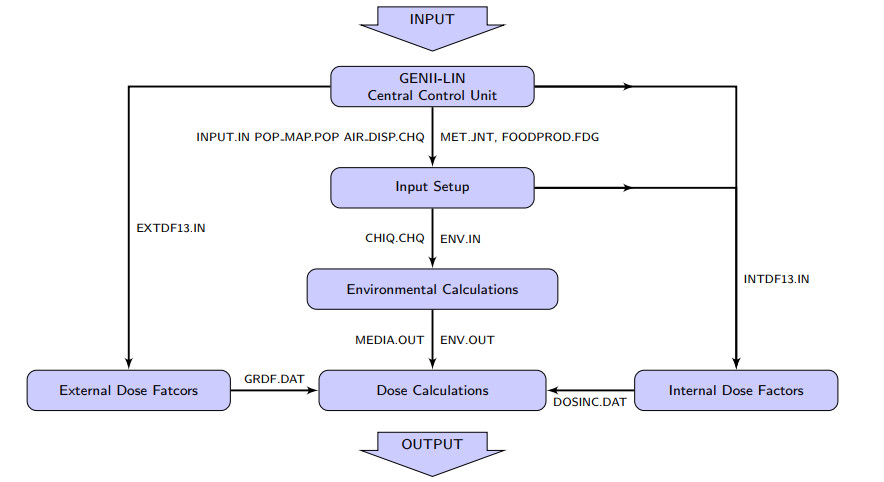
A fundamental step for safety assessment is the study and modeling of the radionuclide transfer through the environment up to reach and expose population to risk. In this vein we are working to provide a reliable and flexible computational framework which can be used for both retrospective and prospective calculations of radiation doses and human health effects, resulting from both routine and uncontrolled releases of radionuclides to the environment and from pre-existing environment contamination. The goal is to provide a multipurpose computational tool to be used for siting facilities, environmental impact statements, and safety analysis reports. The code can handle: external exposure from finite or infinite atmospheric plumes; external exposure from contaminated soil, sediments, and water; external exposure from special geometries; and internal exposures from inhalation, inadvertent intake of soil, consumption of terrestrial foods, aquatic foods, drinking water, and animal products.
Citation: Francesco Teodori. Health physics calculation framework for environmental impact assessment of radiological contamination[J]. AIMS Environmental Science, 2021, 8(4): 403-420. doi: 10.3934/environsci.2021026
[1] | Francesca Giacobbo, Mirko Da Ros, Elena Macerata, Eros Mossini . A case study of management and disposal of TENORMs: radiological risk estimation by TSD Dose and RESRAD-ONSITE. AIMS Environmental Science, 2021, 8(5): 465-480. doi: 10.3934/environsci.2021030 |
[2] | Christina Priavolou . To BIM or not to BIM? Lessons learned from a Greek vernacular museum building. AIMS Environmental Science, 2020, 7(2): 192-207. doi: 10.3934/environsci.2020012 |
[3] | Djordje Vilimanovic, Gangadhar Andaluri, Robert Hannah, Rominder Suri, A. Ronald MacGillivray . Occurrence and aquatic toxicity of contaminants of emerging concern (CECs) in tributaries of an urbanized section of the Delaware River Watershed. AIMS Environmental Science, 2020, 7(4): 302-319. doi: 10.3934/environsci.2020019 |
[4] | Muhammad Arinal Surgama Yusuf, Anwar Mallongi, Anwar Daud, Agus Bintara Birawida, Sukri Palutturi, Lalu Muhammad Saleh, Setiawan Kasim . Environmental health risk analysis from detergent contamination in well water. AIMS Environmental Science, 2025, 12(2): 256-275. doi: 10.3934/environsci.2025013 |
[5] | Philipp Braun, Timm Faulwasser, Lars Grüne, Christopher M. Kellett, Willi Semmler, Steven R. Weller . On the social cost of carbon and discounting in the DICE model. AIMS Environmental Science, 2024, 11(3): 471-495. doi: 10.3934/environsci.2024024 |
[6] | Tamara S. Wilson, Benjamin M. Sleeter, Jason Sherba, Dick Cameron . Land-use impacts on water resources and protected areas: applications of state-and-transition simulation modeling of future scenarios. AIMS Environmental Science, 2015, 2(2): 282-301. doi: 10.3934/environsci.2015.2.282 |
[7] | Siti Rachmawati, Syafrudin, Budiyono, Ellyna Chairani, Iwan Suryadi . Life cycle analysis and environmental cost-benefit assessment of utilizing hospital medical waste into heavy metal safe paving blocks. AIMS Environmental Science, 2024, 11(5): 665-681. doi: 10.3934/environsci.2024033 |
[8] | David P. Dolowitz . Stormwater management the American way: why no policy transfer?. AIMS Environmental Science, 2015, 2(3): 868-883. doi: 10.3934/environsci.2015.3.868 |
[9] | Eliasu Issaka, Mabruk Adams, Enock Adjei Agyekum, Josephine Baffoe, Blessing Tornyeava . Per- and poly-fluoroalkyl substances: A review of sources, properties, chromatographic detection, and toxicological implications. AIMS Environmental Science, 2025, 12(2): 321-351. doi: 10.3934/environsci.2025015 |
[10] | Jorge Sierra, Antoine Richard . Modeling the temporal dynamics of chlordecone in the profile of tropical polluted soils as affected by land use change. AIMS Environmental Science, 2021, 8(4): 304-320. doi: 10.3934/environsci.2021020 |
A fundamental step for safety assessment is the study and modeling of the radionuclide transfer through the environment up to reach and expose population to risk. In this vein we are working to provide a reliable and flexible computational framework which can be used for both retrospective and prospective calculations of radiation doses and human health effects, resulting from both routine and uncontrolled releases of radionuclides to the environment and from pre-existing environment contamination. The goal is to provide a multipurpose computational tool to be used for siting facilities, environmental impact statements, and safety analysis reports. The code can handle: external exposure from finite or infinite atmospheric plumes; external exposure from contaminated soil, sediments, and water; external exposure from special geometries; and internal exposures from inhalation, inadvertent intake of soil, consumption of terrestrial foods, aquatic foods, drinking water, and animal products.
