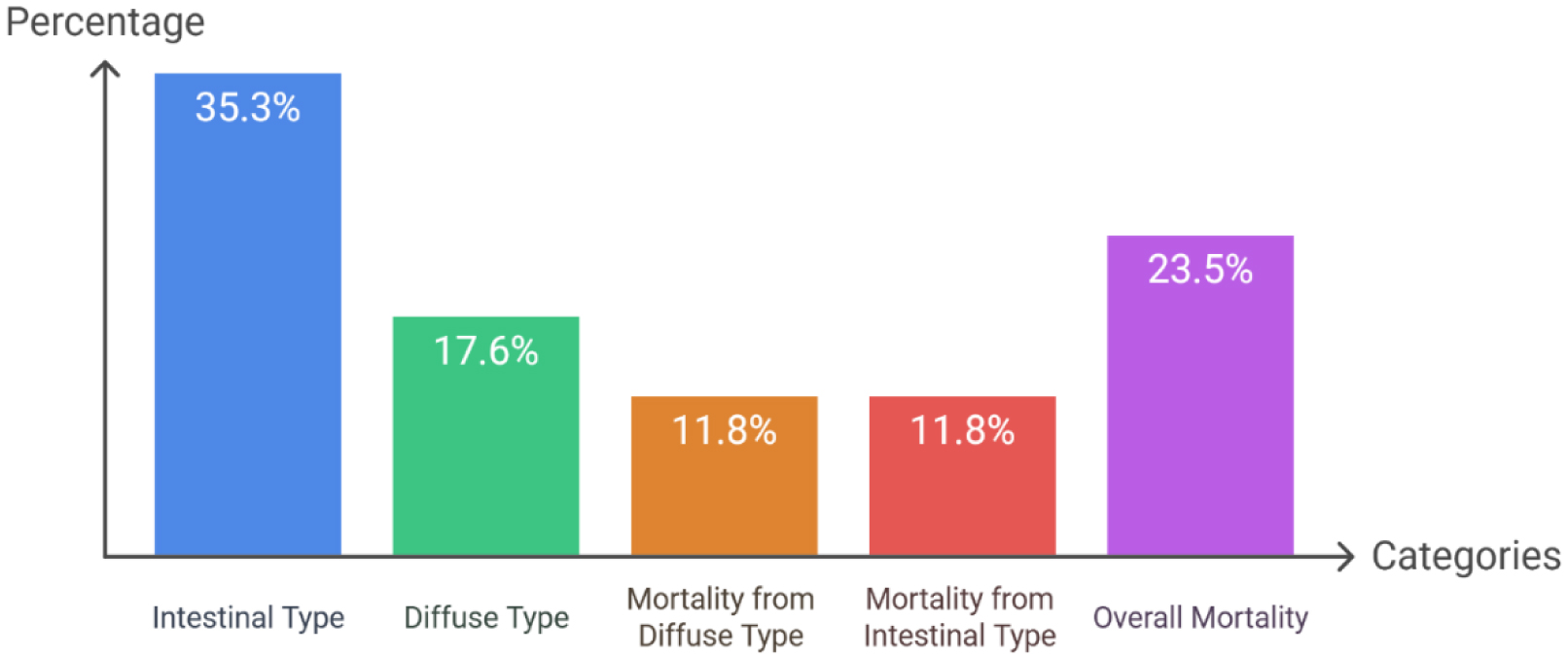
Gastric cancer (GC) remains a significant global health challenge, ranking as the fifth most common cancer and the third leading cause of cancer-related deaths worldwide. Despite declining incidence rates in certain regions, such as the United States, mortality remains high, particularly in Eastern Asia. In this review, we explore innovative immunotherapeutic strategies for GC, including immune checkpoint inhibitors, CAR-T cell therapy, and therapeutic vaccines. The role of the tumor microenvironment (TME), immune cell interactions, tumor acidity, and microbial flora in shaping therapeutic responses is discussed. Emerging epidemiological trends, such as the rise of cardia gastric cancer and shifts in traditional risk factor profiles, underscore the need for targeted prevention strategies. While immunotherapy offers promising opportunities, challenges like therapeutic resistance and personalization of treatment persist. We aimed to highlight advancements in GC immunotherapy to promote research and improve patient outcomes.
Citation: Mujibullah Sheikh, Pranita S. Jirvankar. Innovative immunotherapeutic strategies for gastric cancer: A comprehensive review[J]. AIMS Allergy and Immunology, 2025, 9(1): 27-55. doi: 10.3934/Allergy.2025003
[1] | Kochar Khasro Saleh, Semih Dalkiliç, Lütfiye Kadioğlu Dalkiliç, Bahra Radhaa Hamarashid, Sevda Kirbağ . Targeting cancer cells: from historic methods to modern chimeric antigen receptor (CAR) T-Cell strategies. AIMS Allergy and Immunology, 2020, 4(2): 32-49. doi: 10.3934/Allergy.2020004 |
[2] | Michael D. Caponegro, Jeremy Tetsuo Miyauchi, Stella E. Tsirka . Contributions of immune cell populations in the maintenance, progression, and therapeutic modalities of glioma. AIMS Allergy and Immunology, 2018, 2(1): 24-44. doi: 10.3934/Allergy.2018.1.24 |
[3] | Katarzyna Nazimek . The complex functions of microRNA-150 in allergy, autoimmunity and immune tolerance. AIMS Allergy and Immunology, 2021, 5(4): 195-221. doi: 10.3934/Allergy.2021016 |
[4] | Ken S. Rosenthal, Daniel H. Zimmerman . J-LEAPS vaccines elicit antigen specific Th1 responses by promoting maturation of type 1 dendritic cells (DC1). AIMS Allergy and Immunology, 2017, 1(2): 89-100. doi: 10.3934/Allergy.2017.2.89 |
[5] | Laiba Noor, Syeda Momna Ishtiaq, Farhat Batool, Muhammad Imran Arshad . Age-dependent trends in adverse reactions of SARS-CoV-2 vaccines: A narrative review. AIMS Allergy and Immunology, 2024, 8(3): 146-166. doi: 10.3934/Allergy.2024008 |
[6] | Johan Garssen, Juandy Jo . Genetically-engineered T cells to treat viral hepatitis-associated liver cancer: is it possible?. AIMS Allergy and Immunology, 2017, 1(1): 43-49. doi: 10.3934/Allergy.2017.1.43 |
[7] | Issam Tout, Marie Marotel, Isabelle Chemin, Uzma Hasan . HBV and the importance of TLR9 on B cell responses. AIMS Allergy and Immunology, 2017, 1(3): 124-137. doi: 10.3934/Allergy.2017.3.124 |
[8] | Daniel Danladi Gaiya, Jonathan Danladi Gaiya, Richard Auta, Aliyu Muhammad, Bege Jonathan, Stella Kuyet Udu, Ekpa Emmanuel, Amina Shehu Bature . The journey so far with SARS-CoV-2 variants: Pathogenesis, immunity and treatments. AIMS Allergy and Immunology, 2023, 7(4): 222-250. doi: 10.3934/Allergy.2023016 |
[9] | Arosh S. Perera Molligoda Arachchige . Aging and immunity: Unraveling the complex relationship. AIMS Allergy and Immunology, 2023, 7(3): 213-221. doi: 10.3934/Allergy.2023015 |
[10] | Robert Cote, Laura Lynn Eggink, J. Kenneth Hoober . CLEC receptors, endocytosis and calcium signaling. AIMS Allergy and Immunology, 2017, 1(4): 207-231. doi: 10.3934/Allergy.2017.4.207 |
Gastric cancer (GC) remains a significant global health challenge, ranking as the fifth most common cancer and the third leading cause of cancer-related deaths worldwide. Despite declining incidence rates in certain regions, such as the United States, mortality remains high, particularly in Eastern Asia. In this review, we explore innovative immunotherapeutic strategies for GC, including immune checkpoint inhibitors, CAR-T cell therapy, and therapeutic vaccines. The role of the tumor microenvironment (TME), immune cell interactions, tumor acidity, and microbial flora in shaping therapeutic responses is discussed. Emerging epidemiological trends, such as the rise of cardia gastric cancer and shifts in traditional risk factor profiles, underscore the need for targeted prevention strategies. While immunotherapy offers promising opportunities, challenges like therapeutic resistance and personalization of treatment persist. We aimed to highlight advancements in GC immunotherapy to promote research and improve patient outcomes.
Gastric cancer;
Gastrointestinal stromal tumors;
Mortality-to-incidence ratio;
Carcinoma-associated fibroblasts;
Immune checkpoint inhibitors;
Tumor microenvironment;
Tumor-associated macrophages;
Myeloid-derived suppressor cells;
Colony-stimulating factor 1 receptor;
programmed cell death protein 1;
programmed cell death ligand 1;
Triple-negative breast cancer;
Hepatocellular carcinoma;
Renal cell carcinoma;
Endometrial cancer
Gastric cancer (GC), commonly referred to as stomach cancer, continues to be a major public health problem globally and is the fifth most prevalent cancer and third leading cause of cancer-related death globally. Histories indicating trends in the incidence of gastric cancer indicate that the incidence rate has decreased since the middle of the 20th century, and countries such as the United States have been experiencing declining incidence rates for the past few decades [1]. Nevertheless, this mortality trend is reversed by an increasing trend in gastroesophageal cancers. Adenocarcinoma is the most prevalent variety of gastric cancer that develops in the mucosa of the abdomen, and two more rare types are lymphomas and gastrointestinal stromal tumors (GISTs) [2]. Karimi et al. (2014) noted that it is important to study the incidence, survival and mortality rates of gastric cancer to address this cancer type [3]. According to GLOBOCAN 2018, GC ranks fifth among common malignant neoplasms and third in cancer mortality worldwide, mostly in males of advanced age; therefore, intervention-associated prevention is needed [4]. Furthermore, a general review of the literature revealed that GC-related factors depend on geographical location and lifestyle, including diet and Helicobacter pylori infection [5]. The increasing prevalence of cardia gastric cancer and the declining trend of noncardia types of gastric cancer suggest a changing pattern in the distribution of stomach cancer. These changes demand targeted preventive efforts, such as changing one's diet and quitting smoking [4].
Stomach cancer is one of the most life-threatening diseases in the world, with considerable geographic distinctions in incidence and mortality (Figure 1: Prevalence and mortality of gastric cancer). The number of new cases in the year has reached approximately 1.1 million, and the death toll is nearly 770,000; hence, cancer is prevalent globally [6]. These incidence rates are considerably higher among males, which are 15.8/100000 compared with 7.0/100000 among females, and the ranking countries are Eastern Asia, particularly Japan, as well as Korea [7]. For example, the incidence rate for males is 48.1 per 100000 for Japan, which clearly illustrates the geographical inequality of gastric cancer [6].
The mortality-to-incidence ratio (M:I) is also worrisome; gastric cancer is the second leading cause of cancer mortality in the world, with a high proportion of mortality in high-incidence areas of Eastern Asia [8]. For example, the mortality per 100,000 people in Mongolia is 36.5, which makes it even more crucial to devise efficient ways of prevention and early diagnosis in these countries. Additionally, forecasts suggest that if the trends persist, the rate of new cases could increase to 1.8 million for the year 2040, with a number of deaths of 1.3 million [9].
A study conducted by Miller et al. (2019) revealed that the global incidence of gastric cancer is decreasing; however, mortality from the disease is high, mainly because of regions with high incidences of Helicobacter pylori bacteria [10]. This highlights the need to understand the different epidemiology patterns related to gastric cancer, as noted by Karimi et al. (2014), who noted that risk factors for gastrointestinal cancers are multifactorial and can include diet, genetic, and immunological markers, with serologic detection being important [3]. The statistical overview of research data regarding gastric cancer is more upsetting in some directions; Miller et al. (2022) identified it as the third most lethal cancer globally, with a higher incidence of mortality among males aged over 60 years [11]. Emerging cases of cardia gastric cancer are worrisome and seem to change the epidermiology of gastric malignancies (Rawla & Barsouk, 2018). These changes may represent new dietary and procurement risks and constitute one of the reasons, forcing a deeper investigation into these patterns [4].
