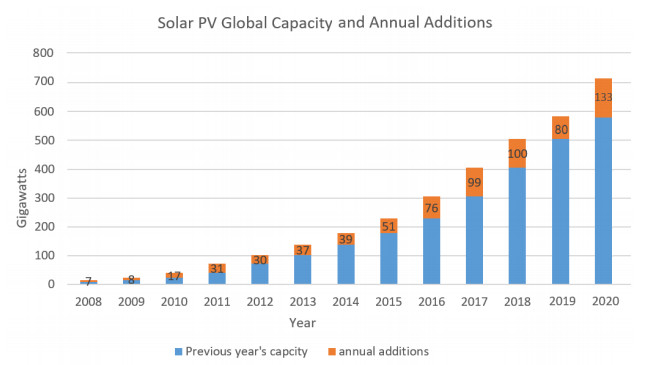
In this work, the use of solar thermal energy in the Nordic Countries, especially in Denmark, is studied. Renewable energies, such as solar, are an important ally in the fight against Climate Change, with more countries betting on them. In the past, it may have been believed that the Nordic Region does not have enough solar radiation for solar energy to be profitable, but changes in the climate and technology have proven this statement to be wrong. The case of Denmark is a clear example, which has become a leading country when it comes to solar thermal energy. A study of the current Danish solar thermal market is conducted in this project in order to understand how this country is making the most out of this energy source. Three different plants are studied (Silkeborg, Vollerup and Gr?sten) and compared to those of other Nordic Countries (Akershus from Norway and H?meenlinna from Finland). It was found that the main difference between them is the size of the Photovoltaic (PV) parks. Whilst Denmark specialised in large-scale plants with a two-phase building process, the other countries are only using small-scale installations. After a deeper understanding of the Danish energy market, it is concluded that there is a solar thermal energy policy that allows its exploitation. Furthermore, an increase in the level of solar radiation in Denmark over the last decade has led Danish experts to believe that solar energy in the Nordic Countries is going to be even more profitable in the following years. It is believed that Denmark will continue its current expansion into this market and also that the other Nordic countries will soon enough join.
Citation: Gemma Graugés Graell, George Xydis. Solar Thermal in the Nordics. A Belated Boom for All or Not?[J]. AIMS Energy, 2022, 10(1): 69-86. doi: 10.3934/energy.2022005
[1] | Hayder S. Al-Madhhachi, Ahmed M. Ajeena, Nihad A. Al-Bughaebi . Dynamic simulation and energy analysis of forced circulation solar thermal system in two various climate cities in Iraq. AIMS Energy, 2021, 9(1): 138-149. doi: 10.3934/energy.2021008 |
[2] | Laveet Kumar, Jahanzaib Soomro, Hafeez Khoharo, Mamdouh El Haj Assad . A comprehensive review of solar thermal desalination technologies for freshwater production. AIMS Energy, 2023, 11(2): 293-318. doi: 10.3934/energy.2023016 |
[3] | Joanna McFarlane, Jason Richard Bell, David K. Felde, Robert A. Joseph III, A. Lou Qualls, Samuel Paul Weaver . Performance and Thermal Stability of a Polyaromatic Hydrocarbon in a Simulated Concentrating Solar Power Loop. AIMS Energy, 2014, 2(1): 41-70. doi: 10.3934/energy.2014.1.41 |
[4] | Akthem Mohi Al-Abdali, Handri Ammari . Thermal energy storage using phase-change material in evacuated-tubes solar collector. AIMS Energy, 2022, 10(3): 486-505. doi: 10.3934/energy.2022024 |
[5] | Chao-Jen Li, Peiwen Li, Kai Wang, Edgar Emir Molina . Survey of Properties of Key Single and Mixture Halide Salts for Potential Application as High Temperature Heat Transfer Fluids for Concentrated Solar Thermal Power Systems. AIMS Energy, 2014, 2(2): 133-157. doi: 10.3934/energy.2014.2.133 |
[6] | Daniel Chuquin-Vasco, Cristina Calderón-Tapia, Nelson Chuquin-Vasco, María Núñez-Moreno, Diana Aguirre-Ruiz, Vanesa G. Lo-Iacono-Ferreira . Mathematical modeling of a binary ORC operated with solar collectors. Case study—Ecuador. AIMS Energy, 2023, 11(6): 1153-1178. doi: 10.3934/energy.2023053 |
[7] | Thiago M. Vieira, Ézio C. Santana, Luiz F. S. Souza, Renan O. Silva, Tarso V. Ferreira, Douglas B. Riffel . A novel experimental procedure for lock-in thermography on solar cells. AIMS Energy, 2023, 11(3): 503-521. doi: 10.3934/energy.2023026 |
[8] | Xian-long Meng, Cun-liang Liu, Xiao-hui Bai, De-hai Kong, Kun Du . Improvement of the performance of parabolic trough solar concentrator using freeform optics and CPV/T design. AIMS Energy, 2021, 9(2): 286-304. doi: 10.3934/energy.2021015 |
[9] | Nassir D. Mokhlif, Muhammad Asmail Eleiwi, Tadahmun A. Yassen . Experimental evaluation of a solar water heater integrated with a corrugated absorber plate and insulated flat reflectors. AIMS Energy, 2023, 11(3): 522-539. doi: 10.3934/energy.2023027 |
[10] | D.M. Reddy Prasad, R. Senthilkumar, Govindarajan Lakshmanarao, Saravanakumar Krishnan, B.S. Naveen Prasad . A critical review on thermal energy storage materials and systems for solar applications. AIMS Energy, 2019, 7(4): 507-526. doi: 10.3934/energy.2019.4.507 |
In this work, the use of solar thermal energy in the Nordic Countries, especially in Denmark, is studied. Renewable energies, such as solar, are an important ally in the fight against Climate Change, with more countries betting on them. In the past, it may have been believed that the Nordic Region does not have enough solar radiation for solar energy to be profitable, but changes in the climate and technology have proven this statement to be wrong. The case of Denmark is a clear example, which has become a leading country when it comes to solar thermal energy. A study of the current Danish solar thermal market is conducted in this project in order to understand how this country is making the most out of this energy source. Three different plants are studied (Silkeborg, Vollerup and Gr?sten) and compared to those of other Nordic Countries (Akershus from Norway and H?meenlinna from Finland). It was found that the main difference between them is the size of the Photovoltaic (PV) parks. Whilst Denmark specialised in large-scale plants with a two-phase building process, the other countries are only using small-scale installations. After a deeper understanding of the Danish energy market, it is concluded that there is a solar thermal energy policy that allows its exploitation. Furthermore, an increase in the level of solar radiation in Denmark over the last decade has led Danish experts to believe that solar energy in the Nordic Countries is going to be even more profitable in the following years. It is believed that Denmark will continue its current expansion into this market and also that the other Nordic countries will soon enough join.
