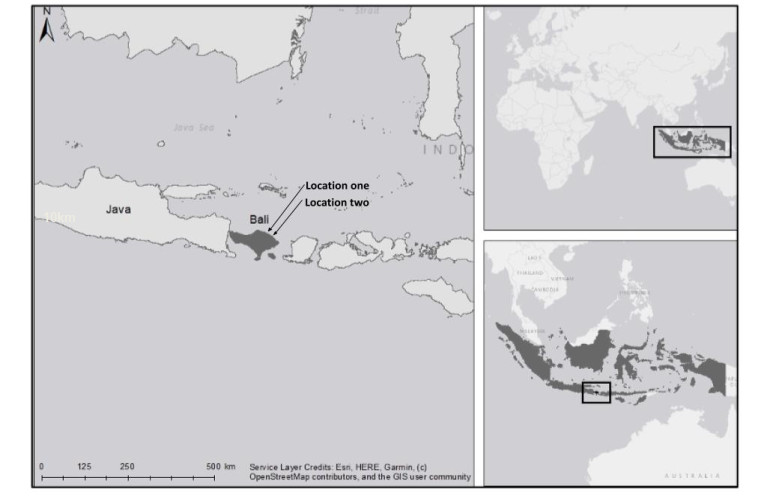
The proposed reconfigurable BPF satisfies the International Telecommunication Unionos (ITU) region 3 spectrum requirement. In transmit mode, the frequency range 11.41-12.92 GHz is used by the direct broadcast service (DBS) and the fixed satellite service (FSS). Direct broadcast service (DBS) in reception mode employs 11.7-12.2 GHz and 17.3-17.8 GHz frequency ranges. Frequency reconfigurable filters are popular because they can cover wide range of frequencies, reducing system cost and space. Another emerging trend is electronic component flexibility or conformability, which allows them to be mounted on non-planar objects and are used in wearable applications. This project contains a frequency-reconfigurable BPF that has been entirely printed on a flexible polimide substrate. Frequency reconfigurability is obtained by using a pin diode HSCH 5318 and it is used to switch between 12 GHz and 18 GHz. The prototype reconfigurable BPF is highly compact and low-cost due to the flexible polimide substrate and the measured results are promising and match the simulated results well.
Citation: Ambati. Navya, Govardhani. Immadi, Madhavareddy. Venkata Narayana. Flexible ku/k band frequency reconfigurable bandpass filter[J]. AIMS Electronics and Electrical Engineering, 2022, 6(1): 16-28. doi: 10.3934/electreng.2022002
[1] | Wei-Mao Qian, Tie-Hong Zhao, Yu-Pei Lv . Refinements of bounds for the arithmetic mean by new Seiffert-like means. AIMS Mathematics, 2021, 6(8): 9036-9047. doi: 10.3934/math.2021524 |
[2] | Ling Zhu . Sharp bounds for Heinz mean by Heron mean and other means. AIMS Mathematics, 2020, 5(1): 723-731. doi: 10.3934/math.2020049 |
[3] | Wei-Mao Qian, Miao-Kun Wang . Sharp bounds for Gauss Lemniscate functions and Lemniscatic means. AIMS Mathematics, 2021, 6(7): 7479-7493. doi: 10.3934/math.2021437 |
[4] | Hamidou Tembine . Mean-field-type games. AIMS Mathematics, 2017, 2(4): 706-735. doi: 10.3934/Math.2017.4.706 |
[5] | Thiyam Thadoi Devi, Khundrakpam Binod Mangang, Sonika Akoijam, Lalhmangaihzuala, Phinao Ramwungzan, Jay Prakash Singh . Mean chain transitivity and almost mean shadowing property of iterated function systems. AIMS Mathematics, 2024, 9(8): 20811-20825. doi: 10.3934/math.20241012 |
[6] | Ahmad Bin Azim, Ahmad ALoqaily, Asad Ali, Sumbal Ali, Nabil Mlaiki, Fawad Hussain . q-Spherical fuzzy rough sets and their usage in multi-attribute decision-making problems. AIMS Mathematics, 2023, 8(4): 8210-8248. doi: 10.3934/math.2023415 |
[7] | Jing-Feng Tian, Ming-Hu Ha, Hong-Jie Xing . Properties of the power-mean and their applications. AIMS Mathematics, 2020, 5(6): 7285-7300. doi: 10.3934/math.2020466 |
[8] | Khuram Ali Khan, Shaista Ayaz, İmdat İşcan, Nehad Ali Shah, Wajaree Weera . Applications of Hölder-İşcan inequality for n-times differentiable (s,m)-convex functions. AIMS Mathematics, 2023, 8(1): 1620-1635. doi: 10.3934/math.2023082 |
[9] | Riffat Jabeen, Aamir Sanaullah, Muhammad Hanif, Azam Zaka . Two-exponential estimators for estimating population mean. AIMS Mathematics, 2021, 6(1): 737-753. doi: 10.3934/math.2021045 |
[10] | Zareen A. Khan, Waqar Afzal, Mujahid Abbas, Jongsuk Ro, Najla M. Aloraini . A novel fractional approach to finding the upper bounds of Simpson and Hermite-Hadamard-type inequalities in tensorial Hilbert spaces by using differentiable convex mappings. AIMS Mathematics, 2024, 9(12): 35151-35180. doi: 10.3934/math.20241671 |
The proposed reconfigurable BPF satisfies the International Telecommunication Unionos (ITU) region 3 spectrum requirement. In transmit mode, the frequency range 11.41-12.92 GHz is used by the direct broadcast service (DBS) and the fixed satellite service (FSS). Direct broadcast service (DBS) in reception mode employs 11.7-12.2 GHz and 17.3-17.8 GHz frequency ranges. Frequency reconfigurable filters are popular because they can cover wide range of frequencies, reducing system cost and space. Another emerging trend is electronic component flexibility or conformability, which allows them to be mounted on non-planar objects and are used in wearable applications. This project contains a frequency-reconfigurable BPF that has been entirely printed on a flexible polimide substrate. Frequency reconfigurability is obtained by using a pin diode HSCH 5318 and it is used to switch between 12 GHz and 18 GHz. The prototype reconfigurable BPF is highly compact and low-cost due to the flexible polimide substrate and the measured results are promising and match the simulated results well.
Coral reefs are important marine habitats, containing over 25% of the world's fish species [1,2]. Well documented anthropogenic activities have caused a worldwide long-term decline in coral biodiversity, abundance and habitat structure [3,4]; altering ecosystem functioning and processes [5]. As a consequence, this has reduced the ability of coral reefs to provide society with ecosystem services [6] such as food provision, shoreline protection, biogeochemical cycling and tourism [7,8].