The applications of nuclear technologies extend well beyond the energy production in power plants and have essential employments across multiple sectors. These include consumer products, food and agriculture, industry, medicine, scientific research, transport, water resources and environment. An effective and safe management of the nuclear systems demands the assessment of the consequences of accidental and chronic releases of radionuclides to the environment, the projects of plans for the protection from radiation, the development of methodologies for contaminated site clean up, and the realization and siting of high activity waste disposal sites. A needfull task for dose and risk assessment is the study and modeling of the processes that govern the transport of radionuclides through the environment up to reach and expose population to risk.
These are the reasons, why we started developing a reliable and flexible multipurpose health physics code to be used for:
● retrospective calculations of potential radiation doses resulting from routine and accidental releases of radionuclides to the environment;
● prospective dose calculations for siting facilities, environmental impact statements, and safety analysis reports.
GENII-LIN descends from the GENII software package [1,2], a thoroughly peer-reviewed [3,4] and well documented open source software system, which was developed at the Pacific Northwest National Laboratory (PNNL). The GENII final stable release was the 1.485 and went to production in the early 90s.
At the very beginning of the development, GENII-LIN was a simple porting of the GENII software package to the Linux operating system. The code was patched to grant compatibility with recent CPU and compilers. The old text based user interface was replaced by a more modern, more advanced, and more featured graphical one, built on the Qt3 libraries [5]. In these years, GENII-LIN has been continuously improved and its capabilities progressively extended. Internal and external dose rate factor generators have been deeply revised ([6,7]) to couple the embedded environmental pathway analysis models with the more recent internal dosimetry models recommended by the ICRP 72 [8] as well as with the radiological risk estimating procedures of the FGR 13 [9]. The internal nuclide databases now comprise most of the short-lived nuclides used in radiopharmaceutical facilities. The air transport models cover a wider variety of release dispersion and exposure scenarios. The input and output management has been necessarily reviewed and the graphical user interface has been accordingly redesigned and built against the more recent Qt4 [10] and Qt5 [11] libraries to increase flexibility and portability [12]. The external dose rate factor generator has been reworked [13]. Now, it calculates organ dose and total body effective dose, by applying the recommendations of ICRP 103 [14], by accessing the data libraries of radionuclide decay information and gamma and beta yields from ICRP 107 [15], and by accessing the kerma in air at body surface to organ dose conversion coefficients from information in ICRP 116 [16]. The soil transfer model has been reworked in a more physically based manner, by implementing a more sophisticated formulation for the transfer phenomena and by increasing the soil compartment number [17,18]. In this work, we want to show the GENII-LIN capabilities, the code structure, its conceptual design in the light of recent improvements.
GENII-LIN is an integrated environment modeling software system capable of addressing radiation dose and risk assessments to individuals or populations from a variety of potential release and exposure scenarios. As needed, it implements system thinking and integrative approaches, which go beyond the traditional single discipline approaches, moving towards multi disciplinary analysis of complex environmental problems. The main features of the GENII-LIN system include:
● capabilities for calculating annual radiation dose, committed radiation dose and accumulated radiation dose from acute and chronic releases;
● capabilities for evaluating a wide variety of exposure scenarios including direct exposure to water (recreational activities), soil (buried and surface sources) and air (semi-infinite cloud and finite cloud model), inhalation pathways and ingestion pathways.
The releases considered are:
● chronic and acute releases to air, from ground level or elevated sources;
● chronic and acute releases to water;
● chronic releases to deep soil from degradation of waste forms;
● existing contamination of soil or surfaces and environmental media.
