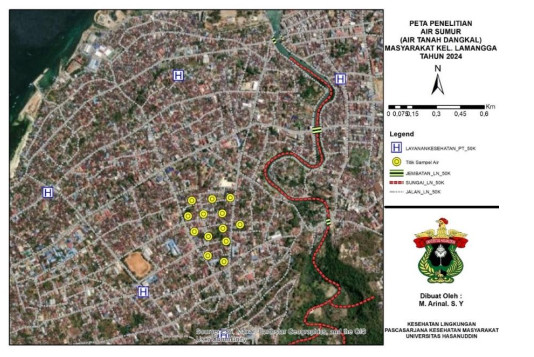
High concentrations of detergent waste disrupt aquatic biota. Detergent content can increase nutrient levels, causing environmental problems. The purpose of this study was to determine the level of public health risk in Lamangga Village, Baubau City, where well water containing phosphate and surfactants is consumed. This was an observational study with environmental health risk analysis. The study was composed of representative samples from 14 wells used as a source of drinking water and 70 respondents. The results showed that the average concentration of phosphate in drinking water sources across the 14 sampling points was 0.020 mg/L and that of surfactants was 0.39 mg/L. The rate of exposure to detergent concentration in raw water (intake rate) is directly proportional to the risk level value (RQ). The RQ of all respondents was ≤ 1. All 70 respondents had a target hazard quotient (THQ) ≤ 1, and no respondents had a THQ value higher than 1. The standardization values issued by the US-EPA Agency and the Regulation of the Minister of Health of the Republic of Indonesia Number 32/2017 set the reference dose (RfD) value of surfactants and phosphate at 0.05 mL/L/d. Surfactant exposure can cause irritation to the skin and eyes and damage the skin's natural protective layer, while phosphate exposure is not directly toxic to humans in small concentrations. Risk management strategies are necessary to control phosphate and surfactant concentrations in drinking water so that they do not result in future non-carcinogenic risk effects.
Citation: Muhammad Arinal Surgama Yusuf, Anwar Mallongi, Anwar Daud, Agus Bintara Birawida, Sukri Palutturi, Lalu Muhammad Saleh, Setiawan Kasim. Environmental health risk analysis from detergent contamination in well water[J]. AIMS Environmental Science, 2025, 12(2): 256-275. doi: 10.3934/environsci.2025013
[1] | Francesco Teodori . Health physics calculation framework for environmental impact assessment of radiological contamination. AIMS Environmental Science, 2021, 8(4): 403-420. doi: 10.3934/environsci.2021026 |
[2] | Acile S. Hammoud, Jessica Leung, Sabitri Tripathi, Adrian P. Butler, May N. Sule, Michael R. Templeton . The impact of latrine contents and emptying practices on nitrogen contamination of well water in Kathmandu Valley, Nepal. AIMS Environmental Science, 2018, 5(3): 143-153. doi: 10.3934/environsci.2018.3.143 |
[3] | Junhua Guo, Bin Hua, Na Li, John Yang . Stabilizing lead bullets in shooting range soil by phosphate-based surface coating. AIMS Environmental Science, 2016, 3(3): 474-487. doi: 10.3934/environsci.2016.3.474 |
[4] | Ebere Enyoh Christian, Qingyue Wang, Wirnkor Verla Andrew, Chowdhury Tanzin . Index models for ecological and health risks assessment of environmental micro-and nano-sized plastics. AIMS Environmental Science, 2022, 9(1): 51-65. doi: 10.3934/environsci.2022004 |
[5] | Fernanda P. Maciel, Joshua M. Peschel . A GIS-based tool for bioaccumulation risk analysis and its application to study polychlorinated biphenyls in the Great Lakes. AIMS Environmental Science, 2018, 5(1): 1-23. doi: 10.3934/environsci.2018.1.1 |
[6] | Ernyasih, Anwar Mallongi, Anwar Daud, Sukri Palutturi, Stang, Abdul RazakThaha, Erniwati Ibrahim, Wesam Al Madhoun, Andriyani . Strategy for mitigating health and environmental risks from vehicle emissions in South Tangerang. AIMS Environmental Science, 2023, 10(6): 794-808. doi: 10.3934/environsci.2023043 |
[7] | Leonardo Martínez, Stephanie Mesías Monsalve, Karla Yohannessen Vásquez, Sergio Alvarado Orellana, José Klarián Vergara, Miguel Martín Mateo, Rogelio Costilla Salazar, Mauricio Fuentes Alburquenque, Ana Maldonado Alcaíno, Rodrigo Torres, Dante D. Cáceres Lillo . Indoor-outdoor concentrations of fine particulate matter in school building microenvironments near a mine tailing deposit. AIMS Environmental Science, 2016, 3(4): 752-764. doi: 10.3934/environsci.2016.4.752 |
[8] | Patricia Morcillo, Maria Angeles Esteban, Alberto Cuesta . Mercury and its toxic effects on fish. AIMS Environmental Science, 2017, 4(3): 386-402. doi: 10.3934/environsci.2017.3.386 |
[9] | Djordje Vilimanovic, Gangadhar Andaluri, Robert Hannah, Rominder Suri, A. Ronald MacGillivray . Occurrence and aquatic toxicity of contaminants of emerging concern (CECs) in tributaries of an urbanized section of the Delaware River Watershed. AIMS Environmental Science, 2020, 7(4): 302-319. doi: 10.3934/environsci.2020019 |
[10] | Nasrul Arahman, Cut Meurah Rosnelly, Sri Mulyati, Wafiq Alni Dzulhijjah, Nur Halimah, Rinal Dia'ul Haikal, Syahril Siddiq, Sharfina Maulidayanti, Muhammad Aziz, Mathias Ulbricht . Investigation of microplastics in community well water in Banda Aceh, Indonesia: a separation technique using polyethersulfone-poloxamer membrane. AIMS Environmental Science, 2025, 12(1): 53-71. doi: 10.3934/environsci.2025003 |
High concentrations of detergent waste disrupt aquatic biota. Detergent content can increase nutrient levels, causing environmental problems. The purpose of this study was to determine the level of public health risk in Lamangga Village, Baubau City, where well water containing phosphate and surfactants is consumed. This was an observational study with environmental health risk analysis. The study was composed of representative samples from 14 wells used as a source of drinking water and 70 respondents. The results showed that the average concentration of phosphate in drinking water sources across the 14 sampling points was 0.020 mg/L and that of surfactants was 0.39 mg/L. The rate of exposure to detergent concentration in raw water (intake rate) is directly proportional to the risk level value (RQ). The RQ of all respondents was ≤ 1. All 70 respondents had a target hazard quotient (THQ) ≤ 1, and no respondents had a THQ value higher than 1. The standardization values issued by the US-EPA Agency and the Regulation of the Minister of Health of the Republic of Indonesia Number 32/2017 set the reference dose (RfD) value of surfactants and phosphate at 0.05 mL/L/d. Surfactant exposure can cause irritation to the skin and eyes and damage the skin's natural protective layer, while phosphate exposure is not directly toxic to humans in small concentrations. Risk management strategies are necessary to control phosphate and surfactant concentrations in drinking water so that they do not result in future non-carcinogenic risk effects.
The global water crisis is one of the greatest challenges of this century, with increased pressure on freshwater resources due to population growth, urbanization, and climate change. Amidst such growing demand, water pollution is a compounding, serious threat. Domestic, industrial, and agricultural waste, including chemicals such as surfactants and phosphates, contaminate freshwater sources, making them unsuitable for consumption or other uses.
Water is a fundamental chemical compound for human life and other living beings, whose function cannot be replaced by any other compound, as it is the main component both in plants and animals, including humans [1]. The need for clean water is directly proportional to the extent of water pollution caused by human activities, which ultimately impacts humans as well for example, the disposal of domestic household waste such as detergent waste, which can accumulate and become toxic [2]. Aquatic ecosystems are particularly affected by water pollution as their abiotic components mostly consist of water [3].
