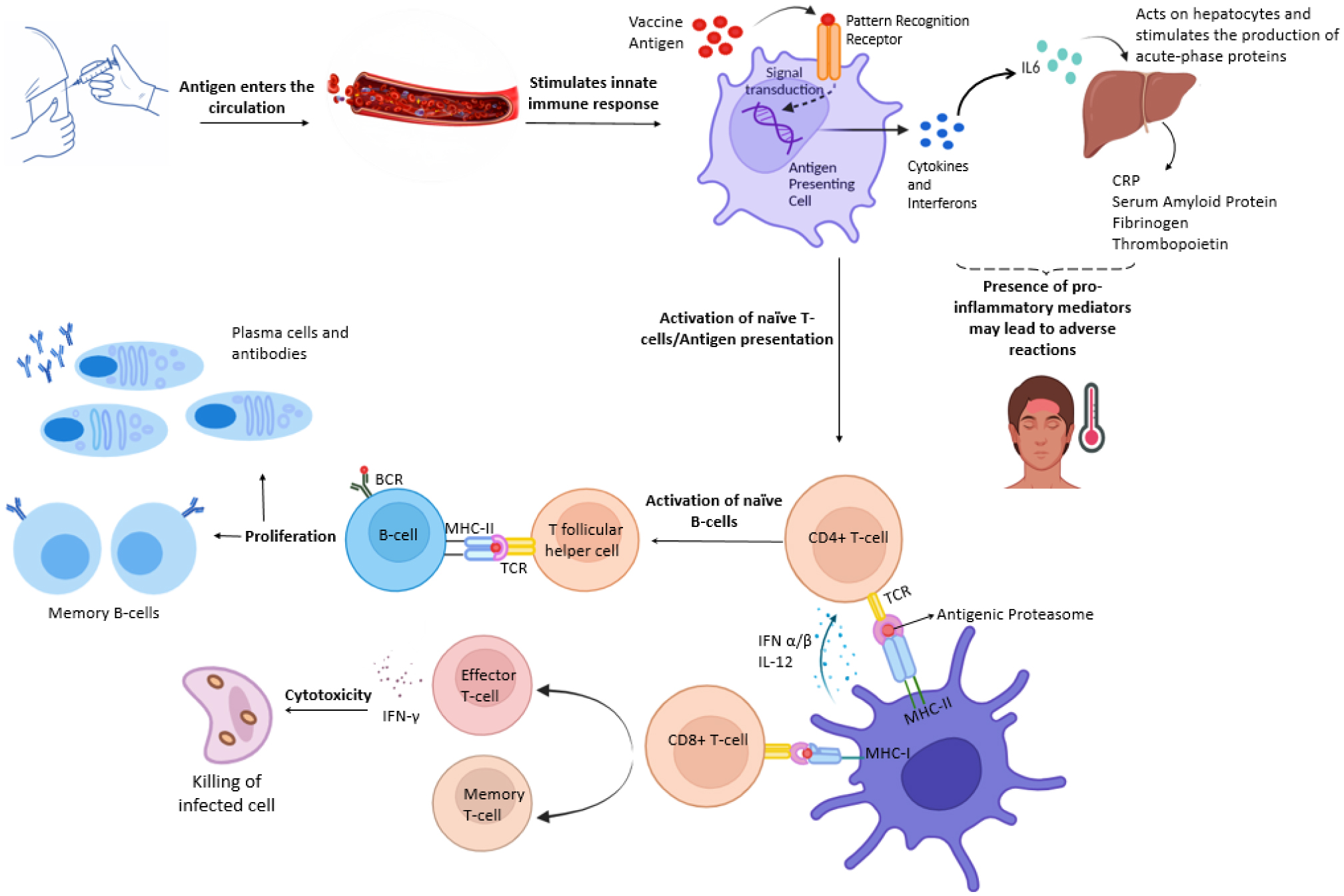
Since the initiation of immunization against SARS-CoV-2, the adverse reactions of vaccines have remained a major contributor to vaccine hesitancy. Their occurrence has been attributed to the immune response against the administered antigen, which is affected by different factors including the age of the vaccine recipient. The trends of the adverse reactions of COVID-19 vaccines have shown a higher frequency in younger adults (18–64 years old) than in the older population (≥65 years). One of the potential reasons is the healthy and efficient immune systems of younger individuals, which provide a robust pro-inflammatory response upon antigen encounter. Their adaptive immune system has a diverse array of naïve T and B lymphocytes, which aids in antigen elimination with the development of a long-lasting immunological memory. Age-related changes in the immune system of the elderly are referred to as immunosenescence, which reduces its efficiency. The phenomenon of cellular senescence and inflammageing can lower the vaccine responses of older recipients. Thymic involution and ageing of the bone marrow can lead to decreased levels of naïve T-cells and memory B-cells. This could compromise the ability to either fight new infections or effectively respond to vaccines and develop a lasting immune memory. Along with clinical trials of senolytic drugs, lifestyle interventions are being studied to mitigate such age-related changes. Further insights into vaccine responses in the elderly population and the means to alleviate the hallmarks of immunosenescence are required.
Citation: Laiba Noor, Syeda Momna Ishtiaq, Farhat Batool, Muhammad Imran Arshad. Age-dependent trends in adverse reactions of SARS-CoV-2 vaccines: A narrative review[J]. AIMS Allergy and Immunology, 2024, 8(3): 146-166. doi: 10.3934/Allergy.2024008
[1] | Darrell O. Ricke . Etiology model for many vaccination adverse reactions, including SARS-CoV-2 spike vaccines. AIMS Allergy and Immunology, 2022, 6(4): 200-215. doi: 10.3934/Allergy.2022015 |
[2] | Yu. A. Desheva, A. S. Mamontov, P. G. Nazarov . Contribution of antibody-dependent enhancement to the pathogenesis of coronavirus infections. AIMS Allergy and Immunology, 2020, 4(3): 50-59. doi: 10.3934/Allergy.2020005 |
[3] | Darrell O. Ricke . Antibodies and infected monocytes and macrophages in COVID-19 patients. AIMS Allergy and Immunology, 2022, 6(2): 64-70. doi: 10.3934/Allergy.2022007 |
[4] | Daniel Danladi Gaiya, Jonathan Danladi Gaiya, Richard Auta, Aliyu Muhammad, Bege Jonathan, Stella Kuyet Udu, Ekpa Emmanuel, Amina Shehu Bature . The journey so far with SARS-CoV-2 variants: Pathogenesis, immunity and treatments. AIMS Allergy and Immunology, 2023, 7(4): 222-250. doi: 10.3934/Allergy.2023016 |
[5] | Kamyar Nasiri, Zohreh Mortezania, Sanaz Oftadehbalani, Mohammad Khosousi Sani, Amin Daemi, Seyyedeh Touran Hosseini, Yusuf Döğüş, Zafer Yönden . Parasitology and Covid-19: epidemiological, immunological, pathological, and therapeutic aspects. AIMS Allergy and Immunology, 2023, 7(1): 92-103. doi: 10.3934/Allergy.2023007 |
[6] | Lia Tsverava, Nazibrola Chitadze, Gvantsa Chanturia, Merab Kekelidze, David Dzneladze, Paata Imnadze, Amiran Gamkrelidze, Vincenzo Lagani, Zaza Khuchua, Revaz Solomonia . Antibody profiling reveals gender differences in response to SARS-COVID-2 infection. AIMS Allergy and Immunology, 2022, 6(1): 6-13. doi: 10.3934/Allergy.2022002 |
[7] | DharmaSaranya Gurusamy, Vasuki Selvamurugesan, Swaminathan Kalyanasundaram, Shantaraman Kalyanaraman . Is COVID 19, a beginning of new entity called chronic viral systemic inflammatory syndrome?. AIMS Allergy and Immunology, 2021, 5(2): 127-134. doi: 10.3934/Allergy.2021010 |
[8] | Mansur Aliyu, Sayed-Hamidreza Mozhgani, Omid Kohandel Gargari, Mustapha Ahmed Yusuf, Ali Akbar Saboor-Yaraghi . The host immune responses to SARS-CoV-2 and therapeutic strategies in the treatment of COVID-19 cytokine storm. AIMS Allergy and Immunology, 2021, 5(4): 240-258. doi: 10.3934/Allergy.2021018 |
[9] | Darrell O. Ricke . Rare dizziness, syncope, loss of consciousness, seizure, and risk of falling after vaccination. AIMS Allergy and Immunology, 2023, 7(2): 164-175. doi: 10.3934/Allergy.2023011 |
[10] | Y. Desheva, T. Shvedova, A. Lerner, P. Kudar, O. Kopteva, D. Petrachkova, I. Koroleva, G. Leontieva, S. Ponkratov, A. Suvorov . Exploring immune response and lab markers across COVID-19 severity levels. AIMS Allergy and Immunology, 2024, 8(4): 216-231. doi: 10.3934/Allergy.2024013 |
Since the initiation of immunization against SARS-CoV-2, the adverse reactions of vaccines have remained a major contributor to vaccine hesitancy. Their occurrence has been attributed to the immune response against the administered antigen, which is affected by different factors including the age of the vaccine recipient. The trends of the adverse reactions of COVID-19 vaccines have shown a higher frequency in younger adults (18–64 years old) than in the older population (≥65 years). One of the potential reasons is the healthy and efficient immune systems of younger individuals, which provide a robust pro-inflammatory response upon antigen encounter. Their adaptive immune system has a diverse array of naïve T and B lymphocytes, which aids in antigen elimination with the development of a long-lasting immunological memory. Age-related changes in the immune system of the elderly are referred to as immunosenescence, which reduces its efficiency. The phenomenon of cellular senescence and inflammageing can lower the vaccine responses of older recipients. Thymic involution and ageing of the bone marrow can lead to decreased levels of naïve T-cells and memory B-cells. This could compromise the ability to either fight new infections or effectively respond to vaccines and develop a lasting immune memory. Along with clinical trials of senolytic drugs, lifestyle interventions are being studied to mitigate such age-related changes. Further insights into vaccine responses in the elderly population and the means to alleviate the hallmarks of immunosenescence are required.
