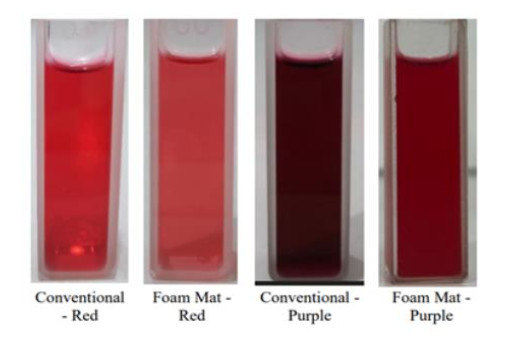
This study evaluated the color stability, bioactive compounds, and antioxidant activity of roselle calyx powders dissolved at different pHs. The results showed that purple roselle calyx powders revealed better color than red roselle, and foam-mat dried powders were better than the conventionally dried powder. The best solution without pH adjustment was found in foam-mat dried purple calyces. pH values significantly affected color stability and quality, bioactive compounds, and antioxidant activity of roselle powder solutions. The lightness, redness, and yellowness of the solutions at different pH values ranged from 27.00 to 74.45, from 0.70 to 52.20, and from 3.9 to 47.50, respectively. The non-adjusted pH solution had a lightness of 33.80–49.05, redness of 19.15–42.45, and yellowness of 7.10–18.35. The color quality, indicated by color intensity at different pHs, ranged from 0.03 to 15.24; the non-adjusted pH solution had a color intensity of 1.37–33.48, revealing that pH decreased color quality. Retention of phenolics and flavonoids was 8.02%–99.69% and 0.00%–81.05%, respectively, indicating the instability of bioactive compounds. Roselle antioxidant activity was observed at different pHs, ranging from 0.00 to 1.11 mg Trolox equivalent (TE)/g for DPPH (2, 2-diphenyl-1-picrylhydrazyl) scavenging activity and 0.46–104.16 mg TE/g for the ABTS [2, 2'-azino-bis(3-ethylbenzothiazoline-6-sulfonic acid)] assay. Acidic pHs preserved better all dependent parameters. In practice, the pH value should be considered when using roselle calyx powders in food products. This study used a solution as a model for a watery food system. Suitable products to use roselle calyx powders are water-based products with an acidic pH, such as syrups, yogurts and yogurt-based products, fermented milk, and sour-taste beverages. Roselle calyx powders are suitable to use as coloring agents or as bioactive compounds to provide healthy functional properties. The use of foam-mat dried powders is suggested due to the better antioxidant activity and color preservation than conventionally dried powder.
Citation: Teti Estiasih, Jatmiko Eko Witoyo, Khofifah Putri Wulandari, Fadhillah Dwi Juniati, Widiastuti Setyaningsih, Hanifah Nuryani Lioe, Miguel Palma, Kgs Ahmadi, Hamidie Ronald Daniel Ray, Elya Mufidah. Stability comparison of conventional and foam-mat red and purple dried roselle calyces powder as a function of pH[J]. AIMS Agriculture and Food, 2025, 10(1): 177-198. doi: 10.3934/agrfood.2025010
[1] | Febiani Dwi Utari, Dessy Agustina Sari, Laeli Kurniasari, Andri Cahyo Kumoro, Mohamad Djaeni, Ching-Lik Hii . The enhancement of sappanwood extract drying with foaming agent under different temperature. AIMS Agriculture and Food, 2023, 8(1): 214-235. doi: 10.3934/agrfood.2023012 |
[2] | Hathairat Chokthaweepanich, Chuleeporn Chumnanka, Sribud Srichaijaroonpong, Rungnapa Boonpawa . Effect of harvesting age and drying condition on andrographolide content, antioxidant capacity, and antibacterial activity in Andrographis paniculata (Burm.f.) Nees. AIMS Agriculture and Food, 2023, 8(1): 137-150. doi: 10.3934/agrfood.2023007 |
[3] | Rossaporn Jiamjariyatam, Orachorn Mekkerdchoo, Pakkapong Phucharoenrak, Lu Zheng . Effects of freeze-drying and vacuum-drying on the quality, total phenolic contents, and antioxidant activities of bee honey in northern Thailand. AIMS Agriculture and Food, 2024, 9(2): 430-444. doi: 10.3934/agrfood.2024025 |
[4] | Ebrahim Falahi, Zohre Delshadian, Hassan Ahmadvand, Samira Shokri Jokar . Head space volatile constituents and antioxidant properties of five traditional Iranian wild edible plants grown in west of Iran. AIMS Agriculture and Food, 2019, 4(4): 1034-1053. doi: 10.3934/agrfood.2019.4.1034 |
[5] | Thi Thuy Le, Trung Kien Nguyen, Nu Minh Nguyet Ton, Thi Thu Tra Tran, Van Viet Man Le . Quality of cookies supplemented with various levels of turmeric by-product powder. AIMS Agriculture and Food, 2024, 9(1): 209-219. doi: 10.3934/agrfood.2024012 |
[6] | María Quelal, Elena Villacrés, Karla Vizuete, Alexis Debut . Physicochemical characterization of sangorache natural colorant extracts (Amaranthus quitensis L.) prepared via spray- and freeze-drying. AIMS Agriculture and Food, 2023, 8(2): 343-358. doi: 10.3934/agrfood.2023019 |
[7] | Jaquelinne Pires Vital da Costa, Camila Fernanda Dias de Oliveira, Francielo Vendruscolo . Cheese whey as a potential substrate for Monascus pigments production. AIMS Agriculture and Food, 2020, 5(4): 785-798. doi: 10.3934/agrfood.2020.4.785 |
[8] | Luh Suriati, I Made Supartha Utama, Bambang Admadi Harsojuwono, Ida Bagus Wayan Gunam . Incorporating additives for stability of Aloe gel potentially as an edible coating. AIMS Agriculture and Food, 2020, 5(3): 327-336. doi: 10.3934/agrfood.2020.3.327 |
[9] | Prakasit Duangpapeng, Abil Dermail, Khundej Suriharn . Phenolics, anthocyanins, and antioxidant capacity in the tassels of purple waxy corn: Effects of temperature and time during storage. AIMS Agriculture and Food, 2024, 9(1): 69-83. doi: 10.3934/agrfood.2024005 |
[10] | Namfon Samsalee, Rungsinee Sothornvit . Physicochemical, functional properties and antioxidant activity of protein extract from spent coffee grounds using ultrasonic-assisted extraction. AIMS Agriculture and Food, 2021, 6(3): 864-878. doi: 10.3934/agrfood.2021052 |
This study evaluated the color stability, bioactive compounds, and antioxidant activity of roselle calyx powders dissolved at different pHs. The results showed that purple roselle calyx powders revealed better color than red roselle, and foam-mat dried powders were better than the conventionally dried powder. The best solution without pH adjustment was found in foam-mat dried purple calyces. pH values significantly affected color stability and quality, bioactive compounds, and antioxidant activity of roselle powder solutions. The lightness, redness, and yellowness of the solutions at different pH values ranged from 27.00 to 74.45, from 0.70 to 52.20, and from 3.9 to 47.50, respectively. The non-adjusted pH solution had a lightness of 33.80–49.05, redness of 19.15–42.45, and yellowness of 7.10–18.35. The color quality, indicated by color intensity at different pHs, ranged from 0.03 to 15.24; the non-adjusted pH solution had a color intensity of 1.37–33.48, revealing that pH decreased color quality. Retention of phenolics and flavonoids was 8.02%–99.69% and 0.00%–81.05%, respectively, indicating the instability of bioactive compounds. Roselle antioxidant activity was observed at different pHs, ranging from 0.00 to 1.11 mg Trolox equivalent (TE)/g for DPPH (2, 2-diphenyl-1-picrylhydrazyl) scavenging activity and 0.46–104.16 mg TE/g for the ABTS [2, 2'-azino-bis(3-ethylbenzothiazoline-6-sulfonic acid)] assay. Acidic pHs preserved better all dependent parameters. In practice, the pH value should be considered when using roselle calyx powders in food products. This study used a solution as a model for a watery food system. Suitable products to use roselle calyx powders are water-based products with an acidic pH, such as syrups, yogurts and yogurt-based products, fermented milk, and sour-taste beverages. Roselle calyx powders are suitable to use as coloring agents or as bioactive compounds to provide healthy functional properties. The use of foam-mat dried powders is suggested due to the better antioxidant activity and color preservation than conventionally dried powder.