Greywater, which includes wastewater from activities such as washing clothes and dishes, differs from blackwater in that it typically contains fewer pathogens but still poses significant risks due to contaminants like detergents, phosphates, and surfactants [4]. Domestic wastewater is commonly contaminated with solid and liquid waste, containing microorganisms and being characterized by low dissolved oxygen values, high organic content, and high biological oxygen demand (BOD) [5].
High concentrations of detergent waste can disrupt aquatic biota, especially fish organs such as the liver, gills, and reproductive organs. The presence of detergent can increase nutrient levels, causing environmental problems [6]. Detergents are the largest source of phosphate, so the risk of pollution increases if laundry wastewater is released carelessly [7].
Detergent contamination is a widespread issue that affects water quality across the globe. The discharge of detergent-laden wastewater, especially from households, has been recognized as a major contributor to water pollution in both urban and rural areas [8]. The presence of excessive phosphate in water bodies can result in a process of nutrient enrichment known as eutrophication, a natural process in which waters gradually age and become more productive for biomass growth [9]. This increase in nutrient levels, especially phosphate and nitrate, triggers excessive algae growth. One of the main sources of these nutrients is detergent waste containing phosphate. Uncontrolled algae growth can cover the water surface, inhibit sunlight penetration, and reduce dissolved oxygen levels, ultimately threatening the lives of aquatic biota. This phenomenon has become a global concern because of its impact on water quality and aquatic biodiversity [10].
Globally, detergent formulations vary depending on the country. Several developed countries have imposed strict regulations to limit or ban the use of phosphates in detergents to reduce the risk of eutrophication [11]. As a result, detergent manufacturers have developed environmentally friendly formulas using biodegradable surfactants and natural ingredients. For example, in Indonesia, environmentally friendly liquid detergents using ethanol extracts of soapberry seeds (Sapindus rarak DC) combined with decyl glucoside and lauryl glucoside surfactants have been developed [12]. The challenges posed by detergent contamination are particularly relevant in developing countries, where wastewater treatment infrastructure may be lacking, leading to increased risks of pollution in groundwater and surface water sources.
A study by Subhan et al. [13] on Barrang Lompo Island, Makassar City, showed that the intake rate of detergent concentration exposure in raw water was directly proportional to the risk level value (RQ). The results of the intake rate from all respondents were still lower than the reference dose value, resulting in a RQ value lower than one (RQ ≤ 1).
A study by Wulandari et al. [14] in the Tukad Ayung River Basin (DAS), Bali, showed that the water phosphate content in the Tukad Ayung DAS ranged from 0.3 to 0.9 mg/L, still below the 1 mg/L threshold set by the PP No. 82/2001 class 3 Quality Standard. This was in line with results by Manurung et al. [15] on Kodingareng Lompo Island, Makassar City, where 11 drinking water wells were sampled. The highest detergent concentration found was 2.98 mg/L and the lowest was 0.005 mg/L, with an average of 0.696 mg/L.
Recent studies have shown that water pollution by surfactants and phosphates, especially from detergent waste, could pose significant health risks in the next five years [16]. Surfactants in detergents can cause skin, eye, and respiratory tract irritation, as well as disrupt soil structure and soil microorganisms, which ultimately affects the quality of groundwater for human consumption 17].
Detergents contain surfactants and phosphates, which are discharged directly into wastewater drains. This highlights the need to analyze the distribution of phosphates and surfactants in well water for the community in Lamangga Village, Baubau City.
The Lamangga sub-district is one of the sub-districts in Murhum District, Baubau City, Southeast Sulawesi Province, Indonesia (Figure 1). The pollution of well water in Lamangga Village is driven by several critical factors, including inadequate management of household waste disposal, insufficient wastewater treatment infrastructure, and high population density. Numerous households in this region discharge detergent waste generated from laundry activities directly into water channels without any prior filtration, thereby elevating the risk of hazardous chemicals permeating the soil and contaminating nearby water sources. Furthermore, the absence of domestic wastewater treatment facilities results in household waste entering the environment unfiltered, which exacerbates the pollution of well water for residents situated near drainage systems. The high density of settlements further exacerbates the situation, as the proximity between wells and pollution sources such as household drainage channels and sub-standard sanitation systems decreases, thereby increasing the likelihood of contamination. Collectively, these factors render the well water in Lamangga Village particularly susceptible to detergent pollution, posing significant health risks to the local community.
Lamangga Village was selected for this study due to its reliance on dug and drilled wells for drinking water, a source that is susceptible to contamination from nearby domestic wastewater. The community's exposure to potential water pollutants such as detergents makes it an ideal case for analyzing the impact of detergent contamination on public health.
Contamination of well water by detergents represents a significant environmental issue with risks to public health, particularly in regions where well water serves as the primary source for daily consumption and other needs. Detergents comprise chemical constituents, including surfactants and phosphates, which have the potential to pollute groundwater. This contamination can lead to alterations in the physical, chemical, and biological properties of water, thereby increasing the likelihood of health problems such as dermal irritations, gastrointestinal disorders, and endocrine disruptions. Consequently, this study is crucial for evaluating the extent of contamination, identifying associated health impacts, and assessing the risk levels faced by affected communities. Through the implementation of an environmental health risk analysis, this research aims to generate recommendations for the management of well water quality and to propose mitigation strategies that can alleviate the adverse effects of detergent contamination, ultimately safeguarding public health and promoting environmental sustainability.
This study uses an observational method (field observations and laboratory analysis of concentrated surfactant and phosphate levels in well water) with the Environmental Risk Quality Analysis (ARKL) approach. ARKL, also referred to as environmental health risk analysis, is a systematic approach employed to assess and evaluate the potential health risks associated with human exposure to environmental pollutants [18]. This analytical framework is utilized to ascertain the degree to which specific contaminants such as detergents, heavy metals, or various chemical compounds can adversely affect human health, taking into account the level of exposure and toxicological properties of the substances in question, namely in the form of a description of the variables studied. In this case, an environmental health risk analysis of phosphate and surfactants in well water for the Lamangga community in Baubau City was conducted.
The sampling methodology employed in the environmental health risk analysis concerning detergent contamination in well water utilized a purposive sampling approach. This involved the deliberate selection of wells with a heightened likelihood of detergent contamination, guided by specific environmental factors and community activities. The selected wells were predominantly situated in proximity to pollution sources, including residential areas where laundry waste is directly discharged into the environment, locations characterized by inadequate sanitation infrastructure, and regions with a high population density. Water samples from the wells were collected utilizing a grab sampling technique, which involved obtaining samples at designated times to accurately reflect the prevailing conditions at the moment of collection. Moreover, samples were obtained from multiple locations to enhance the reliability of the findings concerning the distribution of detergent pollution within the study area. Subsequently, the collected samples were subjected to laboratory analysis to quantify surfactant levels and other chemical indicators associated with detergent contamination, thereby facilitating an assessment of the potential health risks to the community.
In this study, the environmental health risk analysis (EHRA) was implemented in the following stages:
This stage aims to identify the hazards caused by the presence of surfactants and phosphates in the environment. Surfactants, which are often used in detergents, can cause skin, eye, and respiratory tract irritation and have bioaccumulation properties in aquatic organisms. Phosphates, on the other hand, trigger water eutrophication, which has an impact on ecosystems and water quality. In this stage, data on the concentration of chemicals in the environment (such as drinking water or surface water) were collected and compared with safe limits set by international standards, such as WHO and EPA IRIS.