The disparities in the incidence and mortality of cancer across the world imply that the causes of gastric cancer are diverse in nature. These factors, such as diet systems, attitudes, and hereditary qualities, strongly affect these trends. For example, Rugge et al. (2015) suggested that these regional differences must be further considered when designing prevention measures [17]. Cardia gastric cancer incidence is on the rise, whereas the overall rate is decreasing, and this phenomenon compels researchers to consider the shifts in gastric cancer epidemiology, suggesting the possibility that traditional risk factors are changing or that new factors indicating risk are being discovered [18]. Furthermore, a relationship between Helicobacter pylori infection and gastric cancer is clear; several works suggest that Helicobacter pylori infection continues to be one of the factors determining high mortality rates among patients with this pathology [19]. The discussion of serologic markers and the discussion of precursor lesions provides future possibilities for early detection, which could increase SC mortality rates [3]. Risk factor assessment is important because all these measures have the potential to substantially decrease the incidence of gastric cancer.
However, several questions about gastric cancer development and progression remain poorly understood, even in light of more recent findings. Specifically, studies aimed at evaluating the effect of combining genetic testing with other preventive measures, including dietary alterations and smoking cessation, are lacking [20]. Moreover, Miller et al. (2022) noted that there is a need to continue providing public health initiatives that address both incidence and deaths due to gastric cancer [11].
When searching for articles and studies to reference the topic of immunotherapeutic strategies in gastric cancer, a search was conducted in a way that identified both relevant and good quality articles. The choice of databases was crucial, and to ensure that a variety of sources were obtained, only those from PubMed, Scopus, and Web of Science were selected. The search was performed from the perspective of the following specific terms: gastric cancer, immunotherapy, immune checkpoint inhibitors, and adoptive cell therapy, which formed the basis of the review. The inclusion criteria were formalized, and we focused on considering only the articles of the peer-reviewed journals and only the clinical trials that were published within the last five to ten years. The information was then extracted systematically, bearing in mind features such as efficacy, mode of action, and effects on patients. There were specific aspects that were followed during data collection and analysis: Diverse static data collection forms were utilized in each of the implemented strategies to ensure uniformity and minimize the effects of investigator-induced variability, and data extraction was performed independently by the different reviewers. Finally, this strict approach was designed to correlate data that might support subsequent studies and medical treatment of gastric cancer with immunotherapy.
Immunotherapy has become a promising therapeutic option in cancer management, with the principle of using the immune system to fight tumor development [21]. The immune–tumor interaction remains one of the key components of this therapeutic approach to consider if the anticancer effect is to be achieved [22]. The TME is underlain by diverse cell types embedded in a milieu of complementary cellular roles, such as immune cells, fibroblasts, and the ECM, all of which play major roles in modulating the immunological response to cancer. Researchers have therefore sought to reveal the multiple ways by which immunotherapy reprograms the TME and the consequences of such changes on treatment outcomes [23]. These include carcinoma-associated fibroblasts (CAFs) in the process of establishing an immunosuppressive TME, especially in the case of pancreatic cancer. Samstein et al. (2019) reported that FAP+ CAFs mediate the immune exclusion of T cells through the secretion of the chemokine CXCL12. These findings confirm that immunotherapeutic antibodies can be made more effective when directed at CAFs and that the identity of the TME should be considered when developing treatment plans. The removal of FAP+ CAFs not only enables better T-cell infiltration but also reveals which existing immunotherapies can be effective when the TME is enhanced [24].
Moving from the effects of CAFs, the interactions of cancer cells with the immune system through immune checkpoint inhibitors (ICIs) are as follows. Ru and colleagues noted that although ICIs have revolutionized cancer treatment, resistance still poses a major problem. They are highly dependent on the TME, which either supports or negates immune response efforts. The investigation of local immune changes after receiving ICI therapy is critical for improving treatment plans, indicating that future research on the development of resistance mechanisms related to function and relevance to the TME is warranted [25]. Furthermore, the immune context of tumors is shaped by different immune cells, such as tumor-associated macrophages (TAMs) and myeloid-derived suppressor cells (MDSCs). Bagchi et al. (2020) mentioned clearly that such cells are involved in immune suppression and tumor advancement. Accordingly, trying on the colony-stimulating factor 1 receptor (CSF1R) can alter macrophage behavior to improve antigen presentation in addition to T cells. This finding highlights the need to elucidate the intricate TME immune cell interactions and ways to manipulate them to increase immunotherapy efficacy [26].
Thus, assessing cellular interactions also reveals that the physicochemical characteristics of the TME also regulate the immune response. Labani-Motlagh et al. (2020) noted that tumor acidity prevents T-cell activation, which limits immunotherapeutic approaches. In their study, they showed that elimination of tumor acidity improved T-cell penetration and the effectiveness of checkpoint inhibitors, which underlines the role of manipulation of TME properties in immunotherapy optimization [27]. In addition, evidence has revealed the effects of the microbiota on tumor immunity. Zhu et al. (2015) noted that individual bacterial strains can increase T-cell proliferation by releasing metabolites such as inosine to improve immunotherapy outcomes. This has highlighted the unexplored strategy of developing combination therapy by adding microbiome manipulation to existing immunotherapeutic strategies [28].
However, this progress seems to leave some gaps in understanding regarding the relationships of the TME with immunoisolation and immune escape mechanisms. For example, the mechanisms by which tumors modulate PD-L1 to dampen T-cell activity are not fully defined. Research by Henke et al. (2020) revealed that several pathways impact the levels of PD-L1, the implication of which is that understanding these signaling pathways can provide insight into improving the effectiveness of anti-PD-L1 therapy [29]. More research on the role of the ECM should also be conducted in terms of modulating immune responses because, according to Mager et al. (2020), the role of the ECM has also been investigated, and the active participation of the ECM in tumor behavior and therapeutic reactions should be further investigated [30].
As illustrated by the immune response activation process shown in Figure 2, dendritic cells and T cells play crucial roles. It starts with the elimination of antigens by immature dendritic cells. These cells then mature and present the antigens to T cells in presenting the antigens, and most of the maturity occurs on the dendrite. This interaction occurs through the T-cell receptor and major histocompatibility complex molecules. Importantly, antigen presentation occurs through MHC II in T cells for immunoglobulin and MHC I for cytotoxic T cells; in this case, T helper 2 cells appear from CD4+ T cells and, in turn, release interferon-gamma to activate natural killer cells, granulocytes, and macrophages. CD8+ T cells further divide into cytotoxic T cells and kill these tumor cells. Additionally, some T cells become memory T cells with a high ability for immunity to pathogens or foreign substances. The ‘theater of immune response’ you see here identifies elements of the adaptive immune response and explains the actions of cell types and molecules in fighting infections and tumors.
Known as immune-oncology agents, immune checkpoint inhibitors (ICIs) have radically altered approaches to cancer treatment by increasing the immune system's capacity to identify and destroy malignancies [31]. The development of ICIs dates to the discovery of immune checkpoints, which are regulatory mechanisms that tumors use to hide from the immune system. The first major step forward occurred in 2011, when the FDA gave ipilimumab (Yervoy), an antibody against CTLA-4, a green light, the beginning of the modern period of cancer therapy [32]. Pembrolizumab and nivolumab have emerged as PD-1/pD-L1 inhibitors that have revolutionized the treatment of several types of cancer. Immune checkpoint targeting was proposed on the basis of basic discoveries that were made in the late 1990s and early 2000s [33]. The work of James Allison and Tasuku Honjo identified CTLA-4 and PD-1 as avenues of interest for therapy [34]. Based on this work, he revealed that strengthening antitumor immunity with the help of blocking CTLA-4 was possible, which led to the development of ipilimumab. Similarly, young Honjo built upon another researcher's discoveries to establish subsequent PD-1 and PD-L1 inhibitors [35].
Table 1 lists approved immune checkpoint inhibitors from 2010–2024, targets, approval years, indications, and reference information. In this paper, we provide an outline of the state-of-the-art use of ICIs in treating cancer and points to further advancements in this area.
Drug Name | Brand Name | Target | Year Approved | Indications | Ref |
Ipilimumab | Yervoy | CTLA-4 | 2011 | Metastatic melanoma, renal cell carcinoma, colorectal cancer | [36],[37] |
Nivolumab | Opdivo | PD-1 | 2014 | Melanoma, lung cancer, kidney cancer, bladder cancer | [38],[39] |
Pembrolizumab | Keytruda | PD-1 | 2014 | Melanoma, lung cancer, head and neck cancer, Hodgkin's lymphoma | [40] |
Atezolizumab | Tecentriq | PD-L1 | 2016 | Bladder cancer, non-small cell lung cancer, breast cancer | [41] |
Avelumab | Bavencio | PD-L1 | 2017 | Merkel cell carcinoma, urothelial carcinoma | [42] |
Durvalumab | Imfinzi | PD-L1 | 2017 | Non-small cell lung cancer, small cell lung cancer | [43] |
Cemiplimab | Libtayo | PD-1 | 2021 | Squamous cell carcinoma of the skin, non-small cell lung cancer | [44] |
Dostarlimab | Jemperli | PD-1 | 2021 | Endometrial cancer | [45] |
Tremelimumab | Imjudo | CTLA-4 | 2022 | Hepatocellular carcinoma (in combination with Durvalumab) | [46] |
Toripalimab | Loqtorzi | PD-1 | 2023 | Nasopharyngeal carcinoma | [47] |
Spartalizumab | PDR001 | PD-1 | TBD | Under investigation for various solid tumors and lymphomas | |
Abemaciclib | Verzenio | CDK4/6 | 2017 | Breast cancer (in combination with pembrolizumab) | [48] |
Tislelizumab | Baiyin | PD-1 | 2022 | Non-small cell lung cancer | [49] |
Rovalpituzumab | Rova-T | DLL3 | TBD | Small cell lung cancer | [50] |
Programmed cell death protein 1 (PD-1) and its ligand (PD-L1) immune checkpoint inhibitors (ICIs) have revolutionized cancer treatment by engaging the immune system in identifying and eliminating cancer cells. The blockade of PD-1/PD-L1 interactions is of paramount importance during this process since it abrogates the inhibitory signals that otherwise reign in the T-cell response against tumors. A small study based on a multicenter phase 1 trial revealed that the application of an anti-PD-L1 antibody leads to long-term regression of the tumor mass in most advanced cancer patients, as evidenced by the objective response, which varies between 6% and 17% [51]. The historical development of ICIs describes a gradual step from the first approval of anti-CTLA-4 therapy for melanoma to the development of PD-1/PD-L1 inhibitors. These ICIs have been shown to be effective in treating various cancers. Nonetheless, the review highlights a significant challenge: Therapeutic failure and resistance to ICIs mean that the number of patients who can obtain a long-lasting therapeutic benefit remains limited. Understanding the nature of this resistance is critical for modulating treatment regimens, and the mechanisms involved in the development of this resistance remain to be characterized. For example, researchers conducting clinical trials, such as KEYNOTE-649, aim to study combined therapeutic approaches that potentiate PD-1/PD-L1 inhibition to further increase the survival status of patients [23]. A comprehensive understanding of the PD-1/PD-L1 pathway is crucial for the design of cancer immunotherapies. Strong evidence suggests that this pathway is an important way through which tumors are shielded from immune recognition. The presence of the PD-L1 protein on tumor cells can inhibit T cells, leading to an immune response-tolerant checkpoint with certain types of carcinogenesis [52]. As highlighted by findings from this review, it is important that the treatment of this axis calls for new strategies, especially for highly metabolic cancer types such as TNBC. Integrating PD-1/PD-L1 inhibitors with other drugs, including MEK inhibitors, increases the likelihood that the treatment efficiency and survival rates of patients will increase [53]. The combination strategies are summarized effectively in Figure 3 below. There is a new triple regimen of camrelizumab, an anti-PD-1 monoclonal antibody, apatinib, a newly approved angiogenesis inhibitor, and fuzuloparib, a newly formulated PARP inhibitor, which has recently exhibited acceptable safety and preliminary antitumor efficacy in TNBC [54]. The combination of PD-1/PD-L1 inhibitors with angiogenesis inhibitors has also demonstrated activity in third-line therapy for advanced hepatocellular carcinoma (HCC), third- and later-line therapy for renal cell carcinoma (RCC) and endometrial cancer (EC). Furthermore, combining ICIs with angiogenin inhibitors and chemotherapy might overcome the problems that NSCLC patients with EGFR mutations may encounter [55]. The FDA has approved the combination of PD-L1 inhibitors with BRAF-MEK inhibitors for advanced-stage melanoma with BRAFV600 [56]. Moreover, the addition of PD-L1 inhibitors with T-DM1 is favorable among patients with HER2-positive, PD-L1-positive advanced BC [57]. It also has clinically useful results when PD-1/PD-L1 inhibitors and PARP inhibitors are administered to breast cancer patients with germline BRCA1/2 mutations and ovarian cancer (OC) [58].