A more advanced soil transfer model is under development, that will be able to handle acute releases to deep and surface soil.
Potential inputs to the code are total or specified releases or release rates of radioactivity to the environment, as well as input of measured concentrations in specified environmental media.
The code is an integrated environmental modeling system, which is able to perform complex analyses and to make informed predictions, and includes several science based environmental dosimetry modules. Each module is a self supporting standalone system component and is responsible for retrieving, elaborating and producing information autonomously. The very flexible and effective structure of the package is schematized in Figure 1. Each module implements one or more models. When needed, a dedicated user interface allows module specific input and provides implemented model descriptions. Internal and external pre and post data processors grant information transfer by converting module input and output in a form, which is usable by the system and understandable by the user. The main structural components are an environmental transport and human exposure component (collecting most of the implemented models) coupled to internal and external dosimetry modules. Components and modules are governed by an interactive central control unit, by which the user has a full actual control on input setup, on calculation process, and on output management. The central control unit is in charge of
● information transfer from one component to another with unambiguous, shared meaning (syntactic and semantic interoperability);
● institution of local (module specific) and global (system wide) metadata standards of input, output and databases;
● data and information flow management among modules and through the system;
● granting quality control of information exchange, through an effective warning and error handling.
The central control unit manages the computation process at two distinct levels:
● it checks that each individual module runs towards completion successfully;
● it calls all modules one by one, in the correct order, within the workflow.
While some modules are specifically designed to pause to allow user interaction (this is the case of external and internal dosimetry modules, for instance), most of them run sequentially to completion. The individual completion status of modules is monitored and documented by capturing and writing information to the output buffer. The amount of debugging information, to be displayed, is set by the user. The central control unit allows for the complete workflow execution, calling the modules in the appropriate order without interruptions or user action requests. The information exchange among the modules is established by data transfer and buffer files, some of which are reported in Figure 1: these files may be either provided by the user or generated by the modules to transfer information to the downstream ones: the output of one module is stored in a file that feeds the next module in the stream. Most of these files are transparent to the user and can be accessed directly, either for checking intermediate calculation results or for retrieving significant data, like information on intake rates and annual media concentrations. These files are intended to be used as inputs to the dose calculation modules of GENII-LIN and should not be edited without enough programming skills.
The environmental transfer and exposure modules reproduce the behavior of the environmental system by using computational models. These models numerically simulate the biological, physical, and chemical processes that govern the transfer of radionuclides through the environmental media up to reach the final receptors, and calculate human uptake, and human exposure to contaminants that result from the set up exposure scenario. After reading the input files and capturing a conceptual site model, the code sequentially performs numerical simulations to establish air, surface soil, deep soil, and ground and surface water concentrations of each radionuclide at the start of the exposure scenario, and for each year over all the exposure period. Modeled transfer processes account physical decay of preexisting sources, biotic transport of existing subsurface contamination, geohydrology, surface water transport, and soil contamination from continuing atmospheric or irrigation depositions and leaching. Human exposures and intakes of each radionuclide are calculated for:
● external exposure to atmospheric plumes (finite or semi-infinite plume model);
● inhalation from plume submersion, from resuspension of deposited material and from suspension of pre-existing contamination in soil;
● external exposure to contaminated soil, sediments, and water;
● external exposure to arbitrarily shaped sources;
● internal exposure from consumption of terrestrial foods, aquatic foods, drinking water, animal products, and from inadvertent intake of soil.
The different transfer phenomena modeled by the GENII-LIN software system are illustrated in Figure 2, which describes how science based modules can be organized, implemented and mutually interfaced to analyze coherently a specific problem, by merging several usually separate fields of expertise. The data flow diagram explains that known or postulated radioactivity amounts can diffuse through the environment following different available routes up to the final step of exposure scenario definition, and radiation dose calculation. The code flexibility and code capabilities are significantly extended by providing interfaces, through which the user can input calculated or measured values of atmospheric dispersion, ground water concentrations, and surface water concentrations, at specified points in the simulation process (hexagonal boxes). In this way, if the GENII-LIN embedded models are not enough specialized for a given scenario, more accurate and rigorous results may be effectively obtained with minimal effort.
In most of the practical situations, the known input quantities are activity releases or activity release rates, collected as "Release Terms" on the left of Figure 2. These terms may express the total activities or activity per unit time released into the transport media: air, surface water, groundwater, or soil. Specialized numerical simulations of the transfer phenomena (square boxes) turn them to basic and derived concentrations in the corresponding environmental media. When basic or derived concentrations are already known, they can be input directly to the code, through provided interfaces, and the upstream transfer calculations can be skipped. When the human exposure scenario is set up, the dose can be estimated.
The dose calculation modules of the GENII-LIN package capture the exposure scenario assembled by the Environmental Transfer Modules, and convert the annual intake and exposure rates to radiation dose: both effective (total body) dose and equivalent doses to individual organs and tissues. The calculations are done by using precalculated dose factors from external and internal dosimetry modules.