This stage analyzes the relationship between the level of surfactant or phosphate exposure and the biological response it causes. The goal is to determine the threshold at which these chemicals begin to cause harmful effects. Toxicology data from laboratories or epidemiological studies are used to develop a dose-response curve, which includes values such as the no-observed adverse effect level (NOAEL) and the lowest observed adverse effect level (LOAEL). For example, high surfactant exposure can cause more severe irritation effects, while low exposure can be considered safe. The dose-response analysis is carried out based on the standardization values issued by the US-EPA Agency and the Regulation of the Minister of Health of the Republic of Indonesia Number 32/2017 concerning environmental health quality standards and air health requirements namely, the reference dose (RfD) value of surfactants and phosphate was set to 0.05 mL/L/d. The dose and concentration hereinafter referred to as RfD are safe reference values for the non-carcinogenic effects of a risk agent. The RfD value is the result of research from various sources, conducted directly on human objects, from extrapolations from the results of empirical research on human experimental animals related to environmental matrices, and from regulations or standards set for pollutant risk agents, especially in the context of detergent liquid waste.
Duration of exposure refers to the length of time a person or population is exposed to a particular chemical. Exposure can be acute (short term) or chronic (long term). For surfactants and phosphates, chronic exposure duration is usually more relevant because accumulation of chemicals in the ecosystem can lead to long-term impacts on human health. Factors such as frequency of use of contaminated water sources, water consumption habits, and vulnerable populations (children, elderly) are taken into account to determine the duration and intensity of exposure (USEPA).
(1) |
where ADD is the average daily dose (mL/L/day), C is the concentration of contaminant in water (mL/L), IngR is the ingestion rate (L/h), ET is the exposure time (h/d), EF is the frequency of exposure (d/year), ED is the duration (year), BW is the body weight (kg), and AT is the averaging time (d/year).
Risk characterization is the final stage that integrates the results of the previous three steps to provide an overview of the level of risk faced. In this stage, risk is calculated by comparing the actual chemical concentration (from the hazard identification stage) with the value considered safe (from the dose-response analysis). Risk quotient (RQ) is a ratio used in environmental health risk analysis to assess the potential impact of a hazardous substance on humans or ecosystems. RQ is calculated by comparing the level of exposure (exposure concentration, EC) of a substance with a threshold that is considered safe, such as the reference dose (RfD). Risk is usually expressed by RQ or by the probability of a particular health effect occurring. If RQ > 1, it means that the health risk is significant and requires mitigation measures.
(2) |
where RQ is the risk quotient (mL/L/day), and RfD is the reference dose (mL/L/).
The target hazard quotient (THQ) is an indicator used in health risk analysis to assess the potential non-carcinogenic risk of exposure to hazardous chemicals, such as heavy metals, pesticides, or other chemicals. THQ is calculated based on the comparison between the daily exposure dose and the reference value that is safe for humans.
(3) |
where THQ is the target hazard quotient (mL/L/day), fE is the frequency of exposure (d/year), Dt is the duration of exposure (year), R is the ingestion rate (L/h), Mc is the detergent concentration (mL/L), RfD is the reference dose (mL/L), Wb is the body weight (kg), and Tavg is the time average (d/year).
Ethical approval was obtained from the Ethics Committee of the Faculty of Public Health, Hasanuddin University, number 5741/UN4.14.1/TP.01.02/2023, consenting for environmental sample collection, data collection, and handling.
Table 1 shows that the majority of respondents were male (55.7%), aged 31–60 years (50.0%), had completed a college education (50.0%), and were self-employed (42.9%). Table 2 shows that the main source of drinking water used by the respondents was dug wells, and drinking water was processed by boiling.
Characteristics | n | % |
Gender | ||
Male | 39 | 55.7 |
Female | 31 | 43.4 |
Age | ||
0–14 years | 2 | 2.9 |
15–30 years | 25 | 35.7 |
31–60 years | 35 | 50.0 |
> 61 years | 8 | 11.4 |
Level of education | ||
Elementary school | 2 | 2.9 |
Middle school | 4 | 5.7 |
High school | 29 | 41.4 |
College | 35 | 50.0 |
Type of work | ||
Not yet/Not working | 16 | 22.9 |
Student/College Student | 6 | 8.6 |
Self-employed | 30 | 42.9 |
Civil servant | 18 | 25.7 |
*Source: Primary data, 2024. |
Characteristics | n | % |
Types of wells | ||
Dug well | 14 | 100 |
Drilled well | 0 | 0 |
Treatment | ||
Cooked | 70 | 100 |
Uncooked | 0 | 0 |
*Source: Primary data, 2024. |
Table 3 shows the laboratory examination of phosphate concentration in drinking water sources; a minimum value of 0.012 mg/L and a maximum value of 0.032 mg/L were recorded. Phosphate concentration did not exceed the maximum level permitted according to the Regulation of the Minister of Health of the Republic of Indonesia Number 32/2017 (0.05 mg/L). Surfactant concentration in drinking water sources ranged from 0.018 to 0.062 mg/L, exceeding the maximum level permitted according to the same regulation (0.05 mg/L). The distance of the well from the waste water drainage channel (WWDC) used by respondents varied from 5 to 10 m. Table 4 shows that all water sampling locations met the phosphate and surfactant standards.
Location | Distance (m) | Detergent concentration | Respondent amount | |
Phosphate | Surfactant | |||
1 | > 10 | 0.012 | 0.028 | 2 |
2 | > 10 | 0.022 | 0.035 | 9 |
3 | > 10 | 0.018 | 0.024 | 4 |
4 | 5–10 | 0.016 | 0.042 | 7 |
5 | > 10 | 0.028 | 0.018 | 5 |
6 | > 10 | 0.032 | 0.038 | 7 |
7 | > 10 | 0.014 | 0.05 | 5 |
8 | > 10 | 0.016 | 0.045 | 5 |
9 | > 10 | 0.020 | 0.032 | 5 |
10 | 5–10 | 0.014 | 0.062 | 3 |
11 | > 10 | 0.018 | 0.048 | 3 |
12 | 5–10 | 0.016 | 0.055 | 5 |
13 | > 10 | 0.022 | 0.027 | 5 |
14 | > 10 | 0.016 | 0.025 | 5 |
*Note: Distance waste water drainage channel to well water (m). Source: Primary Data, 2024. |
Location | Detergent concentration | Standard | |
Phosphate | Surfactant | ||
1 | 0.012 | 0.028 | 0.05 |
2 | 0.022 | 0.035 | |
3 | 0.018 | 0.024 | |
4 | 0.016 | 0.042 | |
5 | 0.028 | 0.018 | |
6 | 0.032 | 0.038 | |
7 | 0.014 | 0.05 | |
8 | 0.016 | 0.045 | |
9 | 0.020 | 0.032 | |
10 | 0.014 | 0.062 | |
11 | 0.018 | 0.048 | |
12 | 0.016 | 0.055 | |
13 | 0.022 | 0.027 | |
14 | 0.016 | 0.025 | |
*Source: Primary data, 2024. |
Figure 2 shows that detergent contamination of water sources at the study area is still categorized as safe and meets the requirements by the Minister of Health of the Republic of Indonesia Number 32/2017, which is 0.05 mg/L.
Table 5 shows that the age of respondents ranged from 11 to 75 years with a median of 33 years. Respondents' weight ranged from 13 to 130 kg with a median of 61 kg. Respondents' intake rate ranged from 1–2 L/d with a median of 2 L/d. Frequency of exposure (fE) was obtained by asking respondents about how often they left their place of residence each year in days. Extreme values, either very high or very low, can affect the average intake level or frequency of exposure, which ultimately affects the results of the risk analysis. All respondents stated that they consumed drinking water from wells every day.
Characteristics | Minimum | Maximum | Median |
Age (years) | 11 | 75 | 33 |
Body weight (kg) | 13 | 130 | 61 |
Intake rate (L/d) | 317 | 365 | 365 |
Exposure frequency (d/year) | 1 | 2 | 2 |
*Source: Primary Data, 2024. |
Based on Table 6, it can be seen that the intake values meet the standards. Figure 3 is a risk quotient (RQ) projection for exposure duration in Lamangga Village in 5–30 years. Mean RQ values range from 1×10-4 to 6×10-4, all being below 1, indicating that there is no significant risk. As exposure increases from 5 to 30 years, the RQ for surfactant/phosphate exposure shows a gradual increase but remains below the safety threshold (RQ < 1).