Abbreviations: ABTS: 2,2- azinobis-3-ethylbenzothiazoline-6-sulfonic acid; BI: browning index; CI: color intensity; DPPH: 1,1-diphenyl-2-picrylhydrazil; SSL: sodium stearoyl lactylate; TAC: total anthocyanin content; TE: Trolox equivalent; TPC: total phenolic content; VI: violet index
Edible flowers have been used for food and medicine since Ancient Rome and Greece, having nutritional and functional benefits like phytochemicals for promoting good health [1]. The roselle (Hibiscus sabdariffa L.) flower is a popular edible flower widely cultivated and readily available worldwide. Roselle calyces, a crucial component of the plant, are abundant in bioactive compounds like organic acids, phenolic compounds, anthocyanins, and minerals [2]. These bioactive compounds make roselle calyces a valuable source of pharmacological properties with potential health benefits and therapeutic applications [3]. The roselle can be enjoyed directly or used in products such as herbal teas, cakes, candies, syrup, jam, or functional drinks [4]. Its rich anthocyanin content makes it a popular choice for natural food coloring in various products [5]. Like other horticultural products, fresh roselle flowers have a short shelf life and are not stable; processing dried roselle calyces aims to combat this problem. Transforming fresh roselle calyces or their extract into powder via drying processes allows for preserving, easily storing, and further using roselle in various products [6].
The conventional drying method, which utilizes direct heat from sources like sunlight, fire, or electricity, is widely employed. However, this approach has its disadvantages, like requiring prolonged drying temperatures and times, which can result in thermal deterioration of the color, aroma, bioactive compounds, and physicochemical and organoleptic characteristics of roselle products, including low solubility [7,8]. Drum drying is a drying technique that is limited by long exposure to the high-heated surface of the drum. Meanwhile, spray drying is a suitable method due to the short heating time, but the equipment is quite costly. An effective technique is foam-mat drying, where liquid materials are combined with foaming agents and foam stabilizers to produce a stable foam during drying. The benefit of this method is that it hastens the drying process by increasing the surface area of the foam compared to the liquid material. Consequently, the bioactive compounds of roselle are protected from thermal damage, while the resulting solubility is notably high [9]. Moreover, food processing, specifically of fresh roselle calyces and their extracts, might be influenced by various factors such as pH, temperature, aeration, kneading, and processing time. Food can be processed under different pH conditions (i.e., acidic, neutral, or alkaline) [10], thus possibly affecting the stability of roselle powder.
Both red and purple varieties of roselle calyces contain high levels of anthocyanins and other phytochemicals sensitive to pH changes [2,11,12]. Our previous study revealed that the red and purple foam-mat dried roselle calyx powder has a considerable amount of total phenolics content and anthocyanins [13]. However, different pH environments and heat treatment are often applied during food processing, and both factors are frequently detrimental to anthocyanins [12]. The color of anthocyanins is affected by the pH due to the ionic nature of their molecular structure. Anthocyanins are found in different chemical forms, depending on the pH of the solution [14]. Friedman and Jurgens [15] showed that some phenolic compounds such as chlorogenic, gallic, and caffeic acids are not stable in high pHs. The molecular structure changes of anthocyanins in different pH affected their antioxidant activity [16]. The pH sensitivity of antioxidant compounds in food is influenced by the food matrix [17].
Foam-mat dried powder is prepared by water extraction of fresh roselle calyces, foaming by an emulsifier, stabilizing by maltodextrin, and drying the foam [13]. As such, a matrix different from conventionally dried roselle powder is formed that does not involve water extraction. The matrix of conventionally dried powder has a similar composition to that of fresh calyces in water (86.08%, protein (2.95%), fat (0.90%), carbohydrates (6.21%), and crude fiber (2.59%) [18]. Rocha et al [19] reported the presence of 15%–28% mucilage in polysaccharides, depending on roselle varieties. These differences might affect the color, bioactive compounds, and antioxidant activity of the roselle calyx powder. A previous study explored the stability of roselle anthocyanin extract subjected to pH and thermal treatments [12]. This study aims to evaluate the stability of foam-mat and conventionally dried roselle calyx powder solutions at pH 2–13. Although extreme pH values (i.e., high alkaline and very low acidic pHs) are rarely found in food processing, this research will contribute to knowledge about the stability of anthocyanin and phenolic compounds. The main difference between this study and that by Wu et al. [12] is that the latter studied the stability of roselle calyx extracts, while this study will evaluate the stability of powder after water dissolution to obtain different solution pHs. The solutions are prepared from different powder matrices at different pH values that might imply differences on antioxidant activity and color. This study will provide an overview of the roselle powder stability when subjected to different pH values in watery systems during food processing and aid in the selection of the best pH for processing to preserve color, bioactive compounds, and antioxidant activity. The results of this study will guide the food industry in producing roselle powder–containing food products.
Fresh red and purple calyces (the varieties were not identified, and there was no local name available) were obtained from a local farmer. The fresh roselle calyces were collected at the same time (only once) to avoid bias due to different harvesting times. The maturity of roselle calyces was indicated by a size of ±5 cm in length and ±4 cm in width. Before processing into powder, fresh roselle calyces were stored at −18 ℃ to prevent undesirable changes. Maltodextrin (Xingmao, China) with a DE (dextrose equivalent) of 10–12 was used as foam-mat filler and stabilizer. Commercial sodium stearoyl lactylate (SSL) was used as a foaming agent. All chemical reagents (at analytical grades) were purchased from Merck (Darmstadt, Germany) and Sigma-Aldrich (St. Louis, MO, USA).
Fresh red and purple calyces were dried at 60 ℃ in a cabinet dryer until reaching a constant weight (approximately 10–12 h). The dried roselle calyces were then pulverized in a blender (Phillips HR-211) and sieved through 30 mesh sieves. The powder was stored in aluminum foil bags before further use.
Foam-mat dried roselle calyx powder was prepared by blending 100 g of fresh roselle calyces with water in a ratio of 4:10 w/v for 3 min (based on our preliminary research). The roselle calyx water extract was obtained after filtering with filter paper and adding food-grade maltodextrin as a foam stabilizer at a concentration of 10% w/v. The mixture was homogenized in an Ultra-turrax IKA T18 basic homogenizer for 1 min at 6000 rpm. SSL (at a concentration of 1% w/v) was added and the mixture was homogenized again at 14,000 rpm for 7 min. The resulting foam was spread thinly (about 1 mm) in trays and dried for 6 h at 60 ℃ in a cabinet dryer (local design, no brand) with an airflow rate of 8 m/s as specified by the dryer. The position of the trays in the dryer during drying was changed regularly for a uniform heating temperature. Finally, the dried foam sheet was ground and sieved using a 30 mesh to obtain foam-mat dried roselle calyx powder. After drying, the moisture content of the red and purple dried roselle was 9.45% ± 0.1% and 7.58% ± 0.05%, respectively, and that of red and purple foam-mat-dried calyx powder was 6.40% ± 0.33% and 6.16% ± 0.00%, respectively. The powder was stored at −18 ℃. The analysis was conducted for three months after powder production.
The pH of roselle calyx powder was adjusted by dissolving 1 g of sample in 10 mL (0.1 g/mL or 10% w/v) of hot water (80–100 ℃) and then homogenizing. The solution was filtered using a filter paper to separate the precipitate and filtrate. The filtrate was then transferred into a 25 mL measuring cup and the pH was adjusted from pH 2 to pH 13 using HCl 0.1 N or 0.5 N or NaOH 0.1 N, 1 N, or 10 N. The final volume was 15 mL and the final ratio of powder:liquid was 1:15 w/v. Each pH treatment was replicated three times. An equilibrium time of 1 min was allowed for each measurement before the pH value was recorded. pH stability during measurement was ensured by periodically standardizing with a pH 4 buffer solution. The untreated solution of roselle calyx powder was prepared similarly but without pH adjustment.
Color stability was evaluated by measuring the L*, a*, and b* values of the pH-adjusted (pH of 2–13) and non-adjusted solutions of conventionally or foam-mat dried roselle calyx powder by using a chromameter (Minolta Chroma Meter CR-100, Japan). Color quality, including color intensity (CI), violet index (VI), and browning index (BI) were assessed by the method outlined by Marpaung & Paramaputri [20], and the results were calculated using Equations 1–3.
CI=(Aλmax−A700)xDF | (1) |
VI=(A580−A700)/(A520−A700) | (2) |
BI=(A420−A700)/(Aλmax−A700) | (3) |
where Aλmax is the absorbance at the wavelength of maximum absorbance; A580 is the absorbance at 580 nm; A520 is the absorbance at 520 nm; A420 is the absorbance at 420 nm; and A700 is the absorbance at 700 nm.
The pH differential method determined the total anthocyanin content (TAC) [21]. Untreated or pH-treated sample solutions (1 mL) were mixed with 25 mmol/L of potassium chloride pH 1 and 0.4 mol/L of sodium acetate pH 4.5 buffers, followed by incubation for 15 min at room temperature (25 ± 3 ℃). The absorbance was measured at 700 and 510 nm. The absorbance variation (A) was calculated using the formula A = [A510 − A700] pH 1.0 − [A510 − A700] pH 4.5. Equation 4 was used to get the total anthocyanin content.
