor miRNAs because the expression profile of miRNAs in lung is altered in accordance with aging [16].
Molecular biologists have learned that DNA sequences can be read by the Sanger method [17]. The DNA sequencing analysis achieved by this method has not only contributed to the scientific field but also to society. Although the analytical equipment has been greatly improved from the original procedure, the sequence of SARS-CoV-2 was revealed by this method. The reaction for the DNA sequencing uses dideoxy nucleotides ATP, GTP, CTP, and TTP. The elongation of the DNA strand stops by incorporation of the dideoxy nucleotide into the 3′-ends. The Honorable Dr. Frederic Sanger might have left a hint for us to develop a new strategy against viral infectious disease. If transcription is initiated by RNA polymerases, including by RdRp, it must require short RNAs that are originally present in host cells (Figure 1A). Moreover, the inhibitory effect of 3′-deoxyribofuranosyl nucleotides (3′-deoxy UTP and 3′-deoxy TTP) could be synthesized chemically [18] to be added to the 3′-end of the RNA primers. Therefore, 3′-end deoxy RNA may prevent elongation of the transcripts.
For example, 5′-auuaaagguuuauaccuucccagguaacaa(deoxy-a)-3′, which could be hybridized with hsa-mir-2053, would prevent the initiation of +RNA transcription. If other 3′-end deoxy RNA primers were annealed to the -RNA strand, no elongation would occur (Figure 1B). The 3′-end deoxy-RNA primers that hybridize to the adjacent sites of the transcription start sites for viral RNAs should be examined (Figure 1B). These 3′-end deoxy-RNA primers would not be so harmful compared with the use of RNA polymerase inhibitory nucleotide derivatives, including favipiravir [19], because the 3′-end deoxy-RNA primers specifically target the transcription start sites of the viral genome. However, the length of the 3′-end deoxy-RNA primers should be determined. Moreover, not only the very first 5′-end of the genome but also the most effective sites at which the artificial aberrant RNA primers anneal on the -RNA strand must be investigated. Additionally, to improve stability, incorporation of modified ribonucleotides should be examined. Such modified ribonucleotides have been already studied [20].
Antisense octaguanidinium dendrimer-conjugated morpholino oligomers showed an inhibitory effect on enterovirus 71, the genome of which is like that of coronavirus, a single +RNA [21]. Because the 3′-end deoxy (modified) RNA primers are short unelongatable sense strands, they are not equal to the antisense RNAs. The false bait RNA primers rather resemble short 5′-capped oligomer RNAs with 3′-phosphates, which inhibit elongation of the +RNA strand on the minus strand template genome of the influenza virus [22]. However, it would be essential to identify the most frequently occurring transcription initiation site(s) on the -RNA strand of the coronavirus. Quantitative analysis or read count plots [3] of the viral transcripts could provide a way to find the precise sequence. It should be noted that the exo- and endoribonucleases nsp14 and 15, respectively, are encoded on the coronaviral genome [23]. As for the influenza virus, it was suggested that endo-RNA nucleases detach inappropriate ribonucleotide to elongate the RNA strand from the nick [22]. Therefore, the length of the false bait RNAs should be examined. Moreover, to effectively suppress elongation, the development of specific inhibitors for nsp14 and 15 is required.
Next, how should these RNAs be applied clinically to patients? Presently, some of the RNAs or miRNAs have been clinically tested to treat lung cancer [24] and could be applied by inhalation. The most difficult impediment to overcome would be the current pharmaceutical regulations, which, of course, must be obeyed. Because these RNAs originally exist in cells, they might be approved as processed nutrients or supplements. However, because such artificial RNAs have never been tested clinically, we cannot predict the consequences in the future. The discovery of antibiotics greatly contributed to the treatment of bacterial infectious diseases, but their repeated use has resulted in the emergence of antibiotic-resistant bacteria [25]. This kind of problem must be considered and avoided.
