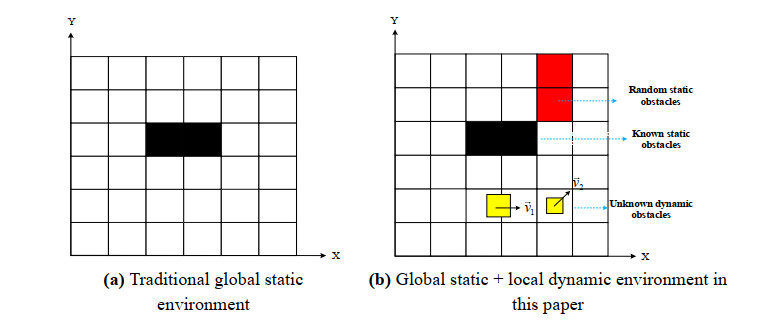
Aquaculture is one of the major economic activities in India, providing livelihoods and nutritional security to millions of people. In recent times, fish diseases have come to the limelight resulting in significant economic losses. We aimed to identify pathogenicity and virulence profiling of thirty-six pathogenic bacterial strains isolated from diseased Labeo rohita in the district of Hooghly, West Bengal, India. The bacterial strains were characterized through a comprehensive approach involving the examination of morphological features, biochemical properties, amplification, and sequencing of the 16S rRNA, species-specific genes, and virulence genes. Considering the prevalence frequency, virulence potential, and statistical significance Aeromonas hydrophila and Pseudomonas aeruginosa were selected for a survival assay followed by the examination of histopathological features to elucidate their effects. The identified bacterial isolates were arranged based on their predominance frequency, i.e., Aeromonas hydrophila (25%), Aeromonas veronii (22%), Pseudomonas aeruginosa (22%), Enterococcus faecalis (14%), Klebsiella pneumoniae (6%), Staphylococcus aureus (6%) and Escherichia coli (5%). Sixteen virulence-associated genes related to pathogenicity were amplified across the thirty-six isolates; aer, alt, lip and hlyA for A. hydrophila; exoS, lasB, toxA, oprL and phzM for P. aeruginosa; entB, fimH and uge in K. pneumoniae; aer in A. veronii; hlyA in E. coli; hlb in S. aureus and gelE for E. faecalis. The log-probit analysis revealed that A. hydrophila was notably more pathogenic than P. aeruginosa, as indicated by its lower lethal dose of 1.5×104 CFU/mL. Additionally, histological examination revealed notable pathological changes, including tissue degeneration, inflammatory cell infiltration and vacuolation observed in the liver, kidney, gill and intestine of the challenged fish. We highlighted several potent aquatic microbial pathogens in order to manage and prevent such aquacultural maladies.
Citation: Abhijit Pakhira, Prasenjit Paria, Biswanath Malakar, Manoharmayum Shaya Devi, Vikash Kumar, Basanta Kumar Das, Asim Kumar Samanta, Santanu Chakrabarti, Bijay Kumar Behera. Identification and virulence gene characterization of pathogenic bacteria from diseased Labeo rohita (Hamilton, 1822): Insight into aquatic animal health management in Indian aquaculture[J]. AIMS Molecular Science, 2024, 11(3): 277-302. doi: 10.3934/molsci.2024017
[1] | Baoye Song, Shumin Tang, Yao Li . A new path planning strategy integrating improved ACO and DWA algorithms for mobile robots in dynamic environments. Mathematical Biosciences and Engineering, 2024, 21(2): 2189-2211. doi: 10.3934/mbe.2024096 |
[2] | Yuzhuo Shi, Huijie Zhang, Zhisheng Li, Kun Hao, Yonglei Liu, Lu Zhao . Path planning for mobile robots in complex environments based on improved ant colony algorithm. Mathematical Biosciences and Engineering, 2023, 20(9): 15568-15602. doi: 10.3934/mbe.2023695 |
[3] | Liwei Yang, Lixia Fu, Ping Li, Jianlin Mao, Ning Guo, Linghao Du . LF-ACO: an effective formation path planning for multi-mobile robot. Mathematical Biosciences and Engineering, 2022, 19(1): 225-252. doi: 10.3934/mbe.2022012 |
[4] | Xuewu Wang, Bin Tang, Xin Zhou, Xingsheng Gu . Double-robot obstacle avoidance path optimization for welding process. Mathematical Biosciences and Engineering, 2019, 16(5): 5697-5708. doi: 10.3934/mbe.2019284 |
[5] | Jian Si, Xiaoguang Bao . A novel parallel ant colony optimization algorithm for mobile robot path planning. Mathematical Biosciences and Engineering, 2024, 21(2): 2568-2586. doi: 10.3934/mbe.2024113 |
[6] | Zhenao Yu, Peng Duan, Leilei Meng, Yuyan Han, Fan Ye . Multi-objective path planning for mobile robot with an improved artificial bee colony algorithm. Mathematical Biosciences and Engineering, 2023, 20(2): 2501-2529. doi: 10.3934/mbe.2023117 |
[7] | Ping Li, Liwei Yang . Conflict-free and energy-efficient path planning for multi-robots based on priority free ant colony optimization. Mathematical Biosciences and Engineering, 2023, 20(2): 3528-3565. doi: 10.3934/mbe.2023165 |
[8] | Tian Xue, Liu Li, Liu Shuang, Du Zhiping, Pang Ming . Path planning of mobile robot based on improved ant colony algorithm for logistics. Mathematical Biosciences and Engineering, 2021, 18(4): 3034-3045. doi: 10.3934/mbe.2021152 |
[9] | Ruiping Yuan, Jiangtao Dou, Juntao Li, Wei Wang, Yingfan Jiang . Multi-robot task allocation in e-commerce RMFS based on deep reinforcement learning. Mathematical Biosciences and Engineering, 2023, 20(2): 1903-1918. doi: 10.3934/mbe.2023087 |
[10] | Jinzhuang Xiao, Xuele Yu, Keke Sun, Zhen Zhou, Gang Zhou . Multiobjective path optimization of an indoor AGV based on an improved ACO-DWA. Mathematical Biosciences and Engineering, 2022, 19(12): 12532-12557. doi: 10.3934/mbe.2022585 |
Aquaculture is one of the major economic activities in India, providing livelihoods and nutritional security to millions of people. In recent times, fish diseases have come to the limelight resulting in significant economic losses. We aimed to identify pathogenicity and virulence profiling of thirty-six pathogenic bacterial strains isolated from diseased Labeo rohita in the district of Hooghly, West Bengal, India. The bacterial strains were characterized through a comprehensive approach involving the examination of morphological features, biochemical properties, amplification, and sequencing of the 16S rRNA, species-specific genes, and virulence genes. Considering the prevalence frequency, virulence potential, and statistical significance Aeromonas hydrophila and Pseudomonas aeruginosa were selected for a survival assay followed by the examination of histopathological features to elucidate their effects. The identified bacterial isolates were arranged based on their predominance frequency, i.e., Aeromonas hydrophila (25%), Aeromonas veronii (22%), Pseudomonas aeruginosa (22%), Enterococcus faecalis (14%), Klebsiella pneumoniae (6%), Staphylococcus aureus (6%) and Escherichia coli (5%). Sixteen virulence-associated genes related to pathogenicity were amplified across the thirty-six isolates; aer, alt, lip and hlyA for A. hydrophila; exoS, lasB, toxA, oprL and phzM for P. aeruginosa; entB, fimH and uge in K. pneumoniae; aer in A. veronii; hlyA in E. coli; hlb in S. aureus and gelE for E. faecalis. The log-probit analysis revealed that A. hydrophila was notably more pathogenic than P. aeruginosa, as indicated by its lower lethal dose of 1.5×104 CFU/mL. Additionally, histological examination revealed notable pathological changes, including tissue degeneration, inflammatory cell infiltration and vacuolation observed in the liver, kidney, gill and intestine of the challenged fish. We highlighted several potent aquatic microbial pathogens in order to manage and prevent such aquacultural maladies.
The technology of autonomous mobile robots is developing rapidly, and it is widely used in entertainment, mining industry, education, medical services, military reconnaissance, agricultural automation, planetary exploration, and other fields [1]. Path planning and obstacle avoidance technology are critical to achieve autonomous robot navigation, which determines the application prospects of mobile robots. Meanwhile, multi-robot obstacle avoidance technology remains a relevant research problem in dynamic and complex environments.
Path planning is generally divided into global and local path planning. Global path planning involves planning an optimal or suboptimal safe path with priori map information [2]. In contrast, local path planning is designed with dynamic obstacle environments in real-time. The robot usually must acquire details about the environment, including the coordinates of static and dynamic obstacles, with the help of a local path planner [3].
Current global path planning in a known environment has attracted significant interest. Numerous algorithms have been explored, including the A* algorithm [4,5,6], ant colony optimization [7,8], particle swarm optimization [9,10], bacterial foraging optimization [11,12], bat algorithm [13,14], and whale optimization algorithm [15,16], etc. With the advantages of a simple structure, facilitated implementation, and fast planning, the A* algorithm has been popular among researchers [17]. Wang et al. [5] used a bidirectional search strategy to improve the A* algorithm, which significantly enhanced the search performance by simultaneously conducting the iterative search in both positive and negative directions. Zhang et al. [6] enhanced the node expansion method of the A* algorithm based on the jump point search (JPS) strategy, which significantly reduced the memory overhead and the search scale. We further mention some research on intelligent optimization algorithms. Miao et al. [8] introduced an angle guidance factor and an obstacle exclusion factor in the transfer probability of ant colony optimization, and the global search ability and convergence speed of the algorithm were balanced. Song et al. [9] combined an adaptive fractional-order, velocity-improved PSO algorithm with the continuous high-degree Bezier curve to plan smooth paths for mobile robots. Hossain et al. [11] searched for the shortest path in a dynamic environment based on the bacterial foraging optimization algorithm. Tang et al. [13] presented the first application of the bat algorithm to a collaborative multi-robot search task in an unknown environment. They used adaptive inertial weights and the Doppler effect to improve the frequency formulation to avoid premature convergence. Yan et al. [15] proposed a whale optimization algorithm based on the forward-looking sonar to solve the 3D path planning problem for UUVs, with strong stability and search capability.
The studies mentioned above [4,5,6,7,8,9,10,11,12,13,14,15,16] conducted some work to improve the efficiency of path planning. However, they yielded a few practical solutions to the obstacle avoidance problem of mobile robots in the actual dynamic environments. Ensuring the safety of robots with the help of local path planning is an effective solution when the environment is dynamic and full of uncertainties [18], and the main popular local path planning algorithms are the dynamic window approach (DWA) [19] and artificial potential field method (APF) [20], etc. DWA is a highly efficient, real-time obstacle avoidance algorithm that transforms the path planning problem into the constrained optimization problem of the velocity space and controls the robot motion by outputting the optimal real-time speed [19]. However, DWA faces problems, such as local optima and a low successful obstacle avoidance rate for dynamic obstacles. Therefore, Chang et al. [21] modified and extended the evaluation function of DWA and used the reinforcement learning method to adaptively adjust parameters, which further enhanced the planning effect. Lin et al. [22] improved the avoidance rate of DWA against dynamic obstacles by using a fuzzy control scheme to evaluate the danger level of moving obstacles through collision risk index and relative distance. Furthermore, there has been significant interest in APF. Cheng et al. [23] introduced optimal control theory to reformulate the UAV path planning problem as a constrained optimization problem with APF. Orozco et al. [24] solved path planning problems in dynamic environments by combining membrane computing with a genetic algorithm and APF. In general, it is popular to combine local path planning algorithms with global ones to cope with environments of increasing complexity and uncertainty. The hybrid algorithms allow the robot to connect global path optimality and stochastic obstacle avoidance to a relatively large extent [25]. Ji et al. [26] combined the A* algorithm with an adaptive DWA for global path planning research that solves robot motion in a complex environment. Wang et al. [27] combined the improved PSO algorithm with APF for USVs to solve the dynamic path planning problem in complex offshore regions.