The first UNESCO global scientific assessment of coral reef decline predicts that all 29 coral-containing World Heritage sites will no longer be functioning coral reef ecosystems by 2100 under a business-as-usual emissions scenario, due to coral bleaching mostly associated with ocean warming and acidification [9]. Alongside aggressive and immediate global-scale interventions (such as the 2015 Paris agreement) to reduce greenhouse gas emissions and their impact on coral reefs (as highlighted in the IPCC "Ocean Systems" report [10]), various other local scale options may be considered to offset the decline of coral reef biodiversity, abundance and habitat structure. Although unlikely to protect large-scale ecosystem function, methods including coral propagation, coral gardening and enforcement of fishing regulation can provide some degree of replacement for localised reef degradation and some local-level ecosystem services [10]. In addition, deployment of artificial reefs (ARs) can also assist with mitigation of negative impacts on coral reef habitats. ARs are structures built of natural or man-made materials, intentionally placed on the seafloor, which are designed to protect, enhance, or restore components of marine ecosystems [11] and have been shown to support comparable levels of fish density, biomass, number of species, and diversity to natural reefs [12]. Number of species and abundance of fish are associated with coral health, and are known to decline in the event of a loss of coral diversity and cover [13,14]. In situations such as these, ARs can be used as method to restore a degraded and/or unproductive ecosystem by providing new resources for both juvenile and adult species [15,16]. Alongside restoration and habitat provision, ARs have multiple other functions in coastal management [17]. Some of these include increasing fisheries yield [18,19], boosting dive tourism [20,21] and preventing trawling [22,23].
There are multiple studies demonstrating the potential of ARs to mitigate habitat loss (e.g. [11,15,24]), increase larval and juvenile recruitment, survival, and growth [18] and maintain biodiversity in marine systems [15]. Despite this, a recent literature review by [12] showed that artificial reefs are not one-size-fits-all tools for habitat enhancement projects, with many factors influencing overall social and ecological success. Firstly, the structural complexity of the AR structure determines the overall diversity, assemblages and community structure [24,25]. Constructing ARs using concrete allows the structures to be built with hiding spaces, more than one exit, shadow against light, high surface area and hollow interior spaces [26,27]. ARs built with this structural complexity allows greater colonisation of biological communities as spawning adults benefit from a textured surface to lay their eggs, whilst juveniles are provided with shelter and protection and therefore use the AR as a nursery [24].
Other factors determining the success of ARs (in terms of increasing fish biodiversity and abundance) may include site selection [28], succession rates over time [29,30,31], fishing regulations on and around the structures [32], size of the structures [33], and the degree of isolation from natural habitats [28,34]. [28] also showed that different factors within AR establishment can lead to different results, for example, how site selection is important for abundance, while the design was important for diversity. Additionally, the "attraction versus production" debate is important in understanding the real success of ARs [30]. It discussed if ARs actually increase net production of a site, or whether they merely cause attraction and redistribution of already existing individuals [35]. To definitively distinguish between new and redistributed production on an AR is difficult [18,36] and can only be calculated with prior knowledge of local habitats and species movements. This debate remains topical in current AR literature [20,37].
Global literature has also highlighted that ARs can cause additional threats to marine ecosystems in certain situations. For example, [38] discussed that ARs pose risks, to nearby natural habitats, through creating changes in food-web structure, connectivity and larval dispersal patterns between habitats, and the introduction of pollutants, diseases and/or marine pests. [38] also discussed how fished ARs can lead to a long term exploitation of targeted species that are attracted to the artificial reef from natural habitats. Other research, such as [39] showed that ARs can alter sound, light, hydrodynamics and organic enrichment, resulting in habitat degradation and displacement of localised flora and fauna. Additionally, [40] highlighted that ARs are physically, hydrologically, and chemically different from natural habitats, and this can in some circumstances be more advantageous to nonindigenous than native species. Certain case studies have shown that artificial reefs can promote the invasion of non-native species, leading to negative impacts for native species (see [41]). [38] concluded that AR programs should undergo evidence based risk assessments and cost-benefit analysis, which considers how a proposed program may lead to negative environmental and social impacts. Additionally, [42] discussed the importance of involving stakeholders in the initial planning, risk assessment and decision making process before an AR program is established. The authors discussed how adequately considering the opinions of stakeholders (for example environmental NGOs, local fishermen and recreational marine users), can help to ensure the proposed project does not lead to social and environmental consequences.
Support from the local community is also a factor in the success or failure of an AR program [43,44]. The social acceptability (sometimes known as "social licence") of a marine management program can lead to the slowing of progress towards achieving environmental objectives, depending on how supported it is by the local community [45]. Deployment of ARs can lead to direct socio-economic benefits for the local community, such as increasing yield for fishers [18,19] and boosting tourism [20,21]. Despite the benefits that can arise as a result of their establishment, in some cases, local communities may choose not to support marine restoration programs due to social, financial, cultural and political reasons [46]. For a program to maintain long-term, sustainable community support, the following factors are also important: (1) The communities perceived benefit from the program; most often a financial gain arising from the conservation work (e.g. a fisher increasing yield due to higher fish biomass) [47], (2) inclusion of local people in conservation decision-making processes [43,48], (e.g. compliance of marine protected area (MPA) regulations have been shown to be higher when local fishers are involved in establishment and enforcement [49]), (3) Influence from local leaders, which have been shown to "bridge the gap" between local people and marine conservation objectives [50], (4) ensuring that the community are empowered with sufficient knowledge of local environmental issues [51]. Recent literature has highlighted that there is still limited knowledge regarding the social issues related to artificial reef habitats, especially in terms of the conflicts between fishing communities and the implementation of artificial reefs [44], and it is encouraged that more research focuses on the social, cultural and political landscape of the communities in which marine management program are proposed [45].
The primary focus of this research is to assess the localised ecological habitat enhancement potential of ARs within north Bali. The aim of this comparative study is to provide some clarity on the benefits that ARs may bring to the local community in an area of previously degraded reef. Specifically, it will test the hypothesis that fish biodiversity and abundance at both ARs will be higher compared to the nearby flat sand bed and similar to the natural coral reefs. The study will also address how community structure varies between habitats and how biodiversity and abundance differ between new and mature ARs.
The studies were conducted at two locations which were approximately 17 km apart on the north east coast of the island of Bali (Figure 1). Location one was at LINI Aquaculture and Training Centre (LATC; 8°07'43.1"S, 115°21'53.4"E) in Les Village, Buleleng regency, and location two, 16km away at North Bali Reef Conservation (NBRC; 8°11'27.5"S, 115°29'42.9"E) in Tianyar Village, Karangasem regency. Within these locations, four habitat types were surveyed; this included a mature artificial reef (MAR) in site one and a flat sand bed (FSB), a new artificial reef (NAR) and coral reef (CR) in site two.
The MAR (Figure 2) sits within location one and is managed by the NGO "LATC", which started working with local communities in Les Village in 2008 after substantial coral degradation had occurred (personal communications with the NGO team). The main cause of degradation of the coral reef at location one was destructive fishing practices previously used by local fishers. Destructive practices may include the use of cyanide and blast (dynamite) fishing, which allows fishers to more effectively catch target species (which are shocked and/or killed after the techniques are used), but at the same time causing physical damage to the local benthic marine environment. LATC started to work with local fishers to stop the use of destructive fishing practices, whilst also deploying artificial reefs in areas that had previously been degraded as a method to speed up recovery rate of local marine habitats. Fishing remains the primary occupation (and fish as the primary food source) of the local people within Les Village, although personal communication with local fishers revealed that destructive practices are no longer used. There was no known MPA established at location one at the time of this study.