Coronaviruses have been a threat to both animals and humans for nearly 90 years [1]. The most recent pandemic of severe acute respiratory syndrome coronavirus-2 (SARS-CoV-2), which causes coronavirus disease 2019 (COVID-19), serves as a vivid example. The first case of COVID-19 was reported in Wuhan, China in December 2019 [2],[3], which resulted in a rapid surge in cases and fatalities. Consequently, on March 11, 2020, the World Health Organization (WHO) declared it a public health emergency of international concern. As of March 2023, COVID-19 has afflicted 676 million individuals and claimed nearly seven million lives, making it one of the deadliest pandemics [3],[4].
Vaccines are considered the most promising approach to control pandemics [5]. Vaccines against COVID-19 have been developed using technologies such as inactivated, viral vectors, nucleic acid, and subunit vaccines [2]. They have unique mechanisms of action based on their respective ingredients and technologies. A study of the Real-World Effectiveness (RWE) of these vaccines showed high protection against COVID-19 [6]. This can be demonstrated by the fact that the WHO declared COVID-19 to be over as a global health emergency on 5th of May 2023. While this indicates the success of our immense immunization efforts, we cannot overlook the limitations of our disease control strategies, which lead to a high number of cases and deaths. This is crucial to prepare for any future infection outbreaks [7].
Strategies such as vaccine optimization are among the primary measures of intervention responses. It involves giving priority to people who are more at risk of becoming seriously ill and potentially dying from the disease. These measures are key to effective prevention through vaccination against infections such as COVID-19. Elderly people have been given priority for vaccination against SARS-CoV-2 because younger people have been shown to have a significantly lower proportion of severe infections [8],[9]. Additionally, the disease mortality rate is higher in older individuals [10]. This can be attributed to the ageing process, which alters the structural and functional capabilities of the elderly. The process of immune system ageing, known as immunosenescence, is a potential reason for the increased susceptibility to infections [11].
Immunosenescence not only increases the chance of severe infections, but can also make the development of long-term immunity through vaccination difficult [12]. This indicates the significance of evaluating the immune system response of the vulnerable aged population to administered vaccines. This will help to understand the issue of the reduced vaccine efficacy among the immunocompromised elderly population and is also necessary to identify the need for any subsequent vaccination strategies. In this review, we discuss the adverse reactions and safety of SARS-CoV-2 vaccines in young and elderly populations, highlighting the relationship between immune ageing and vaccine responses and its impact on developing and maintaining immunological memory, with the need for safer and more effective vaccines for frail elderly people.
Adverse events refer to a series of reactions that can occur after vaccination and are physical manifestations of the immune response to the administered pathogen [13]. The safety profile of any vaccine is determined by the intensity of the adverse events (AEs) that can potentially occur after vaccination. These signs and symptoms include local and systemic responses. They are often mild and self-limiting and do not cause serious medical complications [14].
The most frequently reported AEs associated with SARS-CoV-2 vaccines involve localized reactions of redness or soreness at the injection site and systemic reactions such as malaise, lethargy, headache, fever, chills, myalgia, and diarrhea [15],[16]. Some rare cases of cardiovascular AEs, such as blood clotting in multiple vessels and anaphylaxis, were observed [17]. Along with certain host biological factors including age, sex, and the presence of any comorbidities, the previous COVID-19 infection profile, vaccine types, and doses have also been found to be associated with the occurrence of such severe adverse reactions [13],[18]–[20]. Moreover, several lifestyle factors of vaccinees, including physical activity, non-smokers/smokers, demographics, and ethnicities were also found to significantly impact the prevalence of vaccine side effects [19]–[22]. These studies have concluded the role of increasing age in decreasing the vaccine AEs frequency [23],[24]. Table 1 describes the different types of available SARS-CoV-2 vaccines along with their reported adverse reactions.
The clinical trial results of all available COVID-19 vaccines have found them to be extremely safe and effective [18]. However, in real-world settings, no vaccine has been claimed to be completely free of side effects. Therefore, understanding the immune responses to these vaccines among a range of individuals is helpful to identify effective policies for infection prevention through immunization [13],[25].
As the prevalence of vaccine-related adverse events exacerbates the issue of vaccine hesitancy, there is a dire need to better understand the mechanism of vaccines and the resultant reactions (Figure 1) to scientifically combat this issue. Vaccines contain antigens to stimulate the immune response and to provide protection against a specific disease [26],[27]. Upon entering the body, vaccine antigens are recognized as a potential threat through specific pathogen-associated molecular patterns (PAMPs) on their surface by pattern recognition receptors (PRRs), including the Toll-like receptors of antigen presenting cells (APCs) of innate immunity [28].
This induces the transcription of certain genes in these cells, thus leading to the production of specific proteins including pro-inflammatory cytokines, chemokines, and other soluble factors, such as vasodilators, as a result of their interaction with a viral pathogen [29]. Among cytokines, type 1 interferons (IFN-I) play a primary role in empowering the initial immune response against the administered viral antigen. IFN-I mainly includes IFN-β and IFN-α, which are secreted by APCs, including the dendritic cells (conventional and plasmacytoid DCs) and macrophages. This IFN-I is the main link between innate immunity and the activation of the adaptive immune response. Additionally, IFN-γ is involved in the activation of adaptive immunity [30].
This interferon signaling induces the process of antigen presentation by activated APCs to CD4+ naïve T-cells and CD8+ naïve T-cells by both major histocompatibility complex (MHC) class I and II embedded in the surface of APCs [14],[30]. Additionally, the presence of pro-inflammatory mediators in the bloodstream rapidly increases the levels of various systemic factors including C-reactive and other acute-phase proteins of hepatic origin after vaccine administration [31].
These inflammatory mediators produced following antigen exposure are responsible for vaccine-associated local and systemic adverse reactions. Local AEs are thought to occur because of the activation of local resident immune cells including DCs and macrophages. Systemic reactions are the result of the presence of inflammatory mediators in the circulation, which initiate signals at the blood-brain barrier level and stimulate pyrogenic and flu-like symptoms [13],[32].
The resultant SARS-CoV-2 specific cytotoxic CD8+ T-cells produce IFN-γ with some expression of tumor necrosis factor (TNF) and interleukin-2 to directly kill infected cells [33],[34]. The surviving T-cells produce memory cells [27]. The virus specific helper CD4+ T-cells help to induce B-cells and humoral immune response. This initiates the formation of plasma cells through the production of antigen-specific neutralizing antibodies. After a few days, this process is followed by the apoptosis of short-lived plasma cells and long-lived plasma cells, make the effector memory cells [35]. Moreover, memory B-cells with B-cell receptors on their surface contribute to immune memory generation after antigen encounter [35]. These memory cells travel to reside in the bone marrow niches [27], while the memory T-cells reside in tissues and lymph nodes [27]. This leads to the development of an effective long-term immunological memory [34],[36],[37]. This immune memory is the source of protective immunity against specific pathogens in future encounters [27].
Name | Type | Mode of action | Doses | Efficacy | Reported adverse events |
Pfizer-BioNTech (BNT162b2) | Nucleic acid | Contains mRNA sequence that codes for viral spike protein | ×2 | 89% | Non-serious common vaccine related AEs were reported with some rare incidences of cardiac inflammation and anaphylactic reactions [43],[44]. |
Moderna (mRNA–1273) | Nucleic acid | RNA segment coding for viral spike protein | ×2 | 92% | Reported greater common vaccine AEs compared to BNT162b2 vaccine particularly after both doses. The reports of severe vaccine reactions were lower than BNT162b2 vaccinees [43],[44]. |
AstraZeneca (ChAdOx1) | Viral Vector | Contains genetically modified adenovirus that cannot replicate but can code for SARS-CoV-2 proteins | ×2 | 62%–90% | Mild and short-term common vaccine local and systemic adverse reactions were reported. Some severe adverse reactions including venous thromboembolic events and Guillain–Barré syndrome (GBS) were also found to be the potential safety signals for ChAdOx1 particularly after first dose [45],[46]. |
Janssen | Viral Vector | A non-replicating virus transports the genetic material of SARS-CoV-2 virus | ×1 | 66% | Minor vaccine adverse events of local to general systemic reactions were reported with paresthesia or hypoesthesia, tinnitus and swollen lymph nodes. Rare incidences of blood clotting with thrombocytopenia and anaphylactic reactions were also reported but at a lower rate than ChAdOx1 vaccinees [47],[48]. |
Sputnik V | Viral Vector | A non-replicating virus transports the genetic material of SARS-CoV-2 virus | ×2 | 91% | Mild to moderate injection site and systemic reactions were reported. No severe post-administration complications were observed [49],[50]. |
CanSino | Viral Vector | Non-replicating adenovirus delivers the genetic material of SARS-CoV-2 virus | ×1 | 65% | Common vaccine adverse reactions were reported with a greater prevalence of systemic reactions of fever and fatigue than local adverse events. Some serious events of pneumonia were reported but found to be coincidental [51],[52]. |
Sinopharm | Inactivated | Contains killed virus particles | ×2 | 79% | Mild general vaccine adverse reactions were reported mostly after the first dose. Some rare incidences of cardiac arrest and GBS were also reported. Overall shows a lower prevalence of AEs than mRNA and ChAdOx1 vaccines [52]–[54]. |
Sinovac | Inactivated | Have killed virus particles | ×2 | 51% | Non-serious AEs were observed at a lower rate than most of the other COVID-19 vaccines. Some severe incidences of myocardial infarction and allergic reactions were reported [52],[55]. |
Novavax | Protein Subunit | Contains Isolated virus particles | ×2 | 90% | Moderate adverse reactions with a higher prevalence of local symptoms were observed after both doses. Some cases of myocarditis/pericarditis were reported [56]. |
Individuals belonging to this age group are regarded as high sources of viral transmission. Therefore, to reduce disease morbidity with a higher vaccine coverage, immunization of this population is recommended [8]. While evaluating the occurrence of the available SARS-CoV-2 vaccine related AEs among individuals aged between 18–64 years, studies have indicated that they were more likely to experience such reactions than the older population [57].