The studies mentioned above [19,20,21,22,23,24,25,26,27] explored the global or local path problems from different perspectives, but with less attention to multi-robot obstacle avoidance. The aim of multi-robot path planning is to find a conflict-free path from the start to the target for each robot. The motion of mobile robots is disturbed not only by known factors in the global environment, but also by dynamic obstacles and other autonomous robots, which making it necessary and practical to design an obstacle avoidance system for multiple autonomous robots. In the context of research on multi-robot strategies, the main approaches are either centralized or distributed. The centralized approach considers the cost or objective function, where the constraints for all robots are considered together, thus obtaining the paths of individual robots in a global search. It prioritizes completeness with less attention to the personal robot [28]. One of the more popular ways to employ the centralized approach is the formation control, where the mission planning information and formation information is integrated into a leader robot, while the other robots act as followers. The leader coordinates the actions of each follower to maintain the formation from the start to the end. Dai et al. [29] proposed a multi-robot formation switching strategy incorporating a priority model, where the leader robot with the highest priority is responsible for planning a safe path and guiding the follower robots, and the following robots switch into an obstacle avoidance formation by calculating the desired distance and angle. Sang et al. [30] combined A* and APF for the USVs formation problem, using the A* algorithm to plan the globally optimal path, dividing it into multiple sub-target points, and used the improved APF for path tracking and performing formation obstacle avoidance. In distributed multi-robot path motion planning, each robot independently determined its collision-free trajectory path towards the goal without colliding with static obstacles or colleagues. The navigation problem for a distributed-based multi-robot is divided into path planning and movement phases, planning a globally optimal path for each robot and maintaining the safety of multi-robot movement. Das et al. [31] added the consideration of path deviation and energy consumption optimization by embedding the social and cognitive behavior of an improved particle swarm algorithm (IPSO) into the Newtonian gravity of an enhanced gravity search algorithm (IGSA). They proposed IPSO-IGSA to implement path planning for multiple robots in dynamic environments and improve search capability by simultaneously updating particle positions using IPSO velocity and IGSA acceleration. In subsequent research, the authors [31] further investigated the multi-robot collision-free planning problem by mixing improved particle swarm optimization (IPSO) and evolutionary operators (EOPs) [32]. Further, some scholars [33] set different priorities for each robot by the prioritization method, thus reducing the possibility of robot collisions.
This study proposes a distributed multi-robot navigation and obstacle avoidance method in unknown environments, applying it to path planning and navigation. The main contributions are as follows:
1) In global path planning:
A jump point search strategy and a bidirectional alternating search strategy are introduced to the conventional A* algorithm, and heuristic functions are designed based on its characteristics, called BAJPSA*, which we efficiently obtain the robots' globally optimal path.
2) In local path planning:
➢ First, considering dynamics and environmental constraints, the robots are constructed based on DWA. Then, the adaptive navigation strategy and path deviation evaluation function are proposed for improving the path tracking accuracy and optimality of our robots.
➢ Second, according to the potential collision situations between the robot and dynamic obstacles, we improved the obstacle recognition method and designed three obstacle avoidance rules, which increase the robot's success rate in avoiding dynamic obstacles with a higher move velocity or bigger size.
➢ Finally, the distributed multi-robot systems are extended from our above single-robot obstacle avoidance algorithm. Focusing on the motion conflicts among multiple robots, we propose a collision recognition strategy and fuse it with a task prioritization strategy to coordinate the robots' motion and obstacle avoidance.
This paper is organized as follows: Section 2 describes our BAJPSA* algorithm. Section 3 describes our multi-robot motion planning algorithm. Section 4 discusses the experiments. Section 5 concludes the whole paper and discusses future work.
The A* algorithm is a classical heuristic search algorithm, where the algorithm selects the node with the smallest evaluation value as the next expanded node in the search process [34], and the evaluation function is expressed as:
f(n)=g(n)+h(n) | (1) |
where n is the current node and the evaluation function f(n) is used to calculate the total cost of the current node; g(n) is the actual cost from the starting point to the current node n; h(n) is the heuristic function used to estimate the cost from the current node n to the target point.
The A* algorithm is a search method based on grid traversal [35], such that it must establish a suitable motion environment for mobile robots. A 2D grid map is shown in Figure 1, where Figure 1(a) is the most widely investigated environment for robots with a priori knowledge, containing black obstacle regions and passable white regions. Figure 1(b) represents our multi-robot motion environment considering practical factors, expanding the unknown local elements of the robot, further including unknown static obstacles (red grid) and unknown volume size and motion velocity of the obstacles (yellow grid).
The JPS algorithm was proposed by Daniel Harabor and Alban Grastien [36,37] in 2011, which is based on the A* algorithm to find paths by defining and computing heuristic values for those nodes on the uniform cost grid graph where the jumping rules are satisfied. It is several orders of magnitude faster than the A* algorithm in terms of computational speed, and the memory overhead as well as the computational effort are significantly reduced, which has been proved by Harabor et al. [36]. The main steps of JPS include two parts [36]: (1) pruning rules, which filter out the nodes in the grid map that do not need to be expanded and eliminate them. (2) Jumping rules, which identify the jump nodes in the grid map and evaluate them.
The set of neighboring nodes around node x is defined as the neighbors(x), and the cost of moving one grid in straight is 1, and diagonal is √2. The function of pruning rules are to recursively prune the set of neighbours around each node, which means pruning all nodes that can be reached optimally by a path that does not visit the current node. Besides, the process of pruning rules is performed entirely online, involves no preprocessing and has no memory overhead.
Situation 1: neighbors(x) contains no obstacle
1) Straight moves
When node x is not adjacent to an obstacle, and the algorithm is extended along the straight direction, the node n∈neighbors(x) that satisfies Eq (2) will be pruned.
len(⟨p(x),...,n⟩∖x)≤len(⟨p(x),x,n⟩) | (2) |
where len represents the cost of the path; ⟨p(x),x,n⟩ represents the path from parent node p(x) to node n through node x; ⟨p(x),...,n⟩∖x represents the path from parent node p(x) to node n directly without passing through node x.
As shown in Figure 2(a), there exists a path π'=⟨p(x),2⟩ from node p(x) to node 2 that is shorter than the path π=⟨p(x),x,2⟩ from node p(x) to node 2 via node x. Therefore, the gray nodes{1, 2, 3, 7, 8, 9} need to be pruned according to Eq (2). Only the remaining node 6, marked white, needs to be considered, which is called the natural neighbor of node x.
2) Diagonal moves
When node x is not adjacent to an obstacle, and the algorithm is extended along the diagonal direction, the node n∈neighbors(x) that satisfies Eq (4) will be pruned.
len(⟨p(x),...,n⟩∖x)<len(⟨p(x),x,n⟩) | (3) |
As shown in Figure 2(c), there exists a path π'=⟨p(x),4,1⟩ that is shorter than the path π=⟨p(x),x,1⟩ that goes through node x. All gray nodes n that satisfy this condition, such as 1, 4, 8 and 9 will be pruned, and the leaved white node 2, 3 and 6 are the natural neighbors of the current node x.
Situation 2: neighbors(x) contains an obstacle
Furthermore, when neighbors(x) contains an obstacle, Eq (2) will not be able to prune all non-natural neighbors due to the presence of obstacles. Therefore, the concept of the forced neighbor is introduced. A node n∈neighbors(x) is forced if:
1) n is not a natural neighbor of node x;
2) n satisfies the rule of Eq (3).
len(⟨p(x),x,n⟩)<len(⟨p(x),...,n⟩∖x) | (4) |
As shown in Figure 2(b), node 3 is a non-natural neighbor of node x, and the path π'=⟨p(x),8,6,3⟩ is longer than the path π=⟨p(x),x,3⟩ from node p(x) to node 3 via node x. Therefore, node 3 marked with blue need to be forcedly considered. Similar to Figure 2(b), node 1 in Figure 2(d) is also a forced neighbor of node x
Node y is the jump point from node x, heading in direction →d, if y minimizes the value k such that the y=x+k→d and one of the following conditions holds:
(1) Node y is the target node.
(2) Node y has at least one neighbour that is a forced neighbor.
(3) There exists a node z=y+ki→di that lies ki∈N steps in direction →di and node z is a jump point successor of node y according to condition (1) or condition (2).
where →d represents a diagonal move, and →di represents two straight moves at 45° to →d as →d1 and →d2. y=x+k→d represents that node y can be reached by taking k unit moves from node x in diagonal direction →d. z=y+ki→di represents that node z can be reached by taking ki unit moves from node y in straight direction →di.
Figure 3 shows an example of a jump point identified by Condition 3. The dashed line indicates the process of the JPS algorithm searching along the diagonal direction after failing in a straight direction, and the solid lines indicate the path formed by node x and jump points. According to Condition 2 of the jumping rules, nodes x and z have a forced neighbor w and v, respectively, so nodes x and z are jump points. According to Condition 3, node y can be reached along the horizontal direction from jump point z, such that node y is also a jump point.
Bidirectional search defines the forward search from the starting point to the target point and the reverse search from the target point to the starting point, however, it has the following two problems:
1) As shown in Figure 4, the bidirectional search is conducted from the starting point and the target point at the same time, which may result in two different paths being searched.
2) Theoretically, forward and backward searching simultaneously search toward the target and starting points and meet at their geometric center [38]. In this case, the algorithm has the highest search efficiency. However, the obstacle density and distance between jump points are different, and the paths may not meet at the midpoint.
For the above reasons, we use a bidirectional alternating search strategy, where the forward and backward searches are alternated, and only the forward search finds the jump point before starting the backward search. In this way, the forward and reverse searches meet at the midpoint as much as possible and will benefit the search efficiency of our algorithm. The specific steps of the BAJPS strategy are as follows:
Step 1: Create two OPEN lists and two CLOSE lists: OPEN_1 and CLOSE_1 are used to store the jump points to be checked, and the expanded jump points in the forward expansion process, respectively, and OPEN_2 and CLOSE_2 are used to store the jump points to be checked and the expanded jump points in the reverse expansion process. Add the starting node S to OPEN_1, add the target node T to OPEN_2, and set both CLOSE lists to empty.
Step 2: Alternate forward and reverse iterative jump point searches, starting with the forward search.
(1) If there was at least one node in the list of OPEN_1, select the lowest cost node n based on the valuation function f(n); if node n was the target point, the search process is terminated, and the path returned; otherwise, the node n is removed from the list of OPEN_1 and added to the list of CLOSE_1.
(2) Starting from node n, continue to search jump point pn1 in the direction of its natural successors. Horizontal and vertical search directions are executed preferentially and only consider diagonal directions when obstacles are encountered, or map boundaries are reached.
A. If there was no searched jump point or the returned node pn1 was in CLOSE_1, it is ignored.
B. If the returned node pn1 was not in the OPEN_1, add it to OPEN_1 and calculate its g(n), h(n), and f(n). Regard the node n as the parent node of the node pn1.
C. If the node pn1 was in the OPEN_1, update g(n) and calculate whether g(n) is below its previous value. If yes, change the node n as the parent node of the node pn1. and calculate f(n).
Step 3: The reverse search for jump point pn2, with its corresponding parent node n2, begins as soon as the forward search is completed and obtains jump point pn2.
Step 4: The forward and backward jump points are searched alternately, and when there are the same jump points in the CLOSE list, the search would be finished.
Step 5: From the same jump point that appeared in Step 4, connect the jump points deposited in forward and reverse directions in sequence to obtain the eventual route.
The traditional A* algorithm uses the Euclidean distance, Manhattan distance, or Chebyshev distance to calculate the heuristic function [39] and the distance functions are as follows:
h(n)=√(x2−x1)2+(y2−y1)2 | (5) |
h(n)=|x2−x1|+|y2−y1| | (6) |
h(n)=max(|x2−x1|,|y2−y1|) | (7) |
where (x1,y1) and (x2,y2) denote the coordinates of the current and the target nodes, respectively.
The A* algorithm with Manhattan distance performs a four-directional search. In contrast, considering that the Euclidean distance is expanded to a broader eight-neighborhood, the obstructive effect of obstacles within the environment will lead to the heuristic value of the evaluation function being smaller than the actual value. Therefore, we combine Euclidean distance and Chebyshev distance to design a heuristic function that is more consistent with our BAJPSA* algorithm as Eq (8). The improved heuristic function can appropriately reduce the weight of the Chebyshev distance according to the JPS to improve the solution of the optimal path. The obtained heuristic value is closer to the actual path cost and further reduces the number of nodes to be evaluated, which improves the search efficiency of the algorithm.
h(n)=√(x2−x1)2+(y2−y1)2+max(|x2−x1|,|y2−y1|)2 | (8) |
The pseudo-code of path planning process based on the BAJPSA* algorithm is as follows:
Algorithm 1. Bidirectional Alternating Jump Point Search A* (BAJPSA*) | |
1: | Initialize the grid map; |
2: | Open_1, Close_1; Open_2, Close_2 ← The jump points to be checked and the expanded jump points in the positive and reverse process, respectively; |
Put the starting point into Open_1; Put and the endpoint into Open_2; | |
3: | If Open_1 or Open_2 is an empty node; |
4: | Pathfinding failed; |
5: | Return; |
6: | else |
7: | While Positive node ~=Reverse node do |
8: | Calculate the f (n) value of all nodes in the Open_1 and Open_2 according to Equations (1) and (8); |
9: | Close_1 and Close_2 ← The node with the smallest f (n) value in the Open_1 and Open_2, respectively; |
10: | Positive node and Reverse node = the node with the smallest f (n) value, respectively; |
11: | If Positive node ==Reverse node; |
12: | The optimal path is obtained and the algorithm ends; |
13: | Break; |
14: | Else |
15: | While (Positive node & Reverse node)~= [] do |
16: | Search for jump points horizontally and vertically alone the direction from the parent node to the current node; |
17: | if Encounter obstacles or map edges; |
18: | Search for jump points diagonally alone the direction from the parent node to the current node; |
19: | Elseif Search for jump points; |
20: | Close_1 or Close_2 ← The searched jump points; |
21: | Else |
22: | Ignore the node, continue searching; |
23: | end |
24: | end |
25: | end |
26: | end |
27: | end |
3) Multi-robot motion planning
Research on mobile robots relying on multiple sensor fusion technologies to sense the surrounding environment information, combined with appropriate local path planning algorithms to avoid moving obstacles or seeking dynamic goals, has been among the most popular topics in the field of robotics in recent years [40]. The APF method is favored by scholars owing to its high flexibility and smooth planning trajectory [41]. However, there are problems such as path oscillation and difficulty in ensuring path optimality when facing the actual, more complex natural dynamic environment. This study proposes an improved dynamic window approach (DWA) in Section 3.4 with great real-time and flexibility to make our multi-robot adapt to more complex and changing environments.