Local people commented that location two was previously used as a port for fishers due to its close proximity to the community fish market. The natural reef at this location was said to have been destroyed due to heavy boat traffic and anchoring during these times. Similar to Les Village, fishing remains the primary occupation (and fish as the primary food source) of the local people in Tianyar, however location two is no longer used as a port, so the previous threats no longer persist. The NGO "NBRC" was established in 2017 to work with local fishers to restore the previously degraded coral reef by building ARs and establishing an MPA. To do this, community leaders worked with local fishers to agree on the best method to set up a conservation project. It was agreed that a no-take zone MPA would be established (and enforced by the local community). Whilst fishers were initially concerned that catch within the bay will clearly decrease as a result of the MPA, it was explained that overall yield may increase as a result of the "spill-over effect", as described and demonstrated by [52,53]. Since 2017, the community of Tianyar Village, have also been building artificial reefs, financed mostly by government funding and international donations. As well as the artificial reef habitat, location two also hosts a nearby flat sand bed and coral reef. The FSB, AR and CR (Figure 2) within this study were each approximately 200m apart.
The NAR structures had been deployed for 1–1.5 years at the time of this study (with different sections of the structure deployed 6 months apart). The MAR habitat had AR structures which had been deployed for 8–10 years at the time of this study. ARs at both the NAR and MAR were deployed between a depth of 5–10 m and constructed using a three part mix of cement, calcium and sand. This produced what are known as "roti buaya", 1 × 0.5 m long flat structures with rough textured surface to allow natural recruitment of coral and settlement of other species. The units were deployed on areas of flat sand bed or bare rubble, both lacking in physical complexity. The structures (NAR and MAR) were installed in groups that ranged in numbers between 10–20. Each group covered an area of approximately 10 m², where structures were stacked haphazardly (in a similar configuration between groups, and also locations), with the aim of providing optimal protective space, such as holes, tunnels and caves which provide additional habitat for sheltering fish (Figure 3). There was approximately 10 m spacing between each group.
All four habitat types (Figure 2) were surveyed over a 3 month period (July to September) in the middle of Bali's dry season. These four different habitat types were all studied within the same depth range (5–10 m) and had a daily easterly current at the time of study. Despite the 16 km distance apart, the two locations were relatively similar in terms of environmental conditions. The locations were both N/NE facing, with similar water temperatures, prevailing SW wind directions and easterly currents (authors observations). Permission was given by both organisations to survey the ecosystems. Three sample sites (herein sites) were established (in each of the four habitat types (herein habitats)) for deployment of a Remote Underwater Video (RUV) camera. The sites were chosen haphazardly (approximately 50 m apart from each other), and to allow easy identification, each site was marked with a 30 cm² cement base attached to a metal frame and sign.
RUV is a surveying technique commonly used in marine environments [54]. Described as a cost effective, safe and non-destructive method [34], RUV was used to compare fish biodiversity and abundance between each habitat type. A GoPro Hero 4 HD 1080p underwater camera was fixed to a weighted unit, rope and buoy. The RUV unit was then deployed from a boat, directly on top of the marker at each site. Each marker was 2 meters away from the desired subject (e.g AR or CR) and the camera directly faced it. Recordings were 25 minutes in duration, allowing for an initial 5 minute settlement period and 20 minutes of analysis time. This duration was determined from a 50 minute preliminary deployment used to plot a species accumulation curve (Figure 4), which showed that typically ~ 80% of species were present in the first 20 minutes of a 50 minute recording. It was therefore decided that a 20 minute recording time was an optimal duration to obtain consistent estimates of abundance, number of species and community structure, balanced against constraints of storing large video files and time required to collect data from the videos.
Recordings were taken only on clear mornings, with small/no waves, little/no wind and an average water visibility of 15 m (which was measured using underwater distance markers). Samples were taken between 8–10 am on varying tidal conditions. Videos were taken from the same site 3 times (N = 3) over the 3 month research period, giving a total of 36 samples across all four habitat types. To account for the potential variability in conditions over the 3 month sampling period, recordings were taken evenly across all locations and sites over time (for example: day 1 = FSB site 1, day 2 = NAR site 1, day 3 = CR site 1, day 4 = MAR site 1, day 5 = FSB site 2 etc).
The first 5 minutes of each 25 minute recording were discarded due to possible initial disturbances to fish behaviour and to allow sediments to settle after the RUV unit had been deployed (following [55]). All videos were examined using Quicktime Media Player and only clearly identifiable individuals were recorded. Faunal identification of each 20 minute video was aided by the guide "Tropical Pacific Reef Fish Identification" [56] and in circumstances of uncertainty, advice was sought from local experts working at LATC. Fauna was identified to species level. As a relative measure of abundance, the maximum number of individuals seen in any frame (MaxN; following [57]) during the 20 minute video (each sampling period) was calculated. As a measure of species richness, the maximum number of species seen over the full 20 minute recording (was calculated following [58]). From the MaxN and number of species values of each recording, mean number of species and mean abundance were calculated for each site.
A generalised mixed model nested ANOVA was run separately for MaxN and number of species as dependent variables using the glmer function in the lme4 package in R [59]. Site was a random factor in the ANOVA, and the site was nested within the habitat type. A Poisson link function was used to account for the use of count data, and examination of fitted vs. residual plots indicated the data were appropriate for this statistical model. Significance was tested by dropping the main effect term and as comparing models, as detailed in [60]. Differences between habitat types were examined using post-hoc tests with Tukey corrections (using the emmeans package—Length 2021).
To explore community structure, PERMANOVA was run using PRIMER to assess the difference in mobile assemblages structure between habitat types using MaxN data [61] of the 24 key species highlighted in Table 2. The data was square-root transformed prior to use, to avoid the weighting of common species over rare. A Bray-Curtis resemblance matrix was used with 9999 permutations and PERMANOVA run with unrestricted permutation of raw data. This was followed by a pairwise test, which explored the significant differences between habitat types. Then, following [55], canonical analysis of principal coordinates (CAP) was used to visualise variation between habitat types and to highlight key species which differentiated the different ecological communities at the different habitat types.
There were significant differences with regards to number of species between habitat types. In terms of number of species, multiple comparisons (Table 1) revealed that all hard substrate habitats (CR, NAR and MAR) were significantly different to sand (p < 0.001 each time). The multiple comparisons showed no statistical difference between the CR and the MAR, as well as between the MAR and the NAR (p > 0.05 in all cases). There were no significant differences in abundance (MaxN) between any of the habitat types (Table 1; p > 0.05 in all cases).