Both commonly reported local and systemic AEs have shown a higher prevalence in this age group [58]–[63]. Among these side effects, local reactions including pain and soreness at the injection site are more frequently reported in these individuals than systemic reactions. On the other hand, they are at a lower risk of severe adverse reactions such as side effects leading to hospitalization, any permanent disability, or death compared to older adults [57]. There were certain cases of vaccine-related heart inflammation which led to myocarditis and pericarditis, specifically post-mRNA vaccine administration among younger or adolescent individuals. However, these side effects were found to be self-limiting and were more associated with the gender and age of the vaccine recipients [64],[65]. Other rare cases of thromboembolic events and liver injuries after the administration of different COVID-19 vaccines were also mostly observed among individuals of this age group [41],[66]–[69]. Regardless of the vaccine type and dosage frequency, individuals who belong to younger age groups are at a higher risk of experiencing SARS-CoV-2 vaccines adverse reactions [70].
Along with many extrinsic and intrinsic factors, one of the potential reasons for such vaccine responses is the efficient and active immune system of younger individuals, which vigorously reacts against the antigens of the vaccines [13]. In addition to the high number of circulating monocytic and dendritic cells of the innate immune system, the adaptive immune system of younger individuals with a prodigiously active thymus also has higher inactivated/naïve T-cells (i.e., the T-cells that have not yet encountered any antigen). Thus, with a large repertoire of T-cell receptors (TCR), the younger population produces a varied set of memory T-cells after exposure to a new antigen. Similarly, the intact functional ability of naïve B-cells to differentiate into plasmablasts and produce neutralizing antibodies aids in the development of a long-lasting immune memory [71],[72]. Therefore, prompt antigen detection induces an effective immune response. The resultant production of higher levels of acute inflammatory mediators as a result of this antigen encounter in younger vaccinees increases their risk of experiencing vaccine side effects [13],[73],[74].
Similarly, the unusual post-vaccination manifestations of myocarditis and pericarditis in this age group involve genetic predispositions and a more intense pro-inflammatory response, mainly IFN-γ signaling, which is found to activate the cardiac inflammation pathway [75]–[77]. The AE of SARS-CoV-2 vaccine-induced immune thrombotic thrombocytopenia (VITT) is an autoimmune condition characterized by higher titers of antibodies (IgG) that activate platelets, which leads to thrombosis in arterial and venous circulation [78]. Platelet activation releases platelet factor 4 (PF4), which helps to neutralize the antigen by binding and opsonizing the antigens, which facilitates the binding of anti-PF4 antibodies to it. In the case of VITT, antibodies bind to platelets and induce the release of procoagulant platelet microparticles [78]. Such side effects can be mitigated by early testing for anti-PF4 antibodies and treatment targeting autoimmune processes [78].
Additionally, these adverse reactions have been reported in younger adults and follow the same underlying mechanism of increased levels of pro-inflammatory cytokines, leading to a high grade inflammatory state [79],[80]. On the other hand, certain reports of immune-mediated liver injuries post-vaccination are found to be more associated with the presence of any comorbidity, usage of certain drugs, and the genetic susceptibility of the vaccinee [81]–[83]. For such AEs, therapeutic management, including corticosteroid treatment, is found to be effective [84].
Older people are more vulnerable to severe infections and mortality and are considered a high-risk category in the event of an outbreak. Thus, these individuals are recommended to be prioritized during the early stages of immunization efforts [8]. We reviewed the reported AEs of the COVID-19 vaccines in individuals aged ≥ 65 years. Although they are more susceptible to serious illnesses, they are less likely to experience vaccine side effects.
The individuals belonging to this age group reported reduced trends of adverse reactions to all the available SARS-CoV-2 vaccines [58]–[63]. In contrast, the elderly population was found to be at a higher risk of serious post-vaccination AEs, which led to hospitalization or even death [57]. There are reports of vaccine-related deaths in frail elderly persons in Norway and Germany [85],[86]. On evaluation of these events, it has been determined that the common adverse reactions associated with the available SARS-CoV-2 vaccines may aggravate the underlying illnesses of older populations [86],[87].
The observed higher risk of severe infections and lower rates of common vaccine AEs in the elderly population can be attributed to immunosenescence, which is the gradual decrease in the functional ability of one's immune system with increasing age [88]. This senescence of immune cells is characterized by permanent cell growth arrest, prevents the proliferation of damaged cells and autophagy, and results in the accumulation of impaired immune cells [89]. The key feature of cellular senescence is the release of numerous inflammatory mediators with growth factors and proteases that affect inter-cellular communication [89]. Consequently, it alters antigen sensing, antigen presentation, and pro-inflammatory responses of immune cells post-antigen encounter. Moreover, the expression and function of TLRs in the innate immune system has been found to be imbalanced in elderly individuals [90]. Therefore, this process contributes to the reduced capabilities of the innate and adaptive immune systems and increases the incidence of infections with a decreased response to vaccination [91].
This phenomenon of cell-cycle arrest leads to thymic atrophy, reduces the levels of naïve T-cells, and promotes the accumulation of memory T-cells in the elderly population. Age related changes in the bone marrow include the accumulation of old hematopoietic stem cells (HSCs), which have a decreased reconstitution potential [92]. This ultimately affects the production of naïve B-cells and decreases the efficiency and diversity of the antibody response [91],[93]. A comparison of the vaccine response of an adult's efficient immune system with that of immunocompromised elderly individuals is presented in Figure 2. Additionally, cellular senescence contributes to the process of inflammageing. This term is referred to as chronic low-grade inflammation associated with ageing. Constant inflammation signaling as a result of senescent cells produces a senescence-associated secretory phenotype (SASP), which continuously releases cytokines and chemokines; the prompt of leukocyte recruitment exhausts the immune system, which resultantly affects its response to any new antigen [71]. This leads to an impaired vaccine response and a reduced risk of vaccine reactions in the elderly population.
It is important to understand the relationship between AEs and immunological memory induced by administered vaccines to prevent vaccine hesitancy and promote vaccine uptake. Immunological memory is the ability of the immune system to remember a pathogen and to initiate a rapid and effective response upon subsequent exposure. This allows vaccines to provide protection against disease [94].
While the inflammatory response of innate immunity is rapid and non-specific, the adaptive immune response is highly specific and is responsible for the development of a long-lasting immune memory by the production of specific antibodies and by maintaining memory B and T-cells [95]. Upon pathogen encounter, pro-inflammatory cytokines produced by the natural immune system initiate a more specific adaptive immune response, which is considered to be the perpetrator of vaccine adverse reactions [14]. Additionally, along with taking part in the activation of naïve T-cells of adaptive immunity, the inflammatory cytokines are involved in the regulation of antigen sensitivity, proliferation, and trafficking of both effector and memory T-cells [96],[97].
Most pathogen specific CD8+ T-cells are eliminated through apoptosis upon pathogen destruction, while some effector T-cells survive. Similarly, inflammatory mediators also induce the activation of naïve CD4+ T-cells [98],[99]. These CD4+ and CD8+ T-cells make durable epitope specific memory cells that are found to be stable following 3–6 months after vaccination [100]. Similarly, the antibodies produced as a result of a humoral response are found to be considerably effective after 6-months of COVID-19 vaccination [101].
Therefore, considering the different extrinsic and intrinsic factors that affect immune dynamics, several studies have concluded that those who reported higher rates of adverse reactions had higher peak values of humoral and cellular immunity post-administration [102]–[105]. Specifically, compared to local adverse reactions, individuals who experienced multiple systemic side effects including fever, fatigue, and muscle and joint pain achieved higher antibody titers. One of the attributions of these events could be that the severity of systemic adverse reactions post-vaccination enhances the ability to maintain higher antibody titers against the virus [105].
From our current understanding, in the prevention of infection by pathogens such as SARS-CoV-2, a key role is played by the presence of higher titers of neutralizing antibodies. However, the evasion of the severity of infection is found to play a role in both virus binding specific antibodies and pathogens targeting T and B-cells [106]. Thus, a higher frequency of post-immunization adverse reactions indicates a stronger inflammatory response, which can be the triggering factor for antibody induction and the development of a long-term immune memory [107]. On the other hand, the absence of such side effects does not necessarily mean decreased protection, since their occurrence is influenced by a number of different biological and environmental factors [13],[108]–[110].
