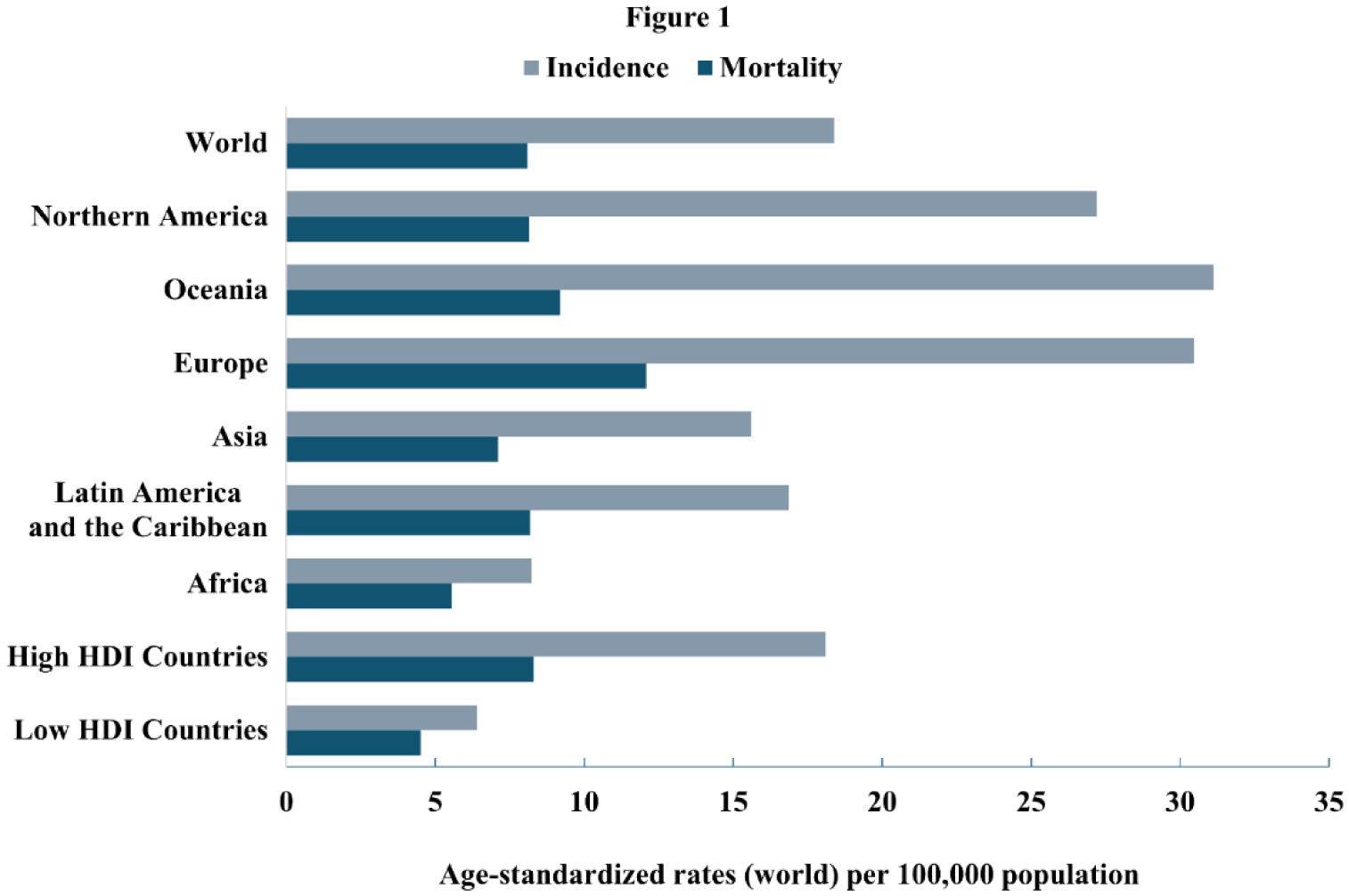
Colorectal cancer (CRC), a cancer of the large intestine and rectum, has one of the highest incidence and mortality rates, with incidences expected to nearly double in the next 25 years. The development of CRC may be caused by a collection of mutations via the chromosomal instability pathway, which results in approximately 70% of sporadic CRC cases, or the microsatellite instability pathway. At the same time, there are various modifiable and non-modifiable risk factors that increase one's chances of developing a CRC causing mutation via these pathways. There are three widely used treatments for CRC: surgical resection, chemotherapy, and immunotherapy. Surgical resection remains the standard for CRC that has not metastasized with various options available such as open surgery, laparoscopy, and robotic surgery. In recent years, there has also been a plethora of new research into chemotherapies and immunotherapies as important treatments for metastatic or complicated CRC cases. In this review, we highlight the pathogenesis, risk factors, and treatments for CRC, while also providing a short summary of the diagnosis. Such a study will be helpful for clinicians to better manage patients with CRC.
Citation: Derek A. Corica, Dylan M. Schaap, Trenton G. Mayberry, Braydon C. Cowan, Mark R. Wakefield, Yujiang Fang. New depths: exploring the current landscape of colorectal cancer[J]. AIMS Medical Science, 2025, 12(1): 105-123. doi: 10.3934/medsci.2025008
[1] | Paul J Ross, Krishnie Srikandarajah, Julien de Naurois . Right versus left-sided colon cancer: Is it time to consider these as different diseases?. AIMS Medical Science, 2018, 5(3): 303-315. doi: 10.3934/medsci.2018.3.303 |
[2] | Vivek Radhakrishnan, Mark S. Swanson, Uttam K. Sinha . Monoclonal Antibodies as Treatment Modalities in Head and Neck Cancers. AIMS Medical Science, 2015, 2(4): 347-359. doi: 10.3934/medsci.2015.4.347 |
[3] | Yuxiang Zou, Jialong Qi, Hui Tang . Regulatory role of FOXQ1 gene and its target genes in colorectal cancer. AIMS Medical Science, 2024, 11(3): 232-247. doi: 10.3934/medsci.2024018 |
[4] | Salma M. AlDallal . Quick glance at Fanconi anemia and BRCA2/FANCD1. AIMS Medical Science, 2019, 6(4): 326-336. doi: 10.3934/medsci.2019.4.326 |
[5] | Federica Martorana, Paolo Vigneri, Stefano Sergio Cordio, Concetta Martines, Giuseppe Novello, Marco Maria Aiello, Roberto Bordonaro, Hèctor J. Soto Parra . Efficacy of A Fluoropyrimidine plus Mitomycin C in Pretreated Patients with Metastatic Colorectal Cancer Eligible for Regorafenib: A Retrospective Study. AIMS Medical Science, 2017, 4(4): 456-464. doi: 10.3934/medsci.2017.4.456 |
[6] | Nádia C. M. Okuyama, Fernando Cezar dos Santos, Kleber Paiva Trugilo, Karen Brajão de Oliveira . Involvement of CXCL12 Pathway in HPV-related Diseases. AIMS Medical Science, 2016, 3(4): 417-440. doi: 10.3934/medsci.2016.4.417 |
[7] | Ahmed Alharbi, Waleed Mansi Alshammari, Turki A K Alreshidi . Expressional correlation of Toll-like Receptor 9 (TLR9) with angiogenic factors and anti-apoptotic markers in cervical cancer cells. AIMS Medical Science, 2021, 8(1): 11-22. doi: 10.3934/medsci.2021002 |
[8] | Payal A. Shah, John Goldberg . Novel Approaches to Pediatric Cancer: Immunotherapy. AIMS Medical Science, 2015, 2(2): 104-117. doi: 10.3934/medsci.2015.2.104 |
[9] | Kwon Yong, Martin Brechbiel . Application of 212Pb for Targeted α-particle Therapy (TAT): Pre-clinical and Mechanistic Understanding through to Clinical Translation. AIMS Medical Science, 2015, 2(3): 228-245. doi: 10.3934/medsci.2015.3.228 |
[10] | Annekathryn Goodman . Vitamin C and Cancer. AIMS Medical Science, 2016, 3(1): 41-51. doi: 10.3934/medsci.2016.1.41 |
Colorectal cancer (CRC), a cancer of the large intestine and rectum, has one of the highest incidence and mortality rates, with incidences expected to nearly double in the next 25 years. The development of CRC may be caused by a collection of mutations via the chromosomal instability pathway, which results in approximately 70% of sporadic CRC cases, or the microsatellite instability pathway. At the same time, there are various modifiable and non-modifiable risk factors that increase one's chances of developing a CRC causing mutation via these pathways. There are three widely used treatments for CRC: surgical resection, chemotherapy, and immunotherapy. Surgical resection remains the standard for CRC that has not metastasized with various options available such as open surgery, laparoscopy, and robotic surgery. In recent years, there has also been a plethora of new research into chemotherapies and immunotherapies as important treatments for metastatic or complicated CRC cases. In this review, we highlight the pathogenesis, risk factors, and treatments for CRC, while also providing a short summary of the diagnosis. Such a study will be helpful for clinicians to better manage patients with CRC.