Dependent Variable | Habitat Type | Habitat Type | Mean Difference (Column 2–Column 3) | Std. Error | Sig. | 95% Confidence Interval | 95% Confidence Interval |
Lower Bound | Upper Bound | ||||||
MaxN | Sand | NAR | 800.000 | 1.647.023 | 0.962 | −366.238 | 526.238 |
CR | 2.800.000 | 1.647.023 | 0.340 | −166.238 | 726.238 | ||
MAR | 1.244.444 | 1.647.023 | 0.874 | −321.794 | 570.683 | ||
NAR | SAND | −800.000 | 1.647.023 | 0.962 | −526.238 | 366.238 | |
CR | 2.000.000 | 1.647.023 | 0.622 | −246.238 | 646.238 | ||
MAR | 444.444 | 1.647.023 | 0.993 | −401.794 | 490.683 | ||
CR | SAND | −2.800.000 | 1.647.023 | 0.340 | −726.238 | 166.238 | |
NAR | −2.000.000 | 1.647.023 | 0.622 | −646.238 | 246.238 | ||
MAR | −1.555.556 | 1.647.023 | 0.781 | −601.794 | 290.683 | ||
MAR | SAND | −1.244.444 | 1.647.023 | 0.874 | −570.683 | 321.794 | |
NAR | −444.444 | 1.647.023 | 0.993 | −490.683 | 401.794 | ||
CR | 1.555.556 | 1.647.023 | 0.781 | −290.683 | 601.794 | ||
Number of Species | SAND | NAR | −18.22222* | 163.865 | 0.000 | −226.619 | −137.825 |
CR | −22.77778* | 163.865 | 0.000 | −272.175 | −183.381 | ||
MAR | −21.00000* | 163.865 | 0.000 | −254.397 | −165.603 | ||
NAR | SAND | 18.22222* | 163.865 | 0.000 | 137.825 | 226.619 | |
CR | −4.55556* | 163.865 | 0.043 | −89.953 | −0.1159 | ||
MAR | −277.778 | 163.865 | 0.343 | −72.175 | 16.619 | ||
CR | SAND | 22.77778* | 163.865 | 0.000 | 183.381 | 272.175 | |
NAR | 4.55556* | 163.865 | 0.043 | 0.1159 | 89.953 | ||
MAR | 177.778 | 163.865 | 0.701 | −26.619 | 62.175 | ||
MAR | SAND | 21.00000* | 163.865 | 0.000 | 165.603 | 254.397 | |
NAR | 277.778 | 163.865 | 0.343 | −16.619 | 72.175 | ||
CR | −177.778 | 163.865 | 0.701 | −62.175 | 26.619 | ||
Note: the mean difference is significant at 0.05 and all significant values are highlighted in bold. |
The total MaxN of 24 key species across all four habitat types made up > 95% of all individuals seen across all habitat types (Table 2) and provided a simplified measure of community structure for subsequent analysis (following [60]). Using these 24 species, PERMANOVA (Table 3) highlighted that there was a significant difference between habitat types (p < 0.001). The pairwise test (Table 4) showed that all habitat types were significantly different from each other (p < 0.001 in all cases).
Species | Scientific name | Number of surveys present (across all 36) | Total MaxN | |||
FSB | NAR | MAR | CR | |||
Lined-bristletooth surgeonfish | Ctenochaetus striatus | 25 | 4 | 38 | 24 | 42 |
Moorish idol | Zanclus cornutus | 24 | 0 | 17 | 12 | 12 |
Three spot damselfish | Dascyllus trimaculatus | 19 | 0 | 34 | 81 | 3 |
Black lip butterflyfish | Chaetodon kleinii | 17 | 0 | 11 | 9 | 9 |
Neon damselfish | Pomacentrus alleni | 16 | 0 | 280 | 101 | 1 |
Red tooth triggerfish | Odonus niger | 15 | 0 | 9 | 98 | 4 |
Checkerboard wrasse | Halichoeres hortulanus | 14 | 0 | 6 | 10 | 1 |
Indopacific-sergeant damselfish | Abudefduf vaigiensis | 13 | 0 | 53 | 82 | 54 |
Tricolour parrotfish | Scarus tricolor | 11 | 0 | 5 | 6 | 2 |
Blue streak wrasse | Labroides dimidiatus | 11 | 0 | 16 | 1 | 3 |
Bicolour-chromis damselfish | Chromis margaritifer | 10 | 0 | 6 | 3 | 146 |
Scaly chromis damselfish | Chromis lepidolepis | 10 | 0 | 65 | 0 | 5 |
Orange spine unicornfish | Naso lituratus | 9 | 0 | 1 | 0 | 15 |
Red mouth grouper | Aethaloperca rogaa | 9 | 0 | 9 | 3 | 3 |
Weber's chromis damselfish | Chromis weberi | 8 | 0 | 0 | 36 | 0 |
Chocolate grouper | Cephalopholis boenak | 8 | 0 | 0 | 7 | 2 |
Pearl scale angelfish | Centropyge vrolikii | 8 | 0 | 1 | 3 | 9 |
Canary wrasse | Halichoeres chrysus | 8 | 0 | 8 | 2 | 0 |
Moon wrasse | Thalassoma lunare | 8 | 0 | 12 | 1 | 0 |
Spotted garden eel | Heteroconger hassi | 8 | 764 | 0 | 0 | 0 |
Bridled monocle bream | Scolopsis bilineata | 7 | 0 | 0 | 4 | 3 |
Pale monocle bream | Scolopsis affinis | 7 | 0 | 0 | 15 | 0 |
Vagabond butterflyfish | Chaetodon vagabundus | 7 | 0 | 0 | 8 | 3 |
Tailspot wrasse | Halichoeres melanurus | 7 | 0 | 6 | 1 | 2 |
Source | df | SS | MS | Peudo-F | P (perm) |
Habitat | 3 | 69709 | 23236 | 21.570 | 0.0001 |
Pairs | Df | SS | R2 | F Model | p value |
FSB vs NAR | 1 | 3.746974 | 68.931574 | 0.8212830 | < 0.001 |
FSB vs CR | 1 | 3.541937 | 49.027088 | 0.7657242 | < 0.001 |
FSB vs MAR | 1 | 3.675652 | 55.874243 | 0.7883575 | < 0.001 |
NAR vs CR | 1 | 1.359395 | 13.690103 | 0.4610999 | < 0.001 |
NAR vs MAR | 1 | 0.751513 | 8.059874 | 0.3349924 | < 0.001 |
CR vs MAR | 1 | 1.417418 | 12.884445 | 0.4460686 | < 0.001 |
The CAP (Figure 6) highlights the considerably smaller magnitude of difference in community structure between the NAR and MAR (despite the statistical difference), with five species driving the majority of difference. As shown by total MaxN in Table 2, from these five species, three are pulling in a stronger direction towards the NAR. These were neon damselfish (280 on NAR, 101 on MAR), bluestreak wrasse (16 on NAR, 1 on MAR) and canary wrasse (8 on NAR, 2 on MAR). The CAP (Figure 6) showed that the community structure of the CR is different to all other habitat types, but is more closely related to the ARs than the FSB, and has four differentiating species (orange spine unicornfish, bicolor chromis, pearl scale angelfish and black lip butterflyfish). It also showed that community structure is most different between the FSB and all other habitats, driven largely by the spotted garden eel which was present in high numbers on the FSB but not in any other habitats (Table 2).
In terms of number of species, the results of this study showed a significant difference between all hard substrate habitats (ARs and coral reefs) and sand habitats. Mean number of species did not differ significantly between coral reefs and mature ARs. There were no significant differences in abundance (MaxN) between the habitats, as highlighted by figure 5b. The study also showed similarity (but a statistical difference) between artificial reefs and natural coral reefs, with a few species driving the differences. There was a large magnitude of difference in community structure between that flat sand bed and all other habitats.
As highlighted in a meta-review by [12], ARs can exhibit similarities to natural reefs in terms of fish density, biomass, number of species and diversity. Well-designed ARs can increase recruitment of juvenile and spawning fish because they provide refuge, bottom relief, heat and shading [28,36]. AR programs now often consider the requirements of local species with certain projects a being shown to specifically accommodate particular species through site selection, materials used, size and surface area, rugosity and vertical relief [62].