Considering the two-wheel differential robot kinematic model shown in Figure 5, x(t),v(t) and θ(t) are the linear velocity, angular velocity and direction of motion of the robot at the current moment t, respectively. Then, the motion state of the robot at the moment t+1 can be expressed as:
{x(t+1)=x(t)+v(t)Δtcos(θ(t))y(t+1)=y(t)+v(t)Δtsin(θ(t))θ(t+1)=θ(t)+ω(t)Δt | (9) |
DWA describes the obstacle avoidance as an optimization problem with constraints in the velocity space. The conditions mainly include the incomplete constraints of the differential robot, the limitations of environmental obstacles, and the dynamics constraints of the robot structure. As shown in Figure 6, the search space of the robot is constrained by its maximum and minimum speed, motor performance, and braking distance to constrain the motion speed (v,w) within a certain range.
According to the velocity limit of the robot, vs is defined as the set of linear and angular velocities of the robot to reflect the maximum range of the search solution, and the velocity constraint of the robot is:
vs={(v,ω)|v∈[vmin,vmax],ω∈[ωmin,ωmax]} | (10) |
In practice, the robot is limited by the motor torque constraints. It is theoretically impossible to reach the maximum and minimum reachable linear velocity v and angular velocity ω, such that the search range of the dynamic window is further reduced. Given the linear velocity vc and angular velocity ωc, the velocity vd in the Δt sampling period under the considered motor constraint is:
vd={(v,ω)|v∈[vc−aminvΔt,vc+amaxvΔt],ω∈[ωc−aminωΔt,ωc+amaxωΔt]} | (11) |
where vc and ωc are the linear and angular velocities at the current moment, respectively; aminv and aminω are the minimum linear and the minimum angular deceleration, respectively; amaxv and amaxω are the maximum linear and the maximum angular accelerations, respectively, and Δt is the sampling time.
The trajectory of the whole robot can be subdivided into several straight lines or circular arcs. To ensure the robot's safety area, the current speed must be able to decelerate to zero before hitting the obstacle under the maximum deceleration condition. Then, the braking distance of the robot is constrained as follows:
va={(v,ω)|v≤√2·dist(v,ω)aminv,ω≤√2·dist(v,ω)aminω} | (12) |
where dist(v,w) is the distance between the simulated trajectory (based on velocity group (v,w)) and the nearest obstacle, that the simulated speed must satisfy 0−v2a=−2dist(v,w)aminv and 0−w2a=−2dist(v,w)aminw to guarantee the robot's safety to a greater extent.
In summary, according to the three constraints of the robot search space, the input range for velocity control can be expressed as follows:
vr=vs∩vd∩va | (13) |
The robot's linear velocity v(t) and angular velocity ω(t) are sampled and combined with its kinematic model to simulate several trajectories within ns. The evaluation function selects the trajectory with the highest evaluation value, and the corresponding velocity group (v,w) is passed to the robot motion. The traditional evaluation function is as follows:
G(v,ω)=σ(α·Head(v,ω)+β·dist(v,ω)+γ·vel(v,ω)) | (14) |
where Head(v,ω) is the navigation function, which indicates the azimuthal deviation between the end direction of the trajectory and the current target point; dist(v,ω) is the obstacle avoidance function, which shows the distance between the trajectory and the nearest obstacle; vel(v,ω) is the evaluation function of the robot motion speed at the current moment; σ is the normalization process; α,β and γ are the weighting coefficients of the corresponding evaluation functions, respectively.
The widest method [26] takes the turning points of global path planning as the crucial waypoint to guide the robot's motion. However, this is not suitable for the case of power inspection robots, where the global path tracking accuracy must be strictly guaranteed. Besides, traditional DWA is ineffective for avoiding dynamic obstacles in an unknown environment and is highly susceptible to collision with such obstacles. To increase the obstacle avoidance and global path tracking capability of our multi-robot systems in a dynamic environment, we enhance the performance of the evaluation function of conventional DWA and propose a solution to the multiple conflicts that exist between dynamic obstacles and multiple robots.
1) Improvement of Head(v,ω) evaluation function target point tracking method
In related studies [42], the most critical nodes that provide navigation information for robots are turning points of the global path, and the path tracking accuracy is poor. We investigated the method of Yang et al. [40] that extracted the nodes of three times B-spline paths as key navigation points, and designed the function Remake[.] to reorganize the path Route generated by BAJPSA*, as shown in Figure 7 and Eq (15):
NewRoute=m∑i=2Remake[Route(i),Route(i−1),nds] | (15) |
where Remake[.] is the crucial navigation point extraction function, designed in the following way: First, connect the adjacent path points Route(i) and Route(i−1), following form a line equation, then solve for the sequence of node coordinates (x,y) that satisfies the desired node distance nds on that line from the starting point Route(i−1) and deposit them into NewRoute in turn until all equations composed of the path Route are cycled through; m is the number of critical nodes of Route (includes: start point, endpoint and turning points).
To avoid continuous acceleration as well as deceleration and improve the accuracy of the robot's trajectory tracking, we set the desired distance d1. When the distance distance(.) from the endpoint tra(x,y) of the optimal trajectory evaluated by Eq (14) to the target point target(t) at moment t is less than the expected distance d2, the critical navigation point at the moment t+1 is obtained from NewRoute (.) in advance.
target(t+1)=NewRoute(floor(j·d1/nds)),distance(tra,target(t))<d2 | (16) |
where target(t+1) represents the navigation information point of the robot at moment t+1, that is, when the distance between the optimal trajectory tra(x,y) and the target point is less than d2, the next navigation point changes from NewRoute(floor(j(1)·d1/nds)) to NewRoute(floor(j(2)·d1/nds)), j is a sequence of consecutive positive integers; floor(.) is a rounding operation and the number of path node intervals can be estimated by the desired distance d1 and the desired inter-node distance nds.
Take Figure 7 as an example, we can analyze the action of Eqs (15) and (16) in more detail: the original path contains three path nodes Route(i−1,i,i+1), then the new path NewRoute with a large amount of node information is generated by Eq (15) and the distance between every two nodes is nds; Next, we set the desired distance d1 in Eq (16) as a way to extract the robot's motion navigation points from the new path NewRoute. Furthermore, when the condition distance(tra,target(t))<d2 is satisfied, the robot will receive the new navigation information in advance.
2) New path(v,ω) evaluation function
As shown in Figure 8, the experimental results in relevant literature indicate that most robots tend to deviate from the global path to some extent near the turning point, primarily due to the complexity of the environment. To make our robot consider the degree of global deviation during local path selection, we propose a new path(v,ω) function based on the original evaluation function to ensure that the robot moves along the global path as much as possible. The improved evaluation function as Eq (17) and the pseudo-code of the improved dynamic window approach is shown in Algorithm 2.
G(v,ω)=σ(A·Head(v,ω)+B·dist(v,ω)+C·vel(v,ω)+D·path(v,ω)) | (17) |
Algorithm 2. Improved dynamic window approach (IDWA) | |
1: | Initializing (grid map, Robot, Evaluation_Factor); |
2: | Robot =[vmax,ωmax,amaxv,amaxω,VelocityResolution,RPMResolution] ; |
3: | Evaluation_Factor = [A; B; C; D]; |
4: | global_path ←Algorithm1 (BAJPSA*); |
5: | sensor_messages ← Robot; |
6: | if the trajectory without obstacles ← the Traditional DWA combined with the strategy in Section 3.4.2 to filter trajectories |
7: | while the local target location is not reached do ← the local target point information is obtained from equations (15-16) |
8: | Speed sampling of robot; |
9: | Simulate motion trajectories; |
10: | Use the improved evaluation function (17) to select the optimal trajectory; |
11: | Robot follows the optimal trajectory to move; |
12: | end |
13: | end |
Considering the complex dynamic environment and environmental characteristics of multi-robot work comprehensively, our robot must solve not only the path fitting problem at the turning point, but also the path offset problem during dynamic obstacle avoidance. To this end, we design three path(v,ω) functions to correct the global path tracking capability of the robot by considering the distance relationship between the robot and the obstacles as well as the global path.
Situation 1: If the robot is far from the obstacle but deviates from the global path to a lesser extent, global path tracking is guaranteed as a priority.
Path(v,ω)=11+min(path),min(dist(v,ω))≥l1&min(path)≤l2 | (18) |
where path=√(x1−x2)2+(y1−y2)2, (x1,y1) and (x2,y2) denote the local path coordinates planned by the robot according to the kinematic model and the global path coordinates obtained by our BAJPSA* algorithm, respectively; min(dist(v,ω)) denotes the closest distance from the end of the predicted trajectory to the edge of the obstacle; min(path) denotes the most relative distance from the robot to the global path; l1 denotes the desired obstacle avoidance distance of the robot from the obstacle in the case of small deviation from the global path; l2 denotes the maximum error of the robot from the global path.
Situation 2: If the robot is close to the obstacle and deviates from the global path to a small extent, the weight D of the Path(v,ω) function is 0. Then, our robot's obstacle avoidance effectiveness is guaranteed preferentially in Eq (17).
{Path(v,ω)=11+min(path)D=0,min(dist(v,ω))<l1&min(path)≤l2 | (19) |
Situation 3: If the robot is far from the obstacle and deviates from the global path to a large extent, the robot is prompted to move closer to the global path by increasing the evaluation metric of Path(v,ω) in Eq (17).
{Path(v,ω)=min(path)D=1,min(dist(v,ω))≥l3&min(path)>l2 | (20) |
where l3 denotes the desired distance of the robot from the obstacle in the case of a large deviation from the global path.
1) Dynamic obstacle recognition area
The traditional DWA does not identify whether the obstacle is dynamic or static when performing trajectory selection, such that the example shown in Figure 9 will mistakenly identify all red trajectories as collision trajectories and discard them. Based on the evaluation function, the robot most likely to select the path with the relatively best score from the green trajectories. However, dynamic obstacles (pedestrians, vehicles, etc.) are in constant motion, and the robot will continue to move in this way by selecting a green trajectory in the following path selection process. The final result is an awkward situation, where either robot collides with the obstacle or gets stuck in a local optimum of following the motion of the obstacle. A typical collision scenario is shown in Figure 10, where a conventional robot lacks effective recognition of dynamic obstacles to make timely decisions, and the collision occurs at the moment t1. In order to improve the safety and reliability of robot motion, our robot adds an appropriate recognition area for such moving obstacles, which reduces the risk of conflict to some extent.
In the natural environment, dynamic obstacles have different volume sizes, so we considered a circular recognition area that can accommodate the whole object. Considering grid environment effects and the movement speed of obstacles, the actual volume of dynamic obstacles in this paper are square-shaped and not more than one grid(1 m), and the robot's circle recognition radius R is as follows:
R=N⋅r and R≤1m | (21) |
where r is the value of the circle's radius that just contains the dynamic obstacle; N is a positive number greater than 1, and the specific value is obtained from experimental. Since the side length of the grid is 1 m, the recognition radius satisfies R≤1m to avoid the situation that the robot cannot search the path effectively to avoid dynamic obstacles in the case of dense global obstacles.