Colorectal cancer;
Chromosomal instability pathway;
Microsatellite instability pathway;
Laparoscopic-assisted surgery;
Robot assisted surgery;
Immunotherapy;
Immune-related adverse events
Cancer continues to be a challenge to healthcare systems across the world due to its high mortality rate. In the United States, cancer is the second leading cause of death, trailing only heart disease [1]. Of the many cancer types, colorectal cancer (CRC) is of concern due to its high incidence and lethality. According to the data published by the Global Cancer Observatory in 2024, there were approximately 1.9 million new CRC diagnoses and 900,000 deaths worldwide in 2022. These numbers rank second in mortality and third in incidence rate among all cancers [2]. Additionally, the incidence and mortality of CRC varies among different geographic regions according to GLOBOCAN data published in 2024 (Figure 1) [3],[4]. Of note, the incidence rates for CRC are about three times higher in nations with a high human development index (HDI) compared to nations with a low HDI. Moreover, Asia, North America, and Europe accounted for over 80% of the world's CRC diagnoses in 2022 [3]. While the current prevalence of CRC is concerning, the future outlook paints a darker picture. It is projected that the global incidence will rise to over three million by 2040 [5]. These increased projections may be explained by the rapid increase in industrialization across the globe, ushering in a lifestyle that has a longer exposure to CRC specific risk factors. The projected increase in CRC incidence punctuates the need for a more complete understanding of CRC to develop novel and effective treatments. This paper aims to provide a general understanding of CRC from a pathological perspective and highlight the currently available treatment methods.
Like many forms of cancer, the developmental pathways of CRC are diverse [6]. The majority of CRC develops from benign, precancerous lesions termed polyps. These polyps can be broken down into two categories: serrated polyps and adenomas [7]. Polyps are composed of aggregates of abnormal cells that collect new mutations within their genome over time [8]. Specific mutations are necessary for cancer development and confer advantages for tumor growth, local tissue invasion, and metastasis [7],[8]. The adenoma size has been correlated with the risk of CRC: adenomas that are 1 cm or greater have a high risk of developing into CRC [9]. There are multiple identified pathways that lead to CRC, including the chromosomal instability pathway and the microsatellite instability pathway [6].
Up to 70% of sporadic CRC can be attributed to chromosomal mutations [6]. The chromosomal instability pathway (CIP) is characterized by changes in the overall chromosome structure, including the amplification and deletion of certain regions, changes in the chromosome number, and a loss of heterozygosity [10]. Chromosomal abnormalities are caused by defects in genes responsible for DNA repair and chromosomal segregation [7],[10]. Chromosomal abnormalities lead to increased mutation rates within key tumor suppressor genes and oncogenes that set the stage for CRC development [10].
Mutations to the tumor suppressor gene Adenomatous Polyposis Coli (APC) have been shown to be the first key mutations in this cascade and are seen in up to 80% of CRC cases [7],[10],[11]. APC functions to control the Wnt signaling pathway by regulating intracellular β-catenin levels. When Wnt binds to its receptor, β-catenin accumulates intracellularly and complexes with the transcription factors T-Cell Factor (TCF)/Lymphoid Enhancer Factor (LEF), which translocate into the nucleus and activates the transcription of pro-proliferative genes [7],[12]. In the absence of Wnt signaling, APC forms a breakdown complex with axis inhibition protein (AXIN), glycogen synthase kinase 3 beta (GSK3β), and casein kinase 1 alpha (CK1α). Together, this complex degrades β-catenin via the ubiquitin-proteasome pathway and prevents cell proliferation [12]. Mutations to the APC gene result in a truncated protein that prevents the formation of the β-catenin breakdown complex. The lack of complex formation leads to unregulated cell proliferation due to the inability to break down β-catenin [7].
The second key mutation in the CIP is the Kirsten Rat Sarcoma (KRAS) oncogene, which is a member of the larger RAS family of proteins that play a crucial role in signaling pathways related to cell proliferation, apoptosis, and survival [10],[13],[14]. The activation of KRAS is dependent on upstream ligand binding to cell surface receptors. Upon ligand binding, a series of enzymatic reactions leads to KRAS transitioning from a GDP-bound inactive state to a GTP-bound active state [15]. Once active, KRAS can activate the RAF/MEK/ERK and PI3K/AKT/mTOR signaling pathways, among others. Both signaling pathways result in increased gene transcription and cell proliferation [13]. Mutations to KRAS result in it being locked in the GTP-bound active state that is independent of upstream ligand binding [15]. This ligand independent activation allows for the constitutive activation of the RAF/MEK/ERK and PI3K/AKT/mTOR pathways that cause unregulated cell proliferation [13],[16]. Mutations to KRAS have been found in 40–45% of CRC patients [10]. Moreover, it has been shown that KRAS mutations are independent of adenoma size, suggesting that KRAS mutations happen early in CRC development [17]. Both findings suggest KRAS mutations may play an important role in the progression of CRC. Additionally, KRAS mutations are associated with worse treatment outcomes and confer resistance to epidermal growth factor receptor (EGFR) targeted therapies [16], owing to the importance of genetic profiling of tumors before treatment initiation.
TP53 is generally regarded as the gatekeeper of the cell cycle and is the most common gene mutation across cancer types. TP53 is a tumor suppressor gene that functions to control the cell cycle by regulating apoptosis, DNA repair, and cell senescence [18]. These processes are regulated through gene activation/inactivation, actions which are carried out by the transcription factor P53 [19]. P53's control of the cell cycle takes place at the G1-S checkpoint in response to DNA damage [20]. Cell cycle inhibition is achieved through the activation of P21, which functions to inhibit cyclin dependent kinases (CDK) and freeze the cell cycle in the late G1 phase [21]. If the DNA damage is too extensive, P53 can induce apoptosis either through the upregulation of extrinsic receptors FAS and TNFRSF10A-D or the intrinsic proapoptotic molecule BAX [19]. Overall, TP53 mutations have been found in over 60% of CRC cases [6] which makes it a key gene in the development of CRC. Interestingly, there is a higher frequency of TP53 mutations in advanced CRC cases [22] and TP53 mutations are found in only 4–26% of adenomas compared to over 50% of CRC cases [23]. Both observations suggest that TP53 mutations occur after significant tumor progression, compared to KRAS and APC, which are early mutations in tumor development. Lastly, studies have shown that TP53 mutations lead to worse outcomes for patients with late-stage CRC [24].
The second pathway leading to CRC is the microsatellite instability pathway (MSI). While not as prevalent as CRC arising from the CIP, MSI has been detected in up to 15% of CRC cases [6]. Microsatellites are repetitive sequences located throughout the genome. Their repetitive nature puts them at an increased risk of incorrect base pairing by DNA polymerase during strand replication. Incorrect base pairing is the result of the insertion or deletion of base pairs within the microsatellite areas [25]. Normally, incorrect base pairing is repaired by the mismatch repair system (MMR), which is composed of many protein dimers (MLH1, MSH2, MSH6, MLH3, MSH3, PMS1, Exo1, and PMS2) that excise mismatched base pairs, thus fixing the initial error [26]. However, defects in MMR have been shown to be a key feature in MSI laden tumors. When the MMR is damaged, incorrect base pairing can no longer be fixed, which results in instability within microsatellite areas [27]. Defects in MMR are implicated in both hereditary and sporadic forms of CRC. Germline mutations in MMR proteins MSH2, MLH1, PMS2, and MSH6 are the basis of Lynch syndrome (otherwise known as Hereditary Nonpolyposis Colorectal Carcinoma [HNPCC]). Lynch syndrome is a common hereditary condition that has been shown to increase the lifetime risk of CRC development [28]. Most of the sporadic forms of MSI related CRC are caused by epigenetic silencing of MLH1 at methylation prone CpG islands [25],[26]. Several genes have been found to have a high mutation frequency in MSI cells, including the anti-proliferative signal transduction molecule TGFβRII and proapoptotic molecules BAX and Caspase-5 [7],[29]. The inactivation of genes responsible for limiting growth and apoptosis are thought to drive the tumorigenesis seen in CRC and other cancers.
One of the strongest associated risk factors of CRC development is age. Many studies have found that the risk increases considerably after 50 years of age in both men and women [30],[31]. Moreover, the incidence rates for CRC in men aged 70–74 years increases threefold compared to males aged 50–54 years [32]. Currently, the United States Preventive Services Task Force (USPSTF) recommends CRC screening for average risk individuals aged 45 years to 75 years old to catch potential CRC cases before late-stage development [33]. In addition to age, having a first degree relative with CRC significantly increases the risk of CRC development [30]. In fact, a large meta-analysis that compared the relative risk facts to the development of CRC found that individuals with a first degree relative diagnosed with CRC had a 1.87 higher chance of developing CRC compared to someone with no family history [34]. Lastly, personal medical history also plays an important role in the risk of CRC development. Diseases that affect the large bowel and rectum, such as Crohn's Disease and Ulcerative Colitis, also increase risk [31],[35].
