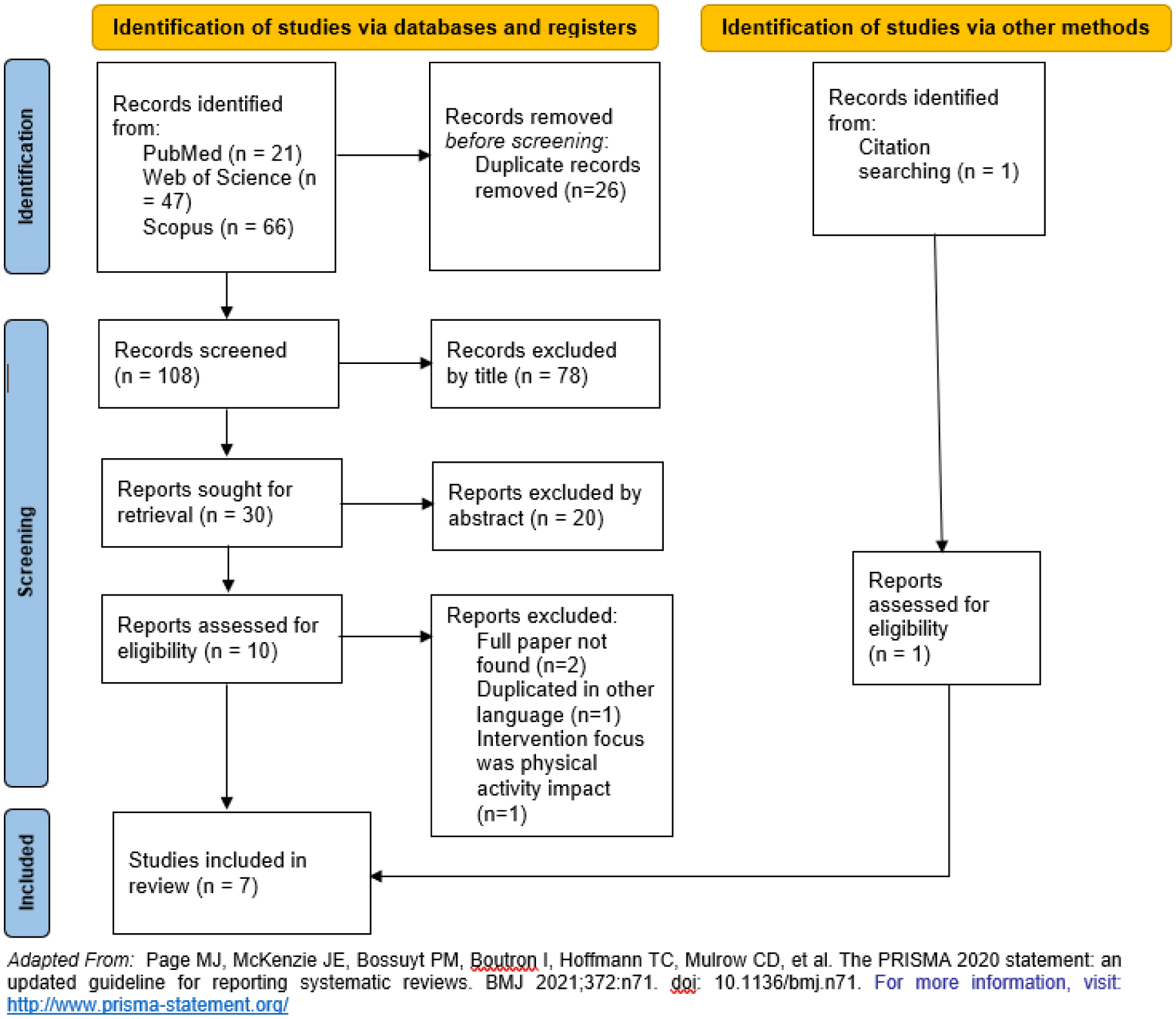
Postsynaptic protein neurogranin (Ng), which plays a role in synaptic plasticity, learning, and memory, has been identified as the candidate biomarker of Alzheimer's disease (AD). Cortical Amyloid β pathology seems to accelerate the onset of clinical symptoms; therefore, it is potentially valuable for early diagnosis of AD and therapeutic intervention. Synaptic pathology was shown to be an early feature of AD. Thus, proteins involved in synaptic function, such as Ng, are of great interest in studying the disease. Some prior human studies have found that Ng, a protein involved in the regulation of synaptic function, is present at greater levels in the cerebrospinal fluid of people with AD compared with those without the disease. High levels of neurogranin are associated with increased levels of synaptic vulnerability and decreased cognitive function in AD patients. This review, therefore, looked at the functionality of Ng in the brain, its association with other synaptic proteins, and its applicability as a diagnostic marker in AD. This study, therefore, sought to expand the knowledge on Ng changes in AD as it relates to synaptic dysfunction and enhanced the search for a better diagnostic and therapeutic approach.