This project aims to study solar thermal energy applications in Nordic Countries. An analysis of the current solar plants Danish market is conducted, aiming at extrapolating the results to other Nordic Countries (Finland and Norway). Background research took place in order to understand the current situation of solar thermal energy around the world, its purpose and deficiencies and its current use in Denmark.
Climate change is one of the most urgent problems of this generation. Based on scientific evidence, human-induced global warming reached 1 ℃ above pre-industrial levels already, and it is increasing at 0.2 ℃ per decade [1].
An active approach to this problem has already been taken by many countries, trying to both reduce their emissions and control the production of greenhouse gasses [2]. One of the keys to stopping climate change and all its consequences is to produce energy in a more sustainable way [3]. After the oil crisis in the early 70s the focus was placed on the cost of energy, but during the past decades the reality of environmental degradation and its risk has changed the way of looking at electricity generation [4]. Renewable energy sources, such as wind, solar, hydro, geothermal, and biomass produce electricity and heating by using natural resources and in some cases, they can even be produced for heating, ventilation, and air-conditioning [5], solar water heating [6], and lighting [7]. These renewable energy technologies have been changing in promising ways in the last decades, dominating the global market for new power generation capacity. In many markets, solar PV and wind are increasingly the cheapest sources of electricity [8]. Moreover, most renewable power sources will become the dominating electricity and heating generating way within the next decade [9]. Nowadays, renewable power generation is growing faster than power demand. In 2019 a huge milestone was reached, when renewable electricity generation increase was higher than that of electricity demand, while fossil-fuel electricity decreased. This was the first time in years when there was a reduction of fossil-fuel based generation while the overall electricity generation increased [9].
The European Commission has set a long-term strategy to lead European countries in global climate action with the main goal of "achieving net-zero greenhouse gas emissions by 2050 through a socially-fair transition in a cost-efficient manner" [1]. In 2016, the Paris Agreement was signed by 181 parties, requiring strong global action to reduce greenhouse emissions in order to stop the global temperature increase. It is with this aim that countries have started developing their policies and strategies around renewable energy [1].
Only three renewable energy sources can be used to produce enough heat energy to power the whole world's demand: solar, geothermal and biomass [10]. Out of these three, solar energy is the one that presents the highest global potential, since geothermal energy is limited to a few locations and biomass is not naturally present in nature. This has created a lot of interest in solar energy and the study of how to make the most out of it.
Solar energy technologies have a long history. From 1860 until the First World War an assortment of technologies was developed to create steam using the sun's heat in order to run engines and irrigation pumps. In 1954, solar PV cells were invented at Bell Labs, United States [11]. The years immediately following the oil-shock in the seventies saw much interest in the commercialization of solar energy [12]. Later on, the sharp decline in oil prices and a lack of policy support stopped this increasing interest in the solar industry. It is since early 2000 when solar energy markets have gained momentum, showing phenomenal growth recently. Both technological improvements resulting in cost reductions and government policies in favour of renewable energies have made the future of solar energy promising [10].
There are two main types of solar panels: PV solar panels and solar thermal collectors. Whilst the first ones convert sunlight into electricity, the latest use the same solar energy to generate heat, converting it into the thermal energy of a fluid.
Solar PVs have become the world's fastest-growing energy sector, with new markets. Demand for solar energy is expanding and it has become the most competitive option for electricity generation in a number of markets (for residential and commercial applications). In Figure 1, the solar PV global capacity from 2008 to 2018 can be found. It can be seen that each year the PV capacity is higher than the previous one, proving the expanding trend of this energy source.
The annual global market for PV surpassed the 100 GW level in 2018 for the first time. Large demands were found in new markets and in Europe due to ongoing price reductions. By the end of 2018, a total of 32 countries presented a cumulative capacity of 1 GB. The top five national markets, which are China, India, United States, Japan and Australia, accounted for about three-quarters of newly installed capacity in 2018. The next five markets were Germany, Mexico, the Republic of Korea, Turkey and the Netherlands [13].