Detergent | Intake | Information | ||
Min | Max | Mean | ||
Surfactant | 2×10-4 | 6×10-3 | 1×10-3 | No health risk detected |
Phosphate | 1×10-4 | 5×10-3 | 7×10-4 | No health risk detected |
*Note: Surfactant RfD = 0.5 mg/kg/day; phosphate RfD = 0.5 mg/kg/day. Source: Primary data, 2024. |
Figure 4 shows the risk quotient (RQ) projection for 5–30 years of exposure duration in the Lamangga sub-district. The mean RQ value ranges from 2×10-4 to 1×10-3, not exceeding the RfD value. Since the RQ < 1, phosphate/surfactant exposure is considered to pose a low risk for adverse health effects. As exposure duration increases from 5 to 30 years, the RQ for surfactant/phosphate exposure shows a gradual increase but remains below the safety threshold.
Based on Table 7, as RQ ≤ 1, the level of exposure is deemed safe for both children and adults, meaning no carcinogenic risk from detergent exposure.
Age group | Risk level | Risk quotient (RQ) | |
Phosphate | Surfactant | ||
Child (n = 4) | > 1 | 0 (0%) | 0 (0%) |
≤ 1 | 2 (100%) | 2 (100%) | |
Adult (n = 68) | > 1 | 0 (0%) | 0 (0%) |
≤ 1 | 68 (100%) | 68 (100%) | |
*Source: Primary data, 2024. |
Figure 5 shows a projection of the target hazard quotient (THQ) in Lamangga Village for phosphate. The mean THQ value ranges from 2×10-7 to 1×10-6, not exceeding the RfD value. Such THQ < 1 means that the risk does not need to be controlled but all conditions must be maintained so that the THQ value does not surpass 1. As exposure duration increases from 5 to 30 years, the RQ for phosphate exposure shows a gradual increase but remains below the safety threshold.
Figure 6 shows the target hazard quotient (THQ) projection in Lamangga Village for surfactant. The mean THQ value ranges from 4×10-7 to 2×10-6, not exceeding the RfD value. All THQ values are below 1, indicating that there is no significant risk; still, an increase over time would have practical implications for long-term exposure and could affect public health. As exposure duration increases from 5 to 30 years, the RQ for surfactant exposure shows a gradual increase but remains below the safety threshold (THQ < 1).
In this study, a total of 70 respondents were interviewed: 33 female (44.3%) and 39 male (55.7%). The respondents' weight ranged from 13 to 130 kg with a median of 61 kg. Respondents ranged from 11 to 75 years, with a median of 33 years.
In risk analysis, body weight will affect the magnitude of the risk value. Theoretically, the heavier a person is, the less likely they are to experience health problems due to risk agents in drinking water sources. Human body weight reflects a person's nutritional status. Poor nutrition will affect a person's immune system and the occurrence of health problems. Based on toxicology studies, there is an inverse relationship between a person's body weight and toxic effects. When a person's body weight increases, the distribution of toxic substances or toxins in their body becomes wider, resulting in a decrease in the average amount of toxic substances per kilogram of body weight. In addition, body weight will affect the nutrients in the human body: People with ideal body weight will have appropriate nutrition so that the presence of metals in the body to replace nutrients will be blocked [19].
Ingestion rate is the amount of groundwater consumed by respondents per day and is used to calculate the risk level of detergents. The intake rate value was calculated based on the answers of respondents, who said they drank water according to their needs, drank when thirsty, drank after eating, or drank according to the recommendation of 8 glasses per day. We took the default value of 2 L/d for adults and 1 L/d for children [20].
The exposure unit for the frequency variable is expressed as days per year. The greater the frequency of exposure, the greater the level of risk due to exposure to surfactants and phosphate. If all respondents have an exposure frequency of 365 days, it means they are all likely to have a fairly high intake value (Ⅰ). The more often and longer an individual is in a polluted environment, the greater the number of risk agents entering the body and the greater the risk of health problems [21].
When calculating intake, it is understood that exposure time, exposure frequency, and exposure duration all have a direct relationship. This means that the longer and more often a person is exposed to a risk agent, the higher the intake value they receive. As a result, their risk of experiencing health problems due to exposure to risk agents also increases.
1) Phosphate
Large phosphate levels in water can be recognized by an unpleasant odor. Also, the color of the water changes to green and becomes increasingly cloudy. This is a sign of eutrophication, a process that reduces the aesthetic and recreational value of lakes, rivers, and streams and reduces the dissolved oxygen content in water, which can result in fish death and other ecosystem disturbances. Eutrophication also causes decreased water clarity and replacement of phytoplankton with blue-green algae that produce toxins that threaten human and animal health. Eutrophication increases water treatment costs (due to taste and odor problems caused by algae) [22]. Eutrophication in marine ecosystems is caused by detergents containing phosphate, which is also influenced by pH, salinity, and temperature. One form of eutrophication is algae blooming, water hyacinth growth, and algae bloom explosions [19].
The presence of phosphate compounds in water greatly affects the balance of aquatic ecosystems. If the phosphate levels in the water are low, the growth of organisms or aquatic plants will be inhibited. On the other hand, if phosphate levels are high, their growth will be unlimited, damaging the sustainability of the aquatic ecosystem [23].
2) Surfactant
Surfactant levels have been found in shallow groundwater. One origin is the direct continuous discharge of detergents into the wastewater drain for periods of tens of years. The age and depth of the well, the design of the waste water drainage channel, and the distance between the waste water drainage channel and the well all influence the surfactant value [13].
Anionic surfactant levels in the samples exceeded the normal limit (0.2 mg/L) set by the Government Regulation Number 82/2001. As such, this study evidences that the raw water used contains quite high amounts of detergents, which is feared to have an effect on human health [24]. This is in line with previous research by Larasati et al., [25] that presented data on methyil blue active benzene (MBAS) testing of detergent content in the Tapak River Estuary Waters of Semarang. The highest MBAS value was 0.026 mg/L at station 1, located in the river body. An MBAS value of 0.017 mg/L was found at station 2, also located in the river body. Stations 3–8 had MBAS results < 0.010 mg/L.
If the use of anionic surfactants exceeds the safe threshold value, negative impacts will occur, namely increased toxicity, a taste in the water, and reduced oxygen absorption, which has the potential to damage the gills and respiratory organs of fish and inhibit plant photosynthesis [24].
1) Phosphate
The RQ value, obtained by multiplying the intake and RfD phosphate values, ranged from 1.5×10-04 to 1.1×10-02 with an average of 1.3×10-03, showing that phosphate remains in the safe category. As the RQ of phosphate in the Lamangga Village (in real time) is < 1, the population is still safe or not at risk when consuming the water sources studied. The RQ result < 1 was also projected for the next 5–30 years, showing that the population in Lamangga Village will still be safe in consuming drinking water. If the concentration of phosphate in raw well water increases every year, the risk level for the community will also increase.
2) Surfactant
The RQ value, obtained by multiplying the intake and RfD surfactant values, ranged from 4.9×10-04 to 1.3×10-02 with an average of 2.2×10-03. This is still in the safe category. Variations in the RQ value can be caused by differences in surfactant levels in water, activity patterns, and respondent anthropometric data.
As the RQ of surfactant in the Lamangga Village (in real time) is < 1, the population is still safe or not at risk when consuming the water sources evaluated. The RQ result < 1 was also projected for the next 5–30 years, showing that the population in Lamangga Village will still be safe in consuming drinking water. If the concentration of surfactants in raw well water increases every year, the risk level for the community will also increase.