TAC=(AxMWxDFxVX1000)ϵxL | (4) |
where A is the absorbance difference, DF is the dilution factor, V is the total volume of extract (mL), L is the cell width (1 cm), ε is the coefficient of molar extinction for cyanidin-3-glucoside (26,900 L/mole-cm), MW is the molecular weight of cyanidin-3-glucoside (449.2 g/mole), and TAC is the total anthocyanin content (mg/g). Anthocyanin retention was determined using the method described by Huang et al. [22] and calculated using Equation 5.
TACRetention(%)=TotalanthocyanincontentafterpHtreatmentTotalanthocyanincontentwithoutpHtreatmentx100 | (5) |
The total phenolic content (TPC) was measured by the Folin–Ciocalteu method, as described by Ifie et al. [23]. Namely, 5 mL of Folin-Ciocalteu's phenol reagent (10%) was added to 1 mL of the untreated and pH-treated roselle calyx powders, which were then incubated in the dark for 5 min. Sodium carbonate solution (75 g/L; 4 mL) was added and the mixture was incubated for 30 min in the dark at room temperature. Finally, the absorbance was measured at 752 nm. Gallic acid was used as the standard to estimate the total phenolic content. The retention of TPC was calculated by dividing the TPC in the pH-adjusted solution with the TPC in the solution without adjusting pH using Equation 6.
TPCRetention(%)=TotalphenoliccontentafterpHtreatmentTotalphenoliccontentwithoutpHtreatmentx100 | (6) |
The antioxidant activity of conventional and foam-mat dried roselle calyx powder was estimated using the DPPH assay and expressed as inhibition percentage (%) and equivalency to Trolox (mg Trolox equivalent/g). The procedure was done following the method by Baliyan et al. [24]; however, as that method used a solid sample and the present study used a solution, slight modifications were made to sample quantity. Untreated and pH-treated sample solutions of 0.5 mL were mixed with 3.5 mL of methanol and 1 mL of 0.2 mM DPPH in methanol and incubated in the dark for 30 min. The absorbance was then measured at a wavelength of 517 nm (As). The antioxidant activity (mg TE/g) was estimated using the Trolox standard curve and expressed as mg Trolox Equivalent (TE)/g [23]. For the DPPH assay, the Trolox standard curve was built at concentrations ranging from 0 to 125 mM and treated as samples.
The method by Thaipong et al. [25] was used to measure the ABTS radical scavenging activity, with minor modifications in absorbance and sample quantity. Freshly prepared ABTS reagent was created by combining ABTS and potassium persulfate solutions in a 1:1 (v/v) ratio and incubating in a dark room for 12–16 h before use. After incubation, 1 mL of the mixture solution was mixed with 50 mL of methanol to create the ready-to-use reagent (absorbance 0.70 ± 0.02). Untreated and pH-treated solutions of roselle calyx powders (0.1 mL) were diluted with 10 mL of methanol. The diluted sample of 0.15 mL was combined with 2.85 mL of the ABTS reagent, and the mixture was incubated for 30 min in the dark. Absorbance was read at 745 nm (As). The antioxidant activity (in mg TE/g) was estimated using the Trolox standard curve, and the antioxidant activity was expressed as mg TE/g. The Trolox standard curve was built at 0–300 mM concentrations, with the same procedure as the sample.
The statistical analysis of the treatments (three replicates) was performed using ANOVA at a significance value of p < 0.05; Tukey's test was applied. Differences in color were analyzed by MANNOVA. The software for statistical analysis was Minitab 17.
A roselle calyx powder dissolved in water without any pH adjustment was used as the control. The color of this roselle calyx powder solution is shown in Figure 1. The foam-mat dried roselle calyx powder solution was brighter than the conventionally dried roselle due to the water extraction during preparation. Conventionally dried purple roselle calyces exhibited a reddish-purple color, while foam-mat dried roselle powder presented a red color. The appearance of both powder solutions was quite similar (Figure 1). Shruthi et al. [24] reported an attractive red color of roselle calyx water extract. The conventional method used harsher conditions, such as longer drying times, compared to the foam-mat method, which also had the protective effect from the maltodextrin.
The color values presented significant differences (p < 0.05): the foam-mat dried powder presented higher lightness, redness, and yellowness compared to the conventionally dried powder. Extraction only dissolved the water-soluble compounds; using maltodextrin as the filler also lightened the color. This interesting difference is possibly related to the type of dissolved anthocyanin in water. Anthocyanins present in the roselle have been identified as delphinidin 3-glucoside, cyanidin 3-sambubioside, delphinidin 3-sambubioside, and cyanidin 3-glucoside [26]. Song et al. [27] reported the presence of cyanidin-3-sambubioside, cyanidin-3-glucoside, delphinidin-3-glucoside, pelargonidin-3-glucoside, and petunidin-3-glucoside in a roselle extract. Yang et al. [28] reported two types of anthocyanin in a roselle extract: delphinidin-3-O-sambubioside and cyanidin-3-O-sambubioside. Unfortunately, these works did not describe the types of roselle and extraction solvent. Cyanidin, delphinidin, pelargonidin, and petunidin present different colors; cyanidin is reddish-purple (magenta), delphinidin is blue-reddish or purple, pelargonidin is red-colored, and petunidin is dark-red or purple [29]. Delphinidin seems to be mainly responsible for the color of purple roselle calyces, and cyanidin is the most influential anthocyanin for the color of the red roselle. Possibly, a mixture of anthocyanins is present in the roselle calyces; therefore, the color of foam-mat dried purple roselle powder differed significantly when compared to the corresponding conventionally dried powder (p < 0.05) due to the fact that not all anthocyanins properly dissolved during extraction.
The foam mat and conventionally dried powder solutions presented higher lightness than the roselle water extract reported by Aishah et al. [30] (29.55). This indicates that water extraction at a roselle-to-water ratio of 1:2 (w/v) resulted in higher anthocyanins than when dissolving roselle calyx powders in water at 10%.
The foam-mat dried purple roselle calyx powder solution presented lower color intensity (CI) than the red powder (Table 1); this CI remarkably decreased when the powder was processed by foam-mat drying (p < 0.05). CI represents the quality of color based on color intensity. Another common parameter for determining anthocyanin color quality is the violet index (VI), which measures the ratio of purple color intensity to red color intensity [31]. Table 1 shows that the VI of the red calyx powder solution was higher than the purple one (p < 0.05). It is hypothesized that the purple calyces comprise a mixture of anthocyanins that exhibit high purple and magenta intensity, thus resulting in lower VI. The browning index (BI) is a parameter for anthocyanin degradation [32]. Due to the higher anthocyanin content, purple calyx powders had a lower browning index, indicating that degradation was more intensive than in red calyces. Presumably, the abundant high-intensity red and purple anthocyanins in the purple calyces might contribute to its deep color.
Parameter | Conventionally dried | Foam-mat dried | ||
Red | Purple | Red | Purple | |
Color | ||||
Lightness (L) | 47.45 ± 0.01b | 33.80 ± 0.00d | 49.05 ± 0.35a | 37.88 ± 2.86c |
Redness (+a) | 19.15 ± 0.00d | 34.38 ± 0.32c | 39.95 ± 0.49b | 42.45 ± 3.04a |
Yellowness (+b) | 7.10 ± 0.00d | 15.68 ± 0.11b | 12.25 ± 0.35c | 18.35 ± 0.78a |
Color quality | ||||
Color intensity (CI) | 8.36 ± 0.01b | 33.48 ± 0.81a | 1.37 ± 0.04d | 6.74 ± 0.14c |
Violet index (VI) | 0.15 ± 0.00b | 0.14 ± 0.01c | 0.18 ± 0.00a | 0.14 ± 0.00c |
Browning index (BI) | 0.39 ± 0.00b | 0.38 ± 0.02c | 0.58 ± 0.00a | 0.35 ± 0.02d |
Total phenolic content (mg GAE/mL) | 6.52 ± 0.03b | 23.07 ± 0.02a | 2.47 ± 0.03d | 5.37 ± 0.02c |
Total anthocyanin content (mg/mL) | 2.34 ± 0.08c | 12.03 ± 0.02a | 0.57 ± 0.02d | 3.78 ± 0.00b |
Antioxidant activity | ||||
DPPH assay | ||||
Trolox equivalent (mg TE/g) | 0.86 ± 0.02a | n.d. | 0.85 ± 0.00b | 0.68 ± 0.00c |
ABTS assay | ||||
Trolox equivalent (mg TE/g) | n.d. | 3.91 ± 0.02b | n.d. | 11.94 ± 0.14a |
Note: n.d. = not detected. Different letters indicate statistical differences for the data in the same row. |
The roselle calyx powder solutions preserved the phenolics and anthocyanins compounds and revealed antioxidant activity, except for the conventionally dried purple roselle, which did not show scavenging activity to DPPH. Not all sample solutions exhibited antioxidant activity equivalent to Trolox, which might occur due to very low antioxidant activity compared to Trolox. The dissolution in water and filtering the solution might reduce the antioxidant compounds, although phenolic and anthocyanin compounds were still detected in the solution.