Three options are available to the user:
ICRP 30 when he wants to follow the recommendations in the ICRP 26 [19], ICRP 30 [20], and ICRP 48 [21], as incorporated in the FGR 11 [22];
FGR 13 when he wants to follow the recommendations in the ICRP 72 [8], as incorporated in the FGR 13 [9];
ICRP 103 when he wants to follow the recommendations in the ICRP 103 [14].
The dose calculation modules may also generate reports of doses by pathway and by radionuclides. The output detail level is set by the user, before starting calculations. If the recommendations of the FGR 13 [9] or ICRP 103 [14] are followed, the outputs are age dependent, and the code also computes risk estimate for health effects to individuals or populations.
The code calculates for types of dose:
● One-Year Dose: radiation dose to individuals or populations due to one year of external irradiation and to one year of internal irradiation from the radioactivity ingested and inhaled in that same year.
● Committed Dose: the ingested or inhaled radionuclides irradiate organs and tissues over time periods, whose lengths may extend well beyond the intake period and depend on nuclide physical half-lives and nuclide persistent keeping within the body; in this vein, incorporated nuclides may continue to irradiate body tissues for many months after the beginning of the intake; in order to regulate exposures to radionuclides and the accumulation of radiation dose over extended periods of time, the ICRP introduced the concept of committed dose. When this option is selected, the code calculates the dose to populations or individuals from one year of external exposure to radioactive materials plus the time integral of the internal dose over an age dependent extended period of time starting from the beginning of the intake. The ICRP recommends commitment periods of 50 years for adults and up to the age of 70 for other people. In most countries, regulatory authorities recommend this dose as a measure of the potential, longer term impact of accidental and routine releases.
For research and investigation purposes, GENII-LIN allows for specifying arbitrarily long commitment periods.
● Cumulative dose: this is the population or individual dose that results from an arbitrarily long external irradiation period plus the committed internal dose from intake of nuclides, via ingestion and inhalation, in the same arbitrarily long period. This kind of calculation requires the knowledge of the time dependent media concentrations of radionuclides in the environment during all the exposure period. The cumulative dose calculation may be useful, when it is required to assess the total potential impacts from exposure scenarios involving chronic releases over periods of several years from residual deposition, resulting from accidental acute releases or from significant residual contamination from decommissioning and spilling operation.
● Maximum Annual Dose: the largest committed dose that occurs over a specified arbitrarily long exposure period. For individuals or populations exposed to a contaminated environment for a long time, the committed dose, as well the annual dose, depends on the number of years spent on site before starting the dose calculation, i.e, before the year of interest. Individuals are exposed to an environment, whose contamination levels change over time by nuclide physical decay and transfer processes. Nuclide concentrations in the body and the annual organ and total body dose rates increase and reach their maximum when equilibrium is established between the body burden and the environment radionuclide concentrations. Dose calculations of this kind imply to accounts for external exposure during the year of interest plus the internal dose from radionuclides accumulated in the body from the beginning of exposure up to the end of the year under investigation. Depending on radionuclide species, and on the biological, physical, and chemical processes that determine transport of radionuclides through environmental media, equilibrium may require many years from the beginning of exposure.
Calculations of this type of dose may be important to verify compliance to the existing dose limits for occupational and public exposure.
The method, by which the code assembles these doses, is illustrated in Figure 3. The internal dose rate factor generators provide the equivalent dose rates, ˙Dt(τ,y), to a specified organ t, at specified ages y, for unit intake of activity of a specific radionuclide at specified time τ. The dose calculation modules calculate the organ equivalent dose over a commitment period of Tc years from the beginning of the intake, i.e., to the age τ+Tc, as
∫τ+Tcτ˙Dt(τ,y)dy | (1) |
If u(τ) is the intake rate of activity at age τ, when the intake extends over a period of Ti years (Ti≤Tc), the accumulated organ equivalent dose is computed as
∫τ+Tiτd˜τu(˜τ)∫˜τ+Tc˜τ˙Dt(˜τ,y)dy | (2) |
The effective (total body) dose to an individual is found by calculating a weighted sum of the equivalent doses to different body organ and tissues. The weighting factors express the different radiosensitivities of the different body tissues to ionizing radiation. The number of organs/tissues considered and the related weighting factors depends on the option selected, (see table 1).