In this study, risk assessment examined exposure to detergents through certain exposure routes (oral/ingestion) by considering activity patterns and human anthropometric measurements. Exposure was compared with reference dose values (detergent RfD = 0.5 mg/kg/day) to estimate the magnitude of future risk. This is in line with research by Subhan et al. [13]. The risk level of detergent residue consumption in drinking water was highest for respondents with an intake of 0.073 mg/kg/day (RQ = 0.146), while the lowest risk level was found for respondents with an intake of 0 mg/kg/day (RQ = 0.0). The risk level value of all respondents was still below one (RQ ≤ 1), classified as safe or not at risk. This is in contrast with the study by Manurung et al. [15]. According to the US-EPA (2006), the RfD for detergents through oral exposure (ingestion) is 0.5 mg/kg/day. The risk level for non-carcinogenic diseases was calculated using Eq 2, and a value of 1.0551 was found. Detergent residues in drinking water in a concentration of 1.28088 mg/L are classified as unsafe or non-carcinogenic risks to public health.
1) Surfactant
Target hazard quotient (THQ) assessment for surfactants is critical in understanding potential ecological and human health risks. Surfactants, which are widely used in a variety of industrial and consumer products, can pose significant environmental hazards, especially when they enter aquatic ecosystems. THQ is a quantitative measure that helps evaluate the risks associated with exposure to these chemicals, particularly in terms of their toxicity and bioaccumulation potential. Surfactants can exhibit varying degrees of toxicity depending on their chemical structure and the specific biological systems they affect. For example, studies have shown that certain biosurfactants, such as rhamnolipids, although beneficial in agricultural and industrial applications, can still pose risks to aquatic organisms if not properly managed [26]. Surfactant toxicity can be assessed using a variety of bioassays, including those involving bacteria, fungi, and aquatic invertebrates, which provide insight into their ecological impacts [27]. In addition, the presence of surfactants in wastewater can cause cumulative toxic effects, requiring a comprehensive risk assessment that takes into account individual and mixture toxicities [28].
The environmental persistence and bioaccumulation of surfactants are also important factors in calculating THQ. For example, quaternary ammonium compounds (QACs), commonly found in personal care products, have been identified as emerging contaminants in urban sediments, raising concerns about their long-term ecological effects [29]. Studies have shown that these compounds can be toxic to a variety of nontarget organisms, including fish and invertebrates, highlighting the importance of evaluating their environmental fate and bioaccumulation potential [30]. Furthermore, the interaction of surfactants with other pollutants can exacerbate their toxic effects, complicating risk assessment [31].
In addition to ecological risks, human health implications of surfactant exposure should also be considered. Surfactants used in agricultural formulations, such as polyethoxylated amines, have been shown to contribute significantly to herbicide toxicity, affecting not only target pests but also nontarget species, including beneficial insects [32]. The lack of a regulatory framework addressing surfactant toxicity further complicates the risk assessment landscape [33].
2) Phosphate
The THQ for phosphate in detergents is an important metric for assessing potential environmental and health impacts associated with the use of these substances. Phosphate, commonly found in laundry detergents, has been linked to a variety of ecological problems, particularly eutrophication in aquatic systems, which can lead to harmful algal blooms and subsequent toxicity to aquatic life and humans [34]. Regulation of phosphate in detergents has become an important focus for environmental health, leading to initiatives to limit its concentration in products to reduce these risks.
Recent studies have shown that the THQ for phosphate in detergents can vary significantly based on the formulation and concentration of phosphate used. For example, Hossain et al. highlighted that certain detergents available in Bangladesh contain varying levels of phosphate, with some brands showing very high concentrations that can cause environmental pollution [35]. This variability underscores the need for strict regulation and consumer awareness of phosphate content in detergents.
In addition, the introduction of phosphate-free alternatives is a response to growing concerns about phosphate pollution. Khamidulina and Proskurina discussed the global shift toward phosphate-free detergents, emphasizing the importance of a regulatory framework that limits phosphate content to levels that do not pose a risk to human health or the environment [34]. Proposed regulations, such as those by Rospotrebnadzor, suggest a maximum permissible phosphate concentration of 0.5% in synthetic detergents, reflecting a proactive approach to reducing potential hazards associated with phosphate use [34].
Assessing the hazard ratio for phosphate in detergents also involves understanding its interactions with other components in the environment. For example, the presence of surfactants and other pollutants in wastewater from detergent use can exacerbate the risks associated with phosphate [36].
1) Phosphate exposure risk management
Phosphate contamination risk management in well water is an important issue related to public health. This contamination can occur due to various factors, including excessive use of phosphate fertilizers in agriculture, industrial waste, and domestic waste disposal. Therefore, the implementation of effective risk management is essential to protect public health and the environment.
The implementation of risk management based on international standards such as ISO 31000 can help in identifying, analyzing, and managing risks associated with phosphate contamination. According to Setiawan and Bandung [37], the implementation of risk management can improve the operational performance and competitive value of an organization by identifying interrelated sources of risk [37]. The risk management process proposed by Yoewono and Prasetyo [38] includes steps ranging from determining the scope to analyzing and treating risks, which are very relevant in the context of phosphate contamination risk management.
In the context of public health, it is important to understand the impact of phosphate contamination. Studies have shown that exposure to high levels of phosphate can cause a variety of health problems, although there is no direct evidence that phosphate specifically causes respiratory and gastrointestinal disorders [39].
Therefore, effective risk management should include clear mitigation strategies, including monitoring water quality and educating the public about the dangers of contamination. Risk analysis methods such as the Ishikawa Diagram and Failure Mode Effect Analysis (FMEA) can also be applied to understand the causes and impacts of phosphate contamination [40]. By using these methods, authorities can identify critical points in the water supply chain that are potentially contaminated and formulate appropriate preventive measures.
Furthermore, research by Trimadya et al. [39] showed that implementing a structured risk management system in the food supply chain can help reduce the risk of contamination, which can also be applied in the context of well water. Thus, a systematic approach to risk management will not only protect public health but also increase public confidence in the quality of the water used.
2) Surfactant
ISO 31000 emphasizes the importance of contextualization, risk identification, risk analysis, and risk management, all of which are relevant in dealing with potential surfactant contamination. Surfactant contamination can occur through various sources, including industrial waste and the use of poorly managed cleaning products [31]. Therefore, it is important to conduct a comprehensive risk analysis to identify sources of contamination and their impact on well water quality. FMEA, for example, can help identify potential failures in a water management system and prioritize risks that need to be addressed [40].
Transparent disclosure of risk management is also important in this context [41]. Companies or organizations involved in water resource management must prepare clear reports on the risks faced and the steps taken to manage them. This not only increases public trust but also helps in better decision-making by stakeholders [42]. Thus, effective risk management focuses not only on risk mitigation but also on clear communication of the risks and strategies implemented.
In its implementation, training and strengthening of human resource (HR) capacity are also key factors in the success of risk management. Trained HR will be better able to recognize risks and implement the necessary mitigation steps [43]. Therefore, training programs that focus on risk management and awareness of well water contamination should be an integral part of a broader risk management strategy.
Limitations:
1) The number and distribution of well water samples tested may be limited, so they cannot represent the entire population of wells in the study area. This may reduce the generalizability of the study results.
2) The level of detergent contamination in well water may fluctuate due to weather factors, changes in land use, and domestic activities of the community. Measurements taken only at one time or a certain season may not describe the broader situation
3) The results of the study may only apply to a specific region or condition and cannot be directly applied to other regions with different environmental or social characteristics.
4) It is difficult to control for other variables that may affect public health, such as hygiene habits, access to clean water, and health services, so the results of the study may be influenced by unmeasured variables.
The analysis of environmental health risks associated with detergent contamination in well water highlights the significant implications for human health and environmental sustainability. Detergents, containing surfactants and phosphates as key components, pose potential risks when they infiltrate well water through domestic, industrial, or agricultural wastewater. Surfactants can lead to skin irritation, respiratory problems, and damage to aquatic ecosystems, while phosphates contribute to eutrophication, compromising water quality and ecosystem balance.