There is an understanding of TMB and PD-L1 as predictive markers of ICI response in many papers. TMB is associated with better clinical results and lasting clinical benefits derived from PD-1/PD-L1 agents [59]. Consequently, these discoveries suggest that TMB and PD-L1 expression should complement the choice of predictive models to improve patient selection in routine care. The relevance for trials such as KEYNOTE-649 is significant since it sets out steps for selecting the patients who are most likely to derive advantages from these advanced treatment methods. Additionally, the combination of anti-PD-1/PD-L1 inhibitors and ibrutinib also suggests another direction for the intensification of antitumor immune reactions (source not provided). These combinations might enhance outcomes in various cancers because the mechanisms of PD-1/PD-L1 blockade are also in agreement. Emerging data suggest that further study of the factors and pathways affecting resistance mechanisms that might impair antigen presentation by HLA class I molecules will limit the efficacy of ICIs [60]. However, many unknowns remain in this area of study, including issues related to working against resistance and selecting the right patient reward profiles for different ICI treatments. Future work should focus on a better understanding of factors dependent on tumor characteristics and the immune milieu and their impact on treatment outcomes. This involves examining the use of biomarkers in prognostication and treatment planning for the neoadjuvant treatment of cancer types such as triple-negative breast cancer plus the identification of biomarkers that may be used to predict combination therapy reactions [61].
The use of anti-CTLA-4 agents, such as ipilimumab, is a major innovation in cancer immunotherapy and is focused mainly on reversing the immune suppression that tumors promote. Blockade of CTLA-4, a coinhibitory receptor that negatively controls T-cell activation, improves antitumor T-cell activity, which is essential for obtaining lasting effects in various types of tumors [62]. In addition, it helps the immune system identify tumor cells and target them; moreover, it highlights the immunological setting of cancer therapy. Extending their scope, researchers have also discussed the impact of gut microbiomes on CTLA-4 inhibitors. For example, a particular class of Bacteroides has been shown to enhance antitumor effects; therefore, the authors contend that the microbiota heavily influences immune reactions during CTLA-4 blockade [63]. This discovery may open up avenues for microbiome-targeted treatments that would help make CTLA-4 inhibitors more effective while ushering in a new era of individual immunotherapy tailored to a patient's microbiome.
Furthermore, the effectiveness of CTLA-4 inhibitors is strongly associated with mutations in tumor-related genes. Thus, a greater mutational burden has been shown to be associated with positive outcomes after treatment with CTLA-4 blockade; thus, comprehensive molecular profiling of tumors is critical for understanding the mechanisms underlying patients' responses [64]. Figure 4: Illustration of the nature of the action of CTLA-4 inhibitors. Tumor cells express B7-1/B7-2, which bind to CTLA-4 on T cells, blocking their ability to kill tumor cells (left). By inhibiting the effective interaction of B7-1/B7-2 with a CTLA-4 inhibitor, T cells can no longer bind to free CTLA-4 receptors, enabling them to kill tumor cells (right). CTLA-4 = cytotoxic T-lymphocyte–associated antigen 4.
However, similar to other immunotherapy strategies, CTLA-4 inhibitors cannot be completely free from immune-related toxicity; for example, colitis can be potentially lethal. The timing and type of these side effects need to be understood so that patient safety can be addressed while achieving optimum therapeutic gain [65]. Special emphasis should be placed on assessing the first signs of toxicity in patients treated with combination therapies, including CTLA-4 blockade, as such combinations may increase toxicity. Another layer of complexity is the tumor microenvironment because factors such as myeloid-derived suppressor cells can also represent a target for overcoming resistance related to CTLA-4 therapies [66]. This resistance highlights the fact that a combined-state approach should be used to target both CTLA-4 and MDSCs, which may improve therapeutic efficacy and the antitumor immune response. Recent improvements in cancer immunotherapy using CTLA-4 inhibitors have led to the FDA approval of many monoclonal antibodies for the inhibition of CTLA-4, but issues concerning patient response remain. An urgent need has been reached for biomarkers that would help in predicting the therapeutic outcomes of these types of treatment, highlighting that the immune response to CTLA-4 blockade is nonlinear and that there is therefore a need for more research on probable factors that determine the immune response to therapies [67]. The use of CTLA-4 and PD-1 inhibitors has also received attention because these agents may have synergistic effects. Moreover, while CTLA-4 blockade promotes T-cell activation and proliferation, the add-on effect of PD-1 blockade may intensify antitumor immunity; therefore, combining these targets would result in improved immune effector function against tumors [67].
Pembrolizumab and nivolumab, PD-1 inhibitors, are at the forefront of immunotherapy for cancer and NSCLC. These agents, which are grouped according to their mechanism-label, exert effectiveness on the basis of genomic features, especially TMB. A positive correlation between TMB and the response to PD-1 blockade has been reported by Rizvi et al. (2015), who reported that the greater the number of mutations, the more responsive the tumor is likely to be to the inhibitor. This work entailed the application of genomic markers in the development of effective slug management plans for the treatment of oncology patients with reference to the peculiarities of the tumor. Moreover, the neoantigen and T-cell responses detected in PD-1-treated patients suggest that these inhibitors act not only on tumor cells but also on the immune system to eliminate cancer [68].
The findings of genomic determination are further supported in subsequent studies and depict the molecular smoking index as well as DNA repair pathway mutations as important factors that predict the efficacy of PD-1 inhibitors (Rizvi et al., 2015). This finding supports the view that an understanding of a patient's polygenic profile is necessary when administering PD-1 therapy in the clinical setting [68]. In addition to genomic inputs, the gut microbiome has been identified as a key area of interest in terms of PD-1 inhibitors. Routy et al. (2018) explained how, through the destruction of immune regulatory microbiota in the gut, the use of antibiotics is capable of worsening treatment results. Some bacterial species, such as Akkermansia muciniphila, are known to be strongly correlated with PD-1 therapy outcome. Thus, these results suggest that a healthy gut microbiome may be necessary to achieve optimal results from PD-1 inhibitors and hence opens a new direction for influencing treatment responses via microbiota modulation [69].
The action of PD-1 inhibitors continues to be discussed in the literature, with an emphasis on the functions of PD-1 and CTLA-4 as immune checkpoints according to Buchbinder and Desai (2016). This blockade can strongly promote specific antitumor immunity, which represents a major shift in cancer therapy strategies. The fact that the early and late phases of immune response regulation are distinct and modulated by CTLA-4 and PD-1 offers important lessons in harnessing these agents for immunotherapies and combinations that yield maximum benefit to patients [70]. However, only a few questions arise related to the restrictions on the use of PD-1 therapy and the problems with its effectiveness. Some aspects have been found to be linked to hyperprogressive disease (HPD) related to PD-1 inhibitors, and important questions arise for choosing patients and screening analysis during treatment. HPD is proof of the need for further investigation and the constant development of effective treatment methods as well as the prevention of essential adverse effects responsible for the decrease in therapeutic outcomes [71]. However, knowledge of the safety profiles of PD-1 inhibitors is crucial for patient management. The side effects of these therapies may differ, meaning that the treatment plans used in their management and treatment should be made to fit everyone. A systematic review of adverse events focused on comparisons of the therapeutic effectiveness of PD-1 inhibitors with possible side effects [71].
PD-L1 inhibitors are the major subtype of ICPs that significantly contribute to cancer immunotherapy, especially through immune checkpoint blockade, which is targeted at improving recognition of tumor cells by the immune system. The exact mode of action of these inhibitors, including both anti-PD-1 and anti-PD-L1 antibodies, is related to the regulation of the relationship between immune and cancer cells toward reversal of the immunosuppressive effects elicited by tumors. In this context, clinical trials have established that although these inhibitors induce substantial antitumor outcomes, they are usually associated with subtype specificity. This could be because PD-L1 is expressed in a variable way within the tumoral microenvironment, implying that there is much to learn about the actual role of PD-L1 in immune escape [71]. Subsequent analyses focused on the role of predictive biomarkers such as the TMB and the extent of PD-L1 expression in defining patient outcomes in response to PD-L1 inhibitors, especially in advanced NSCLC. The TMB increases, and the clinical benefit increases; therefore, the TMB should be added to PD-L1-based models for selecting patients who will benefit from immunotherapy [59]. The requirement for such case-based approaches is further compounded by a newly identified worst-case scenario called hyperprogressive disease (HPD), where some patients experience an increased rate of tumor growth after receiving PD-L1 inhibitors. This raises the need for proper selection of patients, especially elderly patients, for this treatment and close follow-up of any patient's condition [72].
Figure 5 shows the types of immune-limiting proteins: PD-L1, which is present on tumor cells, and PD-L1, which is present on T cells. PD-L1 forms a complex with PD-1, which inhibits the ability of T cells to kill tumor cells within the body (left panel). When this interaction is impaired by immune checkpoint inhibitors (anti-PD-L1 or anti-PD-L1), T cells can once again kill tumor cells.
Furthermore, concerns exist regarding the safety of PD-L1 inhibitors for their therapeutic use in the clinic. Compared with other PD-L1 inhibitors, systematic reviews have revealed that there is inconsistency in the distributions of treatment-related AEs; thus, there is a need to conduct safety analyses continually to assist clinicians in choosing suitable drugs [73]. These results indicate that most PD-L1 inhibitors have favorable risk profiles for major types of adverse effects; thus, more studies related to individual cancers and agents associated with efficacy and safety are needed. Subsequent meta-analysis studies have also supported the use of PD-L1 inhibitors irrespective of the PD-L1 expression level, which contradicts the prior belief that PD-L1 levels should be the basis for treatment choice. The body of knowledge indicates that these therapies could offer significant survival gains; therefore, there should be increased utilization in practice not based on this biomarker [74]. This inevitably raises questions about the interaction of PD-L1 inhibitors with other therapeutic schemes, including combination treatment regimens that can potentially improve overall outcomes. In our view, one exciting area of investigation concerns the use of PD-L1 inhibitors in conjunction with other modalities of treatment, such as IL-6 blockade, especially in difficult-to-treat cancers, including PDAC. Previous research has shown that such combinations may enhance antitumor responses; therefore, the optimization of multiple modality treatment approaches may augment the efficacy features of PD-L1 inhibitors [75].
Conventional cancer treatment primarily encompasses three main modalities: Surgery, chemotherapy, and radiation therapy. These approaches have been standard for oncology therapy for many years and are typically used concurrently to improve effectiveness against tumors [76]. Surgery is often the initial approach, assuming that the tumors are localized and that they are mostly initially located. Surgery for cancer aims at eradicating all the tumor mass while also taking a thin margin of healthy tissues to remove all cancer cells. Surgery is especially beneficial in stage I cancers where tumor removal is likely to remove the disease completely [77]. However, while chemotherapy relies on agents that are toxic to cancerous tissues and cells, the drugs used in chemotherapy are those that selectively target and destroy rapidly dividing cells. This treatment can be performed orally or through injection and is performed either as a primary treatment or an additional treatment after surgery to eradicate cancer cells not captured by surgery [78].
However, chemotherapy is effective in reducing the size of a tumor and increasing the prognosis and survival time and it often has complications that affect normal cells in the body, such as nausea, fatigue and infections. In addition, challenges associated with treatment, such as drug resistance, can be quite definitive [79],[80]. Radiation therapy uses ionizing energy, in the form of rays or particles, including X-rays, to destroy cancer cells. Some types of cancer are confined, and the rays can be accurately aimed at the affected area. Radiation can be used as an initial modality in combination with surgery and/or chemotherapy. However, like chemotherapy, it can also impact other parts of the body and healthy cells, which can cause various side effects [81],[82]. Figure 6 shows an overview of conventional and recent advancements in cancer treatment methods, highlighting surgery, chemotherapy, radiation therapy, and immunotherapy as key approaches in combating cancer. Over the past few years, there has been an increased focus on combinations of focused therapies along with immunotherapies along with conventional treatment plans. These modern methods attempt to pinpoint cancer cells or improve the body's ability to fight tumors, thereby causing less damage to healthy cells than conventional treatments do. However, conventional therapies continue to be important parts of cancer management because they form a solid foundation on which more recent approaches are based [83].