Organs | Tissue Weighting Factors | ||
ICRP 30 | FGR 13 | ICRP 103 | |
Gonads | 0.25 | 0.20 | 0.08 |
Red Bone Marrow | 0.12 | 0.12 | 0.13 |
Colon | - | 0.12 | 0.19 |
Lung | 0.12 | 0.12 | 0.16 |
Stomach | - | 0.12 | 0.12 |
Breasts | 0.15 | 0.05 | 0.12 |
Bladder | - | 0.05 | 0.04 |
Liver | - | 0.05 | 0.04 |
Esophagus | - | 0.05 | 0.04 |
Thyroid | 0.03 | 0.05 | 0.04 |
Skin | - | 0.01 | 0.01 |
Bone surface | 0.03 | 0.01 | 0.01 |
Salivary glands | - | - | 0.01 |
Brain | - | - | 0.01 |
Remainders | 0.30 | 0.05 | 0.12 |
For external exposure, no commitment period needs to be considered and the problem. Being c(y) the activity of the radionuclide in the media at the age y, τ the age at the beginning of the exposure and Te the exposure duration, the external organ dose is computed as
˙Dt∫τ+Teτdyc(y) | (3) |
where ˙Dt is the age independent, radionuclide specific, and organ related external dose rate factor.
For internal exposure, the cancer risk over Tc years for unit intake of radionuclide at specified age τ is calculated according to the recommendations in the FGR 13 [9], by the equation:
∫τ+TcτS(y)rt(y)˙D∗t(τ,y)dyS(τ) | (4) |
In equation 4, the term rt(y) denotes the organ or tissue specific lifetime risk coefficient (LRC): the risk of a subsequent cancer death, per unit absorbed dose. The survival function, S(y), is the fraction of live-born individuals in an unexposed population expected to survive at the age y. ˙D∗t is the sum of the absorbed dose rate factor for low-LET radiation plus the high-LET absorbed dose rate multiplied by the RBE applicable to the cancer type. If the uptake extends over a period of Ti years (Ti≤Tc), the accumulated organ or tissue specific cancer risk to age τ+Tc is calculated as
∫Ti+ττd˜τu(˜τ)∫˜τ+Tc˜τS(y)r(y)˙D∗t(˜τ,y)dyS(˜τ) | (5) |
Both S(y) and r(y) are gender specific and need to be combined by calculating a weighted mean. The total risk is simply the sum of site specific cancer risk over all cancer sites.
For external exposure, the organ or tissue specific cancer risk is computed as
˙Dt∫τ+Teτdyr(y)c(y)S(y)S(τ) | (6) |
To calculate population dose and risk, the code needs to know the composition and the spatial distribution of the individuals over the area of interest. The user may be asked to provide a population grid. When building the grid, the user must pay attention to avoid unsuitable aggregation of individuals and must pay great attention to their age distribution and to the length of the exposure period [14]. When ingestion is considered, the code may also ask the user to provide a food production grid. The output can be requested for the whole population or for specified sectors of the grid.
As shown in Figure 3, annual doses, committed doses and accumulated dosed are assembled starting from the knowledge of annual dose rates. The dose factors available in ICRP 30 [20], ICRP 72 [8] and ICRP 103 [14] cannot be used by the code. An annual dose rate cannot be obtained by dividing the provided committed doses by the commitment period. The internal dose rate factor generators, embedded in the GENII-LIN software system, are conceived to generate the required annual increments of organ and tissue doses, for all the years following an initial intake, up to cover any specified period of time. When the user decides to apply the recommendations in FGR11 [22], the internal dose factor generators simulate the nuclide transport through the human boby, by implementing the biokinetic models and by adopting the nuclide body residency data and body transfer data given in ICRP 30 [20]. After calculating the time dependent organ and tissue nuclide concentrations, the code calculates the organ dose equivalent:
● by accounting the total number of nuclear transformations for all the nuclides in the source organs over the period of interest;
● by accounting the energy absorbed in the unit mass of the target organs, properly modified by applying radiation type specific quality factors, for each transmutation of the radionuclides in the source organs.
The initial value problem, requires the solution of a coupled set of differential equations. The calculation result is the yearly increment of dose to each organ, which is then used by the dose calculation programs to assess annual doses, committed doses, and accumulated doses. The module has been inherited by GENII-1.485 and has been left almost untouched. Very little patches have been applied to solve numerical stability issues, rising from building the code on newer CPUs, by newer compilers. By selecting the FGR 13 or ICRP 103 option, the user calls completely new dose rate factor generators, which implement the more recent internal dosimetry models recommended by ICRP 72 [8] or the recommendations of ICRP 103 [14]. Instead of solving the equation of transfer through human body, the internal dose factor generators provide precalculated dose rate libraries, which contain the nuclide specific dose rates in the organs and tissues, sampled at non-uniformly spaced times following an acute intake by individuals of the six representative ages: infants, 1 year old children, 5 year old children, 10 year old children, 15 year old children and adults. For all the radionuclides specified in the input file, the code extracts data from those libraries and fits each set of collected dose rate data by splines under tension. The piece-wise interpolation curves are integrated over time, generating age dependent libraries of incremental organ equivalent doses for all the years following the initial unit activity intake. The code generates one library for each of the representative ages. Each library contains yearly increments of doses to 29 organs and tissues. These libraries are then used to calculate age dependent annual doses, committed dose, and accumulated doses.