Table 1 shows the DPPH and ABTS scavenging activity of dried roselle calyx powder dissolved in water without pH adjustment. In general, better antioxidant activity was found for conventionally dried than foam-mat dried red roselle powder (p < 0.05). However, the foam-mat dried purple roselle presented better antioxidant activity than the conventionally dried (p < 0.05). Different phenomena of different colors of roselle powder may be related to higher phenolics and anthocyanins in conventionally dried powder (p < 0.05). Both compounds were much higher in purple than red roselle. Interestingly, the purple foam-mat dried roselle powder solution exhibited better antioxidant activity than the conventionally dried (p < 0.05). The high phenolic content might be attributed to the lower antioxidant activity. At high concentrations, some phenolic compounds exhibit pro-oxidant activity [33,34,35]. Phenolics are primary antioxidants that donate electrons to the free radicals and become weak free radicals. The abundance of weak free radicals due to high phenolic concentration leads to pro-oxidant activity, thus reducing antioxidant activity.
The color of roselle calyx powder dissolved at different pH values (from 2 to 13) is shown in Figure 2. All solutions exhibited red color at low pH (2–3) except for conventionally dried purple roselle calyces, which had a purplish color. The lightness, redness, and yellowness of the solution at different pH values ranged from 27.00 to 74.45, from 0.70 to 52.20, and from 3.9 to 47.50, respectively. Meanwhile, the non-adjusted pH solution had a lightness of 33.80–49.05, redness of 19.15–42.45, and yellowness of 7.10–18.35. Foam-mat dried calyx powder had a lighter color than the conventionally dried (p < 0.05) because its preparation involved extraction by water and, thus, not all bound pigments were extracted. Similarly, the red roselle calyx powder produced a lighter solution than the purple roselle. The pattern of color changes was quite different between powder types. The purple or red color appeared at an acidic pH and the bluish color at an alkaline pH; increasing alkaline pH changed the color into yellowish. No color was found at pH 10 for foam-mat dried red roselle calyx powder.
Figure 3 shows that redness (+a) sharply decreased at pH 5–7 depending on the powder types, gradually increasing at higher pH and then declining at pH 13 (p < 0.05). The yellowness (+b) presented a more or less similar pattern: there was a high +b value at a very acidic pH that gradually decreased until reaching a minimum at pH 4–6 depending on the type of powder, and then increased to reach a maximum at pH 13. A different pattern was found for lightness (L*), which increased with increased pH and achieved a small peak at pH 5–8 (depending on the type of powder), and then continued to gradually increase at very high pH.
Aishah et al. [30] reported lightness changes of roselle water extract from pH 2.0 to pH 4.5; lightness increased at pH 2 and decreased to a minimum at pH 4.5. A different patterns was found in this study, in which lightness increased to achieve a peak at pH 5–8. The different roselle concentrations between both studies resulted in different anthocyanin concentrations, which probably explain the distinct findings. Wu et al. [36] showed that the lightness of roselle anthocyanin water extract increased from pH 1 to pH 5 and decreased thereafter. Meanwhile, the +a value decreased sharply from pH 1 to 6 and then increased at pH 7. These changes in +a are in accordance with the findings of the present study. However, a different pattern was found for the +b value: in Wu et al. [36], it decreased from pH 1 to 7 while in this work, it decreased to a minimum at pH 4 or 5 and then increased (Figure 3). Different anthocyanin concentration probably explain the different results. The color expression due to changes in anthocyanin-derived compounds at different pHs seem to be concentration-dependent.
Anthocyanin contributed to the color changing for these solutions due to the different structure at different pHs. According to Horbowicz et al. [37], anthocyanins are intensely orange or red under acidic pH (below 2), but colorless at higher pHs; in alkaline pH, the color changes to bluish. Therefore, *L tended to increase and then decreased at alkaline pHs. At very low pH, anthocyanin presents as a flavylium cation, but at neutral pH uncharged quinones appear. At basic pHs, degradation with a different pathway is likely to occur, producing colorless compounds [16]. Anionic quinoidal species form as pH levels increase, resulting in colorless carbinol pseudo-base and chalcone structures [29].
Minimal coloration was observed at pH 4–5 due to the small amount of quinoidal anion and flavylium cation present in dried roselle calyx powder solutions. At neutral pH levels, deprotonation of the quinoidal species results in resonance-stabilized quinonoid anions, giving anthocyanins their characteristic purple color [29]. Punyachareonnon et al. [38] reported that an anthocyanin solution displayed a violet/blue color in alkaline conditions (pH > 8) due to the formation of quinoidal bases in the R-A-O form. At the same time, the quantity of carbinol pseudo-base decreased, resulting in rapid color formation, thus increasing the +a and +b values.
Foam-mat dried roselle calyx powders, both red and purple, presented lighter colors but exhibited different appearances at similar pHs. This phenomenon might be attributed to the different matrices of both powders. The foam-mat powder was prepared by using SSL as a foaming agent and maltodextrin as a filler and stabilizer. The water-soluble constituents from roselle calyces could be entrapped in the matrix of maltodextrin, thus resulting in a different reactivity to the altered pH from conventionally dried powder. Millinia et al. [39] showed that different matrices of microcapsules of roselle anthocyanin extracts produced different colors when dissolved at different acidic pHs. In roselle calyces, anthocyanins are synthesized in the cytoplasm and stored in vacuoles. Anthocyanins could be released after breaking the cell walls [40], indicating that anthocyanin release is not easy. This fact indicates that the matrix of both powders may be different, which might affect the stability of anthocyanins to different pHs, thus producing a slightly different color.
Color quality, indicated by color intensity, at different pHs ranged from 0.03 to 15.24, and the non-adjusted pH solution had a color intensity of 1.37–33.48, revealing that pH decreases color quality. High color intensity (CI) of anthocyanins is desired because it reflects color density. Figure 4 shows that the foam-mat dried powder had a higher CI than its corresponding conventionally dried powder (p < 0.05) for both red and purple calyces along the tested pHs. The foam-mat powder matrix possibly contributed to making the anthocyanin more stable to pH changes. The red calyx powder had lower CI than the purple for both conventionally and foam-mat dried powders. The purple roselle calyx powder had higher color density and thus presented better CI. The changes in pH also altered CI on roselle calyx powder solutions. Both red roselle powders exhibited similar patterns, in which the CI decreased gradually with increasing pH. Also, a similar pattern was shown by both purple roselle calyx powders. The CI at pH 3 was the highest; at this pH, anthocyanins were deep purple for conventionally dried powder but deep magenta for foam-mat dried, indicating the presence of delphinidin, petunidin (purple color), and cyanidin (magenta color). Increasing pH to 7 decreased the CI, which then increased at pH 11 and 12 for foam-mat and conventionally dried powder, respectively. The foam-mat dried powder exhibited a deep purple color at pH 11; meanwhile, the conventionally dried powder showed a similar color at pH 12. Theoretically, the color of anthocyanins at high basic pH is violet/blue [38].
Degradation of anthocyanins is indicated by the browning index (BI) [32], obtained by measuring A at 520 nm (red color) and 420 nm (brown color). All powders had almost unchanged BI until pH 8 (p < 0.05). Further increases in pH sharply increased the BI for both red roselle calyx powders; this increase was smaller for both purple roselle calyx powders. According to Dorris et al. [32], BI is very strongly pH-dependent. The lower anthocyanin in red calyces resulted in very obvious browning at very basic pHs. The degradation of anthocyanin resulted in chalcone formation, which was further degraded to brown products during heat treatment. Condensation reactions accompanied anthocyanin degradation at pH 3.0; meanwhile, at higher pH, degradation was the main reaction [41]. Anthocyanins are unstable at basic pHs and tend to decompose into dark-brown oxidized compounds [16]. The increasing yellowness (p < 0.05) (Figure 3) was an indicator of browning formation at increased pH (>4). The yellowness of red roselle calyx powder was higher than that of purple roselle, resulting in higher BI. Purple roselle calyx powders were more stable than the red. Interestingly, foam-mat dried powder had lower BI than the conventionally dried, indicating a protective effect of the foam-mat matrix.
The violet index (VI) is an indicator of the color quality of anthocyanins that measures the ratio of purple color intensity (A = 580 nm) to red color intensity (A = 520 nm) [31]. Figure 4 shows that VI tended to increase with increased pH values (p < 0.05), in accordance with the decrease in redness (Figure 3). The purple calyx powders had higher VI, indicating better color quality than the red ones along the evaluated pHs. The foam-mat dried roselle calyx powder revealed higher VI than those of conventionally dried, both for red and purple calyces, indicating a protective role of the powder matrix. In conclusion, foam-mat dried roselle calyx powders, both purple and red, had a better color quality at different pHs, indicated by higher CI and VI but lower BI. The matrix of this powder seemed to protect the anthocyanins from degradation due to pH changes. The matrix in a conventionally dried powder consists of protein, fat, and carbohydrates, including crude fiber and water-soluble polysaccharides, whose stability might also be affected by pH.