The average concentration of phosphate in drinking water sources at 14 sampling points was 0.020 mg/L and that of surfactant was 0.39 mg/L. In samples No. 10 and 12, the surfactant value was 0.062 mg/L and 0.055 mg/L, respectively. These values exceed the quality standard set by the Minister of Health of the Republic of Indonesia Number 32/2017. The intake value (the amount of risk agent concentration consumed) varied greatly. The intake value was calculated for the duration of real-time exposure of 5–30 years and is directly proportional to the risk level value (RQ). The RQ value was lower than one (RQ ≤ 1). The THQ measurement used several variables similar to the RQ measurement; THQ was always less than or equal to 1, and no respondents presented a THQ greater than 1.
This study underscores the importance of evaluating risk factors through systematic approaches such as hazard identification, dose-response analysis, exposure duration assessment, and risk characterization. Elevated levels of surfactants and phosphates in well water may exceed safe thresholds established by organizations like WHO, increasing the likelihood of adverse health outcomes, particularly with prolonged exposure.
Our results showed that no individual samples were at risk of negative health consequences. Health risk management in the Lamangga sub-district regarding phosphate and surfactants in drinking water does not need to be carried out because the risk level value was classified as safe. On the other hand, the concentration of phosphates and surfactants in raw water for drinking water needs to be considered so that it does not increase and cause non-carcinogenic risk effects in the future.
To mitigate detergent pollution in the environment, a multifaceted approach is required, encompassing regulatory measures, technological advancements, and shifts in societal behavior. Governmental bodies must enact policies aimed at restricting or prohibiting the use of phosphates in detergents, while simultaneously promoting the adoption of more sustainable materials. The detergent industry should prioritize the development of surfactant-based formulations that are readily biodegradable and exhibit minimal ecological impact. Furthermore, enhancing the capabilities of both household and industrial wastewater treatment facilities is essential to prevent the direct contamination of water sources with detergent waste. Community education is also vital, focusing on the appropriate usage of detergents, the selection of more environmentally benign products, and the implementation of efficient washing practices, such as the incorporation of filtration technologies prior to wastewater disposal. By undertaking these comprehensive measures, it is possible to significantly reduce detergent pollution, thereby safeguarding both environmental integrity and public health.
The authors declare they have not used Artificial Intelligence (AI) tools in the creation of this article.
The authors would like to thank the Department of Environmental Health and the Research Ethics Commission of Hasanuddin University for processing documents and obtaining research permits.
The authors declare that there is no conflict of interest in this study.
[1] |
Anand AJ, ChuaMC, Khoo SH, et al. (2017) Early discharge planning in preterm low birth weight babies: A quality improvement project. Proc Singap Healthc 26: 98–101. https://doi.org/10.1177/2010105816676827 doi: 10.1177/2010105816676827
![]() |
[2] |
Lestari AD (2022) Pengaruh pencemaran limbah detergen terhadap ekosistem perairan. Jurnal Sains Indonesia 3: 24–36. https://doi.org/10.59897/jsi.v3i1.72 doi: 10.59897/jsi.v3i1.72
![]() |
[3] |
Naufal BS, A'yun DQ (2024) Analisis dampak pencemaran tanah akibat limbah deterjen terhadap lingkungan hidup masyarakat di daerah pedesaan. Stud Res J 2: 231–235. https://doi.org/10.55606/srjyappi.v2i3.1320 doi: 10.55606/srjyappi.v2i3.1320
![]() |
[4] |
Apriyani N (2017) Reduction of surfactant and sulfate levels in laundry waste. Environ Eng Sci Media 2: 37–44. https://doi.org/10.33084/mitl.v2i1.132 doi: 10.33084/mitl.v2i1.132
![]() |
[5] | Al Kholif M (2020) Domestic waste processing, Scopindo: Media Pustaka. |
[6] |
Sulistia S, Septisya AC (2020) Analysis of domestic office wastewater quality. J Environ Eng 12: 41–57. https://doi.org/10.29122/jrl.v12i1.3658 doi: 10.29122/jrl.v12i1.3658
![]() |
[7] |
Pungut P, Al Kholif M, Pratiwi WDI (2021) Reduction of chemical oxygen demand (COD) and phosphate levels in laundry waste using adsorption method. J Environ Sci Te 13: 155–165. https://doi.org/10.20885/jstl.vol13.iss2.art6 doi: 10.20885/jstl.vol13.iss2.art6
![]() |
[8] |
Mukherjee S, Edmunds MBS, Lei X, et al. (2010) Steric acid delivery to corneum from a mild and moisturizing cleanser. J Cosmet Dermatol 3: 202–210. https://doi.org/10.1111/j.1473-2165.2010.00510.x doi: 10.1111/j.1473-2165.2010.00510.x
![]() |
[9] | Effendi H (2003) Water quality assessment: For aquatic resources and environmental management, Kanisius. |
[10] |
Nurrosyidah IH, Putri EN, Klau ICS, et al. (2023) Formulasi Deterjen Eco-Friendly Ekstrak Etanol Biji Buah Lerak (Sapindus rarak DC) Kombinasi Surfaktan Decyl Glucoside dan Lauryl Glucoside. Clin Pharm Anal Pharm Community J 2: 84–91. https://doi.org/10.30651/cam.v2i1.17955 doi: 10.30651/cam.v2i1.17955
![]() |
[11] |
Saleh R, Daud A, Ishak H, et al. (2023) Spatial distribution of microplastic contamination in blood clams (Anadara granosa) on the Jeneponto coast, South Sulawesi. Pharm J 15: 680–690. https://doi.org/10.5530/pj.2023.15.137 doi: 10.5530/pj.2023.15.137
![]() |
[12] |
Suryana T, Makhroji M, Suryani S (2024) Pembuatan Detergen Ekonomis dan Ramah Lingkungan untuk Meningkatkan Pengetahuan Ibu PKK di Desa Karangbenda. Jurnal Kreativitas Pengabdian Kepada Masyarakat 7: 530–540. https://doi.org/10.38204/darmaabdikarya.v3i2.2154 doi: 10.38204/darmaabdikarya.v3i2.2154
![]() |
[13] | Subhan M, Birawida AB, Hatta M (2020) Health risk analysis of detergent concentration in raw water for drinking water on the community in Barrang Lompo Island, Makassar City. J Akademika 17: 25–30. |
[14] | Wulandari N, Perwira IY, Ernawati NM (2021) Phosphate content profile in water in the Tukad Ayung River Basin (DAS), Bali. Jurnal Harian Regional 115: 108–115. Available from: https://jurnal.harianregional.com/ctas/id-59708. |