While age remains a significant risk factor for CRC, it is important to note that an increasing amount of CRC diagnoses are being made in adults under the age of 50. Currently, the annual percent change in the incidence of CRC in adults 18–49 is beginning to outpace the annual percent change in adults >50. Data suggests the development of opposite trends in the incidence in CRC based on the age group. In many countries, the incidence of CRC has either stabilized or declined in adults >50 and increased in adults 18–49 [36],[37]. These trends are currently not well understood, but may be fueled by young adults being exposed to risk factors earlier in life compared to previous generations.
Modifiable risk factors of CRC are a category that involves factors of lifestyle; there is evidence that risk factors can be modified to become protective factors against CRC development. Of note, obesity, or a high body mass index (BMI), is implicated as a risk factor for CRC [38]. Obesity promotes the development of a chronic inflammatory state by increasing the release of proinflammatory cytokines. Long term exposure to chronic inflammation can lead to DNA damage and cell dysregulation that may lead to cancer development [39]. Additionally, both smoking and alcohol consumption are positively correlated with CRC [38]. For alcohol, while there was no correlation found in light consumption, both moderate and heavy alcohol consumption was associated with an increased risk, with heavy consumption having a higher risk than moderate consumption [40]. Smoking is thought to increase the risk for CRC through DNA damage, which is precipitated though intracellular chemical accumulation and the hypoxic environment created by carbon monoxide [30]. Lastly, a diet high in red and processed meats is associated with an increased risk for CRC development [30],[37],[41]. Two theories have been proposed to explain the increased risk for CRC that red meat confers. Cooking meat leads to the development of the procarcinogens heterocyclic aromatic amines and polycyclic aromatic hydrocarbons [41]. Additionally, heme groups derived from red meat may produce carcinogenic substances via lipid peroxidation in the body [35].
As mentioned earlier, the USPSTF's current recommendation for CRC screening is for individuals between the ages of 45 and 75 years who are at average risk [33]. In recent years, there has been an increased emphasis on the development of noninvasive screening methods for CRC. Noninvasive screening methods offer several advantages compared to invasive screening methods. Noninvasive methods offer a quick and convenient option for screening while avoiding the extensive bowel prep and reducing risks that are associated with colonoscopies. The current standard of noninvasive screening involves the detection of blood in the stool. The Fecal Immunochemistry Test (FIT) uses antibodies to detect blood in the stool, and the Guideline Fecal Occult Blood Test (gFOBT) detects activity of peroxidases found in red blood cells [42]. Both tests have been proven effective in detecting CRC, with studies suggesting that the implementation of such tests has reduced the relative incidence of CRC development by 20% [33]. Moreover, studies have shown FIT and gFOBT have sensitivities for CRC of 79% and 70%, respectively [33],[42]. While these numbers are promising, the diagnostic value of stool-based methods is limited by a lack of sensitivity to advanced adenomas, thus posing an important challenge for these screening methods [43],[44]. New cutting-edge screening tests involve the use of body fluid samples, mainly blood, to screen for cancer. These “liquid biopsies” rely on cells shedding DNA, RNA, and proteins into body fluids as they die, which can be readily sampled and analyzed in a blood test [45]. To date, the most validated blood-based screening measure is SEPT9 DNA testing. SEPT9 is a tumor suppressor gene that becomes inactivated through methylation as CRC progresses, and its detection in the blood can signal CRC pathology. Studies have reported a sensitivity of 68% for all stages of CRC [46]. Beyond SEPT9, blood-based screening methods are being developed that harness the ability to detect cancer before the spread to other tissues. Cohen et al. demonstrated that cancerSEEK, which is a screening test that is able to detect vast quantities of cancer specific mutations in eight different cancers, including CRC, reported a 72% and 78% sensitivity for stage II and III cancers, respectively [47]. Of note, cancerSEEK reported an 84% accuracy for CRC on the first prediction and a 100% accuracy for CRC based on its top two predictions [47]. Advances in noninvasive screenings has made screening much more accurate; however, the direct visualization of the large bowel by colonoscopy remains the gold standard to detect CRC [43]. An important aspect of colonoscopy screenings is the dual effect of visualization of growths and the ability to biopsy them, which is critical for diagnosis and staging [48].
Once a biopsy is performed, the sample can be assessed and staged to help with diagnostic clarity. The TNM system created by the American Joint Committee on Cancer (AJCC) and the Union for International Cancer Control (UICC) has been the gold standard for cancer staging for decades [49],[50]. The TNM staging system is broken up into three components: primary tumor growth, lymph node involvement, and distant metastasis. Together, these three components offer valuable insights into the prognosis and guidance on treatment [49]–[51]. Table 1 provides an in-depth look into the TNM staging system.
T-Primary Tumor Growth |
|
T0 | No Evidence of primary tumor |
Tis | Carcinoma in situ |
T1 | Tumor invades the submucosa |
T2 | Tumor invades the muscularis propria |
T3 | Tumor invades the subserosa or non-peritonealized pericolic or perirectal tissue |
T4 | Direct invasion of surrounding organs or structures or perforation of the visceral peritoneum |
T4a | Tumor perforates the visceral peritoneum |
T4b | Tumor invades surrounding organs |
N-Lymph node involvement |
|
N0 | No regional lymph node involvement |
N1 | Spread to 1–3 regional lymph nodes |
N1a | Spread to 1 lymph node |
N1b | Spread to 2–3 lymph nodes |
N1c | Tumor deposits into the subserosa or non-peritonealized pericolic or perirectal tissue without lymph node spread |
N2 | Spread to 4 or more regional lymph nodes |
N2a | Spread to 4–6 regional lymph nodes |
N2b | Spread to 7 or more regional lymph nodes |
M-Metastasis |
|
M0 | No distant metastasis |
M1 | Distant metastasis present |
M1a | Metastasis confined to one organ without peritoneal metastases |
M1b | Metastasis to one or more organs |
M1c | Metastasis to the peritoneum with or without organ involvement |
Note:
CRC is accompanied by a high 5-year survivability prior to metastasizing. However, the survivability drops to 13% following metastasis [52]. Technological limitations, a lack of early diagnostic techniques, and an absence of symptoms during the early stages of CRC progression contribute to approximately 50% of CRC being metastatic at the time of diagnosis [53]. The poor prognosis associated with metastasis of CRC highlights the need for effective treatments and diagnostic techniques to increase early detection and successful treatment regimens.
Surgical resection remains the most effective form of treatment for advanced-stage colorectal cancer despite advancements in chemotherapy, radiotherapy, and immunotherapy [54]. There are two broad categories of surgical procedures that may take place: open surgery and minimally invasive surgery (MIS). MIS can be further separated into conventional laparoscopy, single incision laparoscopic surgery (SILS), robotic surgery, natural orifice transluminal endoscopy surgery (NOTES), natural orifice specimen extraction surgery (NOSES), and transanal total mesenteric excision (TaTME) [55].
Despite advancements in new surgical options, open surgery has been the conventional method used to surgically treat CRC for the past century. Open surgery provides the surgeon with a direct visualization and a greater mobility of instruments, especially in complex cases where minimally invasive techniques make it difficult to maneuver. However, there has been a marked increase in the use of MIS for CRC treatment, with laparoscopic-assisted surgery (LAS) being proposed as an alternative to open surgery in the early 1990s. LAS provides reduced scarring and a better cosmetic outcome along with an improved surgical precision that results from a high-quality, magnifying camera. Additionally, robot-assisted surgery (RAS) has advanced within the last 30 years to overcome the drawbacks associated with conventional LAS, such as a lack of a three-dimensional view and a long time to gain the necessary skill set to perform the surgery [56]. Finally, NOSES has progressed over the past several years to further minimize postoperative complications associated with a conventional laparoscopy and achieve a more pleasing scar-free outcome. Still, NOSES requires more evidence-based research before it becomes more widely accepted [57]. SILS and sole NOTES procedures have failed to gain popularity due to various reasons, such as their complexity and marginal clinical gains [55]. Therefore, much research has centered around determining the best treatment for CRC by evaluating the main outcomes of open surgery, LAS, and RAS.