Total phenolic compounds (TPC) of roselle calyx powder solutions tended to decrease with increased pHs (p < 0.05) (Figure 5). Anthocyanins are the main TPC in roselle calyces (Table 1). Degradation of anthocyanins into brown compounds at basic pHs was likely to occur, as indicated by the increased BI, thus decreasing the TPC. Its retention, compared to the untreated pH solution, also decreased. Pasquet et al. [42] reported that phenolic compounds were stable at acidic pHs but decreased sharply in alkaline pHs. Several studies reported that polyphenols were degraded, dimerized, and oxidized in alkaline solutions, decreasing the total phenolic content [43,44]. In addition, the pH level had a significant impact on the overall total phenolic content degradation. Increased pH caused the total phenolic content to decrease, which is in line with the results by Chen et al. [45].
The TPC of conventionally dried calyx powders was higher than in foam-mat dried roselle powders, and the TPC of purple roselle was higher than red roselle. Conventionally dried and purple calyx powders had higher TPC (Table 1). The retention of phenolics and flavonoids was 8.02%–99.69% and 0.00%–81.05%, respectively, indicating the instability of bioactive compounds. However, the retention of TPC was higher in red than purple calyx powders along the evaluated pHs. A different pattern was observed between foam-mat and conventionally dried red calyx powders. Based on the TPC retention data, conventionally dried red roselle calyx powders preserved TPC better than their corresponding foam-mat dried powders. Meanwhile, an inverse phenomenon was found in purple roselle calyx powders. There was a much higher TPC content in conventionally dried purple calyx powders than in foam-dried powders (Table 1). Abundant phenolic compounds might lead to polymerization at alkaline pHs, thus decreasing TPC and its retention. According to Pasquet et al. [42], phenolic compound transformation to quinones is promoted at high pHs. Quinones are reactive species susceptible to polymerization.
Total anthocyanin content (TAC) decreased with increasing pH (p < 0.05). All samples revealed a sharp decrease until pH 7; further increases of alkaline pH seemed to slowly decrease the TAC. Anthocyanins will degrade according to their substituent groups at a pH higher than 7 [14,46]. Degradation of anthocyanin leads to anthocyanin polymerization [41]. According to Marpaung & Tjahjadi [31], the polymeric anthocyanin was resistant to pH changes in TAC measurements both at pH 1 and 4.5. The TAC measurement was based on the differences in absorbance at pH 1 and 4.5. Anthocyanin presented as red flavylium cation species (AH+) at pH 1, and at pH 4.5 they converted to colorless hemiketal. Hence, absorbance differences indicate the anthocyanin concentration.
The degree of anthocyanin degradation is highly species-dependent. Cabrita et al. [47] showed that in some anthocyanin-3-glucosides, such as malvidin-3-glucosides, the bluish color intensity was relatively stable at the alkaline pH region. Malvidin-3-glucosides exhibits a purple color, which is supposed to be found in purple roselle calyces. Therefore, the TAC of the conventionally dried purple calyx powders was the highest (Figure 5). The main anthocyanins in red roselle calyces are cyanidin 3-O-sambubioside and delphinidin 3-O-sambubioside [36]. Rakic & Ulrih [48] reported that the stability of cyanidin was highly affected by pH values, even being unstable at alkaline pHs. Sui et al. [49] indicated that increasing pH from 5.0 to 6.0 resulted in a significant increase in the degradation rate constants of two species of cyanidins. As such, the TAC of red roselle calyx powders was lower than that of purple roselle. The retention of anthocyanin decreased sharply until pH 7, and further increases in pH only slightly decreased the anthocyanin retention. This means that changes in anthocyanins were very low at alkaline pHs. Foam-mat dried powders exhibited better TAC retention than conventionally dried powders, indicating good protection of the powder matrix. Maltodextrin had a higher interaction affinity with anthocyanins due to its chemical structure [50]. The solubilization at different pH values might still preserve this interaction to some extent.
Anthocyanins are described as antioxidant compounds as they prevent or inhibit oxidation by scavenging free radicals and reducing oxidative radicals [51]. Roselle calyx powder solutions at various pH still exhibited DPPH and ABTS radical scavenging activity (Figure 6). The ability to scavenge ABTS radicals was higher than that of DPPH for all samples. Floegel et al. [52] indicated that the antioxidant activity measurement by ABTS assay was significantly higher than by DPPH assay for vegetables, fruits, and beverages. Hydrophilic and high-pigmented antioxidants were better assayed by ABTS than DPPH methods.
Roselle antioxidant activity was still observed at different pHs, ranging from 0.00 to 1.11 mg TE/g for the DPPH scavenging activity and 0.46–104.16 mg TE/g for the ABTS assay. In general, increasing the pH of solutions tended to decrease DPPH radical scavenging activity, except for conventionally dried purple roselle calyx powders, which clearly showed an increase in antioxidant activity until pH 6, followed by a decrease with further increases in pHs (p < 0.05). This sample also exhibited the lowest DPPH radical scavenging activity, expressed in Trolox equivalent. The ABTS radical scavenging activity of solutions exhibited different patterns than the DPPH, which tended to increase until pH 6, decrease until pH 11, and then increase again at pH higher than 11. Meanwhile, the antioxidant activity expressed as Trolox equivalent only showed slight changes along the evaluated pH values, except for the conventionally dried purple roselle calyx powders, which showed a distinctive pattern (p < 0.05). The antioxidant activity of this sample increased with increasing pH to 6, tended to be stable until pH 9, then decreased and sharply increased at pH 13.
The instability of the phenolic compounds at alkaline pHs may be responsible for the decreasing antioxidant activity of roselle calyx powders at different pH levels assayed by four assays (ABTS•+ scavenging, Fe3+-reducing activity, phosphomolybdate method, and H2O2 scavenging) [53]. Sun et al. [54] also found that the antioxidant activity of sweet potato leaf polyphenols, assayed by DPPH, OH radicals, and oxygen radical methods was stable at pH 5–7 but decreased as the pH became more alkaline. Similarly, Kungsuwan et al. [55] reported that the decreasing antioxidant activity of Clitorea ternatea in alkaline conditions, assayed by the DPPH method, was due to the properties of anthocyanins, which are more stable in acidic conditions than in neutral and alkaline pHs.
The molecular structural changes of anthocyanin and phenolic compounds at different pHs affected the antioxidant activity. At acidic pHs, anthocyanin presents as a flavylium cation structure (AH+); this molecule acts as an acid and directly relates to its antioxidant activity [51]. Thus, at acidic pHs, the antioxidant activity was high and decreased with increasing pH. No detection of antioxidant activity of conventionally dried purple roselle calyces at pH 2 was related to the bias of solution color due to the deep purple at this pH value. Sui et al. [49] also indicated the lowest antioxidant activity of anthocyanin solutions at pH 2.2. Some samples preserved high DPPH scavenging activity at acidic pHs and in this pH region, the ABTS scavenging activity increased (Figure 6). The antioxidant activity was related to phenolic compounds including anthocyanins. According to Yu et al. [56], the phenolic compounds were unstable at pH 7 but had the strongest DPPH radical scavenging capacity; meanwhile, acidic pHs seemed to maintain antioxidant activity better than alkaline conditions. Phenolic compounds were more stable in acidic than alkaline conditions, and this stability prevented their degradation and oxidation [15].
The structural changes of anthocyanins were cation flavylium at pH < 3, carbinol pseudo-bases at pH 4–5, quinoidal bases at pH 6–7, and chalcone at pH > 6 [57]. Quinoidal bases and chalcones of anthocyanins had a double bond conjugated to the keto group and acted as antioxidants for scavenging free radicals [51]. Therefore, the antioxidant activity was preserved at high pHs, although the activity decreased. A sudden increase in ABTS radical scavenging activity at pH 12–13 might be related to the abundance of chalcone. Kudlickova et al. [58] reported higher ABTS radical scavenging activity than DPPH of chalcones. Therefore, in this study, the DPPH radical scavenging activity was not observed at a very high pH value. Foam-mat dried roselle calyx powders had better antioxidant activity both for ABTS and DPPH radical scavenging activity in the range of evaluated pHs, indicating this powder can preserve antioxidant compound stability during pH treatments.
The total phenolic content and total anthocyanin content of the conventionally dried roselle calyx solutions were higher than foam-mat dried roselle calyces (p < 0.05). The preparation of foam-mat calyx powders involves water extraction while the conventionally dried powder is prepared from the whole calyces without extraction. Thereby, the bioactive content of the foam-mat dried powder is lower than that of the conventionally dried powder [13]. However, the retention of phenolic compounds and anthocyanins is better in foam-mat dried powders, indicating better protection of the powder matrix to changes in pH, resulting in better antioxidant activity of the foam-mat powder.