Not only the 3′-deoxy ribonucleotides but also 3′-modified nucleotides could be applied to the inelongatable RNA primers. For example, 3′-fluoro-3′-deoxyadenosine has been shown to have a suppressive effect on double-stranded RNA viruses [26]. The essential tasks are to construct several 3′-deoxy (or modified)-RNA primers and to examine whether they restrict viral replication and are not harmful to normal healthy cells. Further, the conditions for inhalation must be followed according to established procedures [24]. The sooner this can begin, the better. The authors hope that some of the scientists and physicians involved in drug development will focus on RNA-based therapeutics, and we emphasize that studies on transcription will greatly contribute to the development of effective medicines in the future [27],[28]. We further hope that scientists, researchers, medical institutions, chemical and pharmaceutical companies, governments, and anyone who has been inspired by this article take full advantage of all available resources at hand to develop medications to fight COVID-19.
[1] | Carey MF, Peterson CL, Smale ST (2009) A primer on transcriptional regulation in mammalian cells. Transcriptional Regulation in Eukaryotes: Concepts, Strategies, and Techniques NY: Cold Spring Harbor Laboratory Press, 1-45. |
[2] |
Chen Y, Liu Q, Guo D (2020) Emerging coronaviruses: genome structure, replication, and pathogenesis. J Med Virol 92: 418-423. doi: 10.1002/jmv.25681
![]() |
[3] |
Wu F, Zhao S, Yu B, et al. (2020) A new coronavirus associated with human respiratory disease in China. Nature 579: 265-269. doi: 10.1038/s41586-020-2008-3
![]() |
[4] |
Te Velthuis AJW, Arnold JJ, Cameron CE, et al. (2010) The RNA polymerase activity of SARS-coronavirus nsp12 is primer dependent. Nucleic Acids Res 38: 203-214. doi: 10.1093/nar/gkp904
![]() |
[5] |
Ahn DG, Chi JK, Taylor DR, et al. (2012) Biochemical characterization of a recombinant SARS coronavirus nsp12 RNA-dependent RNA polymerase capable of copying viral RNA templates. Arch Virol 157: 2095-2104. doi: 10.1007/s00705-012-1404-x
![]() |
[6] |
Lung J, Lin YS, Yang YH, et al. (2020) The potential chemical structure of anti-SARS-CoV-2 RNA-dependent RNA polymerase. J Med Virol 92: 693-697. doi: 10.1002/jmv.25761
![]() |
[7] |
Tan YW, Fung TS, Shen H, et al. (2018) Coronavirus infectious bronchitis virus non-structural proteins 8 and 12 form stable complex independent of the non-translated regions of viral RNA and other viral proteins. Virology 513: 75-84. doi: 10.1016/j.virol.2017.10.004
![]() |
[8] | Joyce GF, Orgel LE (2006) Progress toward understanding the origin of the RNA world. The RNA World NY: Cold Spring Harbor Laboratory Press, 23-56. |
[9] | Cech TR (2011) The RNA world in context. RNA Worlds: From Life's Origins to Diversity in Gene Regulation NY: Cold Spring Harbor Laboratory Press, 1-5. |
[10] |
Snijder EJ, Bredenbeek PJ, Dobbe JC, et al. (2003) Unique and conserved features of genome and proteome of SARS-coronavirus, an early split-off from the coronavirus group 2 lineage. J Mol Biol 331: 991-1004. doi: 10.1016/S0022-2836(03)00865-9
![]() |
[11] | Song T, Ma K, Zhao C, et al. (2019) MicroRNA-2053 overexpression inhibits the development and progression of hepatocellular carcinoma. Oncol Lett 18: 2043-2049. |
[12] |
Chun N, Coca SG, He JC (2018) A protective role for microRNA-668 in acute kidney injury. J Clin Invest 128: 5216-5218. doi: 10.1172/JCI124923