Solar thermal collectors are practically behaving as heat exchangers, absorbing solar radiation and turning it into internal energy (heat) of a fluid, usually either air, water or other fluid. This heat carried by the fluid can be used for domestic purposes, such as water heating. Otherwise the heat can be saved on a thermal storage tank for later use. The global capacity of solar water heating from 2008 to 2018 can be seen in Figure 2.
By the end of 2018, residential, commercial, and industrial clients from 130 different countries had implemented solar thermal heating. Heating and cooling systems provided around 369 TWh of heat annually, which is equivalent to the energy content of 233 million barrels of oil. The cumulative global operating capacity at the end of the year reached an estimated 480 GW [13].
The rapid electrification worldwide and the massive usage of heat pumps resulted in a decline in sales of solar hot water systems. On top of that, weak demand since in several countries the national support scheme for solar heat projects was not there anymore—as it was for several years—contributed that the collector capacity went down worldwide.
A number of factors such as latitude, diurnal variation, climate and geographic variation are highly responsible for determining the solar intensity in a specific site. For instance, high winds could be proven beneficial for the panels' efficiency [14]. Lately, the floating PVs have gained some space in industrial large-scale applications [15]. The sunniest place on Earth receives about 2,500 kWh/m2. On the other hand, the Nordic Region annual density varies between 1.100 kWh/m2 (in the south of the region) and 700 kWh/m2 (in the North). A sunny day in summer will give about 8 kWh/m2 and a cloudy winter day only gives about 0.002 kWh/m2 [16]. These numbers make one question if Nordic conditions are not apt for solar production. If we look at the case of Finland, it is located between parallels of latitude 60° and 70°. If we take into account that only about 19 million people in the world live above the latitude of 60°, the Finnish population represents about 28% of that [17]. This shows how Finland is one of the most Nordic countries, making it hard to believe solar energy could be used there.
The influence of climate change on solar energy production is mostly based on changes in irradiation, for example, the cloud cover lever, and temperature. For solar energy in the Nordic Region, the ground reflection effect during winter is of high significance due to reflection from the snow [18].
In the last years, an increase in the use of solar energy has been found in the Nordic Region, especially in Denmark. It has become a leading country on solar thermal systems, having the biggest large-scale solar district heating application. By the end of 2018, there was a total of 118 active plants with a total capacity of 970 MW, which makes up 63% of the capacity worldwide [17]. By 2019, the milestone of surpassing 1 GW of solar district heating in Denmark was achieved.
The installed capacity in large-scale solar systems in Denmark between 2010 and 2018 can be seen in Figure 3. The record is in 2016, with 348 MW installed capacity.
The Danish government started using investment subsides during the 1990s to support combined heat power plants, energy efficiency measures and renewable energy generation. Most of these grants are no longer available for new district heating investments. Nonetheless, decentralised combined heat and power operating in the electricity market will receive a premium tariff over the market price. Since the end of 2018, this is only available for combined heat and power plants using renewable energy. An indirect incentive to the solar district heating development in Denmark is the requirement for all district heating utilities to collect a certain amount of energy savings every year. If a plant cannot fulfil these required savings, it has to purchase credits from another utility, which has a surplus of energy savings [17].
Prior agreements between the energy distributors and the Danish Energy Agency allowed some district heating networks to count the first year of solar heat production as energy savings, therefore creating a surplus of valuable credits for the utility allowing to pay back part of the investment. By the end of 2016, this agreement expired and a new one was not released [17]. This caused a general refrain to invest in new solar thermal systems, which is reflected in Figure 3 as a sharp market decline in 2017 and 2018. In 2019, a new agreement was reached in order to help the market recover.
Additionally, a ban on the installation of oil and gas boilers in new buildings was implemented in 2013, and since 2016 oil-fried boilers, installation is prohibited in existing buildings, making more favourable the conditions for solar thermal technologies [20].
Regarding the large-scale solar thermal system, Denmark has a mature commercial market with distinct characteristics. Almost all systems supply heat to district heating networks, with no significant industrial applications. Most combined heat and power plants in Denmark sell electricity at a market price in the Nordic power market. Denmark has very favourable conditions for solar thermal integration into district heating networks, since the country has a long-standing tradition of it. A total of 63% of Danish households are connected to district heating networks [17]. District heating is regulated by the Heat Supply Act regulation. The idea behind it is that the end-users can benefit from this system. This allows the installations to have a long-term investment horizon, which favours solar thermal technologies.
Denmark is also known for having a strong solar thermal industry. Arcon-Sunmark A/S, the main manufacturer of plate collectors in Denmark, has installed over 80% of the large-scale heating plants in Europe [17].
Four solar thermal plants from Denmark are studied in this project: Silkeborg, Vollerup and Gråsten. The technical details of each plant are given in the following subsections. The goal of this project is to conduct in-depth research on the Danish approach to solar thermal energy. To do so, the main country solar thermal plants were studied.
Silkeborg is a Danish town with a population of 46,179. It is located in the middle of the Jutland, slightly west of the geographical centre of Denmark. In 2018, Silkeborg Supply (a municipality-owned utility supplying heat in the area) constructed a solar thermal plant at Sejling Hede, near Silkeborg [21]. The plant has a minimum estimated lifetime of 25 years and it consists of 12,436 solar collectors, making it the biggest solar thermal plant in the world. Its cost was approximately 250 million DKK and it reduces CO2 emissions by 15.000 tons per year. Solar collectors have been the most cost-effective technology in Silkeborg, supplying 22.000 households with renewable heating and helping the town reach its goal of being 100% carbon neutral by 2030 [22].