The foam-mat dried roselle calyx powders, both purple and red, had better color quality at different pHs, indicating higher CI and VI but lower BI. Higher CI and VI and lower BI indicate better protection of the foam-mat matrices during drying and thus better retention of phenolic compounds and anthocyanins, resulting in higher antioxidant activity of foam-mat dried powders.
This study evaluates the color stability, bioactive compounds, and antioxidant activity of roselle calyx powders dissolved in different pHs. This study showed that acidic pHs better preserve roselle color, bioactive compounds, and antioxidant activity. In practice, the pH value should be considered when formulating roselle calyx powders into different food products. This study used a solution as a model for a watery system of foods. Suitable products to use roselle calyx powders are water-based products with acidic pHs such as syrups, yogurts and yogurt-based products, fermented milk, and sour-taste beverages. Roselle calyx powders are suitable to use as coloring agents or for bioactive compound enrichment to provide healthy functional properties. The use of foam-mat dried powder is suggested due to better antioxidant activity and color preservation than conventionally dried powder.
The color change pattern among different roselle powders is quite different at various pHs. The changes in color and color quality at different pH were related to the structural changes of anthocyanin molecules. Foam-mat dried roselle calyx powders, both red and purple, presented lighter colors but different appearances at similar pHs. The foam-mat dried powder was more stable at several pHs than the conventionally dried powder. Protective effects were observed on foam-mat dried powder, indicated by better anthocyanin and phenolic retention and color quality. pH-induced structural changes in anthocyanins affected the antioxidant activity. The ABTS radical scavenging activity was higher than that of DPPH, indicating that the ABTS method was more suitable for roselle calyces. The pattern of changes in antioxidant activity of both assays was also different. The phenolic compounds may also contribute to stability at different pH values since these compounds are also pH-sensitive. Using foam-mat dried roselle calyces for food formulations is recommended; also, purple roselle calyces revealed a better performance than red roselle. pH changes during processing and storage should be a main concern when using roselle calyx powders in food formulation. This study evaluated the stability of roselle calyx powders in solution with different pH values, but these did not fully represent the food model systems. Further work is necessary to study food models with different matrices and processing techniques. Based on the findings of this study, the best pH for conventional and foam-mat dried roselle calyces formulation is an acidic pH, which seems to preserve antioxidant activity, color, and bioactive compounds. Suitable food products for roselle calyx powder formulations are watery high-acid foods such as fruit syrups, yogurt-based drinks, candy, and other sour-taste food products.
The authors declare they have not used Artificial Intelligence (AI) tools in the creation of this article.
All authors would like to thank Universitas Brawijaya, Universitas Gadjah Mada, and Universitas Pendidikan Indonesia for funding this research through Riset Kolaborasi Indonesia Year 2024 and Directorate of Research, Technology, and Community Service, the Ministry of Education, Culture, Research, and Technology, the Republic of Indonesia for facilitating Indonesian Collaborative Research project with Grant Contract No. 1146.4/UN10.Cf10/TU/2024.
The authors declare that there are no financial, professional, or personal conflicts of interest.
Teti Estiasih: Research design and conceptualization, research coordination, research supervision, troubleshooting during experimental research, data analysis and interpretation, manuscript preparation, original manuscript writing, and revision. Jatmiko Eko Witoyo: Manuscript preparation, data analysis, troubleshooting during experimental research, data interpretation, and drafting original article. Khofifah Putri Wulandari and Fadhilah Dwi Juniati: Conducted experimental design and data analysis. Widiastuti Setyaningsih, Hanifah Nuryani Lioe, Miguel Palma, and Hamidie Ronald Daniel Ray: Research design and conceptualization. Kgs Ahmadi: Visualization, statistical analysis.
[1] | El Bayani GF, Marpaung NLE, Simorangkir DAS, et al. (2018) Anti-inflammatory effects of Hibiscus sabdariffa Linn. on the IL-1β/IL-1ra ratio in plasma and hippocampus of overtrained rats and correlation with spatial memory. Kobe J Med Sci 64: E73–E83. |
[2] |
Izquierdo-Vega JA, Arteaga-Badillo DA, Sánchez-Gutiérrez M, et al.(2020) Organic acids from Roselle (Hibiscus sabdariffa L.)-A brief review of its pharmacological effects. Biomedicines 8: 1–16. https://doi.org/10.3390/biomedicines8050100 doi: 10.3390/biomedicines8050100
![]() |
[3] |
Sapian S, Ibrahim Mze AA, Jubaidi FF, et al. (2023) Therapeutic Potential of Hibiscus sabdariffa Linn. in attenuating cardiovascular risk factors. Pharmaceuticals 16: 1–25. https://doi.org/10.3390/ph16060807 doi: 10.3390/ph16060807
![]() |
[4] | Islam A, Jamini TS, Islam M, et al. (2016) Roselle: A functional food with high nutritional and medicinal values. Fundam Appl Agric 1: 44–49. |
[5] |
Tan SL, Sulaiman R (2020) Color and rehydration characteristics of natural red colorant of foam-mat dried Hibiscus sabdariffa L. powder. Int J Fruit Sci 20: 89–105. https://doi.org/10.1080/15538362.2019.1605557 doi: 10.1080/15538362.2019.1605557
![]() |
[6] |
Hamrouni-Sellami I, Rahali FZ, Rebey IB, et al. (2013) Total phenolics, flavonoids, and antioxidant activity of sage (Salvia officinalis L.) plants as affected by different drying methods. Food Bioprocess Technol 6: 806–817. https://doi.org/10.1007/s11947-012-0877-7 doi: 10.1007/s11947-012-0877-7
![]() |
[7] |
Roshanak S, Rahimmalek M, Goli SAH (2016) Evaluation of seven different drying treatments in respect to total flavonoid, phenolic, vitamin C content, chlorophyll, antioxidant activity and color of green tea (Camellia sinensis or C. assamica) leaves. J Food Sci Technol 53: 721–729. https://doi.org/10.1007/s13197-015-2030-x doi: 10.1007/s13197-015-2030-x
![]() |
[8] |
Tajudin NHA, Tasirin SM, Ang WL, et al. (2019) Comparison of drying kinetics and product quality from convective heat pump and solar drying of Roselle calyx. Food Bioprod Process 118: 40–49. https://doi.org/10.1016/j.fbp.2019.08.012 doi: 10.1016/j.fbp.2019.08.012
![]() |
[9] |
Djaeni M, Kumoro AC, Sasongko SB, et al. (2018) Drying rate and product quality evaluation of roselle (Hibiscus sabdariffa L.) calyces extract dried with foaming agent under different temperatures. Int J Food Sci 2018: 9243549. https://doi.org/10.1155/2018/9243549 doi: 10.1155/2018/9243549
![]() |
[10] |
Liu Y, Liu Y, Tao C, et al. (2018) Effect of temperature and pH on stability of anthocyanin obtained from blueberry. J Food Meas Charact 12: 1744–1753. https://doi.org/10.1007/s11694-018-9789-1 doi: 10.1007/s11694-018-9789-1
![]() |
[11] |
Sasongko SB, Djaeni M, Utari FD (2019) Kinetic of anthocyanin degradation in roselle extract dried with foaming agent at different temperatures. Bull Chem React Eng Catal 14: 320–325. https://doi.org/10.9767/bcrec.14.2.2875.320-325 doi: 10.9767/bcrec.14.2.2875.320-325
![]() |
[12] |