Dose from external exposure depends on many factors: source geometry; source composition; nuclide distribution; receptor position; irradiation geometry; shield presence. It is out of question to provide external dose rate factor libraries to be applied to all the arbitrary shaped radiation sources. In environmental radiation protection, most of the used codes commonly provide dose rate factors for simplified and idealized exposure geometries: usually, submersion in a semi-infinite cloud, exposure to ground surface contamination and exposure to soil uniformly contaminated to an infinite depth. The GENII-LIN itself offers built-in dose rate factor libraries, which are suitable for commonly occurring exposure scenarios:
● submersion in a semi-infinite cloud of radioactive air;
● immersion in contaminated water;
● direct exposure to surface ground contamination; and
● direct exposure to top soil contaminated to an infinite depth, with optional uncontaminated soil overburden.
For all those practical situations, where these embedded dose rate factor libraries can not be used without introducing significant errors, GENII-LIN allows for the compilation of dose rate factor libraries for irradiation from arbitrarily shaped sources. The external dose factor generators are specialized and improved versions of the widely tested and well documented shielding code ISOSHLD: [23,24,25,26]. The code has been streamlined by removing the unneeded RIBD/ISOCALC routines, originally implemented to calculate source strength from reactor fission fuel. The originally embedded data libraries, collecting nuclide decay data and gamma and beta yields, have been replaced by updated and extended ones, built on radioactive decay data provided by Kocher [27], and, more recently, further revised by using data retrieved from ICRP 107 [15]. Instead of solving the radiation transfer equation, the ISOSHLD code implements the point kernel method: a macroscopic and widely used approach to compute gamma radiation exposure rate. In the point kernel approach, gamma radiation propagates though media like a beam. Radiation interaction with matter is quantified by using reaction specific macroscopic cross sections. Scattered radiation contributions are accounted by applying appropriate buildup factors, which express the ratio between the total value of the quantity, assessed at the evaluation point, and the value due to only primary radiation. Volumetric sources are ideally divided into an enough large number of elementary individual cells. Each cell acts as an individual point source. Except for few simpler geometries, where analytical solutions are achievable, the radiation field and total dose rate calculation at a given point of interest requires a numerical integration over the source volume. The basic source geometries are the same standard 14 ones available in ISOSHLD. The point kernel calculations result in the assessment of a kerma rate in air, at the point of interest, expressed in Gys or equivalent units. The dose calculation modules require external dose rate factors expressed in terms of tissue dose equivalent and effective dose equivalent. Therefore, the kerma rate in air is converted to dose equivalent by using energy and irradiation geometry dependent surface dose to tissue dose equivalent conversion coefficients. These coefficients express the irradiation geometry dependent ratio between the equivalent tissue dose and air kerma in air at body surface. If the user decides to apply the recommendations in FGR 11 [22], the external dose factor generator adopts the dose rate conversion coefficients collected by Kocher [28]. These libraries are the same originally used by GENII-1.485. Only total body dose rate factors are generated, when this option is selected. When the code is asked to apply the recommendations in FGR 13 [9] or in ICRP 103 [14], the external dose rate factor generators use the newer and more detailed dose rate conversion coefficient libraries provided by ICRP 116 [16]. The computed dose rate factors are organ and tissue specific, and output data are organized for cancer risk calculation.
The Central Control Unit is a centralized management system, by which the user has a complete control over scenario definition, calculation processes and output management. Its object oriented design allows relatively easy inclusion of additional modules and models, promoting further code development. It grants access to external data file and data libraries, optimizes data flow and data collection, minimizing data exchange requirement within GENII-LIN. It provides interfaces to external programs and addresses linkage concerns to system wide shared libraries. The Central Control Unit is controlled by the user, through a full featured Graphical User Interface written in C++, built on the Qt libraries, [10,11]. By browsing wizard like editors, whose startup page is shown in Figure 4, the user can effectively select the required parameters and options for scenario modeling, and can provide input data to the internal and external dose factor generators, and to the environmental dosimetry programs. Well ordered pages, whose number increases or decreases with the scenario complexity, lead the user, through the stepwise process of input setup. The wizard is designed and structured to:
● minimize data entry, by asking only for current scenario pertinent data;
● prevent errors, by checking for option incompatibilities and by alerting the user when these are discovered; no further editing is allowed without solving compatibility issues.
Default values are suggested for all the variables. All input data are checked against reasonable and mandatory bounds.
Before starting calculations the central control unit checks for the presence of all the auxiliary files needed by the current scenario and allows the user for reading, editing, creating and importing them, by activating embedded viewers and editors.
The GUI also gives full access to the output files that can be displayed both through embedded text viewers (Figure 5) and through embedded graphical viewers (Figure 6). The output can also be exported as CSV files or as spreadsheets.