This solar plant supplies around 20% of Silkeborg's district heating annual demand via the district heating network, counting the cold winter months. This equals the yearly district heating demands for 4,400 one-family houses. Up to 100% of the demand has been supplied by the plant on sunny days, with 2.7 litres of water per hour circulating through the system. Extra heat can be stored in tanks for later use [23].
In April 2020, 12,842 MWh were produced in the plant, representing 38.5% of the town's heat consumption. The best day was the 23rd of April when 88.5% of the heat demand was met by the solar plant. The heat production for the first four months of 2020 has been around 25% higher than expected [24].
Vollerup is Sønderborg's easternmost district. Sønderborg is a city and municipality in Southern Jutland, on both sides of Alssund. The majority of the city is located on the island of Als.
The solar thermal park in Vollerup includes solar panels that provide more than 50% of the heat demand for the local area of almost 1,000 households. The fluid in the solar heating installation is water mixed with eco-friendly glycol. If there is an excess of heating, it can be stored in the storage tank, which has a capacity of 4,000 m3. In addition to the thermal solar collectors, there is an array of PV solar cells that also supply the facility's pumps' and instruments' needs [25].
Gråsten is a town on the southeast coast of the Jutland Peninsula, Denmark. It belongs to the Sønderborg municipality in the Region of Southern Denmark. In 2012, a solar thermal plant was built close to Gråsten by Arcon Solar. It consists of 1,519 solar modules with an estimated maximum heat production of 9,700 MWhth. The reduction of CO2 per year caused by the solar heating is 2,800 tons [26].
The other Nordic Countries have yet not taken such a strong approach to solar thermal applications as Denmark has. Some installations can be found, but they are mostly for industrial or private use. The biggest solar thermal projects in Norway and Finland are described in this section.
In 2011, a heating park, Akershus EnergiPark, was built outside Lillestrøm. Lillestrøm is a town in the municipality of Lillestrom in Viken country. The city is located in the Oslo region, in the southeast of Norway. Akershus EnergiPark is based on a close collaboration between Akerhus Energi, business and research and educational institutions in the region.
The main source of heat production of the park is forest wood chips. In addition, it also uses biogas that comes in a 5km long pipeline from the Bøhler landfill and heat pumps. It also includes a solar heating plant that alone supplies 4 Gigawatt-hour thermal (GWhth) annually. The plant is equipped with an accumulator tank of 1,200 m3 for energy storage [27].
The plant provides 150 GWhth annually, which corresponds to the heating demand of 15,000 households, reducing CO2 emissions by over 30,000 T per year [28].
The biggest solar thermal installation in Finland is found in Hämeelinna. Hämeelinna is a city of around 68,200 inhabitants, located in the heart of Häme, in the south of Finland. In 2017, Saco-Solar installed a solar thermal system for the swimming hall in the city. The heat from the panels meets part of the building needs (such as space heating and hot water). If there is any surplus energy, for example in the days when the building is closed to the public, it is distributed to the local district heating network. A total of 124 KW can be obtained from the renewable source in this installation [29].
The goal of this project was to study solar thermal energy in the northern countries and compare its usage in Denmark with Finland and Norway. Three different Danish plants are going to be studied: Silkeborg, Vollerup and Gråsten, chosen mostly due to the abundance of information. For each of these plants, different types of data were collected and analysed [30]. An analysis of the current solar thermal market in Denmark was conducted in order to understand how the country came into the use of this renewable energy source [31] ending up with an educated guess about the future of solar thermal energy in the Nordic Countries. Different national policies in the Nordics were taken into account, since their policies have a huge impact on the use of solar thermal in each country [32].
Two different data sets were used for the project: satellite images and numerical data. The data studied focused on different solar parks - considered in terms of research design as secondary data – defined as "written sources that interpret or record primary data" [33], as they were obtained from free to access governmental sources. The collection of this data was done through the program Google Earth Pro. The online option of "Wayback imagery" was also used to properly validate the data [34]. It is with these tools that images of the solar plants were searched. Then, using the "historical images" option, time is reversed to see how the plant was constructed, paying special interest in large extensions of the plants throughout time. Once the different phases of construction were identified, the "polygon" option is used to draw polygons through the plants' area, allowing one to obtain the total area (in square-meters) of each plant before and after the extensions. This same process was repeated for each of the selected solar plants.
The second type of data collection consisted of numerical data representing the solar heat production of each plant. These were considered secondary data since they were not directly taken by the researcher of this project, but they were extracted by a validated source [35]. It should be noted that not enough data on the Vollerup solar plant were available to conduct a proper analysis, so only the other two plants were studied. Daily and weekly data were retrieved from 2017, 2018 and 2019. Additionally to the data analysis, a short market study of Denmark solar thermal energy status was conducted. To do so, background research was done and presented in section 1.4., from which some conclusions and further discussions were also extracted. Finally, an extrapolation of all the previous analyses will be made to try to predict the future of solar thermal applications in Denmark and other Nordic Countries.