Wu HY, Yang KM, Chiang PY (2018) Roselle anthocyanins: antioxidant properties and stability to heat and pH. Molecules 23: 1357. https://doi.org/10.3390/molecules23061357 doi: 10.3390/molecules23061357
![]() |
[13] |
Estiasih T, Witoyo JE, Runtung GKM, et al. (2024) The characteristics of foam-mat dried red and purple roselle calyces powder prepared with commercial emulsifiers as the foaming agents. CYTA-J Food 22: 2356847. https://doi.org/10.1080/19476337.2024.2356847 doi: 10.1080/19476337.2024.2356847
![]() |
[14] |
Enaru B, Drețcanu G, Pop TD, et al. (2021) Anthocyanins: Factors affecting their stability and degradation. Antioxidants 10: 1967. https://doi.org/10.3390/antiox10121967 doi: 10.3390/antiox10121967
![]() |
[15] |
Friedman M, Jürgens HS (2000) Effect of pH on the stability of plant phenolic compounds. J Agric Food Chem 48: 2101–2110. https://doi.org/10.1021/jf990489j doi: 10.1021/jf990489j
![]() |
[16] |
Mattioli R, Francioso A, Mosca L, et al. (2020) Anthocyanins: A comprehensive review of their chemical properties and health effects on cardiovascular and neurodegenerative diseases. Molecules 25: 3809. https://doi.org/10.3390/molecules25173809. doi: 10.3390/molecules25173809
![]() |
[17] |
Maisto M, Marzocchi A, Ciampaglia R, et al. (2024) Effects of food-derived antioxidant compounds on in vitro heavy metal intestinal bioaccessibility. Antioxidants 13: 610. https://doi.org/10.3390/antiox13050610 doi: 10.3390/antiox13050610
![]() |
[18] |
Shruthi VH, Ramachandra CT, Nidoni U, et al. (2017). Physico-chemical, nutritional and functional properties of roselle (Hibiscus sabdariffa L.). Int J Curr Microbiol Appl Sci 6: 1–7. https://doi.org/10.20546/ijcmas.2017.612.347 doi: 10.20546/ijcmas.2017.612.347
![]() |
[19] |
Rocha IDC, Bonnlaender B, Sievers H, et al. (2014). Hibiscus sabdariffa L.—A phytochemical and pharmacological review. Food Chem 165: 424–443. https://doi.org/10.1016/j.foodchem.2014.05.002. doi: 10.1016/j.foodchem.2014.05.002
![]() |
[20] |
Marpaung AM, Paramaputri A (2023) UV-visible light spectra of Clitoria ternatea L. flower extract during aqueous extraction and storage. Int Food Res J. 30: 764–773. https://doi.org/10.47836/ifrj.30.3.18 doi: 10.47836/ifrj.30.3.18
![]() |
[21] |
Samadi S, Fard FR (2020) Phytochemical properties, antioxidant activity and mineral content (Fe, Zn and Cu) in Iranian produced black tea, green tea and roselle calyces. Biocatal Agric Biotechnol 23: 101472. https://doi.org/10.1016/j.bcab.2019.101472 doi: 10.1016/j.bcab.2019.101472
![]() |
[22] |
Huang J, Hu Z, Chin Y, et al. (2023) Improved thermal stability of roselle anthocyanin by co-pigmented with oxalic acid: preparation, characterization and enhancement mechanism. Food Chem 410: 135407. https://doi.org/10.1016/j.foodchem.2023.135407 doi: 10.1016/j.foodchem.2023.135407
![]() |
[23] |
Ifie I, Marshall LJ, Ho P, et al. (2016) Hibiscus sabdariffa (Roselle) extracts and wine: phytochemical profile, physicochemical properties, and carbohydrase inhibition. J Agric Food Chem 64: 4921–4931. https://doi.org/10.1021/acs.jafc.6b01246. doi: 10.1021/acs.jafc.6b01246
![]() |
[24] |
Baliyan S, Mukherjee R, Priyadarshini A, et al. (2022) Determination of antioxidants by DPPH radical scavenging activity and quantitative phytochemical analysis of Ficus religiosa. Molecules 27: 1326. https://doi.org/10.3390/molecules27041326 doi: 10.3390/molecules27041326
![]() |
[25] |
Thaipong K, Boonprakob U, Crosby K, et al. (2006) Comparison of ABTS, DPPH, FRAP, and ORAC Assays for estimating antioxidant activity from guava fruit extracts. J Food Anal 19: 669–675. https://doi.org/10.1016/j.jfca.2006.01.003 doi: 10.1016/j.jfca.2006.01.003
![]() |
[26] | Shruthi VH, Ramachandra CT (2019) Roselle (Hibiscus sabdariffa L.) calyces: A potential source of natural colour and its health benefits. In: Deka SC, Seth D, Hulle VRS (Eds.), Food bioactive: Functionalities and applications in human health, New York: Apple Academic Press, 169–190. https://doi.org/10.1201/9780429242793 |
[27] |
Song W, Yuan Q, Xie Y, et al. (2024) Formulation and characterization of nanocapsules loaded with roselle anthocyanins extract and enhancement of anthocyanins bioaccessibility. Food Chem 459: 140446. https://doi.org/10.1016/j.foodchem.2024.140446 doi: 10.1016/j.foodchem.2024.140446
![]() |
[28] |
Yang D, Xu HX, Guo YX, et al. (2023) Chemical profile of roselle extract and its inhibitory activities on three digestive enzymes in vitro and in vivo. Int J Biol Macromol 253: 126902. https://doi.org/10.1016/j.ijbiomac.2023.126902 doi: 10.1016/j.ijbiomac.2023.126902
![]() |
[29] |
Khoo HE, Azlan A, Tang ST, et al. (2017) Anthocyanidins and anthocyanins: Colored pigments as food, pharmaceutical ingredients, and the potential health benefits. Food Nutr Res 61: 1361779. https://doi.org/10.1080/16546628.2017.1361779 doi: 10.1080/16546628.2017.1361779
![]() |
[30] | Aishah B, Nursabrina M, Noriham A, et al. (2013), Anthocyanins from Hibiscus sabdariffa, Melastoma malabathricum and Ipomoea batatas and its color properties. Int Food Res J 20: 827–834. |
[31] | Marpaung A, Tjahjadi K (2019) The analysis of monomeric anthocyanin by pH differential method is not appropriate for certain anthocyanins. In: Proceedings of the 16th ASEAN Food Conference (16th AFC 2019)—Outlook and Opportunities of Food Technology and Culinary for Tourism Industry, 26–30. https://doi.org/10.5220/0009985400002964 |
[32] |
Dorris MR, Voss DM, Bollom MA, et al. (2018) Browning index of anthocyanin‐rich fruit juice depends on pH and anthocyanin loss more than the gain of soluble polymeric pigments. J Food Sci 83: 911–921. https://doi.org/10.1111/1750-3841.14106 doi: 10.1111/1750-3841.14106
![]() |
[33] |
Gao Q, Li Y, Li Y, et al. (2022) Antioxidant and prooxidant activities of phenolic acids commonly existed in vegetables and their relationship with structures. Food Sci Technol 42: e07622. https://doi.org/10.1590/fst.07622 doi: 10.1590/fst.07622
![]() |
[34] |
Nowak M, Tryniszewski W, Sarniak A, et al. (2022) Concentration dependence of anti-and pro-oxidant activity of polyphenols as evaluated with a light-emitting Fe2+-Egta-H2O2 System. Molecules 27: 3453. https://doi.org/10.3390/molecules27113453 doi: 10.3390/molecules27113453
![]() |
[35] |
Rajashekar CB (2023) Dual role of plant phenolic compounds as antioxidants and prooxidants. Am J Plant Sci 14: 15–28. https://doi.org/10.4236/ajps.2023.141002 doi: 10.4236/ajps.2023.141002
![]() |
[36] |
Wu HY, Yang KM, Chiang PY (2018) Roselle anthocyanins: antioxidant properties and stability to heat and pH. Molecules 23: 1357. https://doi.org/10.3390/molecules23061357 doi: 10.3390/molecules23061357
![]() |
[37] |
Horbowicz M, Kosson R, Grzesiuk A, et al. (2008). Anthocyanins of fruits and vegetables - their occurrence, analysis and role in human nutrition. Veg Crops Res Bull 68: 5–22. https://doi.org/10.2478/v10032-008-0001-8 doi: 10.2478/v10032-008-0001-8
![]() |
[38] |
Punyachareonnon, Deerattrakul V, Luepong K (2021) The Influence of pH, temperature and time on dyeing of silk fabric by black bean anthocyanin-rich extract as colorant. Prog Color Color Coatings 14: 179–186. https://doi.org/10.30509/pccc.2021.81715 doi: 10.30509/pccc.2021.81715
![]() |
[39] |
Millinia BL, Mashithah D, Nawatila R, et al. (2024) Microencapsulation of roselle (Hibiscus sabdariffa L.) anthocyanins: Effects of maltodextrin and trehalose matrix on selected physicochemical properties and antioxidant activities of spray-dried powder. Future Foods 9: 100300. https://doi.org/10.1016/j.fufo.2024.100300 doi: 10.1016/j.fufo.2024.100300