Citation: Rajkumar Bavaharini, Chaitanya Sree Somala, Konda Mani Saravanan, Thirunavukarasou Anand. Neurogranin in Alzheimer's Disease: Roles in synaptic function, pathology, and potential as a diagnostic biomarker[J]. AIMS Molecular Science, 2024, 11(4): 330-350. doi: 10.3934/molsci.2024020
[1] | Domenico Giuffrè, Angelo Maria Giuffrè . Mediterranean diet and health in the elderly. AIMS Public Health, 2023, 10(3): 568-576. doi: 10.3934/publichealth.2023040 |
[2] | Marte K.R. Kjøllesdal, Gerd Holmboe-Ottesen . Dietary Patterns and Birth Weight—a Review. AIMS Public Health, 2014, 1(4): 211-225. doi: 10.3934/Publichealth.2014.4.211 |
[3] | Angela Groves, Wesley Browning . Peer (dyadic) support: a hypertension feasibility study for older African American women. AIMS Public Health, 2024, 11(3): 937-946. doi: 10.3934/publichealth.2024048 |
[4] | Eduardo Fricovsky, Mudassar Iqbal Arain, Binh Tran, Phuong Thao Nguyen, Tuyet Phan, Natalie Chang . Assessing the impact of a health education outreach project on cervical cancer awareness among Vietnamese-American women in San Diego. AIMS Public Health, 2022, 9(3): 552-558. doi: 10.3934/publichealth.2022038 |
[5] | Elizabeth Procter-Gray, Barbara Olendzki, Kevin Kane, Linda Churchill, Rashelle B. Hayes, Annabella Aguirre, Hyung-joo Kang, Wenjun Li . Comparison of Dietary Quality Assessment Using Food Frequency Questionnaire and 24-hour-recalls in Older Men and Women. AIMS Public Health, 2017, 4(4): 326-346. doi: 10.3934/publichealth.2017.4.326 |
[6] | Eleni L. Tolma, Kimberly Engelman, Julie A. Stoner, Cara Thomas, Stephanie Joseph, Ji Li, Cecily Blackwater, J. Neil Henderson, L. D. Carson, Norma Neely, Tewanna Edwards . The Design of a Multi-component Intervention to Promote Screening Mammography in an American Indian Community: The Native Women’s Health Project. AIMS Public Health, 2016, 3(4): 933-955. doi: 10.3934/publichealth.2016.4.933 |
[7] | Peter Oyovwe, Gillian Woolhead . Exploring health care professionals' and women's perspectives on the barriers to maternal health services: a qualitative study in Eku Town of Delta State, Nigeria. AIMS Public Health, 2021, 8(1): 154-171. doi: 10.3934/publichealth.2021012 |
[8] | Joyce Lo, Sharan Jaswal, Matthew Yeung, Vijay Kumar Chattu, Ali Bani-Fatemi, Aaron Howe, Amin Yazdani, Basem Gohar, Douglas P. Gross, Behdin Nowrouzi-Kia . A systematic review of the literature: Gender-based violence in the construction and natural resources industry. AIMS Public Health, 2024, 11(2): 654-666. doi: 10.3934/publichealth.2024033 |
[9] | Helga Hallgrimsdottir, Leah Shumka, Catherine Althaus, Cecilia Benoit . Fear, Risk, and the Responsible Choice: Risk Narratives and Lowering the Rate of Caesarean Sections in High-income Countries. AIMS Public Health, 2017, 4(6): 615-632. doi: 10.3934/publichealth.2017.6.615 |
[10] | Quynh Nhu (Natasha) B. La Frinere-Sandoval, Catherine Cubbin, Diana M. DiNitto . Perceived neighborhood social cohesion and cervical and breast cancer screening utilization among U.S.-born and immigrant women. AIMS Public Health, 2022, 9(3): 559-573. doi: 10.3934/publichealth.2022039 |
Postsynaptic protein neurogranin (Ng), which plays a role in synaptic plasticity, learning, and memory, has been identified as the candidate biomarker of Alzheimer's disease (AD). Cortical Amyloid β pathology seems to accelerate the onset of clinical symptoms; therefore, it is potentially valuable for early diagnosis of AD and therapeutic intervention. Synaptic pathology was shown to be an early feature of AD. Thus, proteins involved in synaptic function, such as Ng, are of great interest in studying the disease. Some prior human studies have found that Ng, a protein involved in the regulation of synaptic function, is present at greater levels in the cerebrospinal fluid of people with AD compared with those without the disease. High levels of neurogranin are associated with increased levels of synaptic vulnerability and decreased cognitive function in AD patients. This review, therefore, looked at the functionality of Ng in the brain, its association with other synaptic proteins, and its applicability as a diagnostic marker in AD. This study, therefore, sought to expand the knowledge on Ng changes in AD as it relates to synaptic dysfunction and enhanced the search for a better diagnostic and therapeutic approach.