Immunosenescence is defined as the destruction or remodeling of the immune organ structure along with dysfunction of the innate and adaptive immune systems, which leads to enhanced disease susceptibility and poor vaccination or treatment outcomes [111]. Being a complex process with multiple reorganizational and developmentally regulated changes presented in Figure 3, immunosenescence is more than just a unidirectional decline in the immune system. The complete mechanism of this functional decline is still unknown; however, the principle hallmark of immunosenescence has been connected to thymic involution, which leads to the reduced generation of naïve T-cells [111],[112].
Thymic involution is referred to as a structural alteration, as well as the functional decline of the thymus with age. Since the organ has a primary function in T-lymphocyte maturation, progressive thymic atrophy reduces the generation of new T-cells, which leads to the accumulation of memory T-cells [113]. This leads to a reduction in the diversity of T-lymphocytes, along with the available TCRs. Ageing related thymic atrophy is characterized by reduced cellularity (i.e., size and thymocyte number), with the observed accumulation of fibroblasts and adipose tissues in the thymic microstructure. In addition, the presence of senescent cells contributes to an increased thymic inflammatory environment [114].
This age-dependent accumulation of senescent cells and their secretory products, including pro-inflammatory cytokines and chemokines, also contributes to another age-related mechanism referred to as inflammageing [115]. Inflammageing is a state of chronic antigen load and low-grade inflammation, even in the absence of acute infection, and has been implicated in several age-related inflammatory diseases [112]. Similarly, age-related changes in the bone marrow microenvironment reduce the levels of the pro-B-cell survival cytokine interleukin-7, which affects the production of naïve B-cells [115],[116]. Moreover, HSCs dysfunction results in lower frequencies of common lymphoid progenitors, which also contributes to the reduced production of new T and B lymphocytes. Innate immunity becomes aged with the reduced pro-inflammatory responses of antigen presenting cells (dendritic cells and macrophages) to infections and vaccines [91],[115]. Additionally, the antigen uptake and antigen presenting capacity of these primary immune defense agents decline [117].
Thus, these age-related changes in the immune system make the older population vulnerable to severe diseases and impair the development of immunological memory to the administered vaccines. The age-related changes that occur in the innate and adaptive immune systems compromise the response to antigens. With the diminished response of the innate immune system, activation of the adaptive immune response also weakens. Furthermore, the reduced repertoire of TCRs to combat newly encountered antigens and stimulate the humoral immune response makes it difficult to limit any newly encountered foreign agent in time [111],[113].
Another concern is the maintenance of effective long-term memory in response to such encounters for future protection against specific pathogens. The efficacy of immunological memory depends on the primary immune response of the host. As it is diminished in elderly persons, so does the developed immune memory with the produced antibodies to have fewer neutralizing and opsonizing functions [117]. The bone marrow is the preferred survival niche for the produced effector memory T cells and plasma cells to show reduced production of cytokines/chemokines necessary for the survival of these cells. Increased adiposity due to suppressed fatty-acid oxidation and reduced amino acid and nucleotides contribute to major changes in the metabolome of the ageing bone marrow [118]. Additionally, ageing affects the survival of developed memory immune cells in the bone marrow, which makes the maintenance of immunological memory in older populations difficult [98].
With the ever-improving living standards of communities, human life expectancy has increased. This leads to the ageing of the population with diminished physical and physiological functions. Hence, the risk of infection and treatment failure increases, which further increases the possibility of disease recurrence. Different types of SARS-CoV-2 vaccines have shown good safety and efficacy in all age groups [38]. However, vaccines can cause different side effects because of the immune response or the presence of any disease or other individual factors. The successful prevention of diseases such as COVID-19 demands diligent campaigns and effective vaccination responses to prevent the incidence of infection and to mitigate the crisis caused by such outbreaks.
With an efficient immune system, the younger population not only performs well against infections compared to the elderly, but also responds more efficiently to vaccines in developing long-term immunity. Therefore, the immune system and vaccine responses are two correlated parameters that have a profound impact on the development of effective memory against related pathogens [119]. The differentiation of T and B lymphocytes and reduced functional capabilities of dendritic cells and macrophages, with an emphasis on T-cell immunosenescence, are perceived to be the basic hindrance in gaining an effective immune response against any infection and vaccines in older persons. We know this from the experience of annual influenza vaccines, which are only 30–40% effective in this high risk population [120]. In addition, the incidence and case fatality ratios of COVID-19 were higher in countries with large elderly populations [121].
Vaccines should be highly effective against this age group. It requires the alleviation of immunosenescence and age-related health issues by understanding the process of biological ageing. This loss of physiological reserves due to processes such as cellular senescence is accompanied by the hoarding of dysfunctional cells secreting tissue-decaying proteolytic enzymes, pro-inflammatory cytokines (inflammageing), and chemokines, which cause local and systemic consequences. Immune cell senescence (e.g., can be due to developmental fatigue) contributes to thymic atrophy (loss in cellularity of the thymus), which results in the loss of naive T-cells and worsens the age-related immunity decline to novel pathogens and vaccines [122].
The process of ageing is complex and irreversible; however, trials are ongoing to reduce its impact on the functional capabilities of a person, including their immune systems. These include drugs called geroprotectors. These drugs belong to the senolytic class and have been shown to counteract functional loss in different organ systems of the elderly by selectively clearing senescent cells. In preclinical trials, these drugs have reduced the severity and complications due to ageing in different infectious diseases and disorders and have also improved vaccine responses by lowering the detrimental effects of senescent cells and maintaining an efficient immune system [123]. Trials of RTB101 and other mTOR (mechanistic target of rapamycin that regulates different cellular growth and developmental processes) inhibitors are ongoing to increase the effectiveness of the vaccine for the elderly and to help prevent diseases [66].
We need to add more elderly participants and people with multi-morbidities in testing trials of drugs and vaccines against diseases such as COVID-19. Randomized controlled trials of geroprotectors are essential as adjuvant therapies to enhance vaccine responses and to improve the immunities of older people who are at a higher risk of contracting infections such as COVID-19 [66]. The consideration of senolytic drug usage for short term treatments providing long term protection is also available; however, the safety of these drugs is yet to be determined and more research with focused efforts is required [120]. Meanwhile, we need to address other factors that influence the functioning of the immune system, including host nutritional and behavioral factors, and encourage an active and healthy lifestyle to keep the body systems fit to combat any ailment and to develop stronger and lasting memories against vaccines [124].
It has been demonstrated that regular physical activity positively influences both the innate and adaptive immune systems in older individuals [125],[126]. Improvements in immune cell functioning and a reduction in senescent cells have been observed in physically active individuals. Moreover, exercise training seems to act as an anti-inflammatory therapy, which reduces the different inflammatory proteins in sera, thereby alleviating inflammageing [125]. There is compelling evidence that malnourished elderly individuals have poor innate and adaptive immune responses to infections [127]. These lifestyle interventions may help attain the desired immune responses with the least vaccine related complications in the elderly population. More intensive research is required on these strategies. Similarly, specific vaccine associated measures are recommended for frail immunocompromised individuals to make vaccinations as effective as possible. These include the use of adjuvants, changing the route of administration, and changing the vaccine composition for the immunization of elderly individuals [128].
Vaccines are the best tools to fight diseases such as COVID-19. They have some associated adverse reactions, which are determined by many factors including age of the recipient. Adverse reactions to SARS-CoV-2 vaccines were found to be more common among younger than older adults because of the efficient immune system of younger individuals. The immune system becomes less efficient with age, which is known as immunosenescence. This affects the development and maintenance of the immunological memory in response to the administered vaccines. Specific strategies are required to elevate the protection provided by vaccines in older people, which is mandatory to deal with the disease burden.
The authors declare they have not used Artificial Intelligence (AI) tools in the creation of this article.