The objective of this venture is to conduct in-depth inquire about the Danish approach to solar thermal plants. To do so, most nation solar-powered thermal facilities were examined. To ensure internal validity, which is "the degree to which we can appropriately conclude that the changes in X caused the changes in Y" [36], a triangulation of the data is conducted whilst comparing data from different sources and, at the same time, checking the results with the experience of experts on the area. Nevertheless, since the samples taken are also those with higher influence on the outcome, an inductive statement extracted from this paper should be correct for all cases. The reliability of the project is assured with a good explanation of every step taken in the making of it, allowing others to repeat the analysis and assuring they will obtain the same results. In this project, actual data from the solar plants could provide an insight complementing the satellite data. The results from this report will show the potential of solar thermal energy for Nordic Countries, using Denmark as the main example.
There is a specific way solar plants are developed in Denmark in comparison to the other Nordic countries. In Denmark, all the studied plants are built in two phases: first, some panels are implemented and if the result is as predicted, the plant is then extended to its optimal size. In the other Scandinavian countries, a one-stage construction phase is followed for the plants.
If we look at the Silkeborg Plant, the first phase consisted of around 172,000 m2 (two polygons of 45,929 m2 and 125,611 m2). This is then expanded with a 20,360 m2 big area and a small part of 9,077 m2. The total final area of the plant is then around 334,000 m2. This means that the extension of the plant consists of adding a 99.86% of its first size. In other words, the plant size was almost doubled in size. The plant construction phases are shown in Figure 4.
The building process of the Vollerup solar thermal plant follows the same pattern. First, a total of around 12,700 m2 are built (consisting of 4 polygons of 2,128 m2 the top one, 3.167 m2 the left one, 3,606 m2 the right one and 3,816 the bottom one). Later on, two extra polygons of area 1,274 m2 and 1,752 m2 were added, making the total plant area around 15,700 m2. The added area was a 23.62% of the original installation. The plant construction phases and the polygons used to measure it are shown in Figure 5.
The Gråsten solar thermal-plant had an original size of around 39.350 m2, divided into 3 areas of 3,524 m2, 15,082 m2 and 20,750 m2 from left to right. An extra polygon of 24,717 m2 was added later on, making the total plant area about 67,000 m2. The added area is a 70.3% of the original one. The polygons used to measure the plant and its phases of construction are shown in Figure 6.
If the different areas are compared, Silkeborg is 5 times larger than Gråsten and 23 times larger than Vollerup. It is also this plant the one that had the higher percentage of added area. A summary of each plant area can be seen in Table 1. It is noticed that all the plants were constructed until their optimal size using a second construction phase. It should also be pointed out that all the solar plants studied are large-scale plants.
Silkeborg | Vollerup | Gråsten | |
Initial area [m2] | 171,540 | 12,700 | 39,350 |
Added area [m2] | 17, 1305 | 3,000 | 27,650 |
Total area [m2] | 342,845 | 15,700 | 67,000 |
% of added area | 99.8 | 23.6 | 70.3 |
On the other hand, if the area of Akershus (Norway) and Hämeenlinna (Finland) installations are analysed, it can be seen that only one construction phase was used.
As for the Akershus, the total measured area is 28,550 m2, consisting of two polygons of 8,725 m2 and 19,825 m2, as shown in Figure 7.
The Hämeenlinna total area is 515 m2 and it is situated on the roof of a building (the swimming pool hall), as shown in Figure 8. This is a small installation since its only purpose is to supply heat to one building.
Both the Norwegian and Finnish plans have not been taken to their maximum production level, with only one construction phase. These installations are considered small-scale, consisting of a small area if compared with the Danish plants. This is due to the approach to solar energy made by the countries, decided to entrust small-scale applications and industrial utilities instead of large-scale plants. For a higher increase of solar energy use in these countries, the policies prioritising small installations should be revised.
The results of the study of solar heat production of two Danish solar thermal plants (Silkeborg and Gråsten) are now presented. The monthly solar heat production from 2017 until 2019 is shown in Figure 9A, C for Silkeborg and Gråsten respectively. If both graphs are observed it can be seen that they follow a similar sinusoidal pattern, with high heat production during the summer months and low during the winter months. This is a pattern that is repeated every year, indifferently from the plant position or value of solar heat production.
A closer inspection of the last year can be seen in Figure 9B, D. The daily solar heat production is represented for each plant (Silkeborg and Gråsten). It is observed that, whilst the followed pattern is quite similar, the amount of solar heat production in Silkeborg is quite higher than that of Gråsten. This is also related to the size of the solar plants, since the Silkeborg plant is 4 times larger than the one in Gråsten. Silkeborg highest solar heat production is around 4,500 MWh whilst Gråsten's is around 700 MWh.
Once the solar thermal plants have been studied, it is important to know the context in which these are found. A quick study of the Danish market is done. As shown in section 1.4., the Danish solar thermal market has seen a huge increase in the last years, placing it amongst the top countries using this energy source. The main reasons behind this market expansion are summarised below.