Meta-analyses have provided data that supports LAS as a more effective treatment for CRC than open surgery [58],[59]. These studies have shown that patients who underwent LAS had reduced blood loss, length of hospital stay, complication rates, and mortality compared to open surgery. Furthermore, other reviews have focused on comparing the efficacy of LAS and RAS. One review of six randomized controlled trials (RCTs) concluded that LAS and RAS for CRC had similar blood loss, length of hospital stay, complication rates, and conversion to open surgery [60]. Another review of 11 RCTs supported a different conclusion that RAS had decreased blood loss, length of hospital stay, complication rate, and conversion to open surgery compared to LAS. The difference in the two studies was likely due to the sample size, and further large-sample RCTs should be conducted to provide a more conclusive comparison [61]. Although laparoscopic and robotic surgeries are supported as the more effective therapy for CRC, other complications may arise that warrant open surgery. The conversion to open surgery is an inevitable complication in some situations, and it is estimated that between 10%–16% of minimally invasive surgeries become open surgeries. Therefore, open surgery will remain a relevant surgical option in the foreseeable future [62]. Overall, surgical intervention remains the most effective treatment for CRC, and it remains up to the surgeon to determine which procedure to perform.
Chemotherapy is a common treatment for cases of CRC that are not amenable to surgery or have metastasized [63]. Chemotherapeutic approaches involve treatment regimens highlighted by multiple chemotherapeutic agents that have been approved by both the Food and Drug Administration (FDA) and European Medicines Agency (EMA) [64],[65]. The mainstays of these chemotherapeutic regimens include 5-fluorouracil (5-FU), a potent inhibitor of DNA synthesis, and leucovorin, which synergistically acts with 5-FU to increase its effect [63],[66]. 5-FU and leucovorin are combined with either the DNA cross linking agent oxaliplatin (FOLFOX) or the topoisomerase inhibitor irinotecan (FOLFIRI) [63],[67]. FOLFOX and FOLFIRI are two of the most common first line chemotherapeutic regimens offered in the United States [63],[66],[68]. Table 2 provides a deeper look into current chemotherapeutic treatments. Multiple studies looking at the overall survival (OS) for patients with metastatic colorectal cancer (mCRC) being treated with either FOLFOX or FOLFIRI have shown only slight differences between the two [69],[70]. These findings suggest that the clinical selection of chemotherapeutic regimens should be based on patient tolerability and tumor specific profiles.
Toxicity is an important aspect in chemotherapeutic treatments, and along with further metastasis, is a key reason why patients switch regimens during treatment [63]. In a comparison, Colucci found that FOLFOX was more associated with thrombocytopenia (p < 0.0001) and neuropathy (p < 0.0001), while FOLFIRI was more associated with alopecia (p < 0.0001), diarrhea (p < 0.0007) and both nausea and vomiting (p < 0.009) [69]. Therefore, patient information such as age and health status should be considered when selecting first-line treatments.
Agent | Effect | FDA/EMA approval? | Reference |
5-Fluorouracil | Pyrimidine antimetabolite that blocks DNA synthesis | Yes/Yes | [63]–[65] |
Leucovorin | Folic acid derivative that synergizes and increases 5-FU effect | Yes/Yes | [63]–[65] |
Oxaliplatin | Platinum agent that cross links DNA | Yes/Yes | [64],[65],[67] |
Irinotecan | DNA Topoisomerase inhibitor | Yes/Yes | [64],[65],[67] |
Cetuximab | Chimeric monoclonal antibody that inhibits EGFR | Yes/Yes | [64],[65],[71] |
Panitumumab | Human monoclonal antibody that inhibits EGFR | Yes/Yes | [64],[65],[72] |
Bevacizumab | Human monoclonal antibody targeting VEGF-A | Yes/Yes | [64],[65],[73] |
Aflibercept | Recombinant fusion protein that inhibits VEGFR-1 and VEGFR-2 | Yes/Yes | [64],[65],[73] |
Ramucirumab | Human monoclonal antibody that inhibits VEGFR-2 | Yes/Yes | [64],[65],[73] |
Pembrolizumab | PD-1 inhibiting antibody | Yes/Yes | [64],[65],[74] |
Nivolumab | PD-1 inhibiting antibody | Yes/Yes | [64],[65],[75] |
Ipilimumab | CTLA-4 inhibitor | Yes/Yes | [64],[65],[76] |
In recent years there has been an explosion of research into the use of targeted biologic therapies alongside conventional chemotherapeutics in the treatment of mCRC. Biologic agents can be broken up into two categories: vascular endothelial growth factor (VEGF) inhibitors and EGFR inhibitors [68].
EGFR is a member of a larger family of receptors that encompasses erythroblastosis oncogene B (ErbB) and human epidermal growth factor (HER) receptors. These receptors are expressed on the surface of cells and are responsible for cell proliferation and survival via the activation of signaling pathways such as RAS, RAF, and PI3K/AKT, among others. EGFR and HER overexpression have been found in many cancer types, including CRC [77]. This finding has led to the development and study of biologic agents capable of inhibiting these receptors, thus limiting tumor growth. Multiple studies have demonstrated the benefits of using EGFR inhibitors in combination with chemotherapy. In patients with unresectable CRC, Van Cutsem investigated the EGFR inhibitor cetuximab combined with FOLFIRI vs FOLFIRI alone and found an OS time of 24.9 months (95% CI, 22.2 months to 27.8 months) and 21.0 months (95% CI, 19.2 months to 25.7 months), respectively [71]. Another study by Douillard compared the EGFR inhibitor panitumumab combined with FOLFOX vs FOLFOX alone and found an OS of 23.9 months (95% CI, 20.3 to 28.3 months) and 19.7 months (95% CI, 17.6 to 22.6 months), respectively [72].
A key factor in a tumor's growth is the ability to increase its blood supply by building a dense network of new blood vessels. VEGF is an important signaling molecule that stimulates this process, and thus is critical in tumor growth [73]. Biologic agents such as bevacizumab, aflibercept, and ramucirumab have been approved by the Food and Drug Administration to interrupt aspects of the VEGF signaling pathway in an effort to inhibit tumor growth [68],[73]. Saltz demonstrated the median survival time in patients with mCRC was 21.3 months when treated with bevacizumab combined with FOLFOX/XELOX compared to 19.9 months when treated with a placebo combined with FOLFOX/XELOX (HR, 0.89; 97.5% CI, 0.76 to 1.03; p = 0.077) [78]. In addition, Van Cutsem investigated the efficacy of the combination of aflibercept with FOLFIRI compared to FOLFIRI alone in mCRC patients who had previously undergone treatment. This study elucidated an increase in the median survival time of 13.5 months in the combination therapy vs 12.06 months in the chemotherapy only group ([HR], 0.817; 95.34% CI, 0.713 to 0.937; p = 0.0032) [79]. The use of both EGFR and VEGF inhibitors alongside chemotherapy offers a promising future in the treatment of mCRC.
While studies show an increased survival time in mCRC patients treated with a combination therapy, these therapies are not without limitations. Studies have demonstrated that a first line treatment with EGFR inhibitors such as cetuximab resulted in the increased proliferation of KRAS mutations mCRC cells [80]. Additionally, mCRC patients that express high levels of angiopoietin-2, a pro angiogenic factor, were associated with worse treatment outcomes when treated with bevacizumab plus conventional chemotherapy [81]. Lastly, first line administration of bevacizumab has been shown to result in an increase in serum VEGF-A levels. This effect conferred a resistance to the second line use of EGFR inhibitors such as cetuximab [82]. While targeted therapies offer a new range of treatment options for patients with mCRC, natural resistance and tumor plasticity pose challenges for these therapies. More research into the effect of specific mutations and biomarkers is needed to create more thorough tumor profiles to assist with treatment selection and efficacy.