![]() |
[13] |
Wei Q, Sun H, Song S, et al. (2018) MicroRNA-668 represses MTP18 to preserve mitochondrial dynamics in ischemic acute kidney injury. J Clin Invest 128: 5448-5464. doi: 10.1172/JCI121859
![]() |
[14] |
Trobaugh DW, Klimstra WB (2017) MicroRNA regulation of RNA virus replication and pathogenesis. Trends Mol Med 23: 80-93. doi: 10.1016/j.molmed.2016.11.003
![]() |
[15] |
Liu K, Chen Y, Lin R, et al. (2020) Clinical features of COVID-19 in elderly patients: a comparison with young and middle-aged patients. J Infect 80: e14-e18. doi: 10.1016/j.jinf.2020.03.005
![]() |
[16] |
Zagryazhskaya A, Zhivotovsky B (2014) miRNA in lung cancer: a link to aging. Ageing Res Rev 17: 54-67. doi: 10.1016/j.arr.2014.02.009
![]() |
[17] |
Sanger F, Nicklen S, Coulson AR (1977) DNA sequencing with chain-terminating inhibitors. Proc Natl Acad Sci USA 74: 5463-5467. doi: 10.1073/pnas.74.12.5463
![]() |
[18] |
Nakayama C, Saneyoshi M (1985) Differential inhibitory effects of 5-substituted 1-β-d-xylofuranosyluracil 5′-triphosphates and related nucleotides on DNA-dependent RNA polymerases I and II from the cherry salmon (Oncorhynchus masou). J Biochem 98: 417-425. doi: 10.1093/oxfordjournals.jbchem.a135296
![]() |
[19] |
Furuta Y, Komeno T, Nakamura T (2017) Favipiravir (T-705), a broad spectrum inhibitor of viral RNA polymerase. Proc Jpn Acad Ser B 93: 449-463. doi: 10.2183/pjab.93.027
![]() |
[20] |
Gampe C, White ACS, Siva S, et al. (2018) 3′-Modification stabilizes mRNA and increases translation in cells. Bioorg Med Chem Lett 28: 2451-2453. doi: 10.1016/j.bmcl.2018.06.008
![]() |
[21] |
Tan CW, Chan YF, Quah YW, et al. (2014) Inhibition of enterovirus 71 infection by antisense octaguanidinium dendrimer-conjugated morpholino oligomers. Antivir Res 107: 35-41. doi: 10.1016/j.antiviral.2014.04.004
![]() |
[22] |
Chung TD, Cianci C, Hagen M, et al. (1994) Biochemical studies on capped RNA primers identify a class of oligonucleotide inhibitors of the influenza virus RNA polymerase. Proc Natl Acad Sci USA 91: 2372-2376. doi: 10.1073/pnas.91.6.2372
![]() |
[23] |
Subissi L, Imbert I, Ferron F, et al. (2014) SARS-CoV ORF1b-encoded nonstructural proteins 12–16: Replicative enzymes as antiviral targets. Antivir Res 101: 122-130. doi: 10.1016/j.antiviral.2013.11.006
![]() |
[24] |
Fujita Y, Kuwano K, Ochiya T (2015) Development of small RNA delivery systems for lung cancer therapy. Int J Mol Sci 16: 5254-5270. doi: 10.3390/ijms16035254
![]() |
[25] |
Walsh C (2000) Molecular mechanisms that confer antibacterial drug resistance. Nature 406: 775-781. doi: 10.1038/35021219
![]() |
[26] |
Van Aerschot A, Herdewijn P, Janssen G, et al. (1989) Synthesis and antiviral activity evaluation of 3′-fluoro-3′-deoxyribonucleosides: broad-spectrum antiviral activity of 3′-fluoro-3′-deoxyadenosine. Antivir Res 12: 133-150. doi: 10.1016/0166-3542(89)90047-8
![]() |
[27] | Uchiumi F (2018) Introductory chapter: Current studies in transcriptional control system; toward the establishment of therapies against human diseases. Gene Expression and Regulation in Mammalian Cells: Transcription From General Aspects London: InTech OPEN, 3-13. |
[28] | Uchiumi F, Asai M (2018) Gene expression controlling system and its application to medical sciences. Gene Expression and Control London: InTech OPEN, 3-15. |
1. | Fumiaki Uchiumi, 2023, 9780128187876, 1, 10.1016/B978-0-12-818787-6.00008-4 |