![]() |
[40] |
Idham Z, Putra NR, Aziz AHA, et al. (2022) Improvement of extraction and stability of anthocyanins, the natural red pigment from roselle calyces using supercritical carbon dioxide extraction. J CO2 Util 56: 101839. https://doi.org/10.1016/j.jcou.2021.101839 doi: 10.1016/j.jcou.2021.101839
![]() |
[41] |
Jiang T, Mao Y, Sui L, et al. (2019) Degradation of anthocyanins and polymeric color formation during heat treatment of purple sweet potato extract at different pH. Food Chem 274: 460–470. https://doi.org/10.1016/j.foodchem.2018.07.141 doi: 10.1016/j.foodchem.2018.07.141
![]() |
[42] |
Pasquet PL, Julien-David D, Zhao M, et al. (2024) Stability and preservation of phenolic compounds and related antioxidant activity from agro-food matrix: effect of pH and atmosphere. Food Biosci 57: 103586. https://doi.org/10.1016/j.fbio.2024.103586 doi: 10.1016/j.fbio.2024.103586
![]() |
[43] |
Wang Y, Xing L, Zhang J, et al. (2023) Determination of endogenous phenolic compounds in honey by HPLC-MS/MS. LWT 183: 114951. https://doi.org/10.1016/j.lwt.2023.114951 doi: 10.1016/j.lwt.2023.114951
![]() |
[44] | Krungkri W, Areekul V (2019) Effect of heating condition and pH on stability of total phenolic content and antioxidant activities of samui (Micromelum minutum) extract. In: Proceedings of the 16th ASEAN Food Conference (16th AFC 2019)—Outlook and Opportunities of Food Technology and Culinary for Tourism Industry. Bali, Indonesia, 15–32. https://doi.org/10.5220/0009980801260132 |
[45] |
Chen YT, Kao WT, Lin KW (2008) Effects of pH on the total phenolic compound, antioxidative ability and the stability of dioscorin of various yam cultivars. Food Chem 107: 250–257. https://doi.org/10.1016/j.foodchem.2007.08.017 doi: 10.1016/j.foodchem.2007.08.017
![]() |
[46] |
Rosales-Murillo SS, Sánchez-Bodón J, Hernández Olmos SL, et al. (2024) Anthocyanin-loaded polymers as promising nature-based, responsive, and bioactive materials. Polymers 16: 163. https://doi.org/10.3390/polym16010163 doi: 10.3390/polym16010163
![]() |
[47] |
Cabrita L, Fossen T, Andersen ØM (2000) Colour and stability of the six common anthocyanidin 3-glucosides in aqueous solutions. Food Chem 68: 101–107. https://doi.org/10.1016/S0308-8146(99)00170-3 doi: 10.1016/S0308-8146(99)00170-3
![]() |
[48] |
Rakić V, Ulrih NP (2021) Influence of pH on color variation and stability of cyanidin and cyanidin 3-O-β-glucopyranoside in aqueous solution. CyTA-J Food 19: 174–182. https://doi.org/10.1080/19476337.2021.1874539 doi: 10.1080/19476337.2021.1874539
![]() |
[49] |
Sui X, Dong X, Zhou W (2014) Combined effect of pH and high temperature on the stability and antioxidant activity of two anthocyanins in aqueous solution. Food Chem 163: 163–170. https://doi.org/10.1016/j.foodchem.2014.04.075 doi: 10.1016/j.foodchem.2014.04.075
![]() |
[50] |
Rigolon TCB, Silva RRA, de Oliveira TV, et al. (2024) Exploring anthocyanins-polysaccharide synergies in microcapsule wall materials via spray drying: interaction characterization and evaluation of particle stability. Meas Food 13: 100126. https://doi.org/10.1016/j.meafoo.2023.100126 doi: 10.1016/j.meafoo.2023.100126
![]() |
[51] |
Tena N, Martín J, Asuero AG (2020) State of the art of anthocyanins: antioxidant activity, sources, bioavailability, and therapeutic effect in human health. Antioxidants 9: 451. https://doi.org/10.3390/antiox9050451 doi: 10.3390/antiox9050451
![]() |
[52] |
Floegel A, Kim DO, Chung SJ, et al. (2011) Comparison of ABTS/DPPH assays to measure antioxidant activity in popular antioxidant-rich US foods. J Food Compos Anal 24: 1043–1048. https://doi.org/10.1016/j.jfca.2011.01.008 doi: 10.1016/j.jfca.2011.01.008
![]() |
[53] |
Bayliak MM, Burdyliuk NI, Lushchak VI (2016) Effects of pH on antioxidant and prooxidant properties of common medicinal herbs. Open Life Sci 11: 298–307. https://doi.org/10.1515/biol-2016-0040 doi: 10.1515/biol-2016-0040
![]() |
[54] |
Sun HN, Mu TH, Xi LS (2017) Effect of pH, heat, and light treatments on the antioxidant activity of sweet potato leaf polyphenols. Int J Food Prop 20: 318–332. https://doi.org/10.1080/10942912.2016.1160410 doi: 10.1080/10942912.2016.1160410
![]() |
[55] |
Kungsuwan K, Singh K, Phetkao S, et al. (2014) Effect of pH and anthocycnin concentration on colour and antioxidant activity of Clitorea ternatea extract. Food Appl Biosci J 2: 31–46. https://doi.org/10.14456/fabj.2014.3 doi: 10.14456/fabj.2014.3
![]() |
[56] |
Yu L, Wu Y, Liu D, et al. (2022) The kinetic behavior of antioxidant activity and the stability of aqueous and organic polyphenol extracts from navel orange peel. Food Sci Technol 42 :e90621. https://doi.org/10.1590/fst.90621 doi: 10.1590/fst.90621
![]() |
[57] |
Kamiloglu S, Capanoglu E, Grootaert C, et al. (2015) Anthocyanin absorption and metabolism by human intestinal Caco2-cells—A review. Int J Mol Sci 16: 21555–21574. https://doi.org/10.3390/ijms160921555 doi: 10.3390/ijms160921555
![]() |
[58] |
Kudličková Z, Michalková R, Salayová A, et al. (2023) Design, synthesis, and evaluation of novel indole hybrid chalcones and their antiproliferative and antioxidant activity. Molecules 28: 6583. https://doi.org/10.3390/molecules2818658 doi: 10.3390/molecules2818658
![]() |
Parameter | Conventionally dried | Foam-mat dried | ||
Red | Purple | Red | Purple | |
Color | ||||
Lightness (L) | 47.45 ± 0.01b | 33.80 ± 0.00d | 49.05 ± 0.35a | 37.88 ± 2.86c |
Redness (+a) | 19.15 ± 0.00d | 34.38 ± 0.32c | 39.95 ± 0.49b | 42.45 ± 3.04a |
Yellowness (+b) | 7.10 ± 0.00d | 15.68 ± 0.11b | 12.25 ± 0.35c | 18.35 ± 0.78a |
Color quality | ||||
Color intensity (CI) | 8.36 ± 0.01b | 33.48 ± 0.81a | 1.37 ± 0.04d | 6.74 ± 0.14c |
Violet index (VI) | 0.15 ± 0.00b | 0.14 ± 0.01c | 0.18 ± 0.00a | 0.14 ± 0.00c |
Browning index (BI) | 0.39 ± 0.00b | 0.38 ± 0.02c | 0.58 ± 0.00a | 0.35 ± 0.02d |
Total phenolic content (mg GAE/mL) | 6.52 ± 0.03b | 23.07 ± 0.02a | 2.47 ± 0.03d | 5.37 ± 0.02c |
Total anthocyanin content (mg/mL) | 2.34 ± 0.08c | 12.03 ± 0.02a | 0.57 ± 0.02d | 3.78 ± 0.00b |
Antioxidant activity | ||||
DPPH assay | ||||
Trolox equivalent (mg TE/g) | 0.86 ± 0.02a | n.d. | 0.85 ± 0.00b | 0.68 ± 0.00c |
ABTS assay | ||||
Trolox equivalent (mg TE/g) | n.d. | 3.91 ± 0.02b | n.d. | 11.94 ± 0.14a |
Note: n.d. = not detected. Different letters indicate statistical differences for the data in the same row. |
Parameter | Conventionally dried | Foam-mat dried | ||
Red | Purple | Red | Purple | |
Color | ||||
Lightness (L) | 47.45 ± 0.01b | 33.80 ± 0.00d | 49.05 ± 0.35a | 37.88 ± 2.86c |
Redness (+a) | 19.15 ± 0.00d | 34.38 ± 0.32c | 39.95 ± 0.49b | 42.45 ± 3.04a |
Yellowness (+b) | 7.10 ± 0.00d | 15.68 ± 0.11b | 12.25 ± 0.35c | 18.35 ± 0.78a |
Color quality | ||||
Color intensity (CI) | 8.36 ± 0.01b | 33.48 ± 0.81a | 1.37 ± 0.04d | 6.74 ± 0.14c |
Violet index (VI) | 0.15 ± 0.00b | 0.14 ± 0.01c | 0.18 ± 0.00a | 0.14 ± 0.00c |
Browning index (BI) | 0.39 ± 0.00b | 0.38 ± 0.02c | 0.58 ± 0.00a | 0.35 ± 0.02d |
Total phenolic content (mg GAE/mL) | 6.52 ± 0.03b | 23.07 ± 0.02a | 2.47 ± 0.03d | 5.37 ± 0.02c |
Total anthocyanin content (mg/mL) | 2.34 ± 0.08c | 12.03 ± 0.02a | 0.57 ± 0.02d | 3.78 ± 0.00b |
Antioxidant activity | ||||
DPPH assay | ||||
Trolox equivalent (mg TE/g) | 0.86 ± 0.02a | n.d. | 0.85 ± 0.00b | 0.68 ± 0.00c |
ABTS assay | ||||
Trolox equivalent (mg TE/g) | n.d. | 3.91 ± 0.02b | n.d. | 11.94 ± 0.14a |
Note: n.d. = not detected. Different letters indicate statistical differences for the data in the same row. |