[1] |
Abdul-Fattah S, Pal A, Kaka N, et al. (2021) History and recent advances in coronavirus discovery. In Silico Modeling of Drugs Against Coronaviruses. Berlin: Springer 3-24. https://doi.org/10.1007/7653_2020_47 ![]() |
[2] |
Li YD, Chi WY, Su JH, et al. (2020) Coronavirus vaccine development: From SARS and MERS to COVID-19. J Biomed Sci 27: 104. https://doi.org/10.1186/s12929-020-00695-2 ![]() |
[3] | Umakanthan S, Sahu P, Ranade A V, et al. (2020) Origin, transmission, diagnosis and management of coronavirus disease 2019 (COVID-19). Postgrad Med J 96: 753-758. https://doi.org/10.1136/postgradmedj-2020-138234 |
[4] |
Dong E, Du H, Gardner L (2020) An interactive web-based dashboard to track COVID-19 in real time. Lancet Infect Dis 20: 533-534. https://doi.org/10.1016/S1473-3099(20)30120-1 ![]() |
[5] |
Matsumura T, Takano T, Takahashi Y (2023) Immune responses related to the immunogenicity and reactogenicity of COVID-19 mRNA vaccines. Int Immunol 35: 213-220. https://doi.org/10.1093/intimm/dxac064 ![]() |
[6] |
Zheng C, Shao W, Chen X, et al. (2022) Real-world effectiveness of COVID-19 vaccines: A literature review and meta-analysis. Int J Infect Dis 114: 252-260. https://doi.org/10.1016/j.ijid.2021.11.009 ![]() |
[7] |
Wise J (2023) Covid-19: WHO declares end of global health emergency. BMJ 381: 1041. https://doi.org/10.1136/bmj.p1041 ![]() |
[8] |
Matrajt L, Eaton J, Leung T, et al. (2021) Vaccine optimization for COVID-19: Who to vaccinate first?. Sci Adv 7: eabf1374. https://doi.org/10.1126/sciadv.abf1374 ![]() |
[9] | Ruiz Estrada MA (2021) The COVID-19 natural selection by age. SSRN Electron J . https://doi.org/10.2139/ssrn.3773704 |
[10] | Azwar MK, Setiati S, Rizka A, et al. (2020) Clinical profile of elderly patients with COVID-19 hospitalised in Indonesia's national general hospital. Acta Med Indones 52: 199-205. |
[11] |
Witkowski JM, Fulop T, Bryl E (2022) Immunosenescence and COVID-19. Mech Ageing Dev 204: 111672. https://doi.org/10.1016/j.mad.2022.111672 ![]() |
[12] |
Wang J, Tong Y, Li D, et al. (2021) The impact of age difference on the efficacy and safety of COVID-19 vaccines: A systematic review and meta-analysis. Front Immunol 12: 758294. https://doi.org/10.3389/fimmu.2021.758294 ![]() |
[13] |
Hervé C, Laupèze B, Del Giudice G, et al. (2019) The how's and what's of vaccine reactogenicity. Npj Vaccines 4: 39. https://doi.org/10.1038/s41541-019-0132-6 ![]() |
[14] |
Sprent J, King C (2021) COVID-19 vaccine side effects: The positives about feeling bad. Sci Immunol 6: 1-4. https://doi.org/10.1126/sciimmunol.abj9256 ![]() |
[15] |
Sutton N, San Francisco Ramos A, Beales E, et al. (2022) Comparing reactogenicity of COVID-19 vaccines: A systematic review and meta-analysis. Expert Rev Vaccines 21: 1301-1318. https://doi.org/10.1080/14760584.2022.2098719 ![]() |
[16] |
Palanica A, Jeon J (2022) Initial mix-and-match COVID-19 vaccination perceptions, concerns, and side effects across Canadians. Vaccines 10: 93. https://doi.org/10.3390/vaccines10010093 ![]() |
[17] |
Atyabi SMH, Rommasi F, Ramezani MH, et al. (2022) Relationship between blood clots and COVID-19 vaccines: A literature review. Open Life Sci 17: 401-415. https://doi.org/10.1515/biol-2022-0035 ![]() |
[18] |
Dhamanti I, Suwantika AA, Adlia A, et al. (2023) Adverse reactions of COVID-19 vaccines: A scoping review of observational studies. Int J Gen Med 16: 609-618. https://doi.org/10.2147/IJGM.S400458 ![]() |
[19] |
Segni MT, Demissie HF, Adem MK, et al. (2022) Post COVID-19 vaccination side effects and associated factors among vaccinated health care providers in Oromia region, Ethiopia in 2021. PLoS One 17: e0278334. https://doi.org/10.1371/journal.pone.0278334 ![]() |
[20] |
Villanueva P, McDonald E, Croda J, et al. (2024) Factors influencing adverse events following COVID-19 vaccination. Hum Vaccin Immunother 20. https://doi.org/10.1080/21645515.2024.2323853 ![]() |
[21] |
Abbaspour S, Robbins GK, Blumenthal KG, et al. (2022) Identifying modifiable predictors of COVID-19 vaccine side effects: A machine learning approach. Vaccines 10: 1747. https://doi.org/10.3390/vaccines10101747 ![]() |
[22] |
George A, Goble HM, Garlapati S, et al. (2023) Demographic and lifestyle factors associated with patient-reported acute COVID-19 vaccine reactivity. Vaccines 11: 1072. https://doi.org/10.3390/vaccines11061072 ![]() |
[23] |
Tran VN, Nguyen HA, Le TTA, et al. (2021) Factors influencing adverse events following immunization with AZD1222 in Vietnamese adults during first half of 2021. Vaccine 39: 6485-6491. https://doi.org/10.1016/j.vaccine.2021.09.060 ![]() |
[24] |
Mori M, Yokoyama A, Shichida A, et al. (2023) Impact of sex and age on vaccine-related side effects and their progression after booster mRNA COVID-19 vaccine. Sci Rep 13: 19328. https://doi.org/10.1038/s41598-023-46823-4 ![]() |
[25] |
Gonzalez-Dias P, Lee EK, Sorgi S, et al. (2020) Methods for predicting vaccine immunogenicity and reactogenicity. Hum Vaccin Immunother 16: 269-276. https://doi.org/10.1080/21645515.2019.1697110 ![]() |
[26] |
Zhuang CL, Lin ZJ, Bi ZF, et al. (2021) Inflammation-related adverse reactions following vaccination potentially indicate a stronger immune response. Emerg Microbes Infect 10: 365-375. https://doi.org/10.1080/22221751.2021.1891002 ![]() |
[27] |
Pollard AJ, Bijker EM (2021) A guide to vaccinology: from basic principles to new developments. Nat Rev Immunol 21: 83-100. https://doi.org/10.1038/s41577-020-00479-7 ![]() |
[28] |
Li D, Wu M (2021) Pattern recognition receptors in health and diseases. Signal Transduction Targeted Ther 6: 291. https://doi.org/10.1038/s41392-021-00687-0 ![]() |
[29] |
Lacagnina MJ, Watkins LR, Grace PM (2018) Toll-like receptors and their role in persistent pain. Pharmacol Ther 184: 145-158. https://doi.org/10.1038/s41392-021-00687-0 ![]() |
[30] |
Primorac D, Vrdoljak K, Brlek P, et al. (2022) Adaptive immune responses and immunity to SARS-CoV-2. Front Immunol 13. https://doi.org/10.3389/fimmu.2022.848582 ![]() |
[31] |
Destexhe E, Prinsen MK, van Schöll I, et al. (2013) Evaluation of C-reactive protein as an inflammatory biomarker in rabbits for vaccine nonclinical safety studies. J Pharmacol Toxicol Methods 68: 367-373. https://doi.org/10.1016/j.vascn.2013.04.003 ![]() |
[32] |
Levy I, Levin EG, Olmer L, et al. (2022) Correlation between adverse events and antibody titers among healthcare workers vaccinated with BNT162b2 mRNA COVID-19 vaccine. Vaccines 10: 1220. https://doi.org/10.3390/vaccines10081220 ![]() |
[33] |
Grifoni A, Weiskopf D, Ramirez SI, et al. (2020) Targets of T cell responses to SARS-CoV-2 coronavirus in humans with COVID-19 disease and unexposed individuals. Cell 181: 1489-1501.e15. https://doi.org/10.1016/j.cell.2020.05.015 ![]() |
[34] |
Sette A, Crotty S (2022) Immunological memory to SARS-CoV-2 infection and COVID-19 vaccines. Immunol Rev 310: 27-46. https://doi.org/10.1111/imr.13089 ![]() |
[35] |
Bugya Z, Prechl J, Szénási T, et al. (2021) Multiple levels of immunological memory and their association with vaccination. Vaccines 9: 174. https://doi.org/10.3390/vaccines9020174 ![]() |
[36] |
Wherry EJ, Barouch DH (2022) T cell immunity to COVID-19 vaccines. Science 377: 821-822. https://doi.org/10.1126/science.add2897 ![]() |
[37] |
Cohen KW, Linderman SL, Moodie Z, et al. (2021) Longitudinal analysis shows durable and broad immune memory after SARS-CoV-2 infection with persisting antibody responses and memory B and T cells. Cell Reports Med 2: 100354. https://doi.org/10.1016/j.xcrm.2021.100354 ![]() |
[38] |
Chen WH, Strych U, Hotez PJ, et al. (2020) The SARS-CoV-2 vaccine pipeline: An overview. Curr Trop Med Reports 7: 61-64. https://doi.org/10.1007/s40475-020-00201-6 ![]() |
[39] |
Ndwandwe D, Wiysonge CS (2021) COVID-19 vaccines. Curr Opin Immunol 71: 111-116. https://doi.org/10.1016/j.coi.2021.07.003 ![]() |
[40] |
Rueda-Fernández M, Melguizo-Rodríguez L, Costela-Ruiz VJ, et al. (2022) The current status of COVID-19 vaccines. A scoping review. Drug Discov Today 27: 103336. https://doi.org/10.1016/j.drudis.2022.08.004 ![]() |
[41] |
Kyriakidis NC, López-Cortés A, González EV, et al. (2021) SARS-CoV-2 vaccines strategies: A comprehensive review of phase 3 candidates. Npj Vaccines 6: 28. https://doi.org/10.1038/s41541-021-00292-w ![]() |