The invention of vaccines has given a vast chance for the eradication or control of infectious diseases; the first known vaccine was created by Edward Jenner in 1796 on the basis of cowpox and protection against smallpox. In time, vaccines have been used to treat other illnesses, including cancer [84]. The first type of cancer vaccine, which is based on tumor cells and lysates, was developed in 1980 and targeted colorectal cancer via autologous tumor cells. The turning point was the discovery of a new melanoma-associated antigen in the early 1990s, which focused on the development of tumor antigens for vaccines [85]. A major breakthrough was achieved in 2010 when a Sipuleucel-T dendritic cell-based cancer vaccine was successfully used to manage prostate cancer, and there is hope in the field of cancer vaccines. The current COVID-19 vaccination has also advanced great progress in the development of novel cancer vaccines [86]. These vaccines mainly use the concepts of tumor-associated antigens (TAAs) and tumor-specific antigens (TSAs) to work on the immune system and consider both cellular immunity and humoral immunity to prevent tumor formation and eliminate cancer cells. This approach is mostly used for cancer vaccines, which are at the preclinical and clinical levels and therefore require further definitions of more specific antigens and more effective development platforms [87]. Cancer vaccines differ from conventional vaccines in that their major purpose is to elicit selective immune reactions against cancer cells without the need to target usually foreign organisms. There are only two virus-associated preventive cancer vaccines approved by the FDA: The hepatitis B vaccine and the HPV vaccine [88]. In contrast to most typical immunogens where the antigens are exogenous, tumor antigens are endogenous and thus usually weak self-antigens that present low immunogenicity, thereby complicating efforts to elicit immune responses. All these vaccines, particularly cancer vaccines, mainly elicit affinity for CD8+ cytotoxic T lymphocytes and not the more familiar antibodies that are evoked by traditional vaccines [89].
The adaptation of the current approaches and effectiveness of cancer vaccines involves increasing immune system recognition of cancer. New developments have led to the development of approaches that can help eradicate prevailing difficulties, especially those of the tumor stroma and the attributes of cancer stem cells.
Cancer vaccines work on a very strategic basis on the premise of eliciting an immune response against tumor cells using tumor-associated antigens (TAAs) or tumor-specific antigens (TSAs). These antigens are first transported to antigen-presenting cells (APCs), such as dendritic cells, via peptides, proteins, or whole tumor cells. After the APCs capture the antigens, they break them down into fragments and display them on their surface via the MHC [90]. This presentation is important for T-cell activation because T cells express their TCRs on their surface to identify MHC-antigen overload. Illustrated above are the interactions between T cells and APCCs for the effective activation of T cells; T cells need to recognize the MHC-antigen complex and receive costimulatory signals [91]. Once fully activated, CD8+ cytotoxic T lymphocytes (CTLs) are prepared to recognize and kill cancer cells harboring the same antigens [92]. Similarly, helper CD4+ T cells efficiently induce a stronger antitumor immune response via the stimulation of cytokines that support the growth and differentiation of CTLs and assist B cells in producing antibodies against tumor antigens [92]. To improve this response, numerous cancer vaccines contain adjuvants—molecules that can increase the immunoresponse by engaging additional APCs and by increasing costimulative signals. For example, certain vaccines organize Toll-like receptor (TLR) agonists to stimulate innate immune signaling and overproduction of proinflammatory cytokines, which reinforce adaptive immunity [93]. However, the immunosuppressive tumor microenvironment (TME) might obstruct cancer vaccines; this may include situations where regulatory T cells and myeloid-derived suppressor cells recapitulate T cells [94]. To overcome these challenges, scientists are considering the use of cancer vaccines together with immune checkpoint inhibitors, which are molecules that block negative signals on T cells (PD-1/PD-L1 or CTLA-4) [95]. Some of these new advancements in PM have also been spurred by the development of neoantigen vaccines that are designed and developed for a particular tumor type identified in a patient to provoke a strong immune response and subsequently high clinical effectiveness [96]. In aggregate, cancer vaccines are designed to produce enduring antitumor immunity that can manage or eradicate tumors over a period of time, which demonstrates a shift in the immunotherapy of cancer [97]. Figure 7 shows that cancer vaccines utilize antigens and adjuvants to activate the immune system, teaching it to identify and eliminate harmful cells.
Circulating biomarkers are vital in oncology, mainly for the enrichment in patient selection and treatment strategies [98]. These are quantitative expressions of biological states or conditions, which may be present in blood or tissue and can provide useful information on cancer in an individual. Two of the prominent biomarkers identified are PD-L1 and MSI, the latter of which have become highly important predictors for guiding therapies for several cancers, such as stomach cancers [99].
Another typical biomarker is PD-L1 expression, and the value of such biomarkers relies on the use of such characteristics of the tumor to guide clinicians. PD-L1 is a protein that can be overexpressed on the surface of tumor cells, indicating how cancer cells may communicate with the immune system [100]. When PD-L1 proteins are overexpressed, immunostimulatory drugs, generally known as checkpoint inhibitors, are used to enhance immunity against such tumors [101]. For example, the sensitivity of the antitumor immune response in stomach cancer patients with high PD-L1 expression may indicate better results from therapies such as pembrolizumab or nivolumab, which target the PD-1/PD-L1 pathway. This understanding enables oncologists to customize treatment regimens according to the chances of response to immunotherapy and hence enhances the quality of their results while reducing adverse effects from other unproductive therapies [102].
Second, the MSI status is another important prognostic biomarker for patient selection during treatment. MSI refers to the Mutator phenotype associated with defects in the DNA mismatch repair system, which is a state of genetic hypermutability [103]. Cancers that are classified as MSI-high for their high level of MSI are predicted to undergo better immunotherapy because of the high mutation loads, which create neoantigens capable of triggering immune activity. In stomach cancer, the MSI status can be used to inform treatment directions that prioritize immunotherapy as more effective than usual chemotherapy. For example, MSI-high tumors should be treated with pembrolizumab or other checkpoint inhibitors, but MSS tumors should be treated differently [104].
A biomarker test can be performed on different tumor samples through different techniques, including immunohistochemistry, next-generation sequencing, or polymerase chain reaction, among others. These tests can be used to identify targetable mutations or protein expression that can inform therapy [105]. For example, having a biomarker of HER2 amplification following surgery for stomach cancer may mean that this patient may require treatment, such as trastuzumab, which completely changes the treatment options for this patient [106]. In addition, biomarkers also help in choosing the right treatment but also in anticipating the patient's response and assessing the impact of the treatment. With the help of biomarkers that include PD-L1 and MSI status, clinicians have learned how well a particular patient is likely to respond to the treatments recommended [107]. This is a promising feature, as it provides the opportunity to modify the therapy via real-time evaluations of treatment efficacy. The development of biomarker research has also given rise to worldwide tumor-agnostic treatment—medical treatments for various cancer types on the basis of molecular markers regardless of their origin [108]. This change in thinking focuses on biomarkers over traditional dichotomies and offers new possibilities for patients with fewer choices previously. For example, drugs developed to act on certain mutations in BRAF or NTRK can be used in many forms of cancer if these mutations exist in the cancer's origin [109]. However, several issues related to the practical application of biomarker testing in clinical practice remain. Contamination and novel testing suggest that oncologists should be not only well informed of new biomarkers but also that tests are relevant to treatment protocols. Finally, further differences between primary tumors and metastases with respect to biomarkers exist, which can make therapy planning more challenging; for this reason, rebiopsies may sometimes be required for proper evaluation [110].
Table 2 presents various biomarkers relevant to stomach cancer, which are categorized into four main groups. Blood and protein biomarkers include classical tumor markers, circulating biomarkers, genetic and epicongenic biomarkers, and other novel biomarkers. Every row provides information about biomarkers, their usage in the clinic, and their impact on stomach cancer.
Biomarker Type | Biomarker | Clinical Application | Action on Stomach Cancer | Ref |
Classical Tumor Markers | CEA | Diagnosis, monitoring, and predicting recurrence | Higher cancer levels suggest higher stages and possibility of liver metastasis | [111],[112] |
CA19-9 | Diagnosis and monitoring | Linked to other clinicopathological characteristics and employed for predicting relapse | [113],[114] | |
CA72-4 | Follow-up after treatment | Good specificity for gastric cancer; used to identify relapses | [113],[115] | |
Alpha-fetoprotein (AFP) | Diagnosis and prognosis | Indicates aggressive disease and higher metastatic potential | [116],[117] | |
Circulating Biomarkers | Circulating free miRNAs | Early diagnosis and prognosis | Several miRNAs observed in the study are potential players in clinical prognosis: total survival, lymph node metastasis | [118],[119] |
Circulating tumor cells | Monitoring treatment response | Noninvasive method to assess tumor dynamics in real-time | [120] | |
Exosomal lncRNAs | Diagnostic and prognostic indicators | Some lncRNAs could express greatly significant difference in cancer tissues compared with that in healthy individuals | [121],[122] | |
Genetic and Epigenetic Markers | Microsatellite Instability (MSI) | Predictive for immunotherapy response | High mutational load correlates with better survival outcomes | [123],[124] |
EBV status | Prognostic indicator | Connected with a particular type of gastric cancer that defines the therapy approaches | [125],[126] | |
Emerging Biomarkers | Long noncoding RNAs (lncRNAs) | Early detection and prognosis | These lncRNAs have potential for use as stable diagnostic indicators of gastric cancer | [127] |
Circular RNAs (circRNAs) | Diagnostic potential | Some of the circRNA panels have been shown to distinguish gastric cancer tissues from normal ones | [128] |
Findings suggest that Helicobacter pylori infection may be beneficial for gastric cancer immunotherapy, although findings have been mixed across studies. The relationship between H. pylori infection and immunotherapy is complex, with some studies having shown that this enhances the efficacy of drugs like PD-1/PD-L1 therapy by modifying the microenvironment of the tumor [129],[130]. However, other researchers have found that H. pylori infection can negatively impact the outcomes of immunotherapy, particularly in terms of survival rates [131],[132].
The presence of H. pylori can cause chronic inflammation, which may influence immune responses in a way that would promote immunotherapeutic interventions in certain situations. Incorporation of further elaboration of H. pylori infection in scientific journals may be of utmost importance to explain its role in cancer treatment. The role of H. pylori in gut microbiota and different tumor molecular subtypes is equally crucial in assessing the effectiveness of immunotherapy [130],[132]. Further research is needed to fully understand how H. pylori infection influences immunotherapy outcomes across cancer types. The study highlights the importance of testing for H. pylori infection in cancer patients undergoing immunotherapy to tailor treatment strategies effectively. Overall, the interaction between H. pylori and immunotherapy offers promising avenues for improving cancer treatment outcomes. More comprehensive analysis of H. pylori's effects could lead to better personalized treatment approaches for gastric cancer patients.
We identified several limitations in immunotherapy for gastric cancer; however, one of the greatest concerns is the heterogeneity of patient responses. Such variability could be due to genetic factors, tumor-related factors, or the tumor microenvironment. For example, van der Laak et al. reported that the TMB can vary among individual patients and is generally predictive of the response to ICIs [133]. It has been assumed that high TMB is associated with better outcomes because the likelihood of neo-antigen generation is high; however, the relationship is not as direct. Research has revealed that the proportion of high ITH in tumor samples implies that even a high TMB can lead to a poor response because other factors, such as ITH and immune-suppressive cells, can exist in the TME [134].
Furthermore, molecules, including programmed death-1 (PD-1) or cytotoxic T-lymphocyte-associated protein 4 (CTLA-4), can regulate immune responses. These molecules can be upregulated or downregulated and can have quite different levels between patients and, sometimes, between different parts of the same tumor [135]. This variability also threatens the ability to predict which patients will benefit from ICIs. Moreover, the identification of definite molecular profiles that can be used accurately to predict responses to treatments remains a challenge [136]. Although many companies and scientists have been looking for such predictive biomarkers for years, none have been validated as ready-to-use means of choosing appropriate participants in immunotherapy; as a result, many patients receive immunotherapy that is suitable only partially or even not suitable at all [137].