2) Research on dynamic obstacle avoidance strategy
Referring to the research of Liang et al. [43] on the obstacle avoidance scenarios for the unmanned boat with sea surface, we similarly considered multiple types of motion conflicts between the terrestrial robot and dynamic obstacles, and developed the conflict types as well as obstacle avoidance rules, shown in Figure 11 and Table 1 accordingly. After expanding the robot's recognition area of dynamic obstacles, the frontal and rear-end collision problems can be better solved. However, the lateral collision problem (including left collision and right collision) still presents the dilemma shown in Figure 9, for which the following motion constraints are imposed on the robot:
Step 1: When the quantization criteria of robot and obstacle motion direction satisfy the lateral collision scenario, the robot and dynamic obstacle are judged to be in potential motion conflict, based on whether the shortest distance lr from the end of the robot's predicted trajectory group tria to the obstacle identification region is less than the desired obstacle avoidance distance le. If the condition is satisfied, proceed to step 2. If not, the robot performs obstacle avoidance according to our improved DWA.
Step 2: The robot simulates the trajectory group in tf time period, discarding those speed groups (v,w) and trajectories tria that touch the static obstacle and dynamic obstacle recognition areas.
Step 3: Safe driving distance judgments. Evaluate the optimal trajectory best(tria) according to the evaluation function, and calculate the distance l from the end position of the optimal trajectory best(tria) to the dynamic obstacle recognition area. If l<le still exists at this time, the conflict cannot be lifted, and the robot cannot avoid obstacles successfully. Let the optimal trajectory group tria correspond to the velocity group (v,w)=0. Then the robot will stop the motion quickly under the braking constraint.
Step 4: If the distance l from the end position of the optimal trajectory best(tria) to the dynamic obstacle recognition region is greater than the desired obstacle avoidance distance le, the conflict is lifted, and the robot resumes motion according to our DWA.
Situation | Quantitative standard | Avoidance rule |
frontal collision | θ∈[315°,45°] | Avoid obstacles |
rear-end collision | ϕ∈[120°,240°] | Surpass or follow |
right collision | θ∈[45°,120°] | Avoid obstacles or decelerate to stop |
left collision | θ∈[240°,315°] | Avoid obstacles or decelerate to stop |
Note: where θ represents the movement direction of the obstacle towards the robot; ϕ represents the movement direction of the robot relative to the obstacle. |
The path planning problem of multiple mobile robots is extended from the path planning problem of a single mobile robot. We plan a globally optimal path for each mobile robot in the environment by BAJPSA* algorithm. The DWA obstacle avoidance strategy mainly applies to the static environment or local small-scale dynamic environment (low-speed motion with disturbing obstacles, etc.) [44]. When applied to the multi-robot systems, this does not solve the phenomenon of motion conflict between multiple robots more efficiently [45]. Therefore, we studied the robot's obstacle avoidance strategy for dynamic obstacles through extensive experiments and proposed the dynamic obstacle avoidance rules of 3.4.2. In addition, the priority method [46], as one of the current mainstream techniques for coordinated collision avoidance, can dissipate local conflicts between robots to achieve collision avoidance coordination. To yield better results in global multi-robot motion planning, we combine the BAJPSA* algorithm, improved DWA, and dynamic obstacle avoidance strategy with the multi-mobile robot priority strategy. The algorithm's complexity is simplified by reducing the path planning problem to a dynamic path planning problem with a sequential order for a single mobile robot.
When there are multiple moving robots, the limited environment space is not sufficient for all of them to avoid obstacles. There is an optimal local situation if the robots are treated as dynamic obstacles with the dynamic obstacle avoidance strategy we presented in the previous study. Therefore, we introduce a prioritized strategy and a deceleration mechanism. Different robots have different priorities. When the robot with lower priority encounters one with higher priority and creates a motion conflict, this robot decelerates and stops in advance. The robot with higher priority treats this robot as static obstacle avoidance. As shown in Figure 12: It is assumed that the priority of AGV1, AGV2 and AGV3 decreases in that order. When there is a collision conflict among all three robots, the lower priority AGV2 and AGV3 decelerate to zero. After AGV1 leaves the conflict area, there is still a collision conflict between AGV3 and AGV2. AGV3 stops and waits, resuming motion when AGV2 departs and the collision conflict is lifted.
The constraints introduced by the robot's kinematic model and environmental obstacles are considered in our study, making the challenge of the multi-machine priority obstacle avoidance strategy focus on specifying the conflict and coordinating the move. Taking two robots with higher priority, AGV1 and lower priority, AGV2, as an example, we investigated multiple conflict types in Table 2 and obtained the following collision conflict judgments and solutions:
case | Movement Status | Angle Relationship | Potential conflict or not | Conflict resolution strategies |
1 | ![]() |
|α−β|≥90° | NO | —— |
2 | ![]() |
|α−β|≥90° | NO | —— |
3 | ![]() |
|α−β|<90° | YES | AGV1 passes first, AGV2 stops the movement |
4 | ![]() |
|α−β|≥90° | NO | —— |
5 | ![]() |
|α−β|<90° | YES | AGV1 passes first, AGV2 stops the movement |
6 | ![]() |
|α−β|<90° | YES | AGV1 passes first, AGV2 stops the movement |
Step 1: If the actual distance d12 of two robots is less than the desired obstacle avoidance distance de, the potential collision risk.
Step 2: Establish a local coordinate axis with the lower priority robot AGV2 as the center, calculate the angle α between the line of the two robots and the positive direction of the x-axis, and then calculate the relationship between the magnitude of α and the directional angle β of AGV1.
Step 3: Determine whether |α−β|< 90°; if this condition is satisfied, the risk of collision is extremely high. To ensure the safety of multi-robot movement, AGV2 with lower priority decelerates within a short time, according to Eq (22). The AGV1 with higher priority treats AGV2 as an unknown static obstacle and performs obstacle avoidance motion through DWA. When the distance relationship between the two robots is d12>de and |α−β|≥90°, AGV2 resumes motion.
In conjunction with the research presented in this study, most experiments show that the proposed prioritized obstacle avoidance strategy applies to most situations. This is mainly attributed to the fact that robots are defined as conflicting motions only when the above conditions are satisfied in terms of distance and angle relationships, and robots that are not in the conflict range perform the corresponding obstacle avoidance strategies based on our DWA.
{v(t+1)=v(t)−2amaxvΔtω(t+1)=ω(t)−2amaxωΔt | (22) |
We propose a multi-mobile robots obstacle avoidance strategy that guarantees global optimality and safety, which fuses the BAJPSA* algorithm for planning global paths with the DWA local obstacle avoidance algorithm that combines a prioritized obstacle avoidance strategy. The flow of the fusion algorithm is shown in Figure 13.
4) Simulation experiments
Simulation experiment environment: Windows 10 (64-bit), AMD Ryzen5-4600H with 3GHz, 16 GB memory, the simulation platform is MATLAB R2019a.
The relevant parameters of this paper are as follows: the sampling period Δt is 0.1 s; the expected node distance nds is 0.018 m; the robot's expected tracking distance d1 is 1.8 m; the predicted trajectory to the target point expected distance d2 is 1 m; the expected distance parameters l1 and l3 between the robot and the obstacle are 0.4 m and 0.7 m respectively; the maximum error l2 of the robot from the global path is 1 m; the expected obstacle avoidance distance le of the robot to dynamic obstacles is 1.5 m; the expected obstacle avoidance distance de between the multiple robots is 2 m.The robot model parameters are: maximum linear velocity 1m/s; maximum angular velocity 20rad/s; linear acceleration 0.2m/s2; angular acceleration 5rad/s2; linear velocity resolution 0.02m/s; angular velocity resolution 1rad/s2 and the evaluation function coefficients are: A=0.05,B=0.2,C=0.3,D=0; the forecast time period tf is 3.0 s.
To verify the effectiveness of our BAJPSA*, two sets of maps with scales of 30×30 and 100×100 were selected for simulation and compared with the A* and JPS algorithms. The experimental results and simulation data are shown in Figures 14 and15 and Table 3. The green grid depicts the nodes searched by the algorithm, the blue grid is the forced neighbor nodes of JPS and BAJPSA*, while the red path and the orange path in Figures 14 and 15(c) are the paths of our BAJPSA* forward and the reverse searches, respectively.
The green node area in Figures 14 and 15 and the number of OPEN and CLOSE list nodes in Table 3 show that our BAJPSA* dramatically reduces the number of extended nodes compared to the A* algorithm, and the improvement is particularly noticeable in a large-scale map of 100×100. There is a 92.5% reduction in the number of extended nodes and a 91.3% reduction in the running time of our BAJPSA* algorithm compared to A*, with a slight increase of 0.585 m in length. There is an advantage of our BAJPSA* over the JPS algorithm, mainly in terms of the search time, which benefits from the mechanism of bidirectional alternating search and the improvement of the heuristic function. We improve the search time of BAJPSA* by 50 % and reduce the number of expansion nodes by 60 % in the 30×30 scale map. Similarly, our BAJPSA* search time for paths is reduced by 79 %, and the number of expansion nodes is slightly improved by 30 % in the 100×100 large-scale map.
30×30 environment | 100×100 environment | |||||
Path parameters | A* | JPS | BAJPSA* | A* | JPS | BAJPSA* |
Length/m | 43.355 | 43.355 | 43.355 | 158.167 | 158.167 | 158.752 |
Run-times/s | 0.651 | 0.863 | 0.485 | 6.894 | 2.855 | 0.598 |
Number of path nodes |
34 | 24 | 27 | 131 | 55 | 89 |
Number of OPEN list |
253 | 130 | 51 | 2509 | 271 | 187 |
Number of CLOSE list | 394 | 98 | 32 | 6240 | 236 | 121 |
To determine the effect of our improved algorithm, the path with the global path length of 17.3616 m marked in black is obtained based on our BAJPSA* algorithm, as shown in Figure 16. The robot with the two-wheel differential motion model is built for trajectory tracking based on our improved DWA, and the test results in Table 4 and the robot angle variation and path error plots in Figure 17 show the experimental data of three robots with different parameters in detail. The specific exploratory analysis is as follows:
In Path_1, we do not consider the robot's new Path(v,ω) evaluation function, such that the weight D=0. The final path length of the robot travels is 17.7138 m, the robot deviates from the global path by 0.2255 m on average for each movement, and the robot spends 50.8668 s moving from the starting point (1.5, 2.5) to the endpoint (16.5, 10.5).
In Path_2, we set D = 0.2, and the final path length of the robot is 17.3676 m, while the average deviation of the robot from the global path is 0.0577 m. Compared with the conventional DWA of Path_1, we sacrifice some efficiency of the algorithm as we simultaneously consider the four Equations indicators (17-20). Moreover, it is evident from the experimental results that the actual robot motion of Path_2 is basically along the global path, except near the obstacles.
In Path_3, we further increase the optimization effect of the Path(v,ω) indicator, and Path_3 is obtained by setting D = 0.4, which is improved slightly in terms of the path length and path offset indicators compared with Path_2.
Path | Path length/m | Tracking error/m | Search time/s |
Path_1 | 17.7138 | 0.2255 | 50.8668 |
Path_2 | 17.3676 | 0.0577 | 55.8523 |
Path_3 | 17.3536 | 0.0551 | 61.3893 |
To test the effectiveness of our proposed dynamic obstacle avoidance strategy, the following three sets of experiments were conducted for three collision types: frontal, rear-end, and lateral, as shown in Figures 18-21, respectively. The robot model parameters are the same as those in Section 4.2.1.
First, we conducted a frontal collision experiment, where the movement speed of dynamic obstacles was set to 0.2 and 0.4m/s in Figures 18(a), (b), respectively (in Section 3.4.2, we analyzed the actual speed of the robot as vr=vs∩vd∩va, considering the limited search space of the robot, the speed of obstacles in this experiment was less than 0.5 times the maximum speed of the robot). The results show that the robot has a large recognition area for moving obstacles, can avoid them with conflicting frontal motion, and have a specific safety distance. Figure 18(b) shows the environment with high-speed moving obstacles, and the path length of the robot after completing obstacle avoidance is 9.8702 m; it takes 15.62 s to move from the starting point (3.5, 1.5) to the endpoint (3.5, 10.5). The faster the speed of the obstacle movement, the higher the requirement for the robot's obstacle avoidance performance. Thus, the path length of the robot's passage compared to the low-speed moving obstacle in Figure 18(a) increases by 1.9845 m, and the movement duration increases by 1.38 s.
Next, we performed a rear-end collision experiment with an obstacle moving at 0.1m/s. The test results showed that our robot detected a slow-moving impediment in front and successfully avoided it. The final travel length of the robot was 13.4653 m, and it took 19.82 s.