[42] |
Bok K, Sitar S, Graham BS, et al. (2021) Accelerated COVID-19 vaccine development: Milestones, lessons, and prospects. Immunity 54: 1636-1651. https://doi.org/10.1016/j.immuni.2021.07.017 ![]() |
[43] |
Santi Laurini G, Montanaro N, Broccoli M, et al. (2023) Real-life safety profile of mRNA vaccines for COVID-19: An analysis of VAERS database. Vaccine 41: 2879-2886. https://doi.org/10.1016/j.vaccine.2023.03.054 ![]() |
[44] |
Chapin-Bardales J, Myers T, Gee J, et al. (2021) Reactogenicity within 2 weeks after mRNA COVID-19 vaccines: Findings from the CDC v-safe surveillance system. Vaccine 39: 7066-7073. https://doi.org/10.1016/j.vaccine.2021.10.019 ![]() |
[45] |
Faksova K, Walsh D, Jiang Y, et al. (2024) COVID-19 vaccines and adverse events of special interest: A multinational Global Vaccine Data Network (GVDN) cohort study of 99 million vaccinated individuals. Vaccine 42: 2200-2211. https://doi.org/10.1016/j.vaccine.2024.01.100 ![]() |
[46] | GOV.UKArchive: Information for UK recipients on COVID-19 Vaccine AstraZeneca (Regulation 174) (2023). Available from: https://www.gov.uk/government/publications/regulatory-approval-of-covid-19-vaccine-astrazeneca/information-for-uk-recipients-on-covid-19-vaccine-astrazeneca#possible-side-effects |
[47] |
Raethke M, van Hunsel F, Thurin NH, et al. (2023) Cohort event monitoring of adverse reactions to COVID-19 vaccines in seven European countries: Pooled results on first dose. Drug Saf 46: 391-404. https://doi.org/10.1007/s40264-023-01281-9 ![]() |
[48] | Johnson & JohnsonJohnson & Johnson updates U.S. COVID-19 vaccine fact sheet (2022). Available from: https://www.jnj.com/media-center/press-releases/johnson-johnson-updates-u-s-covid-19-vaccine-fact-sheet |
[49] |
Amer SA, Al-Zahrani A, Imam EA, et al. (2024) Exploring the reported adverse effects of COVID-19 vaccines among vaccinated Arab populations: A multi-national survey study. Sci Rep 14: 4785. https://doi.org/10.1038/s41598-024-54886-0 ![]() |
[50] |
Akrami M, Hosamirudsari H, Faraji N, et al. (2023) Sputnik V vaccine-related complications and its impression on inflammatory biomarkers in healthcare providers. Indian J Med Microbiol 43: 79-84. https://doi.org/10.1016/j.ijmmb.2022.10.012 ![]() |
[51] |
Granados Villalpando JM, Romero Tapia S de J, Baeza Flores G del C, et al. (2022) Prevalence and risk factors of adverse effects and allergic reactions after COVID-19 vaccines in a Mexican population: An analytical cross-sectional study. Vaccines 10: 2012. https://doi.org/10.3390/vaccines10122012 ![]() |
[52] |
Hasan SS, Rashid A, Osama S, et al. (2022) Covid-19 vaccine safety and adverse event analysis from Pakistan. Clin Immunol Commun 2: 91-97. https://doi.org/10.1016/j.clicom.2022.05.003 ![]() |
[53] |
Mohebbi A, Eterafi M, Fouladi N, et al. (2023) Adverse effects reported and insights following Sinopharm COVID-19 vaccination. Curr Microbiol 80: 377. https://doi.org/10.1007/s00284-023-03432-8 ![]() |
[54] |
Abu-Halaweh S, Alqassieh R, Suleiman A, et al. (2021) Qualitative assessment of early adverse effects of Pfizer–BioNTech and Sinopharm COVID-19 vaccines by telephone interviews. Vaccines 9: 950. https://doi.org/10.3390/vaccines9090950 ![]() |
[55] |
Lai FTT, Leung MTY, Chan EWW, et al. (2022) Self-reported reactogenicity of CoronaVac (Sinovac) compared with Comirnaty (Pfizer-BioNTech): A prospective cohort study with intensive monitoring. Vaccine 40: 1390-1396. https://doi.org/10.1016/j.vaccine.2022.01.062 ![]() |
[56] |
Smith K, Hegazy K, Cai MR, et al. (2023) Safety of the NVX-CoV2373 COVID-19 vaccine in randomized placebo-controlled clinical trials. Vaccine 41: 3930-3936. https://doi.org/10.1016/j.vaccine.2023.05.016 ![]() |
[57] |
Xiong X, Yuan J, Li M, et al. (2021) Age and gender disparities in adverse events following COVID-19 vaccination: Real-world evidence based on big data for risk management. Front Med 8: 700014. https://doi.org/10.3389/fmed.2021.700014 ![]() |
[58] |
Elnaem MH, Mohd Taufek NH, Ab Rahman NS, et al. (2021) COVID-19 vaccination attitudes, perceptions, and side effect experiences in Malaysia: Do age, gender, and vaccine type matter?. Vaccines 9: 1156. https://doi.org/10.3390/vaccines9101156 ![]() |
[59] | UPMC Health BeatHow age and sex couid affect COVID vaccine side effects (2021). Available from: https://share.upmc.com/2021/04/age-and-sex-covid-19-vaccine/ |
[60] |
Sultana A, Shahriar S, Tahsin MR, et al. (2021) A retrospective cross-sectional study assessing self-reported adverse events following immunization (AEFI) of the COVID-19 vaccine in Bangladesh. Vaccines 9: 1090. https://doi.org/10.3390/vaccines9101090 ![]() |
[61] |
Saeed BQ, Al-Shahrabi R, Alhaj SS, et al. (2021) Side effects and perceptions following Sinopharm COVID-19 vaccination. Int J Infect Dis 111: 219-226. https://doi.org/10.1016/j.ijid.2021.08.013 ![]() |
[62] |
Enayatrad M, Mahdavi S, Aliyari R, et al. (2023) Reactogenicity within the first week after Sinopharm, Sputnik V, AZD1222, and COVIran Barekat vaccines: Findings from the Iranian active vaccine surveillance system. BMC Infect Dis 23: 150. https://doi.org/10.1186/s12879-023-08103-4 ![]() |
[63] | Pagotto V, Ferloni A, Soriano MM, et al. (2021) Active surveillance of the Sputnik V vaccine in health workers. medRxiv : 2021.02.03.21251071. https://doi.org/10.1101/2021.02.03.21251071 |
[64] |
Weintraub ES, Oster ME, Klein NP (2022) Myocarditis or pericarditis following mRNA COVID-19 vaccination. JAMA Netw Open 5: e2218512. https://doi.org/10.1001/jamanetworkopen.2022.18512 ![]() |
[65] |
Buchan SA, Seo CY, Johnson C, et al. (2022) Epidemiology of myocarditis and pericarditis following mRNA vaccination by vaccine product, schedule, and interdose interval among adolescents and adults in ontario, Canada. JAMA Netw Open 5: e2218505. https://doi.org/10.1001/jamanetworkopen.2022.18505 ![]() |
[66] |
Wise J (2021) Covid-19: European countries suspend use of Oxford-AstraZeneca vaccine after reports of blood clots. BMJ 372: n699. https://doi.org/10.1136/bmj.n699 ![]() |
[67] |
Ghorbani H, Rouhi T, Vosough Z, et al. (2022) Drug-induced hepatitis after Sinopharm COVID-19 vaccination: A case study of a 62-year-old patient. Int J Surg Case Rep 93: 106926. https://doi.org/10.1016/j.ijscr.2022.106926 ![]() |
[68] |
Bril F, Al Diffalha S, Dean M, et al. (2021) Autoimmune hepatitis developing after coronavirus disease 2019 (COVID-19) vaccine: Causality or casualty?. J Hepatol 75: 222-224. https://doi.org/10.1016/j.jhep.2021.04.003 ![]() |
[69] |
Clayton-Chubb D, Schneider D, Freeman E, et al. (2021) Autoimmune hepatitis developing after the ChAdOx1 nCoV-19 (Oxford-AstraZeneca) vaccine. J Hepatol 75: 1249-1250. https://doi.org/10.1016/j.jhep.2021.06.014 ![]() |
[70] |
Beatty AL, Peyser ND, Butcher XE, et al. (2021) Analysis of COVID-19 vaccine type and adverse effects following vaccination. JAMA Netw Open 4: e2140364. https://doi.org/10.1001/jamanetworkopen.2021.40364 ![]() |
[71] |
Oh SJ, Lee JK, Shin OS (2019) Aging and the immune system: The impact of immunosenescence on viral infection, immunity and vaccine immunogenicity. Immune Netw 19: e37. https://doi.org/10.4110/in.2019.19.e37 ![]() |
[72] |
Ciocca M, Zaffina S, Fernandez Salinas A, et al. (2021) Evolution of human memory B cells from childhood to old age. Front Immunol 12: 690534. https://doi.org/10.3389/fimmu.2021.690534 ![]() |
[73] |
Collier DA, Ferreira IATM, Kotagiri P, et al. (2021) Age-related immune response heterogeneity to SARS-CoV-2 vaccine BNT162b2. Nature 596: 417-422. https://doi.org/10.1038/s41586-021-03739-1 ![]() |
[74] |
Yousfi M El, Mercier S, Breuillé D, et al. (2005) The inflammatory response to vaccination is altered in the elderly. Mech Ageing Dev 126: 874-881. https://doi.org/10.1016/j.mad.2005.03.008 ![]() |
[75] |
Hajjo R, Sabbah DA, Bardaweel SK, et al. (2021) Shedding the light on post-vaccine myocarditis and pericarditis in COVID-19 and Non-COVID-19 vaccine recipients. Vaccines 9: 1186. https://doi.org/10.3390/vaccines9101186 ![]() |
[76] |
Lazaros G, Klein AL, Hatziantoniou S, et al. (2021) The novel platform of mRNA COVID-19 vaccines and myocarditis: Clues into the potential underlying mechanism. Vaccine 39: 4925-4927. https://doi.org/10.1016/j.vaccine.2021.07.016 ![]() |