Immunotherapy (IT) has recently become the most common form of treatment for tumors that have metastasized or are recurrent solid tumors, thus surpassing both chemotherapy and targeted therapy [53]. Numerous IT modalities exist today, including immune checkpoint inhibitors (ICIs), adoptive cell therapies, monoclonal antibodies, oncolytic viruses, anti-cancer vaccines, and immune system modulators. Of the current options, ICIs have become the leading treatment by restoring the antitumor immune response in patients with colorectal cancer. ICIs can be used to target receptor checkpoint proteins such as PD-1 and CTLA-4, along with their associated ligands, to hinder the growth of cancer [52]. Currently, treatments that have been approved by the FDA include pembrolizumab and nivolumab, which are two PD-1 ICIs, and ipilimumab, which is a CTLA-4 ICI. The KEYNOTE-177 phase III study established pembrolizumab as an effective first-line therapy for dMMR-MSI-H mCRC when compared to chemotherapy [74]. The five-year follow-up assessed a five-year OS of 54.8% with a median OS of 77.5 months with pembrolizumab. This was compared to a median OS of 36.7 months and a five-year OS of 44.2% with chemotherapy [83]. One active study of interest is investigating the therapeutic potential of pembrolizumab in combination with cetuximab and the BRAF inhibitor, encorafenib. This is for a subset of patients with both BRAF V600E mutations and DNA mismatch repair-deficient (dMMR)/microsatellite instability-high (MSI-H) mCRC (NCT05217446). Ipilimumab in conjunction with nivolumab has also been approved to treat dMMR-MSI-H CRC [84]. This stems from the CheckMate 142 phase II trial. This trial has produced important data on the use of nivolumab in conjunction with ipilimumab. It was found that in previously treated patients with DNA mismatch repair-deficient (dMMR)/microsatellite instability-high (MSI-H) mCRC, this combination had an objective response rate (ORR) of 55% and a disease control rate of 80% for at least 12 weeks [76]. These results propose a greater response than the monotherapy nivolumab, which concluded an ORR of 31% and a disease control rate of 69% for at least 12 weeks [75]. Additionally, the 12-month OS was 85% in the combination therapy compared to 73% in monotherapy nivolumab. The CheckMate 142 trial recently evaluated nivolumab plus low-dose ipilimumab as a first-line therapy for patients with the same dMMR-MSI-H mCRC as previously researched. The ORR was 69% and the disease control rate was 84%. This is a promising first-line therapy in which the authors stated a randomized trial is warranted for further investigation [85]. The four-year follow-up of the CheckMate phase II trial on previously treated patients found an increased ORR from 55% to 65% and a slightly increased disease control rate of 81% [86]. There is an active phase III trial assessing nivolumab, nivolumab plus ipilimumab, and the investigator's choice of chemotherapy (NCT04008030). The CheckMate 142 phase II trial also assessed the efficacy of nivolumab plus relatimab in previously treated patients with dMMR-MSI-H mCRC. Relatimab is a lymphocyte-activation gene 3 (LAG-3) inhibitor. The investigators assessed an ORR of 50% and a disease control rate of 70%, along with an OS rate of 56% at three years. Although the results proposed a less efficacious therapy compared to nivolumab plus ipilimumab, this could still be an important therapy for those that are intolerant of a nivolumab and ipilimumab combination [87].
The classification of CRC can be broken down into three types based on their repair system (MMR) and MSI: dMMR-MSI-H tumors resulting from a defective MMR and high mutation burden; pMMR–MSI-L tumors resulting from an intact MMR and low mutation burden; and pMMR-MSS tumors resulting from an intact MMR while also lacking MSI features [84]. Unfortunately, the efficacy of the aforementioned medications is limited to patients with a dMMR-MSI-H classified tumor, which only accounts for 10%–15% of all CRC cases and 5% of mCRC cases. Fortunately, ongoing studies are working to improve the ICI efficacy for patients with the more common pMMR-MMS tumors through combination therapies with either chemotherapy, targeted therapy, or radiotherapy [52]. One such study assessed the efficacy of pembrolizumab with bevacizumab (VEGF-A monoclonal antibody) and binimetinib (MEK inhibitor) in the treatment of refractory pMMR-MSS mCRC patients. Although this treatment combination did not achieve a statistically significant higher ORR (ORR = 12% vs historical ORR = 5%) than the current treatment options, there was an observed efficacy improvement in patients without liver metastasis [88]. IT is a promising treatment modality for CRC, although similar to all other forms of treatment, there is the possibility of related adverse events.
ICIs are stifled by the high occurrence rate of immune-related adverse events (irAEs), which may present as rash, colitis, pneumonitis, endocrine, cardiac, and musculoskeletal dysfunctions [89]. Hepatotoxicity is also a common problem that occurs in 2%–25% of ICI patients. Although high-dose corticosteroids may be used to treat ICI induced hepatotoxicity, they are only found to be effective in approximately 70% of patients with hepatic reactions [90]. Even worse is the evidence of fatal side effects that number over 600 between the years 2009 and 2018 [91]. In short, while ICIs have become a staple in cancer immunotherapy targeted treatments, the side effects involved pose a true challenge for those taking and managing the treatments. Future research is needed to investigate less harmful ICIs and better treatments for irAEs.
CRC is a complex, multifaceted disease that poses many challenges to the modern medical system. This complexity limits the idea of a one size fits all cure. However, complexity should not be mistaken for dejection. While there are still many challenges to overcome, the progress that has already been made shines a light on the future. Advances in tumor profiling have allowed the birth of targeted therapies that match the features of an individual's tumor, thus resulting in better outcomes. Looking forward, an emphasis should be placed on methods to increase the screening compliance and efficacy. Noninvasive methods offer a great alternative for individuals who feel colonoscopies are too invasive while also giving accurate results that warrant further investigations. A large meta-analysis found a strong association between the cancer stage and the length of time between a positive fecal test and colonoscopy [92]. Additionally, the relative 5-year survival rate for individuals with localized CRC is 90.1% [93], while that rate drops to 69.2% for CRC that has spread to local organs/lymph nodes [93]. The rate drops even further to 11.7% for CRC that has metastasized to distant sites [49]. This highlights the importance of screening, and that early detection is the best method to save lives.
Derek A. Corica: writing—original draft, writing—review and editing. Dylan M. Schaap: writing—original draft, writing—review and editing. Trenton G. Mayberry: writing—original draft, writing—review and editing. Braydon C. Cowan: writing—original draft, writing—review and editing. Mark R. Wakefield: writing—review and editing. Yujiang Fang: conceptualization, writing—review and editing. All authors have read and agreed to the published version of the manuscript.
The authors declare they have not used Artificial Intelligence (AI) tools in the creation of this article.
[1] | Kochanek KD, Murphy SL, Xu JQ, et al. (2024) Mortality in the United States, 2022. NCHS Data Brief, no 492. Hyattsville, MD: National Center for Health Statistics. 2024. https://dx.doi.org/10.15620/cdc:135850. |
[2] | Global Cancer Observatory, World Health Organization, Colon and Rectum. Accessed 12 March 2024. Available from: https://gco.iarc.fr/today/en/fact-sheets-cancers |
[3] |