Finally, we set up obstacles with different motion directions and speeds for side collision experiments for three tests, respectively, and added traditional DWA for comparison to reflect the advantages of our obstacle avoidance strategy. In the first group, the speed of obstacle movement is 0.25m/s, and in the second and third groups is 0.5m/s. The experimental effect is shown in Figures 19-21. In the first collision experiment shown in Figure 19, the moving speed of the obstacles we set is relatively slow, such that both the traditional DWA and our DWA can successfully avoid obstacles. The travel path lengths of the conventional and our robot are 7.4440 and 7.6449 m, respectively. Keeping the direction of movement of the obstacle, we increased the movement speed of the obstacle to 0.5m/s and carried out the second collision experiment shown in Figure 20. The travel path lengths of the conventional robot and our robot are 8.1640 m and 7.1840 m, respectively. The experimental results in Figure 21, show that the robot collides with the obstacle under the traditional DWA obstacle avoidance strategy. Our robot can determine conflicting obstacles and slow down and avoid such obstacles that otherwise be avoided successfully. Compared with the first experiment, although our robot has an additional waiting time of 1.68 s, the length of the traveled path is reduced by 0.4609 m, and the safety is guaranteed. For the third test, we changed the direction of movement of this obstacle. From the experimental results of Figure 21, the traditional DWA has the optimal local problem of following the obstacle movement described in Figure 21(a), and the conflict is resolved only when the obstacle stops. The final path length of the robot traveled was 14.8600 m and took 19.16 s. Compared with our robot, the driving distance increases by 1.09 times, and the obstacle avoidance time also increases by 4.17 s.
The four experiments with three robots, all shown in Figures 22-28, were performed in four different environments: a globally known static environment, one with unknown static obstacles, one with unknown static and dynamic obstacles, and a dynamic environment with a large-scale sea area, respectively. The effectiveness and stability of our proposed fusion algorithm of the BAJPSA* algorithm, DWA dynamic obstacle avoidance strategy, and multi-robot priority obstacle avoidance strategy have been verified.
The simulation results for the environment with known global obstacle information are shown in Figures 22-23, where the blue, pink, and red lines are the path situations of the three robots, AGV1, AGV2 and AGV3, respectively; the black grid depicts the static obstacle, for which the robot has a priori information. Figure 22 shows the real-time change of the robot during the planning process. The linear velocity and angular variation curves of the robot are shown in Figures 23(a) and (b), respectively; Figure 23(c) shows the offset of the robot's motion path compared with the global path, where the starting and ending points of AGV1 are (14.5, 7.5) and (1.5, 10.5), respectively; the starting and ending points of AGV2 are (5.5, 6.5) and (13.5, 7.5), respectively; the starting and ending points of AGV3 are (5.5, 6.5) and (13.5, 10.5), respectively.
Figures 22 and 23(a) show that AGV3 decelerates at the 50th motion control node when it detects a collision risk with AGV2, and completely stops at the 80th control node. Then, AGV2 starts decelerating from the 85th control node, until the 95th control node completely stops, as there is a collision conflict with AGV1. AGV1 has the highest priority, such that AGV2 and AGV3 in the stopped state are considered static obstacles, and AGV1 performs reasonable obstacle avoidance based on DWA. Furthermore, Figure 23(c) shows that the actual path of AGV1 movement deviates most from the global path. The collision risk between AGV2 and AGV1 is released, and AGV2 starts to move at the 130th control node. At this time, AGV3 still maintains collision conflict with AGV2. Thus, AGV3 resumes motion at the 160th control node.
Figure 23 shows that AGV3 has the lowest priority, resulting in the most prolonged maintenance state of its stopped motion and less significant changes in the motion direction and path deviation. The additional obstacle avoidance performed by AGV1 and AGV2 for the lower priority robots produced a more noticeable motion angle and global path deviation. However, the whole motion was relatively smooth. The multi-robot motion experiments we conducted took 130.91 s. The travel distances of AGV1, AGV2 and AGV3 were 13.54 m, 8.89 m and 9.63 m, respectively, and the average error of motion deviation from the global path for each motion moment (0.1 s) was 0.3919 m, 0.2056 m, and 0.0120 m, respectively.
The multi-robot global path based on the BAJPSA* algorithm in the global static environment is shown in Figure 24(a), where the starting and ending points of AGV1 are (3.5, 2.5) and (12.5, 14.5), respectively; the starting and ending points of AGV2 are (2.5, 15.5) and (12.5, 2.5), respectively; the starting and ending points of AGV3 are (9.5, 15.5) and (7.5, 1.5), respectively. Then, the randomly distributed unknown static obstacles (the red grid) were increased to conduct the following simulation experiments.
The results shown in Figures 24 and 25 indicate that our multi-robot systems can avoid random static obstacles successfully when moving along the global path in an environment that includes unknown factors. The risk of collision between multi-robot systems is solved successfully with an improved prioritization strategy. What we know from Figures 24(b) and 25(a) is that when the higher priority AGV2 collides with lower priority AGV1, AGV2 decelerates from the 140th control node, and the velocity reaches zero at the 160th control node. The conflict is released once AGV1 moves away from AGV2 and AGV2 resumes motion at the 180th control node and successfully avoids random obstacles.
The variation of the motion speed, travel direction, and global path offset for each robot is relatively significant, as the obstacles of unknown disturbance were considered compared to those in Section 4.3.1. In conclusion, the travel distances for AGV1, AGV2 and AGV3 are 16.36, 19.46 and 14.57 m, respectively. The average values of global path offset for each robot movement are 0.5353, 0.7853, and 0.4287 m, respectively. The robot movement took 160.68 s program running time.
There are several dynamic obstacles we added to the unknown static obstacles in Section 4.3.2 to further test the applicability of single-robot dynamic obstacle avoidance strategy and multi-robot prioritized obstacle avoidance strategy in the given scenario, where the starting and ending points of AGV1 are (1.5, 7.5) and (15.5, 12.5), respectively; the starting and ending points of AGV2 are (15.5, 8.5) and (1.5, 12.5), respectively; the starting and ending points of AGV3 are (13.5, 14.5) and (3.5, 6.5), respectively. The yellow squares shown in Figure 26 are dynamic obstacles without a priori knowledge for the robots, and the red enclosures are the recognizable regions that the robots are assigned. The study of dynamic obstacle motion speed and robot recognition area in Section 3.4.2 indicates that they are positively proportional. Therefore, we set the movement speed for the three dynamic obstacles of the small, medium, and large sizes as 0.5,0.39,and 0.30m/s , respectively; the radius of the recognition area given is 0.55, 0.40 and 0.35 m, respectively; the preset motion path is shown in the last figure of Figure 26.
As shown in Figure 26(b), three robots and three unknown dynamic obstacles are encountered at the center of the map. Figures 26(b) and 27(a) show that AGV1 and AGV2 detect dynamic obstacles in front of them, and cannot avoid them because of the limited environment and rapid movement of the obstacles. According to the dynamic obstacle avoidance rules for motion conflicts in Section 3.4.2, the deceleration of both AGV1 and AGV2 starts from around the 125th control node and stops entirely at the 150th control node. After the dynamic obstacle leaves, both AGV1 and AGV2 resume motion around the 185th control node. Subsequently, the collision risk with AGV1 is detected by AGV3 and AGV2 at the 170th and 200th control nodes, respectively. According to Section 3.4.3, AGV3 and AGV2 decelerate and wait until the conflict is removed in the multi-robot priority obstacle avoidance strategy.
This experimental simulation result demonstrates the effectiveness of our improved single-robot dynamic obstacle avoidance strategy combined with a multi-robot priority avoidance strategy in an environment with random static and unknown dynamic obstacles. As we find from Figures 27(b) and (c), there is obstacle avoidance in AGV1, resulting in a large angle and path offset. The motion distances of the robot are 14.66, 14.11 and 12.38 m, respectively; the errors of the robot's single-step motion offsetting the global path are 0.0288, 0.0361 and 0.0114 m, respectively; the total running time of the algorithm is 292.0472 s.
The multi-robot global path based on the BAJPSA* algorithm in a large-scale sea environment with an accuracy of 10 m is shown in Figure 28(a), where the starting and ending points of AGV1 are (7.5, 55.5) and (59.5, 94.5), respectively; the starting and ending points of AGV2 are (55.5, 55.5) and (84.5, 29.5), respectively; the starting and ending points of AGV3 and endpoints are (33.5, 10.5) and (76.5, 58.5), respectively. The BAJPSA* algorithm takes 0.3328, 0.0972 and 0.0975 s to plan the global path for the three robots; the numbers of extended nodes are 41, 22 and 31, respectively. The global path lengths are 531.481, 323.201 and 636.734 m, respectively. Then, unknown static and dynamic obstacles with random distribution are added, and the following simulation experiments are conducted.
Figure 28 shows that our fusion algorithm is equally effective in the large-scale map environment. As indicated in Figure 28(b-4), AGV3 encounters a dynamic obstacle traveling in the same direction. It overtakes left side to avoid the obstacle traveling ahead, following the dynamic obstacle avoidance rules of the single robot. In Figure 28(b-6), AGV1 successfully avoids the random static obstacle and traverses the map's narrow area. In Figure 28(b-7), AGV2 and AGV3 successfully avoid the random static obstacles. Figure 28(b-9) shows the final travel paths of the three robots. The traces of the robots are smooth and fit the global path.
The experimental simulation results demonstrate the effectiveness of our multi-robot obstacle avoidance strategy with BAJPSA* fusion improved DWA in a large-scale environment. The motion distances of AGV1, AGV2 and AGV3 are 693 m, 388.5 m and 667 m, respectively, and the average errors of motion deviation from the global path at each motion moment (0.1 s) are 4.052 m, 0.895 m and 0.755 m, respectively.
To solve the path planning problem of distributed multiple robots in dynamic environments, we propose a BAJPSA* algorithm fused with adaptive DWA, performing in two stages.
In the first stage, we plan the globally optimal path for each robot by the BAJPSA* algorithm, with simulation results demonstrating the effectiveness of BAJPSA* in global path planning. In the second stage, we perform the local path planning. The adaptive navigation strategy and path deviation evaluation function are proposed to improve the path tracking capability of the traditional DWA. Next, we categorize and discuss multiple unknown static and dynamic obstacle environments with motion conflict scenarios, and propose dynamic obstacle avoidance rules for the single robot. Then, we extend the single-robot to distributed multi-robot with decision rights, discuss multiple classes of motion conflict situations, and achieve cooperative multi-robot avoidance by fusing the prioritizing avoidance rules. The simulation results demonstrate the effectiveness of this algorithm for multi-robot path planning in unknown dynamic environments.
In this study, unknown static, as well as highly dynamic environments are the environments we focus on, and more complex factors (non-flat terrain, large-scale robots, etc.) will be gradually considered in future work. We can also test this algorithm in a robot platform and further on the multi-robot cooperative efficiency problem.
This research was funded by the National Key R & D Program of China, grant number 61163051.
The authors declare there is no conflict of interest.