[77] |
Bozkurt B, Kamat I, Hotez PJ (2021) Myocarditis with COVID-19 mRNA vaccines. Circulation 144: 471-484. https://doi.org/10.1161/CIRCULATIONAHA.121.056135 ![]() |
[78] |
Klok FA, Pai M, Huisman MV, et al. (2022) Vaccine-induced immune thrombotic thrombocytopenia. Lancet Haematol 9: e73-e80. https://doi.org/10.1016/S2352-3026(21)00306-9 ![]() |
[79] |
Afshar ZM, Barary M, Babazadeh A, et al. (2022) SARS-CoV-2-related and Covid-19 vaccine-induced thromboembolic events: A comparative review. Rev Med Virol 32: e2327. https://doi.org/10.1002/rmv.2327 ![]() |
[80] |
Mani A, Ojha V (2022) Thromboembolism after COVID-19 vaccination: A systematic review of such events in 286 patients. Ann Vasc Surg 84: 12-20.e1. https://doi.org/10.1016/j.avsg.2022.05.001 ![]() |
[81] |
Avci E, Abasiyanik F (2021) Autoimmune hepatitis after SARS-CoV-2 vaccine: New-onset or flare-up?. J Autoimmun 125: 102745. https://doi.org/10.1016/j.jaut.2021.102745 ![]() |
[82] |
Palla P, Vergadis C, Sakellariou S, et al. (2022) Letter to the editor: Autoimmune hepatitis after COVID-19 vaccination: A rare adverse effect?. Hepatology 75: 489-490. https://doi.org/10.1002/hep.32156 ![]() |
[83] |
Vuille-Lessard É, Montani M, Bosch J, et al. (2021) Autoimmune hepatitis triggered by SARS-CoV-2 vaccination. J Autoimmun 123: 102710. https://doi.org/10.1016/j.jaut.2021.102710 ![]() |
[84] |
Efe C, Kulkarni AV, Terziroli Beretta-Piccoli B, et al. (2022) Liver injury after SARS-CoV-2 vaccination: Features of immune-mediated hepatitis, role of corticosteroid therapy and outcome. Hepatology 76: 1576-1586. https://doi.org/10.1002/hep.32572 ![]() |
[85] |
Torjesen I (2021) Covid-19: Doctors in Norway told to assess severely frail patients for vaccination. BMJ 372: n167. https://doi.org/10.1136/bmj.n167 ![]() |
[86] |
Edler C, Klein A, Schröder AS, et al. (2021) Deaths associated with newly launched SARS-CoV-2 vaccination (Comirnaty®). Leg Med 51: 101895. https://doi.org/10.1016/j.legalmed.2021.101895 ![]() |
[87] |
Torjesen I (2021) Covid-19: Norway investigates 23 deaths in frail elderly patients after vaccination. BMJ 372: n149. https://doi.org/10.1136/bmj.n149 ![]() |
[88] |
Lee KA, Flores RR, Jang IH, et al. (2022) Immune senescence, immunosenescence and aging. Front Aging 3: 900028. https://doi.org/10.3389/fragi.2022.900028 ![]() |
[89] |
Huang W, Hickson LJ, Eirin A, et al. (2022) Cellular senescence: The good, the bad and the unknown. Nat Rev Nephrol 18: 611-627. https://doi.org/10.1038/s41581-022-00601-z ![]() |
[90] |
Katuuk DA, Lengkong JSJ, Rotty VNJ, et al. (2021) Management of Covid-19 vaccination for the elderly. BIRCI J 4: 2121-2134. https://doi.org/10.33258/birci.v4i2.1902 2121 ![]() |
[91] |
Agrawal A, Weinberger B (2022) Editorial: The impact of immunosenescence and senescence of immune cells on responses to infection and vaccination. Front Aging 3: 882494. https://doi.org/10.3389/fragi.2022.882494 ![]() |
[92] |
Guidi N, Marka G, Sakk V, et al. (2021) An aged bone marrow niche restrains rejuvenated hematopoietic stem cells. Stem Cells 39: 1101-1106. https://doi.org/10.1002/stem.3372 ![]() |
[93] |
de Mol J, Kuiper J, Tsiantoulas D, et al. (2021) The dynamics of B cell aging in health and disease. Front Immunol 12: 733566. https://doi.org/10.3389/fimmu.2021.733566 ![]() |
[94] | Saridena A, Saridena A, Kethar J (2023) Reactions to SARS-CoV-2: The effectiveness of the body's immunological memory. J Student Res 12. https://doi.org/10.47611/jsrhs.v12i1.4359 |
[95] | Shittu A Understanding immunological memory (2023). Available from: https://asm.org/Articles/2023/May/Understanding-Immunological-Memory |
[96] |
Condotta SA, Richer MJ (2017) The immune battlefield: The impact of inflammatory cytokines on CD8+ T-cell immunity. PLOS Pathog 13: e1006618. https://doi.org/10.1371/journal.ppat.1006618 ![]() |
[97] |
Wakui M, Uwamino Y, Yatabe Y, et al. (2022) Assessing anti-SARS-CoV-2 cellular immunity in 571 vaccines by using an IFN-γ release assay. Eur J Immunol 52: 1961-1971. https://doi.org/10.1002/eji.202249794 ![]() |
[98] |
Lee HG, Cho MJ, Choi JM (2020) Bystander CD4+ T cells: Crossroads between innate and adaptive immunity. Exp Mol Med 52: 1255-1263. https://doi.org/10.1038/s12276-020-00486-7 ![]() |
[99] |
Martinez-Sanchez ME, Huerta L, Alvarez-Buylla ER, et al. (2018) Role of cytokine combinations on CD4+ T cell differentiation, partial polarization, and plasticity: Continuous network modeling approach. Front Physiol 9: 877. https://doi.org/10.3389/fphys.2018.00877 ![]() |
[100] |
Kedzierska K, Thomas PG (2022) Count on us: T cells in SARS-CoV-2 infection and vaccination. Cell Reports Med 3: 100562. https://doi.org/10.1016/j.xcrm.2022.100562 ![]() |
[101] |
Hall V, Foulkes S, Insalata F, et al. (2022) Protection against SARS-CoV-2 after Covid-19 vaccination and previous infection. N Engl J Med 386: 1207-1220. https://doi.org/10.1056/NEJMoa2118691 ![]() |
[102] |
Yoshida M, Kobashi Y, Kawamura T, et al. (2023) Association of systemic adverse reaction patterns with long-term dynamics of humoral and cellular immunity after coronavirus disease 2019 third vaccination. Sci Rep 13: 9264. https://doi.org/10.1038/s41598-023-36429-1 ![]() |
[103] |
Kobashi Y, Shimazu Y, Kawamura T, et al. (2022) Factors associated with anti-severe acute respiratory syndrome coronavirus 2 (SARS-CoV-2) spike protein antibody titer and neutralizing activity among healthcare workers following vaccination with the BNT162b2 vaccine. PLoS One 17: e0269917. https://doi.org/10.1371/journal.pone.0269917 ![]() |
[104] |
Naaber P, Tserel L, Kangro K, et al. (2021) Dynamics of antibody response to BNT162b2 vaccine after six months: a longitudinal prospective study. Lancet Reg Heal Eur 10: 100208. https://doi.org/10.1016/j.lanepe.2021.100208 ![]() |
[105] |
Koike R, Sawahata M, Nakamura Y, et al. (2022) Systemic adverse effects induced by the BNT162b2 vaccine are associated with higher antibody titers from 3 to 6 months after vaccination. Vaccines 10: 451. https://doi.org/10.3390/vaccines10030451 ![]() |
[106] |
Chi WY, Li YD, Huang HC, et al. (2022) COVID-19 vaccine update: Vaccine effectiveness, SARS-CoV-2 variants, boosters, adverse effects, and immune correlates of protection. J Biomed Sci 29: 82. https://doi.org/10.1186/s12929-022-00853-8 ![]() |
[107] |
Klingel H, Krüttgen A, Imöhl M, et al. (2023) Humoral immune response to SARS-CoV-2 mRNA vaccines is associated with choice of vaccine and systemic adverse reactions DMD TNR. Clin Exp Vaccine Res 12: 60-69. https://doi.org/10.7774/cevr.2023.12.1.60 ![]() |
[108] |
Hwang YH, Song KH, Choi Y, et al. (2021) Can reactogenicity predict immunogenicity after COVID-19 vaccination?. Korean J Intern Med 36: 1486-1491. https://doi.org/10.3904/kjim.2021.210 ![]() |
[109] |
Bauernfeind S, Salzberger B, Hitzenbichler F, et al. (2021) Association between reactogenicity and immunogenicity after vaccination with BNT162b2. Vaccines 9: 1089. https://doi.org/10.3390/vaccines9101089 ![]() |
[110] |
Lim SY, Kim JY, Park S, et al. (2021) Correlation between reactogenicity and immunogenicity after the ChAdOx1 nCoV-19 and BNT162b2 mRNA vaccination. Immune Netw 21: e41. https://doi.org/10.4110/in.2021.21.e41 ![]() |
[111] |
Liu Z, Liang Q, Ren Y, et al. (2023) Immunosenescence: Molecular mechanisms and diseases. Signal Transducttion Targeted Ther 8: 200. https://doi.org/10.1038/s41392-023-01451-2 ![]() |
[112] |
Accardi G, Caruso C (2018) Immune-inflammatory responses in the elderly: An update. Immun Ageing 15: 11. https://doi.org/10.1186/s12979-018-0117-8 ![]() |
[113] |
Thomas R, Wang W, Su DM (2020) Contributions of age-related thymic involution to immunosenescence and inflammaging. Immun Ageing 17: 2. https://doi.org/10.1186/s12979-018-0117-8 ![]() |
[114] | Thomas R, Su DM (2020) Age-related thymic atrophy: Mechanisms and outcomes. Thymus. Vienna: IntechOpen. https://doi.org/10.5772/intechopen.86412 |
[115] |
Frasca D, Diaz A, Romero M, et al. (2020) B cell immunosenescence. Annu Rev Cell Dev Biol 36: 551-574. https://doi.org/10.1146/annurev-cellbio-011620-034148 ![]() |
[116] |