Bray F, Laversanne M, Sung H, et al. (2024) Global cancer statistics 2022: GLOBOCAN estimates of incidence and mortality worldwide for 36 cancers in 185 countries. CA Cancer J Clin 74: 229-263. https://doi.org/10.3322/caac.21834 ![]() |
[4] | Ferlay J, Ervik M, Lam F, et al. (2024) Global Cancer Observatory: Cancer Today (version 1.1). Lyon, France: International Agency for Research on Cancer. Available from: https://gco.iarc.who.int/today. |
[5] |
Morgan E, Arnold M, Gini A, et al. (2023) Global burden of colorectal cancer in 2020 and 2040: incidence and mortality estimates from GLOBOCAN. Gut 72: 338-344. https://doi.org/10.1136/gutjnl-2022-327736 ![]() |
[6] |
Sullivan BA, Noujaim M, Roper J (2022) Cause, epidemiology, and histology of polyps and pathways to colorectal cancer. Gastrointest Endosc Clin N Am 32: 177-194. https://doi.org/10.1016/j.giec.2021.12.001 ![]() |
[7] |
Nguyen LH, Goel A, Chung DC (2020) Pathways of colorectal carcinogenesis. Gastroenterology 158: 291-302. https://doi.org/10.1053/j.gastro.2019.08.059 ![]() |
[8] |
Simon K (2016) Colorectal cancer development and advances in screening. Clin Interv Aging 11: 967-976. https://doi.org/10.2147/CIA.S109285 ![]() |
[9] |
Pickhardt PJ, Kim DH (2009) Colorectal cancer screening with CT colonography: key concepts regarding polyp prevalence, size, histology, morphology, and natural history. AJR Am J Roentgenol 193: 40-46. https://doi.org/10.2214/AJR.08.1709 ![]() |
[10] |
Pino MS, Chung DC (2010) The chromosomal instability pathway in colon cancer. Gastroenterology 138: 2059-2072. https://doi.org/10.1053/j.gastro.2009.12.065 ![]() |
[11] |
Powell SM, Zilz N, Beazer-Barclay Y, et al. (1992) APC mutations occur early during colorectal tumorigenesis. Nature 359: 235-237. https://doi.org/10.1038/359235a0 ![]() |
[12] |
Rim EY, Clevers H, Nusse R (2022) The Wnt pathway: from signaling mechanisms to synthetic modulators. Annu Rev Biochem 91: 571-598. https://doi.org/10.1146/annurev-biochem-040320-103615 ![]() |
[13] |
Dienstmann R, Connor K, Byrne AT (2020) COLOSSUS consortium. Precision therapy in RAS mutant colorectal cancer. Gastroenterology 158: 806-811. https://doi.org/10.1053/j.gastro.2019.12.051 ![]() |
[14] |
Fernández-Medarde A, Santos E (2011) Ras in cancer and developmental diseases. Genes Cancer 2: 344-358. https://doi.org/10.1177/1947601911411084 ![]() |
[15] |
Simanshu DK, Nissley DV, McCormick F (2017) RAS proteins and their regulators in human disease. Cell 170: 17-33. https://doi.org/10.1016/j.cell.2017.06.009 ![]() |
[16] |
Zhu G, Pei L, Xia H, et al. (2021) Role of oncogenic KRAS in the prognosis, diagnosis and treatment of colorectal cancer. Mol Cancer 20: 143. https://doi.org/10.1186/s12943-021-01441-4 ![]() |
[17] |
McLellan EA, Owen RA, Stepniewska KA, et al. (1993) Lemoine NR. High frequency of K-ras mutations in sporadic colorectal adenomas. Gut 34: 392-396. https://doi.org/10.1136/gut.34.3.392 ![]() |
[18] |
Naccarati A, Polakova V, Pardini B, et al. (2012) Mutations and polymorphisms in TP53 gene--an overview on the role in colorectal cancer. Mutagenesis 27: 211-218. https://doi.org/10.1093/mutage/ger067 ![]() |
[19] |
Fischer M (2017) Census and evaluation of p53 target genes. Oncogene 36: 3943-3956. https://doi.org/10.1038/onc.2016.502 ![]() |
[20] |
Lin D, Shields MT, Ullrich SJ, et al. (1992) Growth arrest induced by wild-type p53 protein blocks cells prior to or near the restriction point in late G1 phase. Proc Natl Acad Sci USA 89: 9210-9214. https://doi.org/10.1073/pnas.89.19.9210 ![]() |
[21] |
Engeland K (2022) Cell cycle regulation: p53-p21-RB signaling. Cell Death Differ 29: 946-960. https://doi.org/10.1038/s41418-022-00988-z ![]() |
[22] |
Iacopetta B, Russo A, Bazan V, et al. (2006) Functional categories of TP53 mutation in colorectal cancer: results of an International Collaborative Study. Ann Oncol 217: 842-847. https://doi.org/10.1093/annonc/mdl035 ![]() |
[23] |
Leslie A, Carey FA, Pratt NR (2002) The colorectal adenoma-carcinoma sequence. Br J Surg 89: 845-860. https://doi.org/10.1046/j.1365-2168.2002.02120.x ![]() |
[24] |
Westra JL, Schaapveld M, Hollema H, et al. (2005) Determination of TP53 mutation is more relevant than microsatellite instability status for the prediction of disease-free survival in adjuvant-treated stage III colon cancer patients. J Clin Oncol 23: 5635-5643. https://doi.org/10.1200/JCO.2005.04.096 ![]() |
[25] |
Söreide K, Janssen EA, Söiland H, et al. (2006) Microsatellite instability in colorectal cancer. Br J Surg 93: 395-406. ![]() |
[26] | De' Angelis GL, Bottarelli L, Azzoni C, et al. (2018) Microsatellite instability in colorectal cancer. Acta Biomed 89: 97-101. https://doi.org/10.23750/abm.v89i9-S.7960 |
[27] | Parsons R, Li GM, Longley MJ, et al. (1993) Hypermutability and mismatch repair deficiency in RER+ tumor cells. Cell 75: 1227-1236. https://doi.org/10.1016/0092-8674(93)90331-j |
[28] |
Hendriks YM, de Jong AE, Morreau H, et al. (2006) Diagnostic approach and management of Lynch syndrome (hereditary nonpolyposis colorectal carcinoma): a guide for clinicians. CA Cancer J Clin 56: 213-225. https://doi.org/10.3322/canjclin.56.4.213 ![]() |
[29] |
Iacopetta B, Grieu F, Amanuel B (2010) Microsatellite instability in colorectal cancer. Asia Pac J Clin Oncol 6: 260-269. https://doi.org/10.1111/j.1743-7563.2010.01335.x ![]() |
[30] |
Sninsky JA, Shore BM, Lupu GV (2022) Crockett SD. Risk factors for colorectal polyps and cancer. Gastrointest Endosc Clin N Am 32: 195-213. https://doi.org/10.1016/j.giec.2021.12.008 ![]() |
[31] |
Lewandowska A, Rudzki G, Lewandowski T (2022) Risk factors for the diagnosis of colorectal cancer. Cancer Control 29. https://doi.org/10.1177/10732748211056692 ![]() |
[32] |
Betesh AL, Schnoll-Sussman FH (2021) Colorectal cancer screening in the elderly. Clin Geriatr Med 37: 173-183. https://doi.org/10.1016/j.cger.2020.08.012 ![]() |
[33] | Gupta S (2022) Screening for colorectal cancer. Hematol Oncol Clin North Am 36: 393-414. https://doi.org/10.1016/j.hoc.2022.02.001 |
[34] |
Mehraban Far P, Alshahrani A, Yaghoobi M (2019) Quantitative risk of positive family history in developing colorectal cancer: A meta-analysis. World J Gastroenterol 25: 4278-4291. https://doi.org/10.3748/wjg.v25.i30.4278 ![]() |
[35] | Mármol I, Sánchez-de-Diego C, Pradilla Dieste A, et al. (2017) Colorectal carcinoma: A general overview and future perspectives in colorectal cancer. Int J Mol Sci 18: 197. https://doi.org/10.3390/ijms18010197 |
[36] |
Siegel RL, Torre LA, Soerjomataram I, et al. (2019) Global patterns and trends in colorectal cancer incidence in young adults. Gut 68: 2179-2185. https://doi.org/10.1136/gutjnl-2019-319511 ![]() |
[37] |
Bailey CE, Hu CY, You YN, et al. (2015) Increasing disparities in the age-related incidences of colon and rectal cancers in the United States, 1975–2010. JAMA Surg 150: 17-22. https://doi.org/10.1001/jamasurg.2014.1756 ![]() |
[38] |
O'Sullivan DE, Sutherland RL, Town S, et al. (2022) Risk factors for early-onset colorectal cancer: A systematic review and Meta-analysis. Clin Gastroenterol Hepatol 20: 1229-1240.e5. https://doi.org/10.1016/j.cgh.2021.01.037 ![]() |
[39] |
Ionescu VA, Gheorghe G, Bacalbasa N, et al. (2023) Colorectal cancer: from risk factors to oncogenesis. Medicina 59: 1646. https://doi.org/10.3390/medicina59091646 ![]() |
[40] |
Zhou X, Wang L, Xiao J, et al. (2022) Alcohol consumption, DNA methylation and colorectal cancer risk: Results from pooled cohort studies and Mendelian randomization analysis. Int J Cancer 151: 83-94. https://doi.org/10.1002/ijc.33945 ![]() |
[41] |
Farvid MS, Sidahmed E, Spence ND, et al. (2021) Consumption of red meat and processed meat and cancer incidence: a systematic review and meta-analysis of prospective studies. Eur J Epidemiol 36: 937-951. https://doi.org/10.1007/s10654-021-00741-9 ![]() |
[42] |
Ladabaum U, Dominitz JA, Kahi C, et al. (2020) Strategies for colorectal cancer screening. Gastroenterology 158: 418-432. https://doi.org/10.1053/j.gastro.2019.06.043 ![]() |
[43] |