[15] |
Manurung MB, Edhi T, Soesilo B, et al. (2022) Health risk analysis of detergent contamination in communities on Kodingareng Lompo Island, Makassar City. Journal Presipitasi 19: 426–435. https://doi.org/10.14710/presipitasi.v19i2.426–435 doi: 10.14710/presipitasi.v19i2.426–435
![]() |
[16] |
Birawida AB, Ibrahim E, Mallongi A, et al. (2021) Clean water supply vulnerability model for improving the quality of public health (environmental health perspective): A case in Spermonde islands, Makassar Indonesia. Gac Sanit 35: S601–S603. https://doi.org/10.1016/j.gaceta.2021.10.095 doi: 10.1016/j.gaceta.2021.10.095
![]() |
[17] | Putri BH, Kurniawan A, Pi S (2021) Kajian Literatur Aplikasi Bakteri Dalam Mendegradasi Surfaktan di Lingkungan Perairan, Diss. Universitas Brawijaya. |
[18] |
Kasim S, Daud A, Birawida AB, et al. (2023) Analysis of environmental health risks from exposure to polyethylene terephthalate microplastics in refilled drinking. Glob J Environ Sci M 9: 301–318. https://doi.org/10.22034/GJESM.2023.09.SI.17 doi: 10.22034/GJESM.2023.09.SI.17
![]() |
[19] |
Hidayat H, La Taha LT, Dewi BS (2022) Risk analysis of lead (Pb) exposure in shellfish in the coastal area of Galesong Village, Palalakkang District, Galesong Regency, Takalar Regency. Sulolipu Media Commun Acad Community 22: 219–225. https://doi.org/10.32382/sulolipu.v22i2.2902 doi: 10.32382/sulolipu.v22i2.2902
![]() |
[20] | Dirjen P2PL (2012) Guidance on environmental health risk analysis. |
[21] |
Diyanah KCS (2022) Risk analysis of dust exposure (total suspended particulate) in Packer Unit PT. X. Environ Health J 9: 100–110. https://doi.org/10.20473/jkl.v9i1.2017.100-101 doi: 10.20473/jkl.v9i1.2017.100-101
![]() |
[22] | Badamasi H, Yaro MN, Ibrahim A, et al. (2019) Impacts of phosphates on water quality and aquatic life. Chem Res J 4: 124–133. Available from: https://chemrj.org/download/vol-4-iss-3-2019/chemrj-2019-04-03-124-133.pdf. |
[23] |
Sutamihardja R, Azizah M, Hardini Y (2018) Study of phosphate compound dynamics in the water quality of the upper Ciliwung River in Bogor City. J Nat Sci 8: 43–49. https://doi.org/10.31938/jsn.v8i1.114 doi: 10.31938/jsn.v8i1.114
![]() |
[24] | Fajriah N, Alawiyah T, Wusko IU (2020) Analysis of anionic surfactant levels (detergents) in Barito River water using visible spectrophotometry method. J Pharm Care Sci 1: 55–61. Available from: https://ejurnal.unism.ac.id/index.php/jpcs/article/view/22. |
[25] |
Larasati NN, Wulandari SY, Maslukah L, et al. (2021) Detergent pollutant content and water quality in the Tapak River Estuary, Semarang. Indones J Oceanography 3: 22–29. https://doi.org/10.14710/ijoce.v3i1.9470 doi: 10.14710/ijoce.v3i1.9470
![]() |
[26] |
Invally K, Ju L (2017) Biolytic effect of rhamnolipid biosurfactant and dodecyl sulfate against phagotrophic alga Ochromonas danica. J Surfactants Deterg 20: 1161–1171. https://doi.org/10.1007/s11743-017-2005-1 doi: 10.1007/s11743-017-2005-1
![]() |
[27] |
Colonna WJ, Martí ME, Nyman JA, et al. (2016). Hemolysis as a rapid screening technique for assessing the toxicity of native surfactin and a genetically engineered derivative. Environ Prog Sustain 36: 505–510. https://doi.org/10.1002/ep.12444 doi: 10.1002/ep.12444
![]() |
[28] |
Ríos F, Lechuga M, Guarnido IL, et al. (2023) Antagonistic toxic effects of surfactants mixtures to Pseudomonas putida and marine microalgae Phaeodactylum tricornutum. Toxics 11: 344–350. https://doi.org/10.3390/toxics11040344 doi: 10.3390/toxics11040344
![]() |
[29] |
Martín PAL, Li X, Bopp RF, et al. (2010) Occurrence of alkyltrimethylammonium compounds in urban estuarine sediments: Behentrimonium as a new emerging contaminant. Environ Sci Tech 44: 7569–7575. https://doi.org/10.1021/es101169a doi: 10.1021/es101169a
![]() |
[30] |
García MT, Kaczerewska O, Ribosa I, et al. (2016) Biodegradability and aquatic toxicity of quaternary ammonium-based gemini surfactants: Effect of the spacer on their ecological properties. Chemosphere 154: 155–160. https://doi.org/10.1016/j.chemosphere.2016.03.109 doi: 10.1016/j.chemosphere.2016.03.109
![]() |
[31] |
Mesnage R, Antoniou M (2018) Ignoring adjuvant toxicity falsifies the safety profile of commercial pesticides. Front Public Health 5: 43–50. https://doi.org/10.3389/fpubh.2017.00361 doi: 10.3389/fpubh.2017.00361
![]() |
[32] |
Mikó Z, Hettyey A (2023) Toxicity of POEA-containing glyphosate-based herbicides to amphibians is mainly due to the surfactant, not to the active ingredient. Ecotoxicology 32: 150–159. https://doi.org/10.1007/s10646-023-02626-x doi: 10.1007/s10646-023-02626-x
![]() |
[33] |
Collins JK, Jackson JM (2022) Application of a screening-level pollinator risk assessment framework to trisiloxane polyether surfactants. Environ Toxicol Chem 41: 3084–3094. https://doi.org/10.1002/etc.5479 doi: 10.1002/etc.5479
![]() |
[34] |
Khamidulina Kh, Proskurina AS (2020) About measures to reduce the risk of cyanotoxins exposure to the health of population by regulating phosphates in synthetic detergents. Toxicol Rev 3: 3–8. https://doi.org/10.36946/0869-7922-2020-3-3-8 doi: 10.36946/0869-7922-2020-3-3-8
![]() |
[35] |
Badmus SO, Amusa HK, Oyehan TA (2021) Environmental risks and toxicity of surfactants: Overview of analysis, assessment, and remediation techniques. Environ Sci Pollut R 28: 62085–62104. https://doi.org/10.1007/s11356-021-16483-w doi: 10.1007/s11356-021-16483-w
![]() |
[36] | Anpilova Y, Yakovliev Y, Trofymchuk O, et al. (2021). Environmental hazards of the Donbas hydrosphere at the final stage of the coal mines flooding, In: Zaporozhets A. Eds., Systems, Decision and Control in Energy Ⅲ, Cham: Springer, 345–350. https://doi.org/10.1007/978-3-030-87675-3_19 |
[37] |
Setiawan I, Bandung PN (2022) Development of a prototype of an ISO 31000-based risk management application. Matrix: J Tech Inform M 10: 26–33. https://doi.org/10.31940/matrix.v10i1.1817 doi: 10.31940/matrix.v10i1.1817
![]() |
[38] |
Yoewono JO, Prasetyo AH (2022) Design and risk management process at PT Surya Harmonica Cita. Estuary Econ Business J 6: 56–62. https://doi.org/10.24912/jmieb.v6i1.12207 doi: 10.24912/jmieb.v6i1.12207
![]() |
[39] |