Menopause is a physiological process marked by the date of a woman's last menstruation that indicates definitive ovarian failure. Menopause is diagnosed retrospectively after 12 consecutive months of amenorrhea without any other pathological or physiological cause [1]. The age at which menopause occurs, generally between 45 and 55 years old, is primarily determined by genetics but also susceptible to the influence of environmental factors such as obesity, multiparity, physical activity, tobacco use, and alcoholism [2].
For women, entering into menopause can be associated with an increased prevalence of obesity, metabolic syndrome, cardiovascular disease, and osteoporosis [3]. Against those risks, diet is particularly relevant given its impact on quality of life and longevity and its modifiability [4]. Because menopause is also viewed as a time in women's lives when they are more susceptible to changing habits and acquiring healthier behaviors [1], it presents an excellent opportunity for health interventions as well.
Of all components of lifestyle, nutrition exerts some of the most significant impacts on postmenopausal women's quality of life and morbidity. Higher adherence to the Mediterranean diet (MD) has been shown to improve the prevention and management of age-associated non-communicable diseases, including cardiovascular and metabolic diseases, neurodegenerative diseases, cancer, depression, respiratory diseases, and fragility fractures [5]. In turn, the MD may also reduce total mortality in the population [6] and lengthen lifespans [7]. Therefore, the MD, currently recognized as one of the healthiest dietary models worldwide [8], may offer many benefits to women in the climacteric phase of life. The MD is characterized by a relatively high intake of vegetable products (i.e., vegetables, fruit, low-refined cereals, legumes, nuts, olives, and olive oil); the moderate consumption of fish, white meat, eggs, and dairy products (preferably in the form of cheese and yogurt); and the relatively low consumption of red and processed meat and wine with meals [9]. Given those characteristics, individuals with better adherence to the dietary pattern also have a better nutritional intake profile, including a high intake of fiber, vitamins, minerals, and phytochemicals (e.g., flavonoids, polyphenols, and carotenoids), along with a low glycemic index, a high monounsaturated: saturated fat intake ratio, and low omega-6: omega-3 fatty acid intake ratio [10].
Considering all of the above, the aim of this systematic review was to examine current evidence about the effectiveness of studies on MD-based interventions conducted among menopausal women.
A systematic search of peer-reviewed studies published up to July 2023 was conducted following the PRISMA guidelines [11], however, the study protocol had not been previously published. The search used the following databases: Scopus, PubMed, and Web of Science. The query question was (“intervention” or “food and nutrition education” or “trial” or “pilot study” or “program”) AND (“mediterranean diet”) AND (“menopause” or “menopausal”). Only studies published in the last 15 years were included. Reference lists of eligible studies were scanned to identify additional pertinent publications, and the bibliographic reference management software EndNote™ 20 software (Clarivate, Philadelphia, USA) was used.