Denmark presents a high market penetration of district heating networks, specifically for the heat of households. In most Scandinavian countries, the share of district heating systems is above 60%. Only Finland has a share of lower than 40% [37]. Moreover, there is high compatibility of solar thermal heat with these district heating loads. Some examples of this compatibility would be the high heat demand and density of the networks and the opportunity of obtaining cheap land for collectors near the supply points. Another important aspect that encourages the use of thermal energy in Denmark is the high taxes on fossil fuels, making coal and natural gas plants less profitable. The situation regarding the cost of natural gas becomes even more complicated for all Europe under the Russo-Ukrainian War and the overall availability and costs for all European countries including, of course, the Nordics [38]. Since this conflict goes if not back to the dissolution of the Soviet Union, definitely back to 2014—where hundreds maybe thousands of enmities took (and still continue to take) place in the borders of the two countries, it is therefore, imperative to focus on other alternatives for district heating apart from natural gas. Furthermore, the presence of market-based subsidies for large-scale thermal systems encourages the use of this energy source. These incentives are focused on district heating facilities instead of small-scale applications or industrial utilities—such as solar air-conditioning [39]—an effective strategy to promote large-scale plants. Finally, the country non-profit orientation regulations regarding heat supply allow a long-term investment horizon and facilitate the availability of easily accessible bank loans for the utilities.
These conditions seem to outweigh the lacking solar resources, making a country like Denmark with a low irradiance levels, competent in the solar thermal market world. Whilst the other countries have a higher rate of solar radiation, Denmark unique policies and market strategy for this energy source allow it to be competent.
Solar thermal energy is an energy source that has gained a lot of importance in the last few years, but there are still improvements to be done. It is believed that its use will grow even more in the following years, since solar costs—including solar thermal applications—have dropped more than 80% over the past decade [40]. Denmark is a pioneer in the large-scale use of solar thermal plants, expanding its utilities until its optimal dimension in order to get as much solar energy as possible. Nowadays, a clear increase in the cumulative amount of annual solar heat production of Danish solar plants is noted, indicating an increase in solar radiation in the Nordic Region over the last years. These climate transformations that we are living in nowadays are a key component in the future development of solar energy in the Nordic Countries. Solar energy may not have been considered a profitable source in the past in these countries, but with climate change, this should be reconsidered [41].
The main difference between Denmark and the other Nordic Countries analysed is the marketplace of solar thermal heat. Whilst Denmark has decided to include it in their district heating, the other countries are using it only still for small-scale installations and industrial purposes. This is mainly due to each country's legislative framework and its approach to renewable energies. Denmark has decided to approach the heat market in a smart way that allows the profitable existence of large-scale solar plants. The other Nordic Countries may change their approach to this energy source in the following years, since it has been proven that it is a profitable renewable energy even for a Nordic country such as Denmark.
In this project, the solar thermal energy in the Nordic countries was studied, mainly though focused on Denmark. In the last years, solar energy has been gaining popularity all around the world as one of the two most dominating renewable energy sources with several innovative applications, and the Nordic Countries are not falling behind in this trend. Whilst it is true that the weather conditions in these countries are not the most favourable for solar energy, Climate Change is giving the Nordic Region a chance. Denmark is one of the pioneers of solar thermal energy. Even if it does not have the highest solar radiation, it is due to its smart approach to this energy source that the country has a big impact on the solar thermal market. Their main use is in large-scale plants connected to district heating distribution, which is possible thanks to the country regulations and favourable agreements. This makes them able to use this energy to its maximum potential whilst other countries are still struggling to integrate it into their energy system.
Other Nordic Countries are yet not as developed in this energy source as Denmark is. In this project, the case of Norway and Finland have been studied. The main difference that has been found between these countries and Denmark is their solar thermal plants' size. Whilst Denmark is betting for large-scale plants with a two-phase system to bring them to their optimal point, the other countries are still using small-scale facilities. They focus mostly on private and self-consuming (running under a net metering contract) installations, not having integrated this energy source into their energy system yet. It has been shown in this project that the amount of solar heat production of Danish plants and, therefore, the solar radiation in this country has had a noticeable increment in the last years. It is also believed that for other Nordic Countries to have a profitable use of solar thermal energy, a change in their approach to it should be made. Taking Denmark as an example, expanding the size of their solar thermal installations should be considered, using solar thermal energy not only for private use but also as one of their main heat energy sources. Policies as those used in Denmark could be used to boost the solar market, offering agreements favourable to the large-scale use of the energy source.
All authors declare no conflicts of interest in this paper.