The second fundamental challenge involves aspects of immune escape strategies deployed by tumors. Tumor cells have developed mechanisms by which they can avoid being recognized and killed by the immune system. The gastric cancer TME not only progresses over time but also changes in tumor immunosuppressive properties and resistance to therapy [138].
Tumor heterogeneity exacerbates this scenario. Preclinical data highlight that gastric tumors are highly diverse within a tumor mass with genetic and immune cell subpopulations. This is why some tumor regions may have more immune cells and vice versa, as well as why there may be different reactions to a particular treatment [139]. For example, while some subclones that arise from the same tumor mass may produce immune checkpoint ligands at high density, others may produce few to no immune ligands, leaving an immune presence in the tumor piecemeal. Furthermore, the process of clonal evolution can lead to outcome-determining clones with a genetically ingrained capacity for immune escape and therefore constitute a genuine threat to immunotherapeutic efficacy.
The interactions between ITH and TMB are also determinants of treatment outcomes. Although a high TMB implies that more likely neo-antigens are produced that could be targeted by an immune response, heterogeneous cancer subclones may be represented by clones dominating tumors that are poorly recognized by the immune system due to various mechanisms, including the loss of antigen-processing machinery or the downregulation of neoantigens. This complexity means that it is necessary to gain deeper insight into how these factors jointly and severally impinge on patients [140].
Another relevant factor in immunotherapy is immune-related adverse events or irAEs. As immunotherapies can inspire vigorous reactions against tumors, they can also react to normal tissues with broad-range dangerous outcomes ranging from mild manifestations to life-threatening conditions [141]. The common organ-specific irAEs are dermatological reactions, colitis, hepatitis, and endocrinopathies. These events may occur randomly and with varying severity in some patients, depending, inter alia, on genetic factors and the presence of other diseases. The management of irAEs is crucial to avoid hazardous conditions for the patient while offering the highest chance of benefit from treatment. The perceived consequences of immunotherapeutic agents commonly act as barriers to early recognition and intervention; however, increased knowledge concerning the side effects of such agents is vital. Furthermore, the ability to achieve a good cancer outcome while avoiding or at least limiting irAEs presents a unique challenge to oncologists. At per protocol doses, some severe adverse events may require dose modification or temporary withdrawal of the therapy, but this does not necessarily imply the abandonment of the protocol treatment [142].
Gastric cancer remains a formidable global health challenge, demanding innovative therapeutic strategies to improve patient outcomes. In this review, we explored the landscape of immunotherapeutic approaches, including immune checkpoint inhibitors, CAR-T cell therapy, and therapeutic vaccines, highlighting their mechanisms and potential. The complex interplay within the tumor microenvironment, involving immune cells, fibroblasts, and the extracellular matrix, significantly influences therapeutic responses and necessitates a deeper understanding. Emerging trends in gastric cancer epidemiology, such as the rise of cardia gastric cancer and shifts in risk factor profiles, underscore the need for targeted prevention and personalized treatment strategies. While immunotherapy offers promising avenues for combating gastric cancer, challenges such as therapeutic resistance and the need for tailored approaches persist. Continued research focused on elucidating the intricacies of the tumor microenvironment, refining immunotherapeutic techniques, and addressing emerging epidemiological trends is crucial for advancing the field, improving the lives of gastric cancer patients.
The authors declare they have not used Artificial Intelligence (AI) tools in the creation of this article.
[1] | Mukkamalla SKR, Recio-Boiles A, Babiker HM (2024) Gastric Cancer. Florida: StatPearls Publishing. Available from: http://www.ncbi.nlm.nih.gov/books/NBK459142/ |
[2] |
De Martel C, Forman D, Plummer M (2013) Gastric cancer. Gastroenterol Clin North Am 42: 219-240. https://doi.org/10.1016/j.gtc.2013.01.003 ![]() |
[3] |
Karimi P, Islami F, Anandasabapathy S, et al. (2014) Gastric cancer: Descriptive epidemiology, risk factors, screening, and prevention. Cancer Epidemiol Biomarkers Prev 23: 700-713. https://doi.org/10.1158/1055-9965.EPI-13-1057 ![]() |
[4] |
Rawla P, Barsouk A (2019) Epidemiology of gastric cancer: Global trends, risk factors and prevention. Gastroenterol Rev 14: 26-38. https://doi.org/10.5114/pg.2018.80001 ![]() |
[5] |
Sitarz R, Skierucha M, Mielko J, et al. (2018) Gastric cancer: Epidemiology, prevention, classification, and treatment. Cancer Manage Res 10: 239-248. https://doi.org/10.2147/CMAR.S149619 ![]() |
[6] |
Morgan E, Arnold M, Camargo MC, et al. (2022) The current and future incidence and mortality of gastric cancer in 185 countries, 2020–40: A population-based modelling study. EClinicalMedicine 47: 101404. https://doi.org/10.1016/j.eclinm.2022.101404 ![]() |
[7] |
Wong MCS, Huang J, Chan PSF, et al. (2021) Global Incidence and mortality of gastric cancer, 1980–2018. JAMA Network Open 4: e2118457. https://doi.org/10.1001/jamanetworkopen.2021.18457 ![]() |
[8] | Hua MJ Staging efficacy: Cancer treatment in contemporary China (2020). https://doi.org/10.6082/uchicago.2753 |
[9] |
Lin JL, Lin JX, Lin GT, et al. (2024) Global incidence and mortality trends of gastric cancer and predicted mortality of gastric cancer by 2035. BMC Public Health 24: 1763. https://doi.org/10.1186/s12889-024-19104-6 ![]() |
[10] |
Miller KD, Nogueira L, Mariotto AB, et al. (2019) Cancer treatment and survivorship statistics, 2019. CA A Cancer J Clinicians 69: 363-385. https://doi.org/10.3322/caac.21565 ![]() |
[11] |
Miller KD, Nogueira L, Devasia T, et al. (2022) Cancer treatment and survivorship statistics, 2022. Ca-Cancer J Clin 72: 409-436. https://doi.org/10.3322/caac.21731 ![]() |
[12] |
Malik A, Ali F, Malik MI, et al. (2024) The risk of infection-caused mortality in gastric adenocarcinoma: A population-based study. Gastroenterol Res 17: 133-145. https://doi.org/10.14740/gr.v17i3.1715 ![]() |
[13] |
Assumpção PP, Barra WF, Ishak G, et al. (2020) The diffuse-type gastric cancer epidemiology enigma. BMC Gastroenterol 20: 223. https://doi.org/10.1186/s12876-020-01354-4 ![]() |
[14] |
Iyer P, Moslim M, Farma JM, et al. (2020) Diffuse gastric cancer: Histologic, molecular, and genetic basis of disease. Transl Gastroenterol Hepatol 5: 52. https://doi.org/10.21037/tgh.2020.01.02 ![]() |
[15] |
Mabula JB, Mchembe MD, Koy M, et al. (2012) Gastric cancer at a university teaching hospital in northwestern Tanzania: A retrospective review of 232 cases. World J Surg Oncol 10: 257. https://doi.org/10.1186/1477-7819-10-257 ![]() |
[16] |
Iwu CD, Iwu-Jaja CJ (2023) Gastric cancer epidemiology: Current trend and future direction. Hygiene 3: 256-268. https://doi.org/10.3390/hygiene3030019 ![]() |
[17] |
Rugge M, Fassan M, Graham DY (2015) Epidemiology of gastric cancer. Gastric Cancer. Cham: Springer International Publishing 23-34. https://doi.org/10.1007/978-3-319-15826-6_2 ![]() |
[18] |
Ferro A, Peleteiro B, Malvezzi M, et al. (2014) Worldwide trends in gastric cancer mortality (1980–2011), with predictions to 2015, and incidence by subtype. Eur J Cancer 50: 1330-1344. https://doi.org/10.1016/j.ejca.2014.01.029 ![]() |
[19] |
Machlowska J, Baj J, Sitarz M, et al. (2020) Gastric cancer: Epidemiology, risk factors, classification, genomic characteristics and treatment strategies. Int J Mol Sci 21: 4012. https://doi.org/10.3390/ijms21114012 ![]() |
[20] |
Hu Y, Huang C, Sun Y, et al. (2016) Morbidity and mortality of laparoscopic versus open D2 distal gastrectomy for advanced gastric cancer: A randomized controlled trial. J Clin Oncol 34: 1350-1357. https://doi.org/10.1200/JCO.2015.63.7215 ![]() |
[21] |
Naran K, Nundalall T, Chetty S, et al. (2018) Principles of immunotherapy: Implications for treatment strategies in cancer and infectious diseases. Front Microbiol 9: 3158. https://doi.org/10.3389/fmicb.2018.03158 ![]() |
[22] |
Hu H, Chen Y, Tan S, et al. (2022) The research progress of antiangiogenic therapy, immune therapy and tumor microenvironment. Front Immunol 13: 802846. https://doi.org/10.3389/fimmu.2022.802846 ![]() |
[23] |
Bilotta MT, Antignani A, Fitzgerald DJ (2022) Managing the TME to improve the efficacy of cancer therapy. Front Immunol 13: 954992. https://doi.org/10.3389/fimmu.2022.954992 ![]() |
[24] |
Samstein RM, Lee CH, Shoushtari AN, et al. (2019) Tumor mutational load predicts survival after immunotherapy across multiple cancer types. Nat Genet 51: 202-206. https://doi.org/10.1038/s41588-018-0312-8 ![]() |
[25] |
Ru B, Wong CN, Tong Y, et al. (2019) TISIDB: An integrated repository portal for tumor–immune system interactions. Bioinformatics 35: 4200-4202. https://doi.org/10.1093/bioinformatics/btz210 ![]() |
[26] |
Bagchi S, Yuan R, Engleman EG (2021) Immune checkpoint inhibitors for the treatment of cancer: Clinical impact and mechanisms of response and resistance. Annu Rev Pathol Mech Dis 16: 223-249. https://doi.org/10.1146/annurev-pathol-042020-042741 ![]() |
[27] |
Labani-Motlagh A, Ashja-Mahdavi M, Loskog A (2020) The tumor microenvironment: A milieu hindering and obstructing antitumor immune responses. Front Immunol 11: 940. https://doi.org/10.3389/fimmu.2020.00940 ![]() |
[28] |
Zhu Y, Knolhoff BL, Meyer MA, et al. (2014) CSF1/CSF1R blockade reprograms tumor-infiltrating macrophages and improves response to T-cell checkpoint immunotherapy in pancreatic cancer models. Cancer Res 74: 5057-5069. https://doi.org/10.1158/0008-5472.CAN-13-3723 ![]() |
[29] |
Henke E, Nandigama R, Ergün S (2020) Extracellular matrix in the tumor microenvironment and its impact on cancer therapy. Front Mol Biosci 6: 160. https://doi.org/10.3389/fmolb.2019.00160 ![]() |
[30] |
Mager LF, Burkhard R, Pett N, et al. (2020) Microbiome-derived inosine modulates response to checkpoint inhibitor immunotherapy. Science 369: 1481-1489. https://doi.org/10.1126/science.abc3421 ![]() |