Wu Z, Li Y, Zhang Y, et al. (2020) Colorectal cancer screening methods and molecular markers for early detection. Technol Cancer Res Treat 19: 1533033820980426. https://doi.org/10.1177/1533033820980426 ![]() |
[44] |
Schreuders EH, Ruco A, Rabeneck L, et al. (2015) Colorectal cancer screening: a global overview of existing programmes. Gut 64: 1637-1649. https://doi.org/10.1136/gutjnl-2014-309086 ![]() |
[45] |
Shaukat A, Levin TR (2022) Current and future colorectal cancer screening strategies. Nat Rev Gastroenterol Hepatol 19: 521-531. https://doi.org/10.1038/s41575-022-00612-y ![]() |
[46] |
Potter NT, Hurban P, White MN, et al. (2014) Validation of a real-time PCR-based qualitative assay for the detection of methylated SEPT9 DNA in human plasma. Clin Chem 60: 1183-1191. https://doi.org/10.1373/clinchem.2013.221044 ![]() |
[47] |
Cohen JD, Li L, Wang Y, et al. (2018) Detection and localization of surgically resectable cancers with a multi-analyte blood test. Science 359: 926-930. https://doi.org/10.1126/science.aar3247 ![]() |
[48] |
Barnell EK, Wurtzler EM, La Rocca J, et al. (2023) Multitarget stool RNA test for colorectal cancer screening. JAMA 330: 1760-1768. https://doi.org/10.1001/jama.2023.22231 ![]() |
[49] |
Mahmoud NN (2022) Colorectal cancer: preoperative evaluation and staging. Surg Oncol Clin N Am 31: 127-141. https://doi.org/10.1016/j.soc.2021.12.001 ![]() |
[50] |
Nagtegaal ID, Quirke P, Schmoll HJ (2011) Has the new TNM classification for colorectal cancer improved care?. Nat Rev Clin Oncol 9: 119-123. https://doi.org/10.1038/nrclinonc.2011.157 ![]() |
[51] |
Chen K, Collins G, Wang H, et al. (2021) Pathological features and prognostication in colorectal cancer. Curr Oncol 28: 5356-5383. https://doi.org/10.3390/curroncol28060447 ![]() |
[52] |
Carlsen L, Huntington KE, El-Deiry WS (2022) Immunotherapy for Colorectal Cancer: Mechanisms and Predictive Biomarkers. Cancers 14: 1028. https://doi.org/10.3390/cancers14041028 ![]() |
[53] |
Zhao W, Jin L, Chen P, et al. (2022) Colorectal cancer immunotherapy-Recent progress and future directions. Cancer Lett 545: 215816. https://doi.org/10.1016/j.canlet.2022.215816 ![]() |
[54] |
Shinji S, Yamada T, Matsuda A, et al. (2022) Recent Advances in the Treatment of Colorectal Cancer: A Review. J Nippon Med Sch 89: 246-254. ![]() |
[55] |
Merchant J, McArthur D, Ferguson H, et al. (2021) Concepts and prospects of minimally invasive colorectal cancer surgery. Clin Radiol 76: 889-895. https://doi.org/10.1016/j.crad.2021.09.013 ![]() |
[56] | Vilsan J, Maddineni SA, Ahsan N, et al. (2023) Open, Laparoscopic, and Robotic Approaches to Treat Colorectal Cancer: A Comprehensive Review of Literature. Cureus 15: e38956. https://doi.org/10.7759/cureus.38956 |
[57] |
Zhang M, Liu Z, Wang X (2022) Is natural orifice specimen extraction surgery the future direction of minimally invasive colorectal surgery?. Surg Open Sci 10: 106-110. https://doi.org/10.1016/j.sopen.2022.08.001 ![]() |
[58] |
Song XJ, Liu ZL, Zeng R, et al. (2019) A meta-analysis of laparoscopic surgery versus conventional open surgery in the treatment of colorectal cancer. Medicine 98: e15347. https://doi.org/10.1097/MD.0000000000015347 ![]() |
[59] |
Luo W, Wu M, Chen Y (2022) Laparoscopic versus open surgery for elderly patients with colorectal cancer: a systematic review and meta-analysis of matched studies. ANZ J Surg 92: 2003-2017. https://doi.org/10.1111/ans.17972 ![]() |
[60] |
Yang L, Fang C, Bi T, et al. (2023) Efficacy of robot-assisted vs. laparoscopy surgery in the treatment of colorectal cancer: A systematic review and meta-analysis. Clin Res Hepatol Gastroenterol 47: 102176. https://doi.org/10.1016/j.clinre.2023.102176 ![]() |
[61] |
Huang Z, Huang S, Huang Y, et al. (2023) Comparison of robotic-assisted versus conventional laparoscopic surgery in colorectal cancer resection: a systemic review and meta-analysis of randomized controlled trials. Front Oncol 13: 1273378. https://doi.org/10.3389/fonc.2023.1273378 ![]() |
[62] |
Zhao Z, Gu J (2022) Open surgery in the era of minimally invasive surgery. Chin J Cancer Res 34: 63-65. https://doi.org/10.21147/j.issn.1000-9604.2022.01.06 ![]() |
[63] |
Biller LH, Schrag D (2021) Diagnosis and treatment of metastatic colorectal cancer: A review. JAMA 325: 669-685. https://doi.org/10.1001/jama.2021.0106 ![]() |
[64] | European Medicines AgencyHomepage. Accessed March 8, 2025. Available from: https://www.ema.europa.eu/en/homepage |
[65] | National Cancer InstituteColorectal cancer treatment drugs. Accessed March 8, 2025. Available from: https://www.cancer.gov/about-cancer/treatment/drugs/colorectal |
[66] |
Kuipers EJ, Grady WM, Lieberman D, et al. (2015) Colorectal cancer. Nat Rev Dis Primers 1: 15065. https://doi.org/10.1038/nrdp.2015.65 ![]() |
[67] |
Glimelius B, Stintzing S, Marshall J, et al. (2021) Metastatic colorectal cancer: Advances in the folate-fluoropyrimidine chemotherapy backbone. Cancer Treat Rev 98: 102218. https://doi.org/10.1016/j.ctrv.2021.102218 ![]() |
[68] |
Shin AE, Giancotti FG, Rustgi AK (2023) Metastatic colorectal cancer: mechanisms and emerging therapeutics. Trends Pharmacol Sci 44: 222-236. https://doi.org/10.1016/j.tips.2023.01.003 ![]() |
[69] |
Colucci G, Gebbia V, Paoletti G, et al. (2005) Phase III randomized trial of FOLFIRI versus FOLFOX4 in the treatment of advanced colorectal cancer: a multicenter study of the Gruppo Oncologico Dell'Italia Meridionale. J Clin Oncol 23: 4866-4875. https://doi.org/10.1200/JCO.2005.07.113 ![]() |
[70] |
Nelson MA, Shetty S, Kulakodlu M, et al. (2011) A comparison of mortality and costs associated with FOLFOX versus FOLFIRI in stage IV colorectal cancer. J Med Econ 14: 179-186. https://doi.org/10.3111/13696998.2011.556693 ![]() |
[71] |
Van Cutsem E, Köhne CH, Hitre E, et al. (2009) Cetuximab and chemotherapy as initial treatment for metastatic colorectal cancer. N Engl J Med 360: 1408-1417. https://doi.org/10.1056/NEJMoa0805019 ![]() |
[72] |
Douillard JY, Siena S, Cassidy J, et al. (2010) Randomized, phase III trial of panitumumab with infusional fluorouracil, leucovorin, and oxaliplatin (FOLFOX4) versus FOLFOX4 alone as first-line treatment in patients with previously untreated metastatic colorectal cancer: the PRIME study. J Clin Oncol 28: 4697-4705. https://doi.org/10.1200/JCO.2009.27.4860 ![]() |
[73] |
Itatani Y, Kawada K, Yamamoto T, et al. (2018) Resistance to anti-angiogenic therapy in Cancer-Alterations to Anti-VEGF Pathway. Int J Mol Sci 19: 1232. https://doi.org/10.3390/ijms19041232 ![]() |
[74] |
Diaz LA, Shiu KK, Kim TW, et al. (2022) Pembrolizumab versus chemotherapy for microsatellite instability-high or mismatch repair-deficient metastatic colorectal cancer (KEYNOTE-177): final analysis of a randomised, open-label, phase 3 study. Lancet Oncol 23: 659-670. https://doi.org/10.1016/S1470-2045(22)00197-8 ![]() |
[75] |
Overman MJ, McDermott R, Leach JL, et al. (2017) Nivolumab in patients with metastatic DNA mismatch repair-deficient or microsatellite instability-high colorectal cancer (CheckMate 142): an open-label, multicentre, phase 2 study. Lancet Oncol 18: 1182-1191. https://doi.org/10.1016/S1470-2045(17)30422-9 ![]() |
[76] |
Overman MJ, Lonardi S, Wong KYM, et al. (2018) Durable clinical benefit with nivolumab plus ipilimumab in DNA mismatch repair-deficient/microsatellite instability-high metastatic colorectal cancer. J Clin Oncol 36: 773-779. https://doi.org/10.1200/JCO.2017.76.9901 ![]() |
[77] |
Xie YH, Chen YX, Fang JY (2020) Comprehensive review of targeted therapy for colorectal cancer. Signal Transduct Target Ther 5: 22. https://doi.org/10.1038/s41392-020-0116-z ![]() |
[78] |
Saltz LB, Clarke S, Díaz-Rubio E, et al. (2008) Bevacizumab in combination with oxaliplatin-based chemotherapy as first-line therapy in metastatic colorectal cancer: a randomized phase III study. J Clin Oncol 26: 2013-2019. https://doi.org/10.1200/JCO.2007.14.9930 ![]() |
[79] |
Van Cutsem E, Tabernero J, Lakomy R, et al. (2012) Addition of aflibercept to fluorouracil, leucovorin, and irinotecan improves survival in a phase III randomized trial in patients with metastatic colorectal cancer previously treated with an oxaliplatin-based regimen. J Clin Oncol 30: 3499-3506. https://doi.org/10.1200/JCO.2012.42.8201 ![]() |