Figure 6 highlighted similarities between AR and CR community structure, a result that is shown by multiple other research papers (e.g. [25] and [12]). Additionally, despite having the highest number of species (Figure 5a), the CR had a lower MaxN than both AR sites, similar to the results of a Brazilian AR study [63]. As discussed by this study, this may be an indicator that coral reef substrata is limited and/or degraded in natural systems, resulting in the ARs offering new habitats that allow greater abundances of fish to colonise. As discussed by [64], new habitats such as ARs are initially sensed by roaming taxa using a variety of navigational senses (such as water chemistry, sound, vibration, light gradients, currents and water pressure). In the case of this study, it is likely that the AR would, in some part, have been colonised by roaming species in search of a more complex habitat.
Compared to the FSB, the AR and CR had greater habitat complexities, likely explaining
why they had higher a number of species (also shown by [34]). [63] showed that AR structures supported a higher number of species and abundance when their design is more complex. Additionally, [40] showed that ARs will be less successful if they fail to mimic the complexity, diversity or other important characteristics of natural reefs. Even when artificial reefs do effectively mimic natural reefs, it has been shown that communities between the two usually remain distinct [34,65].
As highlighted by Figure 6, despite showing similarities, the AR and CR did display differences in terms of fish communities. An example of this was the neon damselfish (Pomacentrus alleni), which was present in large populations on the NAR, yet found in low populations in other habitats (demonstrated by the strong pull towards the AR in Figure 6). Research has shown that adult reef damselfish are not reliant on coral substrata [28] and are frequently segregated by microhabitats, as they are less persistent than more dominant species on the reef [66]. [67] described damselfish as "omnivorous generalists", with potentially opportunistic diets and feeding plasticity, allowing them to populate environments that other species would not. In terms of their ability to be opportunistic, damselfish are unique compared to most species of reef fish, which generally have particular habitat requirements that depend upon certain coral species [68].
In contrast to the damselfish in this study, certain species from the RUV data were found colonising the CR only, for example the pearl scale angelfish (Centropyge vrolikii; as demonstrated by the strong pull towards the CR in Figure 6). [69] showed that angelfish can be highly specialist species, often relying on specific substrata such as corals and sponges as a food source. Until ARs can support the same benthic communities as CRs, it is unlikely that they will be colonised by specialist reef fish, and the distinct community differences will remain. This study has not focused on benthic recruitment on ARs, although its authors acknowledge that there is a limited amount of research on this topic in Indonesia, and tropical reefs in general. The importance of benthic recruitment on ARs (for example corals and sponges) must also be recognised because of their role in supporting the colonisation of many mobile species (see [70] and [71]).
In this study, some species were identified only in the FSB habitat, including the blacktip reef shark (Carcharhinus melanopterus) and tille trevally (Caranx tille). These are generally larger, deep water predatory species that do not require the protection from predators provided by the AR and this may explain why they are only present in the FSB. [72] discussed how large marine predators often utilize resources from different habitats, which usually involves feeding pelagically and resting inshore. The presence of larger predators in the FSB habitat may be because these species were resting during the day, before feeding in other habitats at night.
Additionally, the spotted garden eel (Heteroconger hassi) was the only species within this study that sustained large populations in the FSB habitat, without the presence of a hard substrate. Garden eels are known to reside in self-made burrows from which they protrude their bodies for feeding and courting [73]. They also use these burrows to retract in as a method of predator avoidance [74]. Therefore, unlike most species within this study, the garden eel does not require the structural protection provided by the hard substrata of an artificial or coral reef. It is also worth noting that they are relatively light and small in comparison to a large number of species that were identified on the CR and ARs. Previous studies (e.g. [27]) have aimed to calculate biomass (kg) of a reef and this can be used to compare habitats. However, this requires knowledge of fish length and weight and is therefore beyond the scope of this study. It is highly likely that, if biomass of mobile fauna was compared between habitats in this study, the FSB would have the lowest, despite having the highest overall abundance.
A recent study by [75], highlighted that given fish species frequently move between several different habitats, including sand flats and coral reefs at different times of day and for different purposes. From the RUV data of this study, it was clear that there were several species present in all three habitats, likely because they would move between each habitat for different purposes, such as finding food or shelter. The flat sand bottom, coral reef and new artificial reef were in close proximity to each other (each approximately 200 m apart) and it is possible that mobile species could be swimming between these habitats during the RUV recordings, potentially confounding the results. Despite this, the results show significant differences in community structure between each habitat type, meaning any non-independence of species at a site due to movement from another habitat type did not influence or weaken the significance of the findings. Furthermore, the closest similarity between sites were the two artificial reef sites, separated by more than 16 km away from each other. RUV rather than Baited-RUV was used, partly to prevent this exaggerated movement of species as a result of food. As such, non-independence of sites due to species moving between sites can be dismissed as a possible confounding factor in the results, although it should be noted, some degree of similarity of species between habitat types could be due to this movement between spatially close habitats.
This study compared ARs of different ages and it found that there were no significant differences between number of species and MaxN between the NAR (deployed for 1–1.5 years) and the MAR (deployed for 8–10 years). In terms of community structure, the results from CAP suggest that MAR and NAR communities (and therefore species colonising the structures) are relatively similar. AR fish recruitment rate is generally greatest within the first few months after construction and decreases with time [29,30,31]. [76] demonstrated that new fish populations can increase rapidly on tropical ARs within the first year after deployment, with colonisation rate slowing after this. All AR units within the NAR were at least 1 year old and are therefore likely to have already experienced optimal recruitment rates (as suggested by [76]). This may provide one reason why there is a non-significant difference in MaxN and number of species between the NAR and MAR.
Despite the relative closeness of the NAR and MAR highlighted by Figure 6, PERMANOVA did show that there was a significant difference between the habitat types. Many factors could account for this difference, such as age of the structures (as discussed by [33]) and distance (16 km) between locations. Another factor which may be explained by the difference in community structure, was the difference in fishing pressure between locations. The MAR in this study sits within an area where fishing is known to occur. In contrast, the NAR was within an community managed no-take zone (NTZ), which is well enforced and has high user compliance. As mentioned by local fishers, the community within Tianyar Village (location of the NAR) are highly supportive of the conservation program and its aims to restore localised marine biodiversity. This support from the community, likely explained why compliance of its MPA is high. Previous studies in Bali have shown that most of the islands ARs are regularly fished, resulting in several targeted fish species frequently missing from surveys [77] and it is of general agreement that ARs will have much greater increases in biomass and abundance when they are not subject to fishing pressure [32].
Table 2 showed that there are some species which are present on the NAR but not present/present in much lower numbers on the MAR. There were eight species that had a MaxN which was at least two times higher on the NAR, compared to the MAR. After a discussion with a local fisheries expert working on the MAR, it was revealed that six of these eight species are targeted by fishers there. These six species were neon damselfish (Pomacentrus coelestis), blue streak wrasse (Labroides dimidiatus), red mouth grouper (Aethaloperca rogaa), canary wrasse (Halichoeres chrysus), moon wrasse (Thalassoma lunare) and tailspot wrasse (Halichoeres melanurus). The harvesting of these species by local fishers on the MAR, likely explains why they have a much higher MaxN on the NAR (NTZ), and therefore likely explains the difference in community structure between the two ARs. This is further supported by the Table 2, which showed that the total MaxN of all six species is at least 2–3 times higher (much higher in several cases) on the NAR compared with the MAR.