[31] |
Kon E, Benhar I (2019) Immune checkpoint inhibitor combinations: Current efforts and important aspects for success. Drug Resist Updates 45: 13-29. https://doi.org/10.1016/j.drup.2019.07.004 ![]() |
[32] |
Sobhani N, Tardiel-Cyril DR, Davtyan A, et al. (2021) CTLA-4 in regulatory T cells for cancer immunotherapy. Cancers 13: 1440. https://doi.org/10.3390/cancers13061440 ![]() |
[33] |
Tang Q, Chen Y, Li X, et al. (2022) The role of PD-1/PD-L1 and application of immune-checkpoint inhibitors in human cancers. Front Immunol 13: 964442. https://doi.org/10.3389/fimmu.2022.964442 ![]() |
[34] | Colligan SH Mitigating myeloid-driven pathways of immune suppression to enhance cancer immunotherapy efficacy (2022). |
[35] |
Guzik K, Tomala M, Muszak D, et al. (2019) Development of the inhibitors that target the PD-1/PD-L1 interaction—a brief look at progress on small molecules, peptides and macrocycles. Molecules 24: 2071. https://doi.org/10.3390/molecules24112071 ![]() |
[36] |
Graziani G, Lisi L, Tentori L, et al. (2022) Monoclonal antibodies to CTLA-4 with focus on ipilimumab. Interaction of Immune and Cancer Cells. Cham: Springer International Publishing 295-350. https://doi.org/10.1007/978-3-030-91311-3_10 ![]() |
[37] |
Lipson EJ, Drake CG (2011) Ipilimumab: An anti-CTLA-4 antibody for metastatic melanoma. Clin Cancer Res 17: 6958-6962. https://doi.org/10.1158/1078-0432.CCR-11-1595 ![]() |
[38] |
Rajan A, Kim C, Heery CR, et al. (2016) Nivolumab, anti-programmed death-1 (PD-1) monoclonal antibody immunotherapy: Role in advanced cancers. Hum Vaccines Immunother 12: 2219-2231. https://doi.org/10.1080/21645515.2016.1175694 ![]() |
[39] |
Massard C, Gordon MS, Sharma S, et al. (2016) Safety and efficacy of durvalumab (MEDI4736), an anti–programmed cell death Ligand-1 immune checkpoint inhibitor, in patients with advanced urothelial bladder cancer. J Clin Oncol 34: 3119-3125. https://doi.org/10.1200/JCO.2016.67.9761 ![]() |
[40] |
Peters S, Kerr KM, Stahel R (2018) PD-1 blockade in advanced NSCLC: A focus on pembrolizumab. Cancer Treat Rev 62: 39-49. https://doi.org/10.1016/j.ctrv.2017.10.002 ![]() |
[41] |
Inman BA, Longo TA, Ramalingam S, et al. (2017) Atezolizumab: A PD-L1–blocking antibody for bladder cancer. Clin Cancer Res 23: 1886-1890. https://doi.org/10.1158/1078-0432.CCR-16-1417 ![]() |
[42] |
Teets A, Pham L, Tran EL, et al. (2018) Avelumab: A novel anti-PD-L1 agent in the treatment of merkel cell carcinoma and urothelial cell carcinoma. Crit Rev Immunol 38: 159-206. https://doi.org/10.1615/CritRevImmunol.2018025204 ![]() |
[43] | Rath B, Plangger A, Hamilton G (2020) Non-small cell lung cancer-small cell lung cancer transformation as mechanism of resistance to tyrosine kinase inhibitors in lung cancer. Cancer Drug Res 3: 171. https://doi.org/10.20517/cdr.2019.85 |
[44] |
Villani A, Ocampo-Garza SS, Potestio L, et al. (2022) Cemiplimab for the treatment of advanced cutaneous squamous cell carcinoma. Expert Opin Drug Saf 21: 21-29. https://doi.org/10.1080/14740338.2022.1993819 ![]() |
[45] |
Mirza MR, Chase DM, Slomovitz BM, et al. (2023) Dostarlimab for primary advanced or recurrent endometrial cancer. N Engl J Me 388: 2145-2158. https://doi.org/10.1056/NEJMoa2216334 ![]() |
[46] |
Patel TH, Brewer JR, Fan J, et al. (2024) FDA approval summary: Tremelimumab in combination with durvalumab for the treatment of patients with unresectable hepatocellular carcinoma. Clin Cancer Res 30: 269-273. https://doi.org/10.1158/1078-0432.CCR-23-2124 ![]() |
[47] | Burtness B Toripalimab yields ‘Striking’ PFS improvement in nasopharyngeal carcinoma (2024). Available from: https://www.cancernetwork.com/view/toripalimab-yields-striking-pfs-improvement-in-nasopharyngeal-carcinoma |
[48] |
Rugo HS, Kabos P, Beck JT, et al. (2022) Abemaciclib in combination with pembrolizumab for HR+, HER2− metastatic breast cancer: Phase 1b study. npj Breast Cancer 8: 1-8. https://doi.org/10.1038/s41523-022-00482-2 ![]() |
[49] |
Deng Y, Huang M, Deng R, et al. (2024) Immune checkpoint inhibitor-related adrenal hypofunction and Psoriasisby induced by tislelizumab: A case report and review of literature. Medicine 103: e37562. https://doi.org/10.1097/MD.0000000000037562 ![]() |
[50] |
Reuss JE, Gosa L, Liu SV (2021) Antibody drug conjugates in lung cancer: State of the current therapeutic landscape and future developments. Clin Lung Cancer 22: 483-499. https://doi.org/10.1016/j.cllc.2021.07.011 ![]() |
[51] |
Brahmer JR, Tykodi SS, Chow LQM, et al. (2012) Safety and activity of anti–PD-L1 antibody in patients with advanced cancer. N Engl J Med 366: 2455-2465. https://doi.org/10.1056/NEJMoa1200694 ![]() |
[52] |
Dermani FK, Samadi P, Rahmani G, et al. (2019) PD-1/PD-L1 immune checkpoint: Potential target for cancer therapy. J Cell Physiol 234: 1313-1325. https://doi.org/10.1002/jcp.27172 ![]() |
[53] |
Lai X, Friedman A (2017) Combination therapy for melanoma with BRAF/MEK inhibitor and immune checkpoint inhibitor: A mathematical model. BMC Syst Biol 11: 70. https://doi.org/10.1186/s12918-017-0446-9 ![]() |
[54] |
Zhang Q, Shao B, Tong Z, et al. (2022) A phase Ib study of camrelizumab in combination with apatinib and fuzuloparib in patients with recurrent or metastatic triple-negative breast cancer. BMC Med 20: 321. https://doi.org/10.1186/s12916-022-02527-6 ![]() |
[55] |
Dazio G, Epistolio S, Frattini M, et al. (2022) Recent and future strategies to overcome resistance to targeted therapies and immunotherapies in metastatic colorectal cancer. J Clin Med 11: 7523. https://doi.org/10.3390/jcm11247523 ![]() |
[56] |
Liu Y, Zhang X, Wang G, et al. (2021) Triple combination therapy with PD-1/PD-L1, BRAF, and MEK inhibitor for stage III–IV melanoma: A systematic review and meta-analysis. Front Oncol 11: 693655. https://doi.org/10.3389/fonc.2021.693655 ![]() |
[57] |
Stein MK, Oluoha O, Patel K, et al. (2021) Precision medicine in oncology: A review of multi-tumor actionable molecular targets with an emphasis on non-small cell lung cancer. J Pers Med 11: 518. https://doi.org/10.3390/jpm11060518 ![]() |
[58] |
Musacchio L, Cicala CM, Camarda F, et al. (2022) Combining PARP inhibition and immune checkpoint blockade in ovarian cancer patients: A new perspective on the horizon?. ESMO Open 7: 100536. https://doi.org/10.1016/j.esmoop.2022.100536 ![]() |
[59] |
Rizvi H, Sanchez-Vega F, La K, et al. (2018) Molecular determinants of response to anti–programmed cell death (PD)-1 and anti–programmed death-Ligand 1 (PD-L1) blockade in patients with non–small-cell lung cancer profiled with targeted next-generation sequencing. J Clin Oncol 36: 633-641. https://doi.org/10.1200/JCO.2017.75.3384 ![]() |
[60] |
Gettinger S, Choi J, Hastings K, et al. (2017) Impaired HLA class I antigen processing and presentation as a mechanism of acquired resistance to immune checkpoint inhibitors in lung cancer. Cancer Disco 7: 1420-1435. https://doi.org/10.1158/2159-8290.CD-17-0593 ![]() |
[61] |
Sibaud V (2018) Dermatologic Reactions to immune checkpoint inhibitors: Skin toxicities and immunotherapy. Am J Clin Dermatol 19: 345-361. https://doi.org/10.1007/s40257-017-0336-3 ![]() |
[62] |
Ribas A, Wolchok JD (2018) Cancer immunotherapy using checkpoint blockade. Science 359: 1350-1355. https://doi.org/10.1126/science.aar4060 ![]() |
[63] |
Vétizou M, Pitt JM, Daillère R, et al. (2015) Anticancer immunotherapy by CTLA-4 blockade relies on the gut microbiota. Science 350: 1079-1084. https://doi.org/10.1126/science.aad1329 ![]() |
[64] |
Van Allen EM, Miao D, Schilling B, et al. (2015) Genomic correlates of response to CTLA-4 blockade in metastatic melanoma. Science 350: 207-211. https://doi.org/10.1126/science.aad0095 ![]() |
[65] |
Wang DY, Salem JE, Cohen JV, et al. (2018) Fatal toxic effects associated with immune checkpoint inhibitors: A systematic review and meta-analysis. JAMA Oncol 4: 1721. https://doi.org/10.1001/jamaoncol.2018.3923 ![]() |
[66] |
Rowshanravan B, Halliday N, Sansom DM (2018) CTLA-4: A moving target in immunotherapy. Blood 131: 58-67. https://doi.org/10.1182/blood-2017-06-741033 ![]() |
[67] |
Qin S, Xu L, Yi M, et al. (2019) Novel immune checkpoint targets: Moving beyond PD-1 and CTLA-4. Mol Cancer 18: 155. https://doi.org/10.1186/s12943-019-1091-2 ![]() |
[68] |
Rizvi NA, Hellmann MD, Snyder A, et al. (2015) Mutational landscape determines sensitivity to PD-1 blockade in non–small cell lung cancer. Science 348: 124-128. https://doi.org/10.1126/science.aaa1348 ![]() |
[69] |
Routy B, Le Chatelier E, Derosa L, et al. (2018) Gut microbiome influences efficacy of PD-1-based immunotherapy against epithelial tumors. Science 359: 91-97. https://doi.org/10.1126/science.aan3706 ![]() |
[70] |
Buchbinder EI, Desai A (2016) CTLA-4 and PD-1 pathways: Similarities, differences, and implications of their inhibition. Amn J Clin Oncolo 39: 98-106. https://doi.org/10.1097/COC.0000000000000239 ![]() |
[71] |
Alsaab HO, Sau S, Alzhrani R, et al. (2017) PD-1 and PD-L1 checkpoint signaling inhibition for cancer immunotherapy: Mechanism, combinations, and clinical outcome. Front Pharmacol 8: 561. https://doi.org/10.3389/fphar.2017.00561 ![]() |
[72] |
Champiat S, Dercle L, Ammari S, et al. (2017) Hyperprogressive disease is a new pattern of progression in cancer patients treated by anti-PD-1/PD-L1. Clin Cancer Res 23: 1920-1928. https://doi.org/10.1158/1078-0432.CCR-16-1741 ![]() |
[73] |
Wang Y, Zhou S, Yang F, et al. (2019) Treatment-related adverse events of PD-1 and PD-L1 inhibitors in clinical trials: A systematic review and meta-analysis. JAMA Oncol 5: 1008. https://doi.org/10.1001/jamaoncol.2019.0393 ![]() |
[74] |
Yi M, Zheng X, Niu M, et al. (2022) Combination strategies with PD-1/PD-L1 blockade: Current advances and future directions. Mol Cancer 21: 28. https://doi.org/10.1186/s12943-021-01489-2 ![]() |
[75] |
Akinleye A, Rasool Z (2019) Immune checkpoint inhibitors of PD-L1 as cancer therapeutics. J Hematol Oncol 12: 92. https://doi.org/10.1186/s13045-019-0779-5 ![]() |
[76] |
Debela DT, Muzazu SG, Heraro KD, et al. (2021) New approaches and procedures for cancer treatment: Current perspectives. SAGE Open Med 9. https://doi.org/10.1177/20503121211034366 ![]() |
[77] |
Goldhirsch A, Winer EP, Coates AS, et al. (2013) Personalizing the treatment of women with early breast cancer: Highlights of the St gallen international expert consensus on the primary therapy of early breast cancer 2013. Ann Oncol 24: 2206-2223. https://doi.org/10.1093/annonc/mdt303 ![]() |