[80] | Siravegna G, Mussolin B, Buscarino M, et al. (2015) Clonal evolution and resistance to EGFR blockade in the blood of colorectal cancer patients. Nat Med 21: 795-801. https://doi.org/10.1038/nm.3870 |
[81] |
Goede V, Coutelle O, Neuneier J, et al. (2010) Identification of serum angiopoietin-2 as a biomarker for clinical outcome of colorectal cancer patients treated with bevacizumab-containing therapy. Br J Cancer 103: 1407-1414. https://doi.org/10.1038/sj.bjc.6605925 ![]() |
[82] |
Derangère V, Fumet JD, Boidot R, et al. (2016) Does bevacizumab impact anti-EGFR therapy efficacy in metastatic colorectal cancer?. Oncotarget 7: 9309-9321. https://doi.org/10.18632/oncotarget.7008 ![]() |
[83] |
André T, Shiu KK, Kim TW, et al. (2025) Pembrolizumab versus chemotherapy in microsatellite instability-high or mismatch repair-deficient metastatic colorectal cancer: 5-year follow-up from the randomized phase III KEYNOTE-177 study. Ann Oncol 36: 277-284. https://doi.org/10.1016/j.annonc.2024.11.012 ![]() |
[84] |
Shan J, Han D, Shen C, et al. (2022) Mechanism and strategies of immunotherapy resistance in colorectal cancer. Front Immunol 13: 1016646. https://doi.org/10.3389/fimmu.2022.1016646 ![]() |
[85] |
Lenz HJ, Van Cutsem E, Luisa Limon M, et al. (2022) First-line nivolumab plus low-dose ipilimumab for microsatellite instability-high/mismatch repair-deficient metastatic colorectal cancer: The phase II CheckMate 142 study. J Clin Oncol 40: 161-170. https://doi.org/10.1200/JCO.21.01015 ![]() |
[86] |
André T, Lonardi S, Wong KYM, et al. (2022) Nivolumab plus low-dose ipilimumab in previously treated patients with microsatellite instability-high/mismatch repair-deficient metastatic colorectal cancer: 4-year follow-up from CheckMate 142. Ann Oncol 33: 1052-1060. https://doi.org/10.1016/j.annonc.2022.06.008 ![]() |
[87] |
Overman MJ, Gelsomino F, Aglietta M, et al. (2024) Nivolumab plus relatlimab in patients with previously treated microsatellite instability-high/mismatch repair-deficient metastatic colorectal cancer: the phase II CheckMate 142 study. J Immunother Cancer 12: e008689. https://doi.org/10.1136/jitc-2023-008689 ![]() |
[88] |
Lentz RW, Friedrich TJ, Blatchford PJ, et al. (2024) A phase II study of potentiation of pembrolizumab with binimetinib and bevacizumab in refractory microsatellite-stable colorectal cancer. Clin Cancer Res 30: 3768-3778. https://doi.org/10.1158/1078-0432.CCR-24-0090 ![]() |
[89] |
Poto R, Troiani T, Criscuolo G, et al. (2022) Holistic approach to immune checkpoint inhibitor-related adverse events. Front Immunol 13: 804597. https://doi.org/10.3389/fimmu.2022.804597 ![]() |
[90] |
Cunningham M, Gupta R, Butler M (2024) Checkpoint inhibitor hepatotoxicity: pathogenesis and management. Hepatology 79: 198-212. https://doi.org/10.1097/HEP.0000000000000045 ![]() |
[91] |
Wang DY, Salem JE, Cohen JV, et al. (2018) Fatal toxic effects associated with immune checkpoint inhibitors: A systematic review and Meta-analysis. JAMA Oncol 4: 1721-1728. https://doi.org/10.1001/jamaoncol.2018.3923 ![]() |
[92] |
Forbes N, Hilsden RJ, Martel M, et al. (2021) Association between time to colonoscopy after positive fecal testing and colorectal cancer outcomes: A systematic review. Clin Gastroenterol Hepatol 19: 1344-1354.e8. https://doi.org/10.1016/j.cgh.2020.09.048 ![]() |
[93] |
Siegel R, DeSantis C, Virgo K, et al. (2012) Cancer treatment and survivorship statistics, 2012. CA Cancer J Clin 62: 220-241. https://doi.org/10.3322/caac.21149 ![]() |
T-Primary Tumor Growth |
|
T0 | No Evidence of primary tumor |
Tis | Carcinoma in situ |
T1 | Tumor invades the submucosa |
T2 | Tumor invades the muscularis propria |
T3 | Tumor invades the subserosa or non-peritonealized pericolic or perirectal tissue |
T4 | Direct invasion of surrounding organs or structures or perforation of the visceral peritoneum |
T4a | Tumor perforates the visceral peritoneum |
T4b | Tumor invades surrounding organs |
N-Lymph node involvement |
|
N0 | No regional lymph node involvement |
N1 | Spread to 1–3 regional lymph nodes |
N1a | Spread to 1 lymph node |
N1b | Spread to 2–3 lymph nodes |
N1c | Tumor deposits into the subserosa or non-peritonealized pericolic or perirectal tissue without lymph node spread |
N2 | Spread to 4 or more regional lymph nodes |
N2a | Spread to 4–6 regional lymph nodes |
N2b | Spread to 7 or more regional lymph nodes |
M-Metastasis |
|
M0 | No distant metastasis |
M1 | Distant metastasis present |
M1a | Metastasis confined to one organ without peritoneal metastases |
M1b | Metastasis to one or more organs |
M1c | Metastasis to the peritoneum with or without organ involvement |
Note:
Agent | Effect | FDA/EMA approval? | Reference |
5-Fluorouracil | Pyrimidine antimetabolite that blocks DNA synthesis | Yes/Yes | [63]–[65] |
Leucovorin | Folic acid derivative that synergizes and increases 5-FU effect | Yes/Yes | [63]–[65] |
Oxaliplatin | Platinum agent that cross links DNA | Yes/Yes | [64],[65],[67] |
Irinotecan | DNA Topoisomerase inhibitor | Yes/Yes | [64],[65],[67] |
Cetuximab | Chimeric monoclonal antibody that inhibits EGFR | Yes/Yes | [64],[65],[71] |
Panitumumab | Human monoclonal antibody that inhibits EGFR | Yes/Yes | [64],[65],[72] |
Bevacizumab | Human monoclonal antibody targeting VEGF-A | Yes/Yes | [64],[65],[73] |
Aflibercept | Recombinant fusion protein that inhibits VEGFR-1 and VEGFR-2 | Yes/Yes | [64],[65],[73] |
Ramucirumab | Human monoclonal antibody that inhibits VEGFR-2 | Yes/Yes | [64],[65],[73] |
Pembrolizumab | PD-1 inhibiting antibody | Yes/Yes | [64],[65],[74] |
Nivolumab | PD-1 inhibiting antibody | Yes/Yes | [64],[65],[75] |
Ipilimumab | CTLA-4 inhibitor | Yes/Yes | [64],[65],[76] |
T-Primary Tumor Growth |
|
T0 | No Evidence of primary tumor |
Tis | Carcinoma in situ |
T1 | Tumor invades the submucosa |
T2 | Tumor invades the muscularis propria |
T3 | Tumor invades the subserosa or non-peritonealized pericolic or perirectal tissue |
T4 | Direct invasion of surrounding organs or structures or perforation of the visceral peritoneum |
T4a | Tumor perforates the visceral peritoneum |
T4b | Tumor invades surrounding organs |
N-Lymph node involvement |
|
N0 | No regional lymph node involvement |
N1 | Spread to 1–3 regional lymph nodes |
N1a | Spread to 1 lymph node |
N1b | Spread to 2–3 lymph nodes |
N1c | Tumor deposits into the subserosa or non-peritonealized pericolic or perirectal tissue without lymph node spread |
N2 | Spread to 4 or more regional lymph nodes |
N2a | Spread to 4–6 regional lymph nodes |
N2b | Spread to 7 or more regional lymph nodes |
M-Metastasis |
|
M0 | No distant metastasis |
M1 | Distant metastasis present |
M1a | Metastasis confined to one organ without peritoneal metastases |
M1b | Metastasis to one or more organs |
M1c | Metastasis to the peritoneum with or without organ involvement |
Agent | Effect | FDA/EMA approval? | Reference |
5-Fluorouracil | Pyrimidine antimetabolite that blocks DNA synthesis | Yes/Yes | [63]–[65] |
Leucovorin | Folic acid derivative that synergizes and increases 5-FU effect | Yes/Yes | [63]–[65] |
Oxaliplatin | Platinum agent that cross links DNA | Yes/Yes | [64],[65],[67] |
Irinotecan | DNA Topoisomerase inhibitor | Yes/Yes | [64],[65],[67] |
Cetuximab | Chimeric monoclonal antibody that inhibits EGFR | Yes/Yes | [64],[65],[71] |
Panitumumab | Human monoclonal antibody that inhibits EGFR | Yes/Yes | [64],[65],[72] |
Bevacizumab | Human monoclonal antibody targeting VEGF-A | Yes/Yes | [64],[65],[73] |
Aflibercept | Recombinant fusion protein that inhibits VEGFR-1 and VEGFR-2 | Yes/Yes | [64],[65],[73] |
Ramucirumab | Human monoclonal antibody that inhibits VEGFR-2 | Yes/Yes | [64],[65],[73] |
Pembrolizumab | PD-1 inhibiting antibody | Yes/Yes | [64],[65],[74] |
Nivolumab | PD-1 inhibiting antibody | Yes/Yes | [64],[65],[75] |
Ipilimumab | CTLA-4 inhibitor | Yes/Yes | [64],[65],[76] |