Slamanig SA, Nolte MA (2021) The bone marrow as sanctuary for plasma cells and memory T-cells: Implications for adaptive immunity and vaccinology. Cells 10: 1508. https://doi.org/10.3390/cells10061508 ![]() |
[117] | Bowdish D, Loukov D, Naidoo A (2015) Immunosenescence: Implications for vaccination programs in the elderly. Vaccine Dev Ther 17. https://doi.org/10.2147/VDT.S63888 |
[118] |
Connor KM, Hsu Y, Aggarwal PK, et al. (2018) Understanding metabolic changes in aging bone marrow. Exp Hematol Oncol 7: 13. https://doi.org/10.1186/s40164-018-0105-x ![]() |
[119] |
Bajaj V, Gadi N, Spihlman AP, et al. (2021) Aging, immunity, and COVID-19: How age influences the host immune response to coronavirus infections?. Front Physiol 11: 571416. https://doi.org/10.3389/fphys.2020.571416 ![]() |
[120] |
Cox LS, Bellantuono I, Lord JM, et al. (2020) Tackling immunosenescence to improve COVID-19 outcomes and vaccine response in older adults. Lancet Heal Longev 1: e55-e57. https://doi.org/10.1016/S2666-7568(20)30011-8 ![]() |
[121] | Bardasi EM, Garcia G, Twigg J Covid-19 has exposed the fragilities of aging countries (2021). Available from: https://ieg.worldbankgroup.org/blog/covid-19-has-exposed-fragilities-aging-countries |
[122] |
Goronzy JJ, Weyand CM (2013) Understanding immunosenescence to improve responses to vaccines. Nat Immunol 14: 428-436. https://doi.org/10.1038/ni.2588 ![]() |
[123] |
Chaib S, Tchkonia T, Kirkland JL (2022) Cellular senescence and senolytics: The path to the clinic. Nat Med 28: 1556-1568. https://doi.org/10.1038/s41591-022-01923-y ![]() |
[124] |
Zimmermann P, Curtis N (2019) Factors that influence the immune response to vaccination. Clin Microbiol Rev 32. https://doi.org/10.1128/cmr.00084-18 ![]() |
[125] |
Weyh C, Krüger K, Strasser B (2020) Physical activity and diet shape the immune system during aging. Nutrients 12: 622. https://doi.org/10.3390/nu12030622 ![]() |
[126] |
Tylutka A, Morawin B, Gramacki A, et al. (2021) Lifestyle exercise attenuates immunosenescence; flow cytometry analysis. BMC Geriatr 21: 200. https://doi.org/10.1186/s12877-021-02128-7 ![]() |
[127] |
Bencivenga L, Rengo G, Varricchi G (2020) Elderly at time of COronaVIrus disease 2019 (COVID-19): Possible role of immunosenescence and malnutrition. GeroScience 42: 1089-1092. https://doi.org/10.1007/s11357-020-00218-9 ![]() |
[128] |
Lefebvre JS, Haynes L (2013) Vaccine strategies to enhance immune responses in the aged. Curr Opin Immunol 25: 523-528. https://doi.org/10.1016/j.coi.2013.05.014 ![]() |
Name | Type | Mode of action | Doses | Efficacy | Reported adverse events |
Pfizer-BioNTech (BNT162b2) | Nucleic acid | Contains mRNA sequence that codes for viral spike protein | ×2 | 89% | Non-serious common vaccine related AEs were reported with some rare incidences of cardiac inflammation and anaphylactic reactions [43],[44]. |
Moderna (mRNA–1273) | Nucleic acid | RNA segment coding for viral spike protein | ×2 | 92% | Reported greater common vaccine AEs compared to BNT162b2 vaccine particularly after both doses. The reports of severe vaccine reactions were lower than BNT162b2 vaccinees [43],[44]. |
AstraZeneca (ChAdOx1) | Viral Vector | Contains genetically modified adenovirus that cannot replicate but can code for SARS-CoV-2 proteins | ×2 | 62%–90% | Mild and short-term common vaccine local and systemic adverse reactions were reported. Some severe adverse reactions including venous thromboembolic events and Guillain–Barré syndrome (GBS) were also found to be the potential safety signals for ChAdOx1 particularly after first dose [45],[46]. |
Janssen | Viral Vector | A non-replicating virus transports the genetic material of SARS-CoV-2 virus | ×1 | 66% | Minor vaccine adverse events of local to general systemic reactions were reported with paresthesia or hypoesthesia, tinnitus and swollen lymph nodes. Rare incidences of blood clotting with thrombocytopenia and anaphylactic reactions were also reported but at a lower rate than ChAdOx1 vaccinees [47],[48]. |
Sputnik V | Viral Vector | A non-replicating virus transports the genetic material of SARS-CoV-2 virus | ×2 | 91% | Mild to moderate injection site and systemic reactions were reported. No severe post-administration complications were observed [49],[50]. |
CanSino | Viral Vector | Non-replicating adenovirus delivers the genetic material of SARS-CoV-2 virus | ×1 | 65% | Common vaccine adverse reactions were reported with a greater prevalence of systemic reactions of fever and fatigue than local adverse events. Some serious events of pneumonia were reported but found to be coincidental [51],[52]. |
Sinopharm | Inactivated | Contains killed virus particles | ×2 | 79% | Mild general vaccine adverse reactions were reported mostly after the first dose. Some rare incidences of cardiac arrest and GBS were also reported. Overall shows a lower prevalence of AEs than mRNA and ChAdOx1 vaccines [52]–[54]. |
Sinovac | Inactivated | Have killed virus particles | ×2 | 51% | Non-serious AEs were observed at a lower rate than most of the other COVID-19 vaccines. Some severe incidences of myocardial infarction and allergic reactions were reported [52],[55]. |
Novavax | Protein Subunit | Contains Isolated virus particles | ×2 | 90% | Moderate adverse reactions with a higher prevalence of local symptoms were observed after both doses. Some cases of myocarditis/pericarditis were reported [56]. |
Name | Type | Mode of action | Doses | Efficacy | Reported adverse events |
Pfizer-BioNTech (BNT162b2) | Nucleic acid | Contains mRNA sequence that codes for viral spike protein | ×2 | 89% | Non-serious common vaccine related AEs were reported with some rare incidences of cardiac inflammation and anaphylactic reactions [43],[44]. |
Moderna (mRNA–1273) | Nucleic acid | RNA segment coding for viral spike protein | ×2 | 92% | Reported greater common vaccine AEs compared to BNT162b2 vaccine particularly after both doses. The reports of severe vaccine reactions were lower than BNT162b2 vaccinees [43],[44]. |
AstraZeneca (ChAdOx1) | Viral Vector | Contains genetically modified adenovirus that cannot replicate but can code for SARS-CoV-2 proteins | ×2 | 62%–90% | Mild and short-term common vaccine local and systemic adverse reactions were reported. Some severe adverse reactions including venous thromboembolic events and Guillain–Barré syndrome (GBS) were also found to be the potential safety signals for ChAdOx1 particularly after first dose [45],[46]. |
Janssen | Viral Vector | A non-replicating virus transports the genetic material of SARS-CoV-2 virus | ×1 | 66% | Minor vaccine adverse events of local to general systemic reactions were reported with paresthesia or hypoesthesia, tinnitus and swollen lymph nodes. Rare incidences of blood clotting with thrombocytopenia and anaphylactic reactions were also reported but at a lower rate than ChAdOx1 vaccinees [47],[48]. |
Sputnik V | Viral Vector | A non-replicating virus transports the genetic material of SARS-CoV-2 virus | ×2 | 91% | Mild to moderate injection site and systemic reactions were reported. No severe post-administration complications were observed [49],[50]. |
CanSino | Viral Vector | Non-replicating adenovirus delivers the genetic material of SARS-CoV-2 virus | ×1 | 65% | Common vaccine adverse reactions were reported with a greater prevalence of systemic reactions of fever and fatigue than local adverse events. Some serious events of pneumonia were reported but found to be coincidental [51],[52]. |
Sinopharm | Inactivated | Contains killed virus particles | ×2 | 79% | Mild general vaccine adverse reactions were reported mostly after the first dose. Some rare incidences of cardiac arrest and GBS were also reported. Overall shows a lower prevalence of AEs than mRNA and ChAdOx1 vaccines [52]–[54]. |
Sinovac | Inactivated | Have killed virus particles | ×2 | 51% | Non-serious AEs were observed at a lower rate than most of the other COVID-19 vaccines. Some severe incidences of myocardial infarction and allergic reactions were reported [52],[55]. |
Novavax | Protein Subunit | Contains Isolated virus particles | ×2 | 90% | Moderate adverse reactions with a higher prevalence of local symptoms were observed after both doses. Some cases of myocarditis/pericarditis were reported [56]. |