[1] | Béné C, Barange M, Subasinghe R, et al. (2015) Feeding 9 billion by 2050–Putting fish back on the menu. Food Sec 7: 261-274. https://doi.org/10.1007/s12571-015-0427-z |
[2] | Hall SJ, Delaporte A, Phillips MJ, et al. (2011) Blue frontiers: Managing the environmental costs of aquaculture. Penang, Malaysia: The WorldFish Center. |
[3] | Department of Fisheries, Ministry of Fisheries, Animal Husbandry & Dairying, Government of IndiaHandbook of fisheries statistics 2023 (2023). |
[4] | Mishra SS, Rakesh D, Dhiman M, et al. (2017) Present status of fish disease management in freshwater aquaculture in India: state-of-the-art-review. J Aquac Fisheries 1: 003. http://doi.org/10.24966/AAF-5523/100003 |
[5] | Lai XH, Wang SY, Edebro H, et al. (2003) Francisella strains express hemolysins of distinct characteristics. FEMS Microbiol Lett 224: 91-95. https://doi.org/10.1016/S0378-1097(03)00431-2 |
[6] | Sun J, Zhang X, Gao X, et al. (2016) Characterization of virulence properties of Aeromonas veronii isolated from diseased Gibel Carp (Carassius gibelio). Int J Mol Sci 17: 496. https://doi.org/10.3390/ijms17040496 |
[7] | Sambrook J, Russel DW (2001) Molecular cloning: A laboratory manual, third edition. CSH Laboratory Press, Cold Spring Harbor . |
[8] | Behera BK, Paria P, Das A, et al. (2021) Molecular identification and pathogenicity study of virulent Citrobacter freundii associated with mortality of farmed Labeo rohita (Hamilton 1822), in India. Aquaculture 547: 737437. https://doi.org/10.1016/j.aquaculture.2021.737437 |
[9] | Saitou N, Nei M (1987) The neighbor-joining method: A new method for reconstructing phylogenetic trees. Mol Biol Evol 4: 406-425. https://doi.org/10.1093/oxfordjournals.molbev.a040454 |
[10] | Kumar S, Stecher G, Li M, et al. (2018) MEGA X: Molecular evolutionary genetics analysis across computing platforms. Mol Biol Evol 35: 1547-1549. https://doi.org/10.1093/molbev/msy096 |
[11] | Tamura K, Nei M, Kumar S (2004) Prospects for inferring very large phylogenies by using the neighbor-joining method. Proc Natl Acad Sci 101: 11030-11035. https://doi.org/10.1073/pnas.0404206101 |
[12] | Felsenstein J (1985) Confidence limits on phylogenies: An approach using the bootstrap. Evolution 39: 783-791. https://doi.org/10.2307/2408678 |
[13] | Martino ME, Fasolato L, Montemurro F, et al. (2011) Determination of microbial diversity of Aeromonas strains on the basis of multilocus sequence typing, phenotype, and presence of putative virulence genes. Appl Environ Microbiol 77: 4986-5000. https://doi.org/10.1128/aem.00708-11 |
[14] | Persson S, Al-Shuweli S, Yapici S, et al. (2015) Identification of clinical Aeromonas species by rpoB and gyrB sequencing and development of a multiplex PCR method for detection of Aeromonas hydrophila, A. caviae, A. veronii, and A. media. J. Clin Microbiol 53: 653-656. https://doi.org/10.1128/jcm.01963-14 |
[15] | Wirth T, Falush D, Lan R, et al. (2006) Sex and virulence in Escherichia coli: An evolutionary perspective. Mol Microbiol 60: 1136-1151. https://doi.org/10.1111/j.1365-2958.2006.05172.x |
[16] | Guo C, Yang X, Wu Y, et al. (2015) MLST-based inference of genetic diversity and population structure of clinical Klebsiella pneumoniae, China. Sci Rep 5: 7612. https://doi.org/10.1038/srep07612 |
[17] | Enright MC, Day NPJ, Davies CE, et al. (2000) Multilocus sequence typing for characterization of methicillin-resistant and methicillin-susceptible clones of Staphylococcus aureus. J Clin Microbiol 38: 1008-1015. https://doi.org/10.1128/jcm.38.3.1008-1015.2000 |
[18] | Curran B, Jonas D, Grundmann H, et al. (2004) Development of a multilocus sequence typing scheme for the opportunistic pathogen Pseudomonas aeruginosa. J Clin Microbiol 42: 5644-5649. https://doi.org/10.1128/jcm.42.12.5644-5649.2004 |
[19] | Ruiz-Garbajosa P, Bonten MJM, Robinson DA, et al. (2006) Multilocus sequence typing scheme for Enterococcus faecalis reveals hospital-adapted genetic complexes in a background of high rates of recombination. J Clin Microbiol 44: 2220-2228. https://doi.org/10.1128/jcm.02596-05 |
[20] | Kong RYC, Lee SKY, Law SHW, et al. (2002) Rapid detection of six types of bacterial pathogens in marine waters by multiplex PCR. Water Res 36: 2802-2812. https://doi.org/10.1016/s0043-1354(01)00503-6 |
[21] | Yang ZS, Fang H (2003) Human and animal pathogenic bacteriology. Shijiazhuan, China: Hebei Science and Technology Press 1550-1610. |
[22] | Nawaz M, Khan SA, Khan AA, et al. (2010) Detection and characterization of virulence genes and integrons in Aeromonas veronii isolated from catfish. Food Microbiol 27: 327-331. https://doi.org/10.1016/j.fm.2009.11.007 |
[23] | Sen K, Rodgers M (2004) Distribution of six virulence factors in Aeromonas species isolated from US drinking water utilities: A PCR identification. J. Appl Microbiol 97: 1077-1086. https://doi.org/10.1111/j.1365-2672.2004.02398.x |
[24] | Wong CYF, Heuzenroeder MW, Flower RLP (1998) Inactivation of two haemolytic toxin genes in Aeromonas hydrophila attenuates virulence in a suckling mouse model. Microbiology 144: 291-298. https://doi.org/10.1099/00221287-144-2-291 |
[25] | Kerényi M, Allison HE, Bátai I, et al. (2005) Occurrence of hlyA and sheA genes in extraintestinal Escherichia coli strains. J Clin Microbiol 43: 2965-2968. https://doi.org/10.1128/jcm.43.6.2965-2968.2005 |
[26] | Chapman TA, Wu XY, Barchia I, et al. (2006) Comparison of virulence gene profiles of Escherichia coli strains isolated from healthy and diarrheic swine. Appl Environ Microbiol 72: 4782-4795. https://doi.org/10.1128/aem.02885-05 |
[27] | Paton AW, Paton JC (1998) Detection and characterization of Shiga toxigenic Escherichia coli by using multiplex PCR assays for stx 1, stx 2, eaeA, enterohemorrhagic E. coli hlyA, rfb O111, and rfb O157. J Clin Microbiol 36: 598-602. https://doi.org/10.1128/jcm.36.2.598-602.1998 |
[28] | Russo TA, Olson R, MacDonald U, et al. (2014) Aerobactin mediates virulence and accounts for increased siderophore production under iron-limiting conditions by hypervirulent (hypermucoviscous) Klebsiella pneumoniae. Infect Immun 82: 2356-2367. https://doi.org/10.1128/iai.01667-13 |
[29] | Alcántar-Curiel MD, Blackburn D, Saldaña Z, et al. (2013) Multi-functional analysis of Klebsiella pneumoniae fimbrial types in adherence and biofilm formation. Virulence 4: 129-138. https://doi.org/10.4161/viru.22974 |
[30] | Zhang S, Yang G, Ye Q, et al. (2018) Phenotypic and genotypic characterization of Klebsiella pneumoniae isolated from retail foods in China. Front Microbiol 9: 289. https://doi.org/10.3389/fmicb.2018.00289 |
[31] | Fang CT, Chuang YP, Shun CT, et al. (2004) A novel virulence gene in Klebsiella pneumoniae strains causing primary liver abscess and septic metastatic complications. J Exp Med 199: 697-705. https://doi.org/10.1084/jem.20030857 |
[32] | Nadasy KA, Domiati-Saad R, Tribble MA (2007) Invasive Klebsiella pneumoniae syndrome in North America. Clin Infect Dis 45: e25-e28. https://doi.org/10.1086/519424 |
[33] | Jarraud S, Mougel C, Thioulouse J, et al. (2002) Relationships between Staphylococcus aureus genetic background, virulence factors, agr groups (alleles), and human disease. Infect Immun 70: 631-641. https://doi.org/10.1128/iai.70.2.631-641.2002 |
[34] | Li X, Huang T, Xu K, et al. (2019) Molecular characteristics and virulence gene profiles of Staphylococcus aureus isolates in Hainan, China. BMC Infect Dis 19: 873. https://doi.org/10.1186/s12879-019-4547-5 |
[35] | Mehrotra M, Wang G, Johnson WM (2000) Multiplex PCR for detection of genes for Staphylococcus aureus enterotoxins, exfoliative toxins, toxic shock syndrome toxin 1, and methicillin resistance. J Clin Microbiol 38: 1032-1035. https://doi.org/10.1128/jcm.38.3.1032-1035.2000 |
[36] | Fazeli N, Momtaz H (2014) Virulence gene profiles of multidrug-resistant Pseudomonas aeruginosa isolated from Iranian hospital infections. Iran Red Crescent Med J 16: e15722. https://doi.org/10.5812/ircmj.15722 |
[37] | Xu J, Moore JE, Murphy PG, et al. (2004) Early detection of Pseudomonas aeruginosa – comparison of conventional versus molecular (PCR) detection directly from adult patients with cystic fibrosis (CF). Ann Clin Microbiol Antimicrob 3: 21. https://doi.org/10.1186/1476-0711-3-21 |
[38] | Matar GM, Ramlawi F, Hijazi N, et al. (2002) Transcription levels of Pseudomonas aeruginosa exotoxin A gene and severity of symptoms in patients with otitis externa. Curr Microbiol 45: 350-354. https://doi.org/10.1007/s00284-002-3703-z |
[39] | Finnan S, Morrissey JP, O'gara F, et al. (2004) Genome diversity of Pseudomonas aeruginosa isolates from cystic fibrosis patients and the hospital environment. J Clin Microbiol 42: 5783-5792. https://doi.org/10.1128/jcm.42.12.5783-5792.2004 |
[40] | Vankerckhoven V, Van Autgaerden T, Vael C, et al. (2004) Development of a multiplex PCR for the detection of asa1, gelE, cylA, esp, and hyl genes in enterococci and survey for virulence determinants among European hospital isolates of Enterococcus faecium. J Clin Microbiol 42: 4473-4479. https://doi.org/10.1128/jcm.42.10.4473-4479.2004 |
[41] | Creti R, Imperi M, Bertuccini L, et al. (2004) Survey for virulence determinants among Enterococcus faecalis isolated from different sources. J Clin Microbiol 53: 13-20. https://doi.org/10.1099/jmm.0.05353-0 |
[42] | Maheshwari DG, Shaikh NK (2016) An overview on-toxicity testing method. Int J Pharm Technol 8: 3834-3849. |
[43] | Luna LG (1968) Manual of histologic staining methods of the Armed Forces Institute of Pathology. New York: Blakiston Division, McGraw-Hill. |
[44] | Sherwani RAK, Shakeel H, Awan WB, et al. (2021) Analysis of COVID-19 data using neutrosophic Kruskal Wallis H test. BMC Med Res Methodol 21: 215. https://doi.org/10.1186/s12874-021-01410-x |
[45] | Snieszko SF (1972) Nutritional fish diseases. Fish Nutr : 403-437. |
[46] | Martin-Carnahan A, Joseph SW (2005) Family I. Aeromonadaceae. Bergey's manual of systematic bacteriology 2: 556-587. https://doi.org/10.1007/0-387-28022-7_12 |
[47] | Beaz-Hidalgo R, Figueras MJ (2013) Aeromonas spp. whole genomes and virulence factors implicated in fish disease. J Fish Dis 36: 371-388. https://doi.org/10.1111/jfd.12025 |
[48] | Samayanpaulraj V, Sivaramapillai M, Palani SN, et al. (2020) Identification and characterization of virulent Aeromonas hydrophila Ah17 from infected Channa striata in river Cauvery and in vitro evaluation of shrimp chitosan. Food Sci Nutr 8: 1272-1283. https://doi.org/10.1002/fsn3.1416 |
[49] | Das A, Acharya S, Behera BK, et al. (2018) Isolation, identification and characterization of Klebsiella pneumoniae from infected farmed Indian Major Carp Labeo rohita (Hamilton 1822) in West Bengal, India. Aquaculture 482: 111-116. https://doi.org/10.1016/j.aquaculture.2017.08.037 |
[50] | Visnuvinayagam S, Joseph TC, Murugadas V, et al. (2015) Status on methicillin resistant and multiple drug resistant Staphylococcus aureus in fishes of Cochin and Mumbai coast, India. J Environ Biol 36: 571-575. |
[51] | Vaiyapuri M, Joseph TC, Rao BM, et al. (2019) Methicillin-resistant Staphylococcus aureus in seafood: Prevalence, laboratory detection, clonal nature, and control in seafood chain. J Food Sci 84: 3341-3351. https://doi.org/10.1111/1750-3841.14915 |