This study is one of few initial evaluations of the use of ARs in Indonesia, and has highlighted their potential to provide localised increases in fish abundance and biodiversity. These results may be useful for communities particularly reliant on the ecosystem services provided coral reefs, especially those that have experienced a decline in the health of their natural reefs. The overall similarity in results between the NAR and MAR, as shown by Figures 5 and 6, as well as the non-significant differences shown between mean MaxN and number of species, suggest that ARs can generate near—immediate increases in fish abundance and biodiversity. However, due to the difference in fishing pressure between the NAR and MAR habitat types, it is not possible to directly compare them. Further work is needed to quantify ecological and socio-economic benefits of ARs, and the combined benefits of ARs and no-take MPAs. It is clear that local people can benefit from coral reef conservation, and that communities in North Bali, especially Tianyar village, support projects aiming to protect the marine environment. Further research to assess the extent and drivers of this support is recommended, and would provide valuable information to conservation projects that are aiming better involve the community.
A research permit was obtained from Indonesia's Ministry of Research (RISTEK). Research permit number: 34/TU.B5.4/SIP/VII/2021.
Location one was managed by LINI and location two by NBRC. Both NGOs, as well as their associated fisher community gave permission for this fieldwork to be conducted.
Zach Boakes, the first author of this paper, was supported by a studentship with Bournemouth University, UK. This research did not receive any other specific funding.
The authors declare no conflicts of interest.
[1] |
Arain S, Vryonides P, Abbasi M, et al. (2018) Reconfigurable bandwidth bandpass filter with enhanced out-of-band rejection using π-section loaded ring resonator. IEEE Microw Wirel Co 28: 28-30. https://doi.org/10.1109/LMWC.2017.2776212 doi: 10.1109/LMWC.2017.2776212
![]() |
[2] |
Cheng T, Tam K (2017) A wideband bandpass filter with reconfigurable bandwidth based on cross-shaped resonator. IEEE Microw Wirel Co 27: 909-91. https://doi.org/10.1109/LMWC.2017.2746679 doi: 10.1109/LMWC.2017.2746679
![]() |
[3] |
Zhang X, Wong S, Guo S, et al. (2020) Design of notched-wideband bandpass filters with reconfigurable bandwidth based on terminated cross-shaped resonators. IEEE Access 8: 37416-37427. https://doi.org/10.1109/ACCESS.2020.2975379 doi: 10.1109/ACCESS.2020.2975379
![]() |
[4] |
Sanchez-Soriano MA, Gomez-Garcia R, Torregrosa-Penalva G, et al. (2013) Reconfigurable-bandwidth bandpass filter within 10-50%. IET Microw Antenna P 7: 502-509. https://doi.org/10.1049/iet-map.2012.0274 doi: 10.1049/iet-map.2012.0274
![]() |
[5] |
Borja AL, Carbonell J, Martinez JL, et al. (2011) A controllable bandwidth filter using varactor-loaded metamaterial-inspired transmission lines. IEEE Antenn Wirel Pr 10: 1575-1578. https://doi.org/10.1109/LAWP.2012.2183111 doi: 10.1109/LAWP.2012.2183111
![]() |
[6] |
Chen N, Jeng SK (2012) Reconfigurable bandpass filter with separately relocatable passband edge. IEEE Microw Wirel Co 22: 559-561. https://doi.org/10.1109/LMWC.2012.2225606 doi: 10.1109/LMWC.2012.2225606
![]() |
[7] |
Wang XM, Bi XK, Guo SH, et al. (2020) Synthesis design of equal-ripple and quasi-elliptic wideband BPFs with independently reconfigurable lower passband edge. IEEE Access 8: 76856-76866. https://doi.org/10.1109/ACCESS.2020.2989449 doi: 10.1109/ACCESS.2020.2989449
![]() |
[8] |
Gomez-Garcia R, Guyette AC, Psychogiou D, et al. (2019) Quasi-elliptic multi-band filters with center-frequency and bandwidth tenability. IEEE Microw Wirel Co 26: 192-194. https://doi.org/10.1109/LMWC.2016.2526026 doi: 10.1109/LMWC.2016.2526026
![]() |
[9] |
Huang BC, Chen NW, Jeng SK (2014) A reconfigurable bandpass filter based on a varactor-perturbed, T-shaped dual-mode resonator. IEEE Microw Wirel Co 24: 297-299. https://doi.org/10.1109/LMWC.2014.2306893 doi: 10.1109/LMWC.2014.2306893
![]() |
[10] |
Sanchez-Renedo M, Gomez-Garcia R, Alonso JI, et al. (2005) Tunable combline filter with continuous control of center frequency and bandwidth. IEEE T Microw Theory 53: 191-199. https://doi.org/10.1109/TMTT.2004.839309 doi: 10.1109/TMTT.2004.839309
![]() |
[11] |
Wong PW, Hunter IC (2009) Electronically reconfigurable microwave bandpass filter. IEEE T Microw Theory 57: 3070-3079. https://doi.org/10.1109/TMTT.2009.2033883 doi: 10.1109/TMTT.2009.2033883
![]() |
[12] |
Tsai H, Chen N, Jeng S (2013) Center frequency and bandwidth controllable microstrip bandpass filter design using loop-shaped dual-mode resonator. IEEE T Microw Theory 61: 3590-3600. https://doi.org/10.1109/TMTT.2013.2280129 doi: 10.1109/TMTT.2013.2280129
![]() |
[13] |
Guo H, Hong J (2018) Varactor-tuned dual-mode bandpass filter with nonuniform Q distribution. IEEE Microw Wirel Co 28: 1002-1004. https://doi.org/10.1109/LMWC.2018.2870934 doi: 10.1109/LMWC.2018.2870934
![]() |
[14] |
Schuster C, Wiens A, Schmidt F, et al. (2017) Performance analysis of reconfigurable bandpass filters with continuously tunable center frequency and bandwidth. IEEE T Microw Theory 65: 4572-4583. https://doi.org/10.1109/TMTT.2017.2742479 doi: 10.1109/TMTT.2017.2742479