[1] | European Comission. (2018) Communication from the Comission to the European Parlament, the European Council, the council, the European economic and social comittee, the Comittee of the regions and the european investment bank. Brussels. |
[2] |
Shahsavari A, Akbari M (2018) Potential of solar energy in developing countries for reducing energy-related emissions. Renewable Sustainable Energy Rev 90: 275-291. https://doi.org/10.1016/j.rser.2018.03.065 doi: 10.1016/j.rser.2018.03.065
![]() |
[3] |
Koroneos C, Xydis G, Polyzakis A (2013) The optimal use of renewable energy sources—The case of Lemnos Island. Int J Green Energy 10: 860-875. https://doi.org/10.1080/15435075.2012.727929 doi: 10.1080/15435075.2012.727929
![]() |
[4] |
Khan J, Arsalan MH (2016) Solar power technologies for sustainable electricity generation—A review. Renewable Sustainable Energy Rev 55: 414-425. https://doi.org/10.1016/j.rser.2015.10.135 doi: 10.1016/j.rser.2015.10.135
![]() |
[5] | Koroneos C, Nanaki E, Xydis G (2009) Solar air-conditioning systems impact on the built environment—A thermodynamic approach. Proceeding of the 45th ISOCARP Congress, 1-16. Available from: https://www.researchgate.net/publication/322661842_Solar_Air-conditioning_Systems_Impact_on_the_Built_Environment-A_Thermodynamic_Approach. |
[6] |
Islam MR, Sumathy K, Khan SU (2013) Solar water heating systems and their market trends. Renewable and Sustainable Energy Rev 17: 1-25. https://doi.org/10.1016/j.rser.2012.09.011 doi: 10.1016/j.rser.2012.09.011
![]() |
[7] |
Wong S (2012) Overcoming obstacles against effective solar lighting interventions in South Asia. Energy Policy 40: 110-120. https://doi.org/10.1016/j.enpol.2010.09.030 doi: 10.1016/j.enpol.2010.09.030
![]() |
[8] |
Timilsina GR (2021) Are renewable energy technologies cost competitive for electricity generation? Renewable Energy 180: 658-672. https://doi.org/10.1016/j.renene.2021.08.088 doi: 10.1016/j.renene.2021.08.088
![]() |
[9] | IRENA (2020) Global Renewables Outlook. Abu Dhabi: International Renewable Energy Agency, Available from: https://irena.org/-/media/Files/IRENA/Agency/Publication/2020/Apr/IRENA_GRO_Summary_2020.pdf?la=en&hash=1F18E445B56228AF8C4893CAEF147ED0163A0E47. |
[10] |
Kabir E, Kumar P, Kumar S, et al. (2018). Solar energy: Potential and future prospects. Renewable Sustainable Energy Rev 82: 894-900. https://doi.org/10.1016/j.rser.2017.09.094 doi: 10.1016/j.rser.2017.09.094
![]() |
[11] | Fraas LM (2014) History of solar cell development. In Low-Cost Solar Electric Power. Cham.: Springer, 1-12. https://doi.org/10.1007/978-3-319-07530-3_1 |
[12] | Silvi C (2003) Can the history of energy technology and use educate us for a solar energy future? The Italian case. In Proceedings. ISES Solar World Congress. Göteborg, Sweden: ISES. Avaiable from: https://www.fondazionemicheletti.eu/contents/documentazione/archivio/Altronovecento/Arc.Altronovecento.09.03.pdf. |
[13] | Zhongming Z, Linong L, Xiaona Y, et al. (2021) World Adds Record New Renewable Energy Capacity in 2020, Available from: http://resp.llas.ac.cn/C666/handle/2XK7JSWQ/321399. |
[14] |
Xydis G (2013) The wind chill temperature effect on a large‐scale PV plant—an exergy approach. Prog Photovoltaics 21: 1611-1624. https://doi.org/10.1002/pip.2247 doi: 10.1002/pip.2247
![]() |
[15] |
Gorjian S, Sharon H, Ebadi H, et al. (2021) Recent technical advancements, economics and environmental impacts of floating photovoltaic solar energy conversion systems. J Cleaner Prod 278: 124285. https://doi.org/10.1016/j.jclepro.2020.124285 doi: 10.1016/j.jclepro.2020.124285
![]() |
[16] | Fenger J (2007) Impacts of climate change on renewable energy sources: Their role in the Nordic energy system. Aarhus: Nordic Energy Research, Available from http://www.norden.org/da/publikationer/publikationer/2007-003/at_download/publicationfile. |
[17] |
Tschopp D, Tian Z, Berberich M, et al. (2020) Large-scale solar thermal systems in leading countries: A review and comparative study of Denmark, China, Germany and Austria. Appl Energy 270: 114997. https://doi.org/10.1016/j.apenergy.2020.114997 doi: 10.1016/j.apenergy.2020.114997
![]() |
[18] |
Kallio-Myers V, Riihelä A, Lahtinen P, et al. (2020) Global horizontal irradiance forecast for Finland based on geostationary weather satellite data. Sol Energy 198: 68-80. https://doi.org/10.1016/j.solener.2020.01.008 doi: 10.1016/j.solener.2020.01.008
![]() |
[19] | Solvarmedata (2021). solvarmedata.dk. Retrieved from plants. Available from: https://solvarmedata.dk/plants/. |
[20] |
Zuberi MJ, Chambers J, Patel MK (2021) Techno-economic comparison of technology options for deep decarbonization and electrification of residential heating. Energy Effic 14: 1-18. https://doi.org/10.1007/s12053-021-09984-7 doi: 10.1007/s12053-021-09984-7
![]() |
[21] | State of Green (2020) State of Green. Retrieved from World largest solar heating plant in Silkeborg. Available from: https://stateofgreen.com/en/partners/ramboll/solutions/world-largest-solar-heating-plant-in-silkeborg/. |
[22] | Silkeborf forsyning Available from: https://www.silkeborgforsyning.dk/varme/produktionsanl%C3%A6g/solvarmeanl%C3%A6g. |