[52] | Eissa NME, El-Ghiet EA, Shaheen AA, et al. (2010) Characterization of Pseudomonas species isolated from tilapia “Oreochromis niloticus” in Qaroun and Wadi-El-Rayan lakes, Egypt. Global Vet 5: 116-121. http://dx.doi.org/10.13140/2.1.5002.4961 |
[53] | Paria P, Behera BK, Mohapatra PKD, et al. (2021) Virulence factor genes and comparative pathogenicity study of tdh, trh and tlh positive Vibrio parahaemolyticus strains isolated from Whiteleg shrimp, Litopenaeus vannamei (Boone, 1931) in India. Infect Genet Evol 95: 105083. https://doi.org/10.1016/j.meegid.2021.105083 |
[54] | Zhang XJ, Qin GM, Bing XW, et al. (2011) Phenotypic and molecular characterization of Photobacterium damselae, a pathogen of the cultured tongue sole Cynoglossus semilaevis in China. N Z J Mar Freshwater Res 45: 1-13. https://doi.org/10.1080/00288330.2010.531745 |
[55] | Cole JR, Wang Q, Cardenas E, et al. (2009) The Ribosomal Database Project: Improved alignments and new tools for rRNA analysis. Nucleic Acids Res 37: D141-D145. https://doi.org/10.1093/nar/gkn879 |
[56] | Wang G, Clark CG, Liu C, et al. (2003) Detection and characterization of the hemolysin genes in Aeromonas hydrophila and Aeromonas sobria by multiplex PCR. J Clin Microbiol 41: 1048-1054. https://doi.org/10.1128/jcm.41.3.1048-1054.2003 |
[57] | Devi MS, Paria P, Kumar V, et al. (2022) Molecular identification and pathogenicity study of virulent Vibrio cholerae non O1/O139 serotype associated with mortality of farmed Labeo rohita (Hamilton, 1822), in India. Aquaculture 547: 737529. https://doi.org/10.1016/j.aquaculture.2021.737529 |
[58] | Wang X, Wang Q, Xiao J, et al. (2010) Hemolysin EthA in Edwardsiella tarda is essential for fish invasion in vivo and in vitro and regulated by two-component system EsrA–EsrB and nucleoid protein HhaEt. Fish Shellfish Immun 29: 1082-1091. https://doi.org/10.1016/j.fsi.2010.08.025 |
[59] | Preena PG, Dharmaratnam A, Swaminathan TR (2022) A peek into mass mortality caused by antimicrobial resistant Edwardsiella tarda in goldfish, Carassius auratus in Kerala. Biologia 77: 1161-1171. https://doi.org/10.1007/s11756-022-01007-9 |
[60] | Locke JB, Colvin KM, Varki N, et al. (2007) Streptococcus iniae β-hemolysin streptolysin S is a virulence factor in fish infection. Dis Aquat Organ 76: 17-26. https://doi.org/10.3354/dao076017 |
[61] | Batt CA (2016) Virulence. Reference module in food science. Amsterdam, The Netherlands: Elsevier. https://doi.org/10.1016/B978-0-08-100596-5.03453-3 |
[62] | Remya PA, Shanthi M, Sekar U (2019) Characterisation of virulence genes associated with pathogenicity in Klebsiella pneumoniae. Indian J Med Microbiol 37: 210-218. https://doi.org/10.4103/ijmm.ijmm_19_157 |
[63] | Sivaraman GK, Rajan V, Vijayan A, et al. (2021) Antibiotic resistance profiles and molecular characteristics of extended-spectrum beta-lactamase (ESBL)-producing Escherichia coli and Klebsiella pneumoniae isolated from shrimp aquaculture farms in Kerala, India. Front Microbiol 12: 622891. https://doi.org/10.3389/fmicb.2021.622891 |
[64] | Regué M, Hita B, Piqué N, et al. (2004) A gene, uge, is essential for Klebsiella pneumoniae virulence. Infect Immun 72: 54-61. https://doi.org/10.1128/iai.72.1.54-61.2004 |
[65] | Abrami L, Fivaz M, Glauser PE, et al. (2003) Sensitivity of polarized epithelial cells to the pore-forming toxin aerolysin. Infect Immun 71: 739-746. https://doi.org/10.1128/IAI.71.2.739-746.2003 |
[66] | Nhinh DT, Le DV, Van KV, et al. (2021) Prevalence, virulence gene distribution and alarming the multidrug resistance of Aeromonas hydrophila associated with disease outbreaks in freshwater aquaculture. Antibiotics 10: 532. https://doi.org/10.3390/antibiotics10050532 |
[67] | Muduli C, Tripathi G, Paniprasad K, et al. (2021) Virulence potential of Aeromonas hydrophila isolated from apparently healthy freshwater food fish. Biologia 76: 1005-1015. https://doi.org/10.2478/s11756-020-00639-z |
[68] | Chopra AK, Xu XJ, Ribardo D, et al. (2000) The cytotoxic enterotoxin of Aeromonas hydrophila induces proinflammatory cytokine production and activates arachidonic acid metabolism in macrophages. Infect Immun 68: 2808-2818. https://doi.org/10.1128/iai.68.5.2808-2818.2000 |
[69] | Ribardo DA, Kuhl KR, Boldogh I, et al. (2002) Early cell signaling by the cytotoxic enterotoxin of Aeromonas hydrophila in macrophages. Microb Pathog 32: 149-163. https://doi.org/10.1006/mpat.2001.0490 |
[70] | Hayati HR, Hassan MD, Ong BL, et al. (2015) Virulence genes detection of Aeromonas hydrophila originated from diseased freshwater fishes. Adv Environ Biol 9: 22-26. |
[71] | Tomás JM (2012) The main Aeromonas pathogenic factors. ISRN Microbiol 2012: 256261. https://doi.org/10.5402/2012/256261 |
[72] | Ristow LC, Welch RA (2016) Hemolysin of uropathogenic Escherichia coli: A cloak or a dagger?. BBA-Biomembranes 1858: 538-545. https://doi.org/10.1016/j.bbamem.2015.08.015 |
[73] | Chuang YC, Chiou SF, Su JH, et al. (1997) Molecular analysis and expression of the extracellular lipase of Aeromonas hydrophila MCC-2. Microbiology 143: 803-812. https://doi.org/10.1099/00221287-143-3-803 |
[74] | Lee KK, Ellis AE (1990) Glycerophospholipid: Cholesterol acyltransferase complexed with lipopolysaccharide (LPS) is a major lethal exotoxin and cytolysin of Aeromonas salmonicida: LPS stabilizes and enhances toxicity of the enzyme. J Bacteriol 172: 5382-5393. https://doi.org/10.1128/jb.172.9.5382-5393.1990 |
[75] | Pemberton JM, Kidd SP, Schmidt R (1997) Secreted enzymes of Aeromonas. FEMS Microbiol Lett 152: 1-10. https://doi.org/10.1111/j.1574-6968.1997.tb10401.x |
[76] | Nawaz M, Khan SA, Khan AA, et al. (2010) Detection and characterization of virulence genes and integrons in Aeromonas veronii isolated from catfish. Food Microbiol 27: 327-331. https://doi.org/10.1016/j.fm.2009.11.007 |
[77] | Chen F, Sun J, Han Z, et al. (2019) Isolation, identification and characteristics of Aeromonas veronii from diseased crucian carp (Carassius auratus gibelio). Front Microbiol 10: 2742. https://doi.org/10.3389/fmicb.2019.02742 |
[78] | Kong C, Neoh HM, Nathan S (2016) Targeting Staphylococcus aureus toxins: A potential form of anti-virulence therapy. Toxins 8: 72. https://doi.org/10.3390/toxins8030072 |
[79] | Alagarsamy S, Thampuran N, Joseph TC (2010) Virulence genes, serobiotypes and antibiotic resistance profile of Escherichia coli strains isolated from aquaculture and other sources. Aquac Res 41: 1003-1014. https://doi.org/10.1111/j.1365-2109.2009.02384.x |
[80] | Bauer ME, Welch RA (1996) Characterization of an RTX toxin from enterohemorrhagic Escherichia coli O157: H7. Infect Immun 64: 167-175. https://doi.org/10.1128/iai.64.1.167-175.1996 |
[81] | Gentschev I, Dietrich G, Goebel W (2002) The E. coli α-hemolysin secretion system and its use in vaccine development. Trends Microbiol 10: 39-45. https://doi.org/10.1016/S0966-842X(01)02259-4 |
[82] | Welch RA, Dellinger EP, Minshew B, et al. (1981) Haemolysin contributes to virulence of extra-intestinal E. coli infections. Nature 294: 665-667. https://doi.org/10.1038/294665a0 |
[83] | Benz R (2020) RTX-toxins. Toxins 12: 359. https://doi.org/10.3390/toxins12060359 |
[84] | Alonso B, Fernández-Barat L, Di Domenico EG, et al. (2020) Characterization of the virulence of Pseudomonas aeruginosa strains causing ventilator-associated pneumonia. BMC Infect Dis 20: 909. https://doi.org/10.1186/s12879-020-05534-1 |
[85] | Algammal AM, Mabrok M, Sivaramasamy E, et al. (2020) Emerging MDR-Pseudomonas aeruginosa in fish commonly harbor oprL and toxA virulence genes and blaTEM, blaCTX-M, and tetA antibiotic-resistance genes. Sci Rep 10: 15961. https://doi.org/10.1038/s41598-020-72264-4 |
[86] | Vareechon C, Zmina SE, Karmakar M, et al. (2017) Pseudomonas aeruginosa effector ExoS inhibits ROS production in human neutrophils. Cell Host Microbe 21: 611-618. https://doi.org/10.1016/j.chom.2017.04.001 |
[87] | Kaminski A, Gupta KH, Goldufsky JW, et al. (2018) Pseudomonas aeruginosa ExoS induces intrinsic apoptosis in target host cells in a manner that is dependent on its GAP domain activity. Sci Rep 8: 14047. https://doi.org/10.1038/s41598-018-32491-2 |
[88] | Rust L, Pesci EC, Iglewski BH (1996) Analysis of the Pseudomonas aeruginosa elastase (lasB) regulatory region. J Bacteriol 178: 1134-1140. https://doi.org/10.1128/jb.178.4.1134-1140.1996 |
[89] | Todar K Bacterial Protein Toxins In: Todar's Online textbook of bacteriology (2012). Available from: http://textbookofbacteriology.net/ |
[90] | Nowroozi J, Sepahi AA, Rashnonejad A (2012) Pyocyanine biosynthetic genes in clinical and environmental isolates of Pseudomonas aeruginosa and detection of pyocyanine's antimicrobial effects with or without colloidal silver nanoparticles. Cell J 14: 7-18. |
[91] | Lim A, De Vos D, Brauns M, et al. (1997) Molecular and immunological characterization of OprL, the 18 KDa outer-membrane peptidoglycan-associated lipoprotein (PAL) of Pseudomonas aeruginosa. Microbiology 143: 1709-1716. https://doi.org/10.1099/00221287-143-5-1709 |
[92] | Remans K, Vercammen K, Bodilis J, et al. (2010) Genome-wide analysis and literature-based survey of lipoproteins in Pseudomonas aeruginosa. Microbiology 156: 2597-2607. https://doi.org/10.1099/mic.0.040659-0 |
[93] | Bradbury RS, Roddam LF, Merritt A, et al. (2010) Virulence gene distribution in clinical, nosocomial and environmental isolates of Pseudomonas aeruginosa. J Med Microbiol 59: 881-890. https://doi.org/10.1099/jmm.0.018283-0 |
[94] | Cezairliyan B, Vinayavekhin N, Grenfell-Lee D, et al. (2013) Identification of Pseudomonas aeruginosa phenazines that kill Caenorhabditis elegans. PLoS Pathog 9: e1003101. https://doi.org/10.1371/journal.ppat.1003101 |
[95] | Aljebory IS (2018) PCR detection of some virulence genes of Pseudomonas aeruginosa in Kirkuk city, Iraq. J Pharm Sci Res 10: 1068-1071. |
[96] | Zheng JX, Wu Y, Lin ZW, et al. (2017) Characteristics of and virulence factors associated with biofilm formation in clinical Enterococcus faecalis isolates in China. Front Microbiol 8: 2338. https://doi.org/10.3389/fmicb.2017.02338 |
[97] | Anderson AC, Jonas D, Huber I, et al. (2016) Enterococcus faecalis from food, clinical specimens, and oral sites: prevalence of virulence factors in association with biofilm formation. Front Microbiol 6: 1534. https://doi.org/10.3389/fmicb.2015.01534 |
[98] | Kayaoglu G, Ørstavik D (2004) Virulence factors of Enterococcus faecalis: Relationship to endodontic disease. Crit Rev Oral Biol Med 15: 308-320. https://doi.org/10.1177/154411130401500506 |
[99] | Park SY, Kim KM, Lee JH, et al. (2007) Extracellular gelatinase of Enterococcus faecalis destroys a defense system in insect hemolymph and human serum. Infect Immun 75: 1861-1869. https://doi.org/10.1128/iai.01473-06 |
[100] | Thomas VC, Thurlow LR, Boyle D, et al. (2008) Regulation of autolysis-dependent extracellular DNA release by Enterococcus faecalis extracellular proteases influences biofilm development. J Bacteriol 190: 5690-5698. https://doi.org/10.1128/jb.00314-08 |
[101] | Mohamed JA, Murray BE (2005) Lack of correlation of gelatinase production and biofilm formation in a large collection of Enterococcus faecalis isolates. J Clin Microbiol 43: 5405-5407. https://doi.org/10.1128/JCM.43.10.5405-5407.2005 |