[78] |
Shi J, Kantoff PW, Wooster R, et al. (2017) Cancer nanomedicine: Progress, challenges and opportunities. Nat Rev Cancer 17: 20-37. https://doi.org/10.1038/nrc.2016.108 ![]() |
[79] |
Bukowski K, Kciuk M, Kontek R (2020) Mechanisms of multidrug resistance in cancer chemotherapy. Int J Mol Sci 21: 3233. https://doi.org/10.3390/ijms21093233 ![]() |
[80] |
Jing X, Yang F, Shao C, et al. (2019) Role of hypoxia in cancer therapy by regulating the tumor microenvironment. Mol Cancer 18: 157. https://doi.org/10.1186/s12943-019-1089-9 ![]() |
[81] |
Yao Y, Zhou Y, Liu L, et al. (2020) Nanoparticle-based drug delivery in cancer therapy and its role in overcoming drug resistance. Front Mol Biosci 7: 193. https://doi.org/10.3389/fmolb.2020.00193 ![]() |
[82] |
Sun T, Zhang YS, Pang B, et al. (2014) Engineered nanoparticles for drug delivery in cancer therapy. Angew Chem Int Ed 53: 12320-12364. https://doi.org/10.1002/anie.201403036 ![]() |
[83] |
Anjum S, Ishaque S, Fatima H, et al. (2021) Emerging applications of nanotechnology in healthcare systems: Grand challenges and perspectives. Pharmaceuticals 14: 707. https://doi.org/10.3390/ph14080707 ![]() |
[84] |
Moore ZS, Seward JF, Lane JM (2006) Smallpox. Lancet 367: 425-435. https://doi.org/10.1016/S0140-6736(06)68143-9 ![]() |
[85] |
Hoover HC, Surdyke MG, Dangel RB, et al. (1985) Prospectively randomized trial of adjuvant active-specific immunotherapy for human colorectal cancer. Cancer 55: 1236-1243. https://doi.org/10.1002/1097-0142(19850315)55:6<1236::aid-cncr2820550616>3.0.co;2-# ![]() |
[86] |
Miao L, Zhang Y, Huang L (2021) mRNA vaccine for cancer immunotherapy. Mol Cancer 20: 41. https://doi.org/10.1186/s12943-021-01335-5 ![]() |
[87] |
Saxena M, van der Burg SH, Melief CJM, et al. (2021) Therapeutic cancer vaccines. Nat Rev Cancer 21: 360-378. https://doi.org/10.1038/s41568-021-00346-0 ![]() |
[88] |
Le DT, Pardoll DM, Jaffee EM (2010) Cellular vaccine approaches. Cancer J 16: 304. https://doi.org/10.1097/PPO.0b013e3181eb33d7 ![]() |
[89] |
Farhood B, Najafi M, Mortezaee K (2019) CD8+ cytotoxic T lymphocytes in cancer immunotherapy: A review. J Cell Physiol 234: 8509-8521. https://doi.org/10.1002/jcp.27782 ![]() |
[90] |
Fang RH, Hu CMJ, Luk BT, et al. (2014) Cancer cell membrane-coated nanoparticles for anticancer vaccination and drug delivery. Nano Lett 14: 2181-2188. https://doi.org/10.1021/nl500618u ![]() |
[91] |
Yada K, Nogami K, Ogiwara K, et al. (2013) Activated prothrombin complex concentrate (APCC)-mediated activation of factor (F)VIII in mixtures of FVIII and APCC enhances hemostatic effectiveness. J Thromb Haemostasis 11: 902-910. https://doi.org/10.1111/jth.12197 ![]() |
[92] |
Kennedy R, Celis E (2008) Multiple roles for CD4+ T cells in anti-tumor immune responses. Immunol Rev 222: 129-144. https://doi.org/10.1111/j.1600-065X.2008.00616.x ![]() |
[93] | Rossella S, Trovato M, Manco R, et al. (2021) Exploiting viral sensing mediated by Toll-like receptors to design innovative vaccines. NPJ Vaccines 6. https://doi.org/10.1038/s41541-021-00391-8 |
[94] |
Asiry S, Kim G, Filippou PS, et al. (2021) The cancer cell dissemination machinery as an immunosuppressive niche: A new obstacle towards the era of cancer immunotherapy. Front Immunol 12. https://doi.org/10.3389/fimmu.2021.654877 ![]() |
[95] |
Ott PA, Hodi FS, Robert C (2013) CTLA-4 and PD-1/PD-L1 blockade: New immunotherapeutic modalities with durable clinical benefit in melanoma patients. Clin Cancer Res 19: 5300-5309. https://doi.org/10.1158/1078-0432.CCR-13-0143 ![]() |
[96] |
Hargrave A, Mustafa AS, Hanif A, et al. (2023) Recent advances in cancer immunotherapy with a focus on FDA-approved vaccines and neoantigen-based vaccines. Vaccines 11: 1633. https://doi.org/10.3390/vaccines11111633 ![]() |
[97] |
Osipov A, Murphy A, Zheng L (2019) Chapter two-from immune checkpoints to vaccines: The past, present and future of cancer immunotherapy. Advances in Cancer Research. New York: Academic Press 63-144. https://doi.org/10.1016/bs.acr.2019.03.002 ![]() |
[98] |
Danila DC, Pantel K, Fleisher M, et al. (2011) Circulating tumors cells as biomarkers: Progress toward biomarker qualification. Cancer J 17: 438. https://doi.org/10.1097/PPO.0b013e31823e69ac ![]() |
[99] |
Rizzo A, Ricci AD, Brandi G (2021) PD-L1, TMB, MSI, and other predictors of response to immune checkpoint inhibitors in biliary tract cancer. Cancers 13: 558. https://doi.org/10.3390/cancers13030558 ![]() |
[100] |
Chae YK, Pan A, Davis AA, et al. (2016) Biomarkers for PD-1/PD-L1 blockade therapy in non–small-cell lung cancer: Is PD-L1 expression a good marker for patient selection?. Clin Lung Cancer 17: 350-361. https://doi.org/10.1016/j.cllc.2016.03.011 ![]() |
[101] |
Li K, Tian H (2019) Development of small-molecule immune checkpoint inhibitors of PD-1/PD-L1 as a new therapeutic strategy for tumour immunotherapy. J Drug Targeting 27: 244-256. https://doi.org/10.1080/1061186X.2018.1440400 ![]() |
[102] |
Long J, Lin J, Wang A, et al. (2017) PD-1/PD-L blockade in gastrointestinal cancers: Lessons learned and the road toward precision immunotherapy. J Hematol Oncol 10: 146. https://doi.org/10.1186/s13045-017-0511-2 ![]() |
[103] |
Mas-Ponte D, McCullough M, Supek F (2022) Spectrum of DNA mismatch repair failures viewed through the lens of cancer genomics and implications for therapy. Clin Sc 136: 383-404. https://doi.org/10.1042/CS20210682 ![]() |
[104] |
Mardis ER (2019) Neoantigens and genome instability: Impact on immunogenomic phenotypes and immunotherapy response. Genome Med 11: 71. https://doi.org/10.1186/s13073-019-0684-0 ![]() |
[105] |
Shimozaki K, Hayashi H, Tanishima S, et al. (2021) Concordance analysis of microsatellite instability status between polymerase chain reaction based testing and next generation sequencing for solid tumors. Sci Rep 11: 20003. https://doi.org/10.1038/s41598-021-99364-z ![]() |
[106] |
Gomez-Martín C, Lopez-Rios F, Aparicio J, et al. (2014) A critical review of HER2-positive gastric cancer evaluation and treatment: From trastuzumab, and beyond. Cancer Lett 351: 30-40. https://doi.org/10.1016/j.canlet.2014.05.019 ![]() |
[107] |
Duffy MJ, Crown J (2019) Biomarkers for predicting response to immunotherapy with immune checkpoint inhibitors in cancer patients. Clin Chem 65: 1228-1238. https://doi.org/10.1373/clinchem.2019.303644 ![]() |
[108] |
Riboldi M, Orecchia R, Baroni G (2012) Real-time tumour tracking in particle therapy: Technological developments and future perspectives. Lancet Oncol 13: e383-e391. https://doi.org/10.1016/S1470-2045(12)70243-7 ![]() |
[109] |
Danesi R, Fogli S, Indraccolo S, et al. (2021) Druggable targets meet oncogenic drivers: Opportunities and limitations of target-based classification of tumors and the role of Molecular Tumor Boards. ESMO Open 6: 100040. https://doi.org/10.1016/j.esmoop.2020.100040 ![]() |
[110] |
Sanchez A, Bocklage T (2019) Precision cytopathology: Expanding opportunities for biomarker testing in cytopathology. J Am Soc Cytopathol 8: 95-115. https://doi.org/10.1016/j.jasc.2018.12.003 ![]() |
[111] |
Łukaszewicz-Zając M, Pączek S, Muszyński P, et al. (2019) Comparison between clinical significance of serum CXCL-8 and classical tumor markers in oesophageal cancer (OC) patients. Clin Exp Med 19: 191-199. https://doi.org/10.1007/s10238-019-00548-9 ![]() |
[112] |
Roessler S, Jia HL, Budhu A, et al. (2010) A unique metastasis gene signature enables prediction of tumor relapse in early-stage hepatocellular carcinoma patients. Cancer Res 70: 10202-10212. https://doi.org/10.1158/0008-5472.CAN-10-2607 ![]() |
[113] |
Sun Z, Zhang N (2014) Clinical evaluation of CEA, CA19-9, CA72-4 and CA125 in gastric cancer patients with neoadjuvant chemotherapy. World J Surg Onc 12: 397. https://doi.org/10.1186/1477-7819-12-397 ![]() |
[114] |
Lakemeyer L, Sander S, Wittau M, et al. (2021) Diagnostic and prognostic value of CEA and CA19-9 in colorectal cancer. Diseases 9: 21. https://doi.org/10.3390/diseases9010021 ![]() |
[115] |
Jing JX, Wang Y, Xu XQ, et al. (2015) Tumor markers for diagnosis, monitoring of recurrence and prognosis in patients with upper gastrointestinal tract cancer. Asian Pac J Cancer Prev 15: 10267-10272. https://doi.org/10.7314/APJCP.2014.15.23.10267 ![]() |
[116] |
Galle PR, Foerster F, Kudo M, et al. (2019) Biology and significance of alpha-fetoprotein in hepatocellular carcinoma. Liver Int 39: 2214-2229. https://doi.org/10.1111/liv.14223 ![]() |
[117] |
He R, Yang Q, Dong X, et al. (2017) Clinicopathologic and prognostic characteristics of alpha-fetoprotein–producing gastric cancer. Oncotarget 8: 23817. https://doi.org/10.18632/oncotarget.15909 ![]() |
[118] |
Madhavan D, Peng C, Wallwiener M, et al. (2016) Circulating miRNAs with prognostic value in metastatic breast cancer and for early detection of metastasis. Carcinogenesis 37: 461-470. https://doi.org/10.1093/carcin/bgw008 ![]() |
[119] |
Hamam R, Hamam D, Alsaleh KA, et al. (2017) Circulating microRNAs in breast cancer: Novel diagnostic and prognostic biomarkers. Cell Death Dis 8: e3045-e3045. https://doi.org/10.1038/cddis.2017.440 ![]() |
[120] |
Li RY, Liang ZY (2020) Circulating tumor DNA in lung cancer: real-time monitoring of disease evolution and treatment response. Chin Med J 133: 2476-2485. https://doi.org/10.1097/CM9.0000000000001097 ![]() |
[121] |
Zhou H, Shen W, Zou H, et al. (2020) Circulating exosomal long non-coding RNA H19 as a potential novel diagnostic and prognostic biomarker for gastric cancer. J Int Med Res 48: 0300060520934297. https://doi.org/10.1177/0300060520934297 ![]() |
[122] |
Jiang L, Gu Y, Du Y, et al. (2019) Exosomes: Diagnostic biomarkers and therapeutic delivery vehicles for cancer. Mol Pharmaceutics 16: 3333-3349. https://doi.org/10.1021/acs.molpharmaceut.9b00409 ![]() |
[123] |
Huyghe N, Benidovskaya E, Stevens P, et al. (2022) Biomarkers of response and resistance to immunotherapy in microsatellite stable colorectal cancer: Toward a new personalized medicine. Cancers 14: 2241. https://doi.org/10.3390/cancers14092241 ![]() |
[124] |
Colle R, Cohen R, Cochereau D, et al. (2017) Immunotherapy and patients treated for cancer with microsatellite instability. Bull Cancer 104: 42-51. https://doi.org/10.1016/j.bulcan.2016.11.006 ![]() |
[125] |