![]() |
[15] |
Kingsly S, Kanagasabai M, Alsath MG, et al. (2018) Compact frequency and bandwidth tunable bandpass-band stop microstrip filter. IEEE Microw Wirel Co 28: 786-788. https://doi.org/10.1109/LMWC.2018.2858005 doi: 10.1109/LMWC.2018.2858005
![]() |
[16] |
Ghaderi A, Golestanifar A, Shama F (2017) Design of a compact microstrip tunable dual-band bandpass filter. AEU-Int J Electron C 82: 391-396. https://doi.org/10.1016/j.aeue.2017.10.002 doi: 10.1016/j.aeue.2017.10.002
![]() |
[17] |
Qin W, Cai J, Li YL, et al. (2017) Wideband tunable bandpass filter using optimized varactor-loaded SIRs. IEEE Microw Wirel Co 27: 812-814. https://doi.org/10.1109/LMWC.2017.2734848 doi: 10.1109/LMWC.2017.2734848
![]() |
[18] | Karim MF, Guo YX, Chen ZN, et al. (2009) Miniaturized reconfigurable and switchable filter from UWB to 2.4 GHz WLAN using PIN diodes. In IEEE Microwave Symposium Digest. MTT-S International, 509-512. https://doi.org/10.1109/MWSYM.2009.5165745 |
[19] | Elelimy AM, El-Tager AM, Sobih AG (2013) A compact size switched reconfigurable tri-band BPF for modern wireless applications. In 56th International Midwest Symposium on Circuits and Systems (MWSCAS), 772-775. https://doi.org/10.1109/MWSCAS.2013.6674763 |
[20] |
Boutejdar A (2016) Design of 5 GHz-compact reconfigurable DGS-bandpass filter using varactor-diode device and coupling matrix technique. Microw Opt Techn Let 58: 304-309. https://doi.org/10.1002/mop.29561 doi: 10.1002/mop.29561
![]() |
[21] | Zhang ZC, Liu H (2018) A ultra-compact wideband bandpass filter using a quad mode stub-loaded resonator. Prog Electrom Res Le 77: 35-40. |
[22] |
Navya A, Immadi G, Narayana MV (2021) A Low-Profile Wideband BPF for Ku Band Applications, Prog Electrom Res Le 100: 127-135. https://doi.org/10.2528/PIERL21082101 doi: 10.2528/PIERL21082101
![]() |
[23] |
Ambati N, Immadi G, Narayana MV, et al. (2021), Parametric Analysis of the Defected Ground Structure-Based Hairpin Band Pass Filter for VSAT System on Chip Applications. Eng Technol Appl Sci 11: 7892-7896. https://doi.org/10.48084/etasr.4495 doi: 10.48084/etasr.4495
![]() |
1. | Zach Boakes, Luh Putu Mahyuni, Alice E. Hall, Marin Cvitanovic, Richard Stafford, Can Coral Reef Restoration Programmes Facilitate Changes in Environmental Attitudes? A Case Study on a Rural Fisher Community in North Bali, Indonesia, 2023, 51, 0300-7839, 891, 10.1007/s10745-023-00452-7 | |
2. | Zach Boakes, I. Gusti Ngurah A. Suryaputra, Alice E. Hall, Daniel J. Franklin, Richard Stafford, Nutrient dynamics, carbon storage and community composition on artificial and natural reefs in Bali, Indonesia, 2023, 170, 0025-3162, 10.1007/s00227-023-04283-4 |
Dependent Variable | Habitat Type | Habitat Type | Mean Difference (Column 2–Column 3) | Std. Error | Sig. | 95% Confidence Interval | 95% Confidence Interval |
Lower Bound | Upper Bound | ||||||
MaxN | Sand | NAR | 800.000 | 1.647.023 | 0.962 | −366.238 | 526.238 |
CR | 2.800.000 | 1.647.023 | 0.340 | −166.238 | 726.238 | ||
MAR | 1.244.444 | 1.647.023 | 0.874 | −321.794 | 570.683 | ||
NAR | SAND | −800.000 | 1.647.023 | 0.962 | −526.238 | 366.238 | |
CR | 2.000.000 | 1.647.023 | 0.622 | −246.238 | 646.238 | ||
MAR | 444.444 | 1.647.023 | 0.993 | −401.794 | 490.683 | ||
CR | SAND | −2.800.000 | 1.647.023 | 0.340 | −726.238 | 166.238 | |
NAR | −2.000.000 | 1.647.023 | 0.622 | −646.238 | 246.238 | ||
MAR | −1.555.556 | 1.647.023 | 0.781 | −601.794 | 290.683 | ||
MAR | SAND | −1.244.444 | 1.647.023 | 0.874 | −570.683 | 321.794 | |
NAR | −444.444 | 1.647.023 | 0.993 | −490.683 | 401.794 | ||
CR | 1.555.556 | 1.647.023 | 0.781 | −290.683 | 601.794 | ||
Number of Species | SAND | NAR | −18.22222* | 163.865 | 0.000 | −226.619 | −137.825 |
CR | −22.77778* | 163.865 | 0.000 | −272.175 | −183.381 | ||
MAR | −21.00000* | 163.865 | 0.000 | −254.397 | −165.603 | ||
NAR | SAND | 18.22222* | 163.865 | 0.000 | 137.825 | 226.619 | |
CR | −4.55556* | 163.865 | 0.043 | −89.953 | −0.1159 | ||
MAR | −277.778 | 163.865 | 0.343 | −72.175 | 16.619 | ||
CR | SAND | 22.77778* | 163.865 | 0.000 | 183.381 | 272.175 | |
NAR | 4.55556* | 163.865 | 0.043 | 0.1159 | 89.953 | ||
MAR | 177.778 | 163.865 | 0.701 | −26.619 | 62.175 | ||
MAR | SAND | 21.00000* | 163.865 | 0.000 | 165.603 | 254.397 | |
NAR | 277.778 | 163.865 | 0.343 | −16.619 | 72.175 | ||
CR | −177.778 | 163.865 | 0.701 | −62.175 | 26.619 | ||
Note: the mean difference is significant at 0.05 and all significant values are highlighted in bold. |
Species | Scientific name | Number of surveys present (across all 36) | Total MaxN | |||
FSB | NAR | MAR | CR | |||
Lined-bristletooth surgeonfish | Ctenochaetus striatus | 25 | 4 | 38 | 24 | 42 |
Moorish idol | Zanclus cornutus | 24 | 0 | 17 | 12 | 12 |
Three spot damselfish | Dascyllus trimaculatus | 19 | 0 | 34 | 81 | 3 |
Black lip butterflyfish | Chaetodon kleinii | 17 | 0 | 11 | 9 | 9 |
Neon damselfish | Pomacentrus alleni | 16 | 0 | 280 | 101 | 1 |
Red tooth triggerfish | Odonus niger | 15 | 0 | 9 | 98 | 4 |
Checkerboard wrasse | Halichoeres hortulanus | 14 | 0 | 6 | 10 | 1 |
Indopacific-sergeant damselfish | Abudefduf vaigiensis | 13 | 0 | 53 | 82 | 54 |
Tricolour parrotfish | Scarus tricolor | 11 | 0 | 5 | 6 | 2 |
Blue streak wrasse | Labroides dimidiatus | 11 | 0 | 16 | 1 | 3 |
Bicolour-chromis damselfish | Chromis margaritifer | 10 | 0 | 6 | 3 | 146 |