Trimadya NM, Hardjomidjojo H, Anggraeni E (2018) Contamination risk management system in the food supply chain (case study: pasteurized milk). J Agric Ind Technol 28: 162–170. https://doi.org/10.24961/j.tek.ind.pert.2018.28.2.162 doi: 10.24961/j.tek.ind.pert.2018.28.2.162
![]() |
[40] |
Hisprastin Y, Musfiroh I (2020) Ishikawa diagram and failure mode effect analysis (FMEA) as methods frequently used in quality risk management in industry. Farmasetika Magazine 6: 1–10. https://doi.org/10.24198/mfarmasetika.v6i1.27106 doi: 10.24198/mfarmasetika.v6i1.27106
![]() |
[41] |
Fachrezi MI (2021) Information technology asset security risk management using ISO 31000:2018 Salatiga City Communication and Information Service. J Inform Eng Inform Syst 8: 764–773. https://doi.org/10.35957/jatisi.v8i2.789 doi: 10.35957/jatisi.v8i2.789
![]() |
[42] |
Wardoyo DU, Ramdhani ND, Ramadhan R (2022) The influence of solvency, institutional ownership, and independent commissioners on risk management disclosure. J-Ceki: Jurnal Cendekia Ilmiah 1: 57–64. https://doi.org/10.56799/jceki.v1i2.128 doi: 10.56799/jceki.v1i2.128
![]() |
[43] |
Mudrifah M, Wisyastuti A (2021) Strengthening the characteristics of human resources in the implementation of risk-based management at Lazis Muhammadiyah (Lazismu) Malang Regency. Indones National Serv J 2: 19–27. https://doi.org/10.35870/jpni.v2i1.26 doi: 10.35870/jpni.v2i1.26
![]() |
Characteristics | n | % |
Gender | ||
Male | 39 | 55.7 |
Female | 31 | 43.4 |
Age | ||
0–14 years | 2 | 2.9 |
15–30 years | 25 | 35.7 |
31–60 years | 35 | 50.0 |
> 61 years | 8 | 11.4 |
Level of education | ||
Elementary school | 2 | 2.9 |
Middle school | 4 | 5.7 |
High school | 29 | 41.4 |
College | 35 | 50.0 |
Type of work | ||
Not yet/Not working | 16 | 22.9 |
Student/College Student | 6 | 8.6 |
Self-employed | 30 | 42.9 |
Civil servant | 18 | 25.7 |
*Source: Primary data, 2024. |
Characteristics | n | % |
Types of wells | ||
Dug well | 14 | 100 |
Drilled well | 0 | 0 |
Treatment | ||
Cooked | 70 | 100 |
Uncooked | 0 | 0 |
*Source: Primary data, 2024. |
Location | Distance (m) | Detergent concentration | Respondent amount | |
Phosphate | Surfactant | |||
1 | > 10 | 0.012 | 0.028 | 2 |
2 | > 10 | 0.022 | 0.035 | 9 |
3 | > 10 | 0.018 | 0.024 | 4 |
4 | 5–10 | 0.016 | 0.042 | 7 |
5 | > 10 | 0.028 | 0.018 | 5 |
6 | > 10 | 0.032 | 0.038 | 7 |
7 | > 10 | 0.014 | 0.05 | 5 |
8 | > 10 | 0.016 | 0.045 | 5 |
9 | > 10 | 0.020 | 0.032 | 5 |
10 | 5–10 | 0.014 | 0.062 | 3 |
11 | > 10 | 0.018 | 0.048 | 3 |
12 | 5–10 | 0.016 | 0.055 | 5 |
13 | > 10 | 0.022 | 0.027 | 5 |
14 | > 10 | 0.016 | 0.025 | 5 |
*Note: Distance waste water drainage channel to well water (m). Source: Primary Data, 2024. |
Location | Detergent concentration | Standard | |
Phosphate | Surfactant | ||
1 | 0.012 | 0.028 | 0.05 |
2 | 0.022 | 0.035 | |
3 | 0.018 | 0.024 | |
4 | 0.016 | 0.042 | |
5 | 0.028 | 0.018 | |
6 | 0.032 | 0.038 | |
7 | 0.014 | 0.05 | |
8 | 0.016 | 0.045 | |
9 | 0.020 | 0.032 | |
10 | 0.014 | 0.062 | |
11 | 0.018 | 0.048 | |
12 | 0.016 | 0.055 | |
13 | 0.022 | 0.027 | |
14 | 0.016 | 0.025 | |
*Source: Primary data, 2024. |
Characteristics | Minimum | Maximum | Median |
Age (years) | 11 | 75 | 33 |
Body weight (kg) | 13 | 130 | 61 |
Intake rate (L/d) | 317 | 365 | 365 |
Exposure frequency (d/year) | 1 | 2 | 2 |
*Source: Primary Data, 2024. |
Detergent | Intake | Information | ||
Min | Max | Mean | ||
Surfactant | 2×10-4 | 6×10-3 | 1×10-3 | No health risk detected |
Phosphate | 1×10-4 | 5×10-3 | 7×10-4 | No health risk detected |
*Note: Surfactant RfD = 0.5 mg/kg/day; phosphate RfD = 0.5 mg/kg/day. Source: Primary data, 2024. |
Age group | Risk level | Risk quotient (RQ) | |
Phosphate | Surfactant | ||
Child (n = 4) | > 1 | 0 (0%) | 0 (0%) |
≤ 1 | 2 (100%) | 2 (100%) | |
Adult (n = 68) | > 1 | 0 (0%) | 0 (0%) |
≤ 1 | 68 (100%) | 68 (100%) | |
*Source: Primary data, 2024. |
Characteristics | n | % |
Gender | ||
Male | 39 | 55.7 |
Female | 31 | 43.4 |
Age | ||
0–14 years | 2 | 2.9 |
15–30 years | 25 | 35.7 |
31–60 years | 35 | 50.0 |
> 61 years | 8 | 11.4 |
Level of education | ||
Elementary school | 2 | 2.9 |
Middle school | 4 | 5.7 |
High school | 29 | 41.4 |
College | 35 | 50.0 |
Type of work | ||
Not yet/Not working | 16 | 22.9 |
Student/College Student | 6 | 8.6 |
Self-employed | 30 | 42.9 |
Civil servant | 18 | 25.7 |
*Source: Primary data, 2024. |
Characteristics | n | % |
Types of wells | ||
Dug well | 14 | 100 |
Drilled well | 0 | 0 |
Treatment | ||
Cooked | 70 | 100 |
Uncooked | 0 | 0 |
*Source: Primary data, 2024. |
Location | Distance (m) | Detergent concentration | Respondent amount | |
Phosphate | Surfactant | |||
1 | > 10 | 0.012 | 0.028 | 2 |
2 | > 10 | 0.022 | 0.035 | 9 |
3 | > 10 | 0.018 | 0.024 | 4 |
4 | 5–10 | 0.016 | 0.042 | 7 |
5 | > 10 | 0.028 | 0.018 | 5 |
6 | > 10 | 0.032 | 0.038 | 7 |
7 | > 10 | 0.014 | 0.05 | 5 |
8 | > 10 | 0.016 | 0.045 | 5 |
9 | > 10 | 0.020 | 0.032 | 5 |
10 | 5–10 | 0.014 | 0.062 | 3 |
11 | > 10 | 0.018 | 0.048 | 3 |
12 | 5–10 | 0.016 | 0.055 | 5 |
13 | > 10 | 0.022 | 0.027 | 5 |
14 | > 10 | 0.016 | 0.025 | 5 |
*Note: Distance waste water drainage channel to well water (m). Source: Primary Data, 2024. |
Location | Detergent concentration | Standard | |
Phosphate | Surfactant | ||
1 | 0.012 | 0.028 | 0.05 |
2 | 0.022 | 0.035 | |
3 | 0.018 | 0.024 | |
4 | 0.016 | 0.042 | |
5 | 0.028 | 0.018 | |
6 | 0.032 | 0.038 | |
7 | 0.014 | 0.05 | |
8 | 0.016 | 0.045 | |
9 | 0.020 | 0.032 | |
10 | 0.014 | 0.062 | |
11 | 0.018 | 0.048 | |
12 | 0.016 | 0.055 | |
13 | 0.022 | 0.027 | |
14 | 0.016 | 0.025 | |
*Source: Primary data, 2024. |
Characteristics | Minimum | Maximum | Median |
Age (years) | 11 | 75 | 33 |
Body weight (kg) | 13 | 130 | 61 |
Intake rate (L/d) | 317 | 365 | 365 |
Exposure frequency (d/year) | 1 | 2 | 2 |
*Source: Primary Data, 2024. |
Detergent | Intake | Information | ||
Min | Max | Mean | ||
Surfactant | 2×10-4 | 6×10-3 | 1×10-3 | No health risk detected |
Phosphate | 1×10-4 | 5×10-3 | 7×10-4 | No health risk detected |
*Note: Surfactant RfD = 0.5 mg/kg/day; phosphate RfD = 0.5 mg/kg/day. Source: Primary data, 2024. |
Age group | Risk level | Risk quotient (RQ) | |
Phosphate | Surfactant | ||
Child (n = 4) | > 1 | 0 (0%) | 0 (0%) |
≤ 1 | 2 (100%) | 2 (100%) | |
Adult (n = 68) | > 1 | 0 (0%) | 0 (0%) |
≤ 1 | 68 (100%) | 68 (100%) | |
*Source: Primary data, 2024. |