[23] | Silkeborg municipality (2019). Sustainable solutions in Silkeborg. Available from: https://www.silkeborg.com/sites/silkeborg.com/files/2019-09/8070_B%C3%A6redygtig_2019_engelsk.pdf. |
[24] | Silkeborg forsynin (2020) Available from: https://www.silkeborgforsyning.dk/solvarmen-i-april. |
[25] | Goodsite ME, Juhola S (2017) Green Defense Technology: Triple Net Zero Energy, Water and Waste Models and Applications. Springer. https://doi.org/10.1007/978-94-017-7600-4 |
[26] | Cubic (2012) Available from: https://www.cubic.eu/references/large-scale-solar-heating-plant--renewable-energy. |
[27] | Aosvold KA (2018) Store Norske Leksikon. Available from: https://snl.no/Akershus_EnergiPark. |
[28] | Bor JI (2018) Akershus County Municipality. Available from: https://www.akershus.no/nyheter/?article_id=200691. |
[29] | Savosolar (2017) Available from: https://savosolar.com/savo-solar-delivers-solar-thermal-heating-systems-in-finland/. |
[30] | Creswell JW (2014) Research Design: Qualitative, Quantitative and Mixed Methods Approaches. Thousand Oaks: SAGE Publications, Inc. Available from: https://www.ucg.ac.me/skladiste/blog_609332/objava_105202/fajlovi/Creswell.pdf. |
[31] |
Tian Z, Zhang S, Deng J, et al. (2019) Large-scale solar district heating plants in Danish smart thermal grid: Developments and recent trends. Energy Convers Manage 189: 67-80. https://doi.org/10.1016/j.enconman.2019.03.071 doi: 10.1016/j.enconman.2019.03.071
![]() |
[32] |
Hirvonen J, Jokisalo J, Kosonen R, et al. (2020) EU emission targets of 2050: costs and CO2 emissions comparison of three different solar and heat pump-based community-level district heating systems in nordic conditions. Energies 13: 4167. https://doi.org/10.3390/en13164167 doi: 10.3390/en13164167
![]() |
[33] | Walliman N (2011) Research Methods: The Basics. 1st Edition, New York: Taylor & Francis Ltd, 208. https://doi.org/10.4324/9780203836071 |
[34] |
Kerski JJ (2019) Teaching demography and population change using web GIS tools and data. Geogr Teach 16: 126-132. https://doi.org/10.1080/19338341.2019.1619608 doi: 10.1080/19338341.2019.1619608
![]() |
[35] |
Lake AA, Burgoine T, Greenhalgh F, et al. (2010) The foodscape: classification and field validation of secondary data sources. Health Place 16: 666-673. https://doi.org/10.1016/j.healthplace.2010.02.004 doi: 10.1016/j.healthplace.2010.02.004
![]() |
[36] | Seltman HJ (2018) Experimental design and analysis. Chapter 11: Two-way Aova. Available from: https://www.stat.cmu.edu/~hseltman/309/Book/Book.pdf. |
[37] | Energy Monitor (2022) Europe makes progress integrating renewables in district heating. Available from: https://www.energymonitor.ai/sectors/heating-cooling/europe-makes-progress-integrating-renewables-in-district-heating. |
[38] | Tzogopoulos GN (2022) The Ukraine Crisis. Policy. Available from: https://www.eliamep.gr/en/publication/%CE%BA%CF%81%CE%AF%CF%83%CE%B7-%CF%83%CF%84%CE%B7%CE%BD-%CE%BF%CF%85%CE%BA%CF%81%CE%B1%CE%BD%CE%AF%CE%B1-%CF%85%CF%80%CE%AC%CF%81%CF%87%CE%B5%CE%B9-%CE%B1%CE%BA%CF%8C%CE%BC%CE%B7-%CF%80%CE%B5%CF%81/. |
[39] |
Koroneos C, Nanaki E, Xydis G (2010). Solar air conditioning systems and their applicability—an exergy approach. Resour Conserv Recycl 55: 74-82. https://doi.org/10.1016/j.resconrec.2010.07.005 doi: 10.1016/j.resconrec.2010.07.005
![]() |
[40] | The World Economic Forum (2022) The price of solar power has fallen by over 80% since 2010. Here's why. Available from: https://www.weforum.org/agenda/2021/11/renewable-energy-cost-fallen. |
[41] |
Costoya X, deCastro M, Carvalho D, et al. (2022) Combining offshore wind and solar photovoltaic energy to stabilize energy supply under climate change scenarios: A case study on the western Iberian Peninsula. Renewable Sustainable Energy Rev 157: 112037. https://doi.org/10.1016/j.rser.2021.112037 doi: 10.1016/j.rser.2021.112037
![]() |
1. | Ioan Sarbu, Matei Mirza, Daniel Muntean, Integration of Renewable Energy Sources into Low-Temperature District Heating Systems: A Review, 2022, 15, 1996-1073, 6523, 10.3390/en15186523 | |
2. | Ioan Sarbu, Alexandru Dorca, Matei Mirza, Exhaustive overview of advances in integrating renewable energy sources into district heating systems, 2024, 116, 22106707, 105897, 10.1016/j.scs.2024.105897 | |
3. | Kristian Emil Schrøder Hansen, George Xydis, Long-term heat storage opportunities of renewable energy for district heating networks, 2024, 1751-4223, 1, 10.1680/jener.23.00023 | |
4. | Qianyun Wen, Axel Lindfors, Yang Liu, How should you heat your home in the green energy transition? A scenario-based multi-criteria decision-making approach, 2023, 421, 09596526, 138398, 10.1016/j.jclepro.2023.138398 | |
5. | Georgios Martinopoulos, Domestic hot water solar thermal systems as an energy-security alternative in the EU: A parametric analysis, 2024, 69, 22131388, 103921, 10.1016/j.seta.2024.103921 |
Silkeborg | Vollerup | Gråsten | |
Initial area [m2] | 171,540 | 12,700 | 39,350 |
Added area [m2] | 17, 1305 | 3,000 | 27,650 |
Total area [m2] | 342,845 | 15,700 | 67,000 |
% of added area | 99.8 | 23.6 | 70.3 |