Scaly chromis damselfish | Chromis lepidolepis | 10 | 0 | 65 | 0 | 5 |
Orange spine unicornfish | Naso lituratus | 9 | 0 | 1 | 0 | 15 |
Red mouth grouper | Aethaloperca rogaa | 9 | 0 | 9 | 3 | 3 |
Weber's chromis damselfish | Chromis weberi | 8 | 0 | 0 | 36 | 0 |
Chocolate grouper | Cephalopholis boenak | 8 | 0 | 0 | 7 | 2 |
Pearl scale angelfish | Centropyge vrolikii | 8 | 0 | 1 | 3 | 9 |
Canary wrasse | Halichoeres chrysus | 8 | 0 | 8 | 2 | 0 |
Moon wrasse | Thalassoma lunare | 8 | 0 | 12 | 1 | 0 |
Spotted garden eel | Heteroconger hassi | 8 | 764 | 0 | 0 | 0 |
Bridled monocle bream | Scolopsis bilineata | 7 | 0 | 0 | 4 | 3 |
Pale monocle bream | Scolopsis affinis | 7 | 0 | 0 | 15 | 0 |
Vagabond butterflyfish | Chaetodon vagabundus | 7 | 0 | 0 | 8 | 3 |
Tailspot wrasse | Halichoeres melanurus | 7 | 0 | 6 | 1 | 2 |
Source | df | SS | MS | Peudo-F | P (perm) |
Habitat | 3 | 69709 | 23236 | 21.570 | 0.0001 |
Pairs | Df | SS | R2 | F Model | p value |
FSB vs NAR | 1 | 3.746974 | 68.931574 | 0.8212830 | < 0.001 |
FSB vs CR | 1 | 3.541937 | 49.027088 | 0.7657242 | < 0.001 |
FSB vs MAR | 1 | 3.675652 | 55.874243 | 0.7883575 | < 0.001 |
NAR vs CR | 1 | 1.359395 | 13.690103 | 0.4610999 | < 0.001 |
NAR vs MAR | 1 | 0.751513 | 8.059874 | 0.3349924 | < 0.001 |
CR vs MAR | 1 | 1.417418 | 12.884445 | 0.4460686 | < 0.001 |
Dependent Variable | Habitat Type | Habitat Type | Mean Difference (Column 2–Column 3) | Std. Error | Sig. | 95% Confidence Interval | 95% Confidence Interval |
Lower Bound | Upper Bound | ||||||
MaxN | Sand | NAR | 800.000 | 1.647.023 | 0.962 | −366.238 | 526.238 |
CR | 2.800.000 | 1.647.023 | 0.340 | −166.238 | 726.238 | ||
MAR | 1.244.444 | 1.647.023 | 0.874 | −321.794 | 570.683 | ||
NAR | SAND | −800.000 | 1.647.023 | 0.962 | −526.238 | 366.238 | |
CR | 2.000.000 | 1.647.023 | 0.622 | −246.238 | 646.238 | ||
MAR | 444.444 | 1.647.023 | 0.993 | −401.794 | 490.683 | ||
CR | SAND | −2.800.000 | 1.647.023 | 0.340 | −726.238 | 166.238 | |
NAR | −2.000.000 | 1.647.023 | 0.622 | −646.238 | 246.238 | ||
MAR | −1.555.556 | 1.647.023 | 0.781 | −601.794 | 290.683 | ||
MAR | SAND | −1.244.444 | 1.647.023 | 0.874 | −570.683 | 321.794 | |
NAR | −444.444 | 1.647.023 | 0.993 | −490.683 | 401.794 | ||
CR | 1.555.556 | 1.647.023 | 0.781 | −290.683 | 601.794 | ||
Number of Species | SAND | NAR | −18.22222* | 163.865 | 0.000 | −226.619 | −137.825 |
CR | −22.77778* | 163.865 | 0.000 | −272.175 | −183.381 | ||
MAR | −21.00000* | 163.865 | 0.000 | −254.397 | −165.603 | ||
NAR | SAND | 18.22222* | 163.865 | 0.000 | 137.825 | 226.619 | |
CR | −4.55556* | 163.865 | 0.043 | −89.953 | −0.1159 | ||
MAR | −277.778 | 163.865 | 0.343 | −72.175 | 16.619 | ||
CR | SAND | 22.77778* | 163.865 | 0.000 | 183.381 | 272.175 | |
NAR | 4.55556* | 163.865 | 0.043 | 0.1159 | 89.953 | ||
MAR | 177.778 | 163.865 | 0.701 | −26.619 | 62.175 | ||
MAR | SAND | 21.00000* | 163.865 | 0.000 | 165.603 | 254.397 | |
NAR | 277.778 | 163.865 | 0.343 | −16.619 | 72.175 | ||
CR | −177.778 | 163.865 | 0.701 | −62.175 | 26.619 | ||
Note: the mean difference is significant at 0.05 and all significant values are highlighted in bold. |
Species | Scientific name | Number of surveys present (across all 36) | Total MaxN | |||
FSB | NAR | MAR | CR | |||
Lined-bristletooth surgeonfish | Ctenochaetus striatus | 25 | 4 | 38 | 24 | 42 |
Moorish idol | Zanclus cornutus | 24 | 0 | 17 | 12 | 12 |
Three spot damselfish | Dascyllus trimaculatus | 19 | 0 | 34 | 81 | 3 |
Black lip butterflyfish | Chaetodon kleinii | 17 | 0 | 11 | 9 | 9 |
Neon damselfish | Pomacentrus alleni | 16 | 0 | 280 | 101 | 1 |
Red tooth triggerfish | Odonus niger | 15 | 0 | 9 | 98 | 4 |
Checkerboard wrasse | Halichoeres hortulanus | 14 | 0 | 6 | 10 | 1 |
Indopacific-sergeant damselfish | Abudefduf vaigiensis | 13 | 0 | 53 | 82 | 54 |
Tricolour parrotfish | Scarus tricolor | 11 | 0 | 5 | 6 | 2 |
Blue streak wrasse | Labroides dimidiatus | 11 | 0 | 16 | 1 | 3 |
Bicolour-chromis damselfish | Chromis margaritifer | 10 | 0 | 6 | 3 | 146 |
Scaly chromis damselfish | Chromis lepidolepis | 10 | 0 | 65 | 0 | 5 |
Orange spine unicornfish | Naso lituratus | 9 | 0 | 1 | 0 | 15 |
Red mouth grouper | Aethaloperca rogaa | 9 | 0 | 9 | 3 | 3 |
Weber's chromis damselfish | Chromis weberi | 8 | 0 | 0 | 36 | 0 |
Chocolate grouper | Cephalopholis boenak | 8 | 0 | 0 | 7 | 2 |
Pearl scale angelfish | Centropyge vrolikii | 8 | 0 | 1 | 3 | 9 |
Canary wrasse | Halichoeres chrysus | 8 | 0 | 8 | 2 | 0 |
Moon wrasse | Thalassoma lunare | 8 | 0 | 12 | 1 | 0 |
Spotted garden eel | Heteroconger hassi | 8 | 764 | 0 | 0 | 0 |
Bridled monocle bream | Scolopsis bilineata | 7 | 0 | 0 | 4 | 3 |
Pale monocle bream | Scolopsis affinis | 7 | 0 | 0 | 15 | 0 |
Vagabond butterflyfish | Chaetodon vagabundus | 7 | 0 | 0 | 8 | 3 |
Tailspot wrasse | Halichoeres melanurus | 7 | 0 | 6 | 1 | 2 |
Source | df | SS | MS | Peudo-F | P (perm) |
Habitat | 3 | 69709 | 23236 | 21.570 | 0.0001 |
Pairs | Df | SS | R2 | F Model | p value |
FSB vs NAR | 1 | 3.746974 | 68.931574 | 0.8212830 | < 0.001 |
FSB vs CR | 1 | 3.541937 | 49.027088 | 0.7657242 | < 0.001 |
FSB vs MAR | 1 | 3.675652 | 55.874243 | 0.7883575 | < 0.001 |
NAR vs CR | 1 | 1.359395 | 13.690103 | 0.4610999 | < 0.001 |
NAR vs MAR | 1 | 0.751513 | 8.059874 | 0.3349924 | < 0.001 |
CR vs MAR | 1 | 1.417418 | 12.884445 | 0.4460686 | < 0.001 |