[102] | Behera BK, Paria P, Das A, et al. (2017) Molecular characterization and pathogenicity of a virulent Acinetobacter baumannii associated with mortality of farmed Indian Major Carp Labeo rohita (Hamilton 1822). Aquaculture 471: 157-162. https://doi.org/10.1016/j.aquaculture.2017.01.018 |
[103] | Abdelhamed H, Ibrahim I, Baumgartner W, et al. (2017) Characterization of histopathological and ultrastructural changes in channel catfish experimentally infected with virulent Aeromonas hydrophila. Front Microbiol 8: 1519. https://doi.org/10.3389/fmicb.2017.01519 |
![]() |
![]() |
1. | Liwei Yang, Ping Li, Song Qian, He Quan, Jinchao Miao, Mengqi Liu, Yanpei Hu, Erexidin Memetimin, Path Planning Technique for Mobile Robots: A Review, 2023, 11, 2075-1702, 980, 10.3390/machines11100980 | |
2. | Xiaohui Jia, Chunrui Tang, Xiangbo Zhang, Jinyue Liu, Research on dual robot collaboration method based on improved double ant colony algorithm, 2024, 51, 0143-991X, 424, 10.1108/IR-12-2023-0316 | |
3. | Jingze Zhong, Mengjie Zhang, Zonghai Chen, Jikai Wang, Dynamic Obstacle Avoidance for Mobile Robots Based on 2D Differential Euclidean Signed Distance Field Maps in Park Environment, 2024, 15, 2032-6653, 320, 10.3390/wevj15070320 | |
4. | Nour AbuJabal, Tamer Rabie, Mohammed Baziyad, Ibrahim Kamel, Khawla Almazrouei, Path Planning Techniques for Real-Time Multi-Robot Systems: A Systematic Review, 2024, 13, 2079-9292, 2239, 10.3390/electronics13122239 | |
5. | Xinyi He, Xiuping Han, Tengda Wei, Xiaodi Li, Tracking control of wheeled mobile robots via intermittent control, 2024, 21, 1551-0018, 3774, 10.3934/mbe.2024167 | |
6. | Min Tan, Zhenlai Liu, Peng Wang, 2024, Application and Optimization of Robot Systems in Industrial Production Line, 979-8-3503-7329-5, 701, 10.1109/ICICI62254.2024.00119 | |
7. | Nour Ayman Abujabal, Tamer Rabie, Ibrahim Kamel, 2023, Path Planning Techniques for Multi-robot Systems: A Systematic Review, 979-8-3503-8239-6, 1, 10.1109/IIT59782.2023.10366472 | |
8. | Shiliang Shao, Jin Zhang, Ting Wang, Achyut Shankar, Carsten Maple, Dynamic Obstacle-Avoidance Algorithm for Multi-Robot Flocking Based on Improved Artificial Potential Field, 2024, 70, 0098-3063, 4388, 10.1109/TCE.2023.3340327 | |
9. | Ge-An Wei, Jian-Qiang Zhang, Research on formation navigation of USV based on improved A* and DWA fusion algorithm, 2024, 24, 14727978, 3191, 10.3233/JCM-247567 | |
10. | Jiahui Wang, Jun Wang, Ruofan Chen, Kai Yang, Baolei Wu, Yongqiang Qi, Formation collaborative obstacle avoidance with multiple UGVs in restricted environments based on adaptive DWA, 2024, 09574174, 125870, 10.1016/j.eswa.2024.125870 | |
11. | Liu Yang, Mengru Yang, Annan Wang, A multi-robot conflict elimination path planning approach for dynamic environments, 2025, 36, 0957-0233, 016340, 10.1088/1361-6501/ad9d6d | |
12. | Nour AbuJabal, Mohammed Baziyad, Raouf Fareh, Brahim Brahmi, Tamer Rabie, Maamar Bettayeb, A Comprehensive Study of Recent Path-Planning Techniques in Dynamic Environments for Autonomous Robots, 2024, 24, 1424-8220, 8089, 10.3390/s24248089 | |
13. | Yong Han, Changyong Li, Zhaohui An, Based on the Integration of the Improved A* Algorithm with the Dynamic Window Approach for Multi-Robot Path Planning, 2025, 15, 2076-3417, 406, 10.3390/app15010406 | |
14. | Juan Dai, Yanzhang Jing, Zhong Su, Cui Zhu, Mobile robot path planning based on ORCA and improved DWA method, 2025, 0020-7179, 1, 10.1080/00207179.2025.2454905 | |
15. | Shanze Li, Kai Gao, Bo Gong, Qiannan Li, 2024, Multi-robot local path planning based on an improved dynamic window algorithm, 979-8-3503-8980-7, 160, 10.1109/IRAC63143.2024.10871558 | |
16. | Liben Yang, Yu Li, Jianwen Tian, Dong Wang, Fully autonomous anti-interference flight control of vertical takeoff and landing unmanned aerial vehicles in satellite positioning rejection environment, 2025, 36, 0957-0233, 036214, 10.1088/1361-6501/adb98a |
Algorithm 1. Bidirectional Alternating Jump Point Search A* (BAJPSA*) | |
1: | Initialize the grid map; |
2: | Open_1, Close_1; Open_2, Close_2 ← The jump points to be checked and the expanded jump points in the positive and reverse process, respectively; |
Put the starting point into Open_1; Put and the endpoint into Open_2; | |
3: | If Open_1 or Open_2 is an empty node; |
4: | Pathfinding failed; |
5: | Return; |
6: | else |
7: | While Positive node ~=Reverse node do |
8: | Calculate the f (n) value of all nodes in the Open_1 and Open_2 according to Equations (1) and (8); |
9: | Close_1 and Close_2 ← The node with the smallest f (n) value in the Open_1 and Open_2, respectively; |
10: | Positive node and Reverse node = the node with the smallest f (n) value, respectively; |
11: | If Positive node ==Reverse node; |
12: | The optimal path is obtained and the algorithm ends; |
13: | Break; |
14: | Else |
15: | While (Positive node & Reverse node)~= [] do |
16: | Search for jump points horizontally and vertically alone the direction from the parent node to the current node; |
17: | if Encounter obstacles or map edges; |
18: | Search for jump points diagonally alone the direction from the parent node to the current node; |
19: | Elseif Search for jump points; |
20: | Close_1 or Close_2 ← The searched jump points; |
21: | Else |
22: | Ignore the node, continue searching; |
23: | end |
24: | end |
25: | end |
26: | end |
27: | end |
Algorithm 2. Improved dynamic window approach (IDWA) | |
1: | Initializing (grid map, Robot, Evaluation_Factor); |
2: | Robot =[vmax,ωmax,amaxv,amaxω,VelocityResolution,RPMResolution] ; |
3: | Evaluation_Factor = [A; B; C; D]; |
4: | global_path ←Algorithm1 (BAJPSA*); |
5: | sensor_messages ← Robot; |
6: | if the trajectory without obstacles ← the Traditional DWA combined with the strategy in Section 3.4.2 to filter trajectories |
7: | while the local target location is not reached do ← the local target point information is obtained from equations (15-16) |
8: | Speed sampling of robot; |
9: | Simulate motion trajectories; |
10: | Use the improved evaluation function (17) to select the optimal trajectory; |
11: | Robot follows the optimal trajectory to move; |
12: | end |
13: | end |
Situation | Quantitative standard | Avoidance rule |
frontal collision | θ∈[315°,45°] | Avoid obstacles |
rear-end collision | ϕ∈[120°,240°] | Surpass or follow |
right collision | θ∈[45°,120°] | Avoid obstacles or decelerate to stop |
left collision | θ∈[240°,315°] | Avoid obstacles or decelerate to stop |
Note: where θ represents the movement direction of the obstacle towards the robot; ϕ represents the movement direction of the robot relative to the obstacle. |
case | Movement Status | Angle Relationship | Potential conflict or not | Conflict resolution strategies |
1 | ![]() |
|α−β|≥90° | NO | —— |
2 | ![]() |
|α−β|≥90° | NO | —— |
3 | ![]() |
|α−β|<90° | YES | AGV1 passes first, AGV2 stops the movement |
4 | ![]() |
|α−β|≥90° | NO | —— |
5 | ![]() |
|α−β|<90° | YES | AGV1 passes first, AGV2 stops the movement |
6 | ![]() |
|α−β|<90° | YES | AGV1 passes first, AGV2 stops the movement |
30×30 environment | 100×100 environment | |||||
Path parameters | A* | JPS | BAJPSA* | A* | JPS | BAJPSA* |
Length/m | 43.355 | 43.355 | 43.355 | 158.167 | 158.167 | 158.752 |
Run-times/s | 0.651 | 0.863 | 0.485 | 6.894 | 2.855 | 0.598 |
Number of path nodes |
34 | 24 | 27 | 131 | 55 | 89 |
Number of OPEN list |
253 | 130 | 51 | 2509 | 271 | 187 |
Number of CLOSE list | 394 | 98 | 32 | 6240 | 236 | 121 |
Path | Path length/m | Tracking error/m | Search time/s |
Path_1 | 17.7138 | 0.2255 | 50.8668 |
Path_2 | 17.3676 | 0.0577 | 55.8523 |
Path_3 | 17.3536 | 0.0551 | 61.3893 |
Algorithm 1. Bidirectional Alternating Jump Point Search A* (BAJPSA*) | |
1: | Initialize the grid map; |
2: | Open_1, Close_1; Open_2, Close_2 ← The jump points to be checked and the expanded jump points in the positive and reverse process, respectively; |
Put the starting point into Open_1; Put and the endpoint into Open_2; | |
3: | If Open_1 or Open_2 is an empty node; |
4: | Pathfinding failed; |
5: | Return; |
6: | else |
7: | While Positive node ~=Reverse node do |
8: | Calculate the f (n) value of all nodes in the Open_1 and Open_2 according to Equations (1) and (8); |
9: | Close_1 and Close_2 ← The node with the smallest f (n) value in the Open_1 and Open_2, respectively; |
10: | Positive node and Reverse node = the node with the smallest f (n) value, respectively; |
11: | If Positive node ==Reverse node; |
12: | The optimal path is obtained and the algorithm ends; |
13: | Break; |
14: | Else |
15: | While (Positive node & Reverse node)~= [] do |
16: | Search for jump points horizontally and vertically alone the direction from the parent node to the current node; |
17: | if Encounter obstacles or map edges; |
18: | Search for jump points diagonally alone the direction from the parent node to the current node; |
19: | Elseif Search for jump points; |
20: | Close_1 or Close_2 ← The searched jump points; |
21: | Else |
22: | Ignore the node, continue searching; |
23: | end |
24: | end |
25: | end |
26: | end |
27: | end |
Algorithm 2. Improved dynamic window approach (IDWA) | |
1: | Initializing (grid map, Robot, Evaluation_Factor); |
2: | Robot =[vmax,ωmax,amaxv,amaxω,VelocityResolution,RPMResolution] ; |
3: | Evaluation_Factor = [A; B; C; D]; |
4: | global_path ←Algorithm1 (BAJPSA*); |
5: | sensor_messages ← Robot; |
6: | if the trajectory without obstacles ← the Traditional DWA combined with the strategy in Section 3.4.2 to filter trajectories |
7: | while the local target location is not reached do ← the local target point information is obtained from equations (15-16) |
8: | Speed sampling of robot; |
9: | Simulate motion trajectories; |
10: | Use the improved evaluation function (17) to select the optimal trajectory; |
11: | Robot follows the optimal trajectory to move; |
12: | end |
13: | end |
Situation | Quantitative standard | Avoidance rule |
frontal collision | θ∈[315°,45°] | Avoid obstacles |
rear-end collision | ϕ∈[120°,240°] | Surpass or follow |
right collision | θ∈[45°,120°] | Avoid obstacles or decelerate to stop |
left collision | θ∈[240°,315°] | Avoid obstacles or decelerate to stop |
Note: where θ represents the movement direction of the obstacle towards the robot; ϕ represents the movement direction of the robot relative to the obstacle. |
case | Movement Status | Angle Relationship | Potential conflict or not | Conflict resolution strategies |
1 | ![]() |
|α−β|≥90° | NO | —— |
2 | ![]() |
|α−β|≥90° | NO | —— |
3 | ![]() |
|α−β|<90° | YES | AGV1 passes first, AGV2 stops the movement |
4 | ![]() |
|α−β|≥90° | NO | —— |
5 | ![]() |
|α−β|<90° | YES | AGV1 passes first, AGV2 stops the movement |
6 | ![]() |
|α−β|<90° | YES | AGV1 passes first, AGV2 stops the movement |
30×30 environment | 100×100 environment | |||||
Path parameters | A* | JPS | BAJPSA* | A* | JPS | BAJPSA* |
Length/m | 43.355 | 43.355 | 43.355 | 158.167 | 158.167 | 158.752 |
Run-times/s | 0.651 | 0.863 | 0.485 | 6.894 | 2.855 | 0.598 |
Number of path nodes |
34 | 24 | 27 | 131 | 55 | 89 |
Number of OPEN list |
253 | 130 | 51 | 2509 | 271 | 187 |
Number of CLOSE list | 394 | 98 | 32 | 6240 | 236 | 121 |
Path | Path length/m | Tracking error/m | Search time/s |
Path_1 | 17.7138 | 0.2255 | 50.8668 |
Path_2 | 17.3676 | 0.0577 | 55.8523 |
Path_3 | 17.3536 | 0.0551 | 61.3893 |