He CY, Qiu MZ, Yang XH, et al. (2020) Classification of gastric cancer by EBV status combined with molecular profiling predicts patient prognosis. Clin Transl Med 10: 353-362. https://doi.org/10.1002/ctm2.32 ![]() |
[126] |
Shen H, Zhong M, Wang W, et al. (2017) EBV infection and MSI status significantly influence the clinical outcomes of gastric cancer patients. Clin Chim Acta 471: 216-221. https://doi.org/10.1016/j.cca.2017.06.006 ![]() |
[127] |
Yuan L, Xu ZY, Ruan SM, et al. (2020) Long non-coding RNAs towards precision medicine in gastric cancer: Early diagnosis, treatment, and drug resistance. Mol Cancer 19: 96. https://doi.org/10.1186/s12943-020-01219-0 ![]() |
[128] |
Cao F, Hu Y, Chen Z, et al. (2021) Circulating long noncoding RNAs as potential biomarkers for stomach cancer: A systematic review and meta-analysis. World J Surg Onc 19: 89. https://doi.org/10.1186/s12957-021-02194-6 ![]() |
[129] |
Li J, Wu Z, Lin R (2024) Impact of Helicobacter pylori on immunotherapy in gastric cancer. J Immunother Cancer 12: e010354. https://doi.org/10.1136/jitc-2024-010354 ![]() |
[130] | Zhong X, Zheng H, Zhao S, et al. (2024) Effects and mechanisms of Helicobacter pylori on cancers development and immunotherapy. Front Immunol 15. https://doi.org/10.3389/fimmu.2024.1469096 |
[131] |
Wang Y, Shatila M, Sperling G, et al. (2024) Helicobacter pylori infection and response of gastric cancer to immunotherapy. J Clin Oncol 42: 277-277. https://doi.org/10.1200/JCO.2024.42.3_suppl.277 ![]() |
[132] |
Magahis PT, Maron SB, Cowzer D, et al. (2023) Impact of Helicobacter pylori infection status on outcomes among patients with advanced gastric cancer treated with immune checkpoint inhibitors. J Immunother Cancer 11: e007699. https://doi.org/10.1136/jitc-2023-007699 ![]() |
[133] |
Hegde PS, Chen DS (2020) Top 10 challenges in cancer immunotherapy. Immunity 52: 17-35. https://doi.org/10.1016/j.immuni.2019.12.011 ![]() |
[134] |
Chen K, Shuen TWH, Chow PKH (2024) The association between tumour heterogeneity and immune evasion mechanisms in hepatocellular carcinoma and its clinical implications. Br J Cancer 131: 420. https://doi.org/10.1038/s41416-024-02684-w ![]() |
[135] |
Chowell D, Morris LGT, Grigg CM, et al. (2018) Patient HLA class I genotype influences cancer response to checkpoint blockade immunotherapy. Science 359: 582-587. https://doi.org/10.1126/science.aao4572 ![]() |
[136] |
Schneider BJ, Naidoo J, Santomasso BD, et al. (2021) Management of immune-related adverse events in patients treated with immune checkpoint inhibitor therapy: ASCO guideline update. J Clin Oncol 39: 4073-4126. https://doi.org/10.1200/JCO.21.01440 ![]() |
[137] |
Gopalakrishnan V, Spencer CN, Nezi L, et al. (2018) Gut microbiome modulates response to anti–PD-1 immunotherapy in melanoma patients. Science 359: 97-103. https://doi.org/10.1126/science.aan4236 ![]() |
[138] |
Pagliuca S, Gurnari C, Rubio MT, et al. (2022) Individual HLA heterogeneity and its implications for cellular immune evasion in cancer and beyond. Front Immunol 13. https://doi.org/10.3389/fimmu.2022.944872 ![]() |
[139] |
Mitchell AL, Gandhi A, Scott-Coombes D, et al. (2016) Management of thyroid cancer: United kingdom national multidisciplinary guidelines. J Laryngol Otol 130: S150-S160. https://doi.org/10.1017/S0022215116000578 ![]() |
[140] |
Dhatchinamoorthy K, Colbert JD, Rock KL (2021) Cancer Immune evasion through loss of MHC class I antigen presentation. Front Immunol 12: 636568. https://doi.org/10.3389/fimmu.2021.636568 ![]() |
[141] |
Postow MA, Sidlow R, Hellmann MD (2018) Immune-related adverse events associated with immune checkpoint blockade. N Engl J Med 378: 158-168. https://doi.org/10.1056/NEJMra1703481 ![]() |
[142] |
Brahmer JR, Lacchetti C, Schneider BJ, et al. (2018) Management of immune-related adverse events in patients treated with immune checkpoint inhibitor therapy: American society of clinical oncology clinical practice guideline. J Clin Oncol 36: 1714-1768. https://doi.org/10.1200/JCO.2017.77.6385 ![]() |
Drug Name | Brand Name | Target | Year Approved | Indications | Ref |
Ipilimumab | Yervoy | CTLA-4 | 2011 | Metastatic melanoma, renal cell carcinoma, colorectal cancer | [36],[37] |
Nivolumab | Opdivo | PD-1 | 2014 | Melanoma, lung cancer, kidney cancer, bladder cancer | [38],[39] |
Pembrolizumab | Keytruda | PD-1 | 2014 | Melanoma, lung cancer, head and neck cancer, Hodgkin's lymphoma | [40] |
Atezolizumab | Tecentriq | PD-L1 | 2016 | Bladder cancer, non-small cell lung cancer, breast cancer | [41] |
Avelumab | Bavencio | PD-L1 | 2017 | Merkel cell carcinoma, urothelial carcinoma | [42] |
Durvalumab | Imfinzi | PD-L1 | 2017 | Non-small cell lung cancer, small cell lung cancer | [43] |
Cemiplimab | Libtayo | PD-1 | 2021 | Squamous cell carcinoma of the skin, non-small cell lung cancer | [44] |
Dostarlimab | Jemperli | PD-1 | 2021 | Endometrial cancer | [45] |
Tremelimumab | Imjudo | CTLA-4 | 2022 | Hepatocellular carcinoma (in combination with Durvalumab) | [46] |
Toripalimab | Loqtorzi | PD-1 | 2023 | Nasopharyngeal carcinoma | [47] |
Spartalizumab | PDR001 | PD-1 | TBD | Under investigation for various solid tumors and lymphomas | |
Abemaciclib | Verzenio | CDK4/6 | 2017 | Breast cancer (in combination with pembrolizumab) | [48] |
Tislelizumab | Baiyin | PD-1 | 2022 | Non-small cell lung cancer | [49] |
Rovalpituzumab | Rova-T | DLL3 | TBD | Small cell lung cancer | [50] |
Biomarker Type | Biomarker | Clinical Application | Action on Stomach Cancer | Ref |
Classical Tumor Markers | CEA | Diagnosis, monitoring, and predicting recurrence | Higher cancer levels suggest higher stages and possibility of liver metastasis | [111],[112] |
CA19-9 | Diagnosis and monitoring | Linked to other clinicopathological characteristics and employed for predicting relapse | [113],[114] | |
CA72-4 | Follow-up after treatment | Good specificity for gastric cancer; used to identify relapses | [113],[115] | |
Alpha-fetoprotein (AFP) | Diagnosis and prognosis | Indicates aggressive disease and higher metastatic potential | [116],[117] | |
Circulating Biomarkers | Circulating free miRNAs | Early diagnosis and prognosis | Several miRNAs observed in the study are potential players in clinical prognosis: total survival, lymph node metastasis | [118],[119] |
Circulating tumor cells | Monitoring treatment response | Noninvasive method to assess tumor dynamics in real-time | [120] | |
Exosomal lncRNAs | Diagnostic and prognostic indicators | Some lncRNAs could express greatly significant difference in cancer tissues compared with that in healthy individuals | [121],[122] | |
Genetic and Epigenetic Markers | Microsatellite Instability (MSI) | Predictive for immunotherapy response | High mutational load correlates with better survival outcomes | [123],[124] |
EBV status | Prognostic indicator | Connected with a particular type of gastric cancer that defines the therapy approaches | [125],[126] | |
Emerging Biomarkers | Long noncoding RNAs (lncRNAs) | Early detection and prognosis | These lncRNAs have potential for use as stable diagnostic indicators of gastric cancer | [127] |
Circular RNAs (circRNAs) | Diagnostic potential | Some of the circRNA panels have been shown to distinguish gastric cancer tissues from normal ones | [128] |
Drug Name | Brand Name | Target | Year Approved | Indications | Ref |
Ipilimumab | Yervoy | CTLA-4 | 2011 | Metastatic melanoma, renal cell carcinoma, colorectal cancer | [36],[37] |
Nivolumab | Opdivo | PD-1 | 2014 | Melanoma, lung cancer, kidney cancer, bladder cancer | [38],[39] |
Pembrolizumab | Keytruda | PD-1 | 2014 | Melanoma, lung cancer, head and neck cancer, Hodgkin's lymphoma | [40] |
Atezolizumab | Tecentriq | PD-L1 | 2016 | Bladder cancer, non-small cell lung cancer, breast cancer | [41] |
Avelumab | Bavencio | PD-L1 | 2017 | Merkel cell carcinoma, urothelial carcinoma | [42] |
Durvalumab | Imfinzi | PD-L1 | 2017 | Non-small cell lung cancer, small cell lung cancer | [43] |
Cemiplimab | Libtayo | PD-1 | 2021 | Squamous cell carcinoma of the skin, non-small cell lung cancer | [44] |
Dostarlimab | Jemperli | PD-1 | 2021 | Endometrial cancer | [45] |
Tremelimumab | Imjudo | CTLA-4 | 2022 | Hepatocellular carcinoma (in combination with Durvalumab) | [46] |
Toripalimab | Loqtorzi | PD-1 | 2023 | Nasopharyngeal carcinoma | [47] |
Spartalizumab | PDR001 | PD-1 | TBD | Under investigation for various solid tumors and lymphomas | |
Abemaciclib | Verzenio | CDK4/6 | 2017 | Breast cancer (in combination with pembrolizumab) | [48] |
Tislelizumab | Baiyin | PD-1 | 2022 | Non-small cell lung cancer | [49] |
Rovalpituzumab | Rova-T | DLL3 | TBD | Small cell lung cancer | [50] |
Biomarker Type | Biomarker | Clinical Application | Action on Stomach Cancer | Ref |
Classical Tumor Markers | CEA | Diagnosis, monitoring, and predicting recurrence | Higher cancer levels suggest higher stages and possibility of liver metastasis | [111],[112] |
CA19-9 | Diagnosis and monitoring | Linked to other clinicopathological characteristics and employed for predicting relapse | [113],[114] | |
CA72-4 | Follow-up after treatment | Good specificity for gastric cancer; used to identify relapses | [113],[115] | |
Alpha-fetoprotein (AFP) | Diagnosis and prognosis | Indicates aggressive disease and higher metastatic potential | [116],[117] | |
Circulating Biomarkers | Circulating free miRNAs | Early diagnosis and prognosis | Several miRNAs observed in the study are potential players in clinical prognosis: total survival, lymph node metastasis | [118],[119] |
Circulating tumor cells | Monitoring treatment response | Noninvasive method to assess tumor dynamics in real-time | [120] | |
Exosomal lncRNAs | Diagnostic and prognostic indicators | Some lncRNAs could express greatly significant difference in cancer tissues compared with that in healthy individuals | [121],[122] | |
Genetic and Epigenetic Markers | Microsatellite Instability (MSI) | Predictive for immunotherapy response | High mutational load correlates with better survival outcomes | [123],[124] |
EBV status | Prognostic indicator | Connected with a particular type of gastric cancer that defines the therapy approaches | [125],[126] | |
Emerging Biomarkers | Long noncoding RNAs (lncRNAs) | Early detection and prognosis | These lncRNAs have potential for use as stable diagnostic indicators of gastric cancer | [127] |
Circular RNAs (circRNAs) | Diagnostic potential | Some of the circRNA panels have been shown to distinguish gastric cancer tissues from normal ones | [128] |