The code development platform is a PC running openSUSE Linux. The code has been successfully built an tested on many other Linux distributions, as confirmed by positive feedbacks from a large number of users. Either to run or to build the code from sources, the Qt4 or Qt5 libraries are mandatory. The large data libraries require at least 500 MB of free space on disk. Code performances on modern CPU are very good: few minute long typical runtimes and low resource demand. Programming skills may be useful for error debugging, but are not required to run calculations. The code is completely open source, available for free, and does not contain any commercial software. The experienced user, who wants to build the code from sources, needs a C++ compiler for the Graphical User interface, and a FORTRAN compiler for the computational modules. Being the one used for the development, the GNU compiler collection is recommended, but not mandatory. Other compilers have been used with success, but not intensively tested.
We started developing a multipurpose health physics code able to perform:
● retrospective calculations of potential radiation doses resulting from routine and accidental releases of radionuclides to the environment;
● prospective calculations for siting facilities, environmental impact statements, and safety analysis reports.
Our work shows how several science based environmental dosimetry components, built by merging usually separate fields of expertise, can be organized, linked and used to synergically assess the diffusion of known or postulated amounts of radioactivity through the environment, along different available routes up to the final step of exposure scenario definition, and radiation dose calculations.
The result is a reliable and flexible tool, with an effective modular and object oriented design, capable for:
● calculating radiation dose for acute and chronic releases to environmental media;
● evaluating a large variety of exposure pathways including direct exposure via water, soil and air, inhalation and ingestion.
The code can handle many exposure pathways, comprising:
● external exposure from finite or infinite atmospheric plumes;
● external exposure to arbitrarily shaped sources;
● external exposure to contaminated soil, sediments, and water;
● internal exposure from inhalation, consumption of terrestrial foods, aquatic foods, drinking water, animal products, and inadvertent intake of soil.
The fully featured graphical interface provide a file editor conceived like a wizard, which effectively guides the user step by step through the scenario definition process. The code is available for free, completely open source. The software design is modular and strictly object oriented, reason why code improvement and patching is easy. The inclusion of new modules and the replacement of the implemented ones require a relatively little effort. The code has been widely and successfully used for environmental impact and safety assessments regarding several nuclear fuel cycle and radiopharmaceutical facilities.
At present, we are working to enhance the deep soil transfer model.
[1] | Napier B A, Peloquin R A, Strenge D L, et al (1988) GENII - The Hanford Environmental Radiation Dosimetry Software System. 1: Conceptual Representation, PNL-6584. |
[2] | Napier B A, Peloquin R A, Strenge D L, et al (1988) GENII - The Hanford Environmental Radiation Dosimetry Software System. 2: Users' Manual, PNL-6584. |
[3] | DOE, (2003) Software Quality Assurance Plan and Criteria for the Safety Analysis Toolbox Codes, Defense Nuclear Facilities Safety Board Recommendation 2002-1 Software Quality Assurance Improvement Plan Commitment 4.2.1.2, U.S. Department of Energy, Washington, D.C. J Amer Math Soc |
[4] | DOE (2004) Defense Nuclear Facilities Safety Board Recommendation 2002-1, Software Quality Assurance Improvement Plan, Commitment 4.2.1.3: Software Quality Assurance Improvement Plan: GENII Gap Analysis. DOE-EH-4.2.1.3-GENII-Gap Analysis U.S. Department of Energy, Washington, D.C. |
[5] | Sumini M, Teodori F, Cantoro N (2005) GENII-LIN: a new object-oriented interface for the GENII Code. Radiat Prot Dosim 116: 597-600. |
[6] | Sumini M, Teodori F (2005) GENII-LIN: a Multipurpose Health Physics Code Built on GENII-1. 485. J Radiol Prot 4: 36-42. |
[7] | Teodori F, Sumini M (2008) GENII-LIN-2.1 an open source software system for calculating radiation dose and risk from radionuclides released to the environment. J Radiol Prot 27: 465-470. |
[8] | ICRP (1995) Age-dependent Doses to the Members of the Public from Intake of Radionuclides - Part 5 Compilation of Ingestion and Inhalation Coefficients, ICRP Publication 72, Ann. ICRP 26 (1). |
[9] | EPA 2002 Federal Guidance Report 13 Cancer Risk Coefficients for Environmental Exposure to Radionuclides: CD Supplement, EPA 402-C-99-001, Rev. 1 (Oak Ridge National Laboratory, Oak Ridge, TN; U.S. Environmental Protection Agency, Washington, DC) |
[10] | Molkentin (2007) The Book of Qt 4: The Art of Building Qt Applications (1st ed.), No Starch Press, 440, ISBN 978-1593271473 |
[11] | Lee Zhi Eng (2018) Hands-On GUI Programming with C++ and Qt5, Birmingham: Packt Publishing Limited, 4040, ISBN 9781788397827 |
[12] | Teodori F, Sumini M (2014) GENII-LIN project: a Multipurpose Health Physics Code to Estimate Radiation Dose and Risk from Environment Contamination Radiat Phys Chem 104: 15-22. |
[13] | Teodori F (2019) The new external dose rate factor generator of the GENII-LIN health physics code. Radiat Phys Chem 155: 107-114. |
[14] | ICRP (2007) The 2007 Recommendations of the International Commission on Radiological Protection, ICRP Publication 103, Ann. ICRP 37 (2-4). |
[15] | ICRP (2008) Nuclear Decay Data for Dosimetric Calculations, ICRP Publication 107, Ann. ICRP 38. |
[16] | ICRP (2010) Conversion Coefficients for Radiological Protection Quantities for External Radiation Exposures. ICRP Publication 116, Ann. ICRP 40 (2-5). |
[17] | Teodori F (2017) A new nuclide transport model in soil in the GENII-LIN health physics code. Radiat Phys Chem 140: 146-149. |
[18] | Teodori F (2020) The latest development and the new extended capabilities of the GENII-LIN soil transfer model. Radiat Phys Chem 174: 108949 |
[19] | ICRP (1977) Recommendations of the ICRP, ICRP Publication 26, Ann. ICRP 1 (3). |
[20] | ICRP (1982) Limits for Intakes of Radionuclides by Workers, ICRP Publication 30 (Index), Ann. ICRP 8 (4). |
[21] | ICRP (1986) The Metabolism of Plutonium and Related Elements, ICRP Publication 48, Ann. ICRP 16 (2-3). |
[22] | EPA (1988) Limiting Values of Radionuclide Intake and Air Concentration and Dose Conversion Factors for Inhalation, Submersion, and Ingestion, FGR No. 11, (U.S. Environmental Protection Agency, Washington, DC). |
[23] | Engel R L Greenborg J and Hendrickson M M (1966) ISOSHLD - A Computer Code for General Purpose Isotope Shieldinq Analysis, BNWL-236, Pacific Northwest Laboratory, Richland, Washington. |
[24] | Simmons G L, Regimbal J J, Greenborg J, Kelley E L Jr, Van Tuyl H H (1967), ISOSHLD-II: Code Revision to Include Calculation of Dose Rate from Shielded Bremsstrahlunq Sources, BNWL-236 SUP 1, Pacific Northwest Laboratory, Richland, Washington. |
[25] | Mansius C A (1969) A Revised Photon Probabilitv Library For Use With ISOSHLD-III, BNWL-236 SUP 2, Pacific Northwest Laboratory, Richland, Washington. |
[26] | Greenborg J Schmittroth F A Rittman P D and Roetman V E (1996) ISOSHLD 4.0, Westinghouse Hanford Company, Richland, Washington. |
[27] | Kocher D C (1981) Radioactive Decay Data Tables: A Handbook of Decay Data for Application to Radiation Dosimetry and Radiological Assessments, DOE/TIC-11026, (U. S. Department of Energy, Energ-y, Washington, D.C.). |
[28] | Kocher D C (1981) Dose-Rate Conversion Factors for External Exposure to Photons and Electrons, NUREG/CR-1918 (ORNL/NUREG-79) |
1. | María Sancho, Radioactive waste management and disposal – introduction to the special issue, 2023, 10, 2372-0352, 125, 10.3934/environsci.2023007 |
Organs | Tissue Weighting Factors | ||
ICRP 30 | FGR 13 | ICRP 103 | |
Gonads | 0.25 | 0.20 | 0.08 |
Red Bone Marrow | 0.12 | 0.12 | 0.13 |
Colon | - | 0.12 | 0.19 |
Lung | 0.12 | 0.12 | 0.16 |
Stomach | - | 0.12 | 0.12 |
Breasts | 0.15 | 0.05 | 0.12 |
Bladder | - | 0.05 | 0.04 |
Liver | - | 0.05 | 0.04 |
Esophagus | - | 0.05 | 0.04 |
Thyroid | 0.03 | 0.05 | 0.04 |
Skin | - | 0.01 | 0.01 |
Bone surface | 0.03 | 0.01 | 0.01 |
Salivary glands | - | - | 0.01 |
Brain | - | - | 0.01 |
Remainders | 0.30 | 0.05 | 0.12 |
Organs | Tissue Weighting Factors | ||
ICRP 30 | FGR 13 | ICRP 103 | |
Gonads | 0.25 | 0.20 | 0.08 |
Red Bone Marrow | 0.12 | 0.12 | 0.13 |
Colon | - | 0.12 | 0.19 |
Lung | 0.12 | 0.12 | 0.16 |
Stomach | - | 0.12 | 0.12 |
Breasts | 0.15 | 0.05 | 0.12 |
Bladder | - | 0.05 | 0.04 |
Liver | - | 0.05 | 0.04 |
Esophagus | - | 0.05 | 0.04 |
Thyroid | 0.03 | 0.05 | 0.04 |
Skin | - | 0.01 | 0.01 |
Bone surface | 0.03 | 0.01 | 0.01 |
Salivary glands | - | - | 0.01 |
Brain | - | - | 0.01 |
Remainders | 0.30 | 0.05 | 0.12 |