All studies that provided the description of interventions with MD in menopausal women published in the last 15 years (2008–2023) were included. This encompassed randomized trials, pilot intervention without a control arm or experimental study. Exclusion criteria included studies not exclusively targeted on dietary intervention but involving various health behaviors (e.g., physical activity), as well as protocols, commentaries, and opinion papers. Articles were not excluded because of age, sample size, sampling methodology, or geographical location. The titles and abstracts of retrieved articles were independently evaluated by two researchers (CG and RS), not blinded to authorships.
Relevant information was extracted regarding the country, participants (number, age, and inclusion and exclusion criteria), study design, enrolment (start and end date), length of participant intervention and follow-up, as well as recruitment and sampling procedures, intervention description, and outcomes. When extracting data related to outcomes, the authors opted to focus on the parameters most mentioned in the literature regarding anthropometric/body composition parameters (weight, body mass index (BMI), total fat mass, visceral fat, fat-free mass, and waist circumference), blood pressure (systolic blood pressure (SBP) and diastolic blood pressure (DBP)), dietary (MD adherence, energy, fat, saturated fat, polyunsaturated fatty acids (PUFA), monosaturated fatty acids (MUFA), ω6: ω3 ratio, carbohydrates and fiber), and blood outcomes (ω6: ω3 ratio, triglycerides, total cholesterol, high density lipoproteins (HDL), low density lipoproteins (LDL), fasting glucose, homocysteine, C-reactive protein (CRP), and nitric oxide level (NO)). Data extraction was carried out by one researcher, CG, in consultation with a second researcher, RS, when required.
The quality of randomized intervention studies were assessed by the revised Cochrane risk-of-bias tool for randomized trials (RoB 2 tool) [12]. This tool evaluates bias in five domains: (1) randomization process, (2) deviations from intended interventions, (3) missing outcome data, (4) measurement of the outcome, and (5) selection of the reported results. The possible risk-of-bias judgements in each domain are low risk of bias, some concerns, or high risk of bias. Furthermore, an overall risk-of-bias judgement of the study was done giving the evaluation of each domain. Studies were considered to have a high risk of bias if they were judged to be at least in one domain with high risk of bias. They were considered to have some concerns of bias if they were judged to raise some concerns for at least one domain. They were considered low risk of bias only if they were judged to be at low risk of bias for all domains.
The quality of non-randomised intervention studies were assessed by “Risk Of Bias In Non-randomised Studies - of Interventions” (ROBINS-I tool) [13]. This tool considered the assessment of bias in seven domains: (1) confounding, (2) selection of participants, (3) classification of interventions, (4) deviations from intended interventions, (5) missing data, (6) measurement of outcomes, and (7) selection of the reported result. The categories for risk of bias judgements are low, moderate, serious, and critical risk of bias. An overall risk-of-bias judgement was determined to be low risk of bias if the study was low risk for all domains, moderate risk of bias if the study was of low or moderate risk for all domains, serious risk of bias if the study was at serious risk in at least one domain, and critical risk of bias if the study was at critical risk in at least one domain. The risk-of-bias plots were generated using the app robvis [14].
The first search yielded 134 potentially relevant publications (Figure 1). Following the removal of duplicates and title and abstract screening, 10 publications were retrieved for full-text screening, and at the end six were included in the systematic review. One study was included resulting from the reference list of eligible studies.
From the seven intervention studies, three were conducted in countries bordering the Mediterranean (Spain [15] and Italy [16],[17]), three were from of Northern Europe (Poland [18]–[20]) and one was from USA [21]. The number of participants varies between 16 and 230, and all studies included menopausal women with mean ages ranging from 49–77 years old (Table 1). The supplementary table provide details in inclusion and exclusion criteria for participants selection.
Two studies were non-randomized intervention studies. One was a controlled before-and-after study involving 16 menopausal women following the MD [21] and the other was a case-control study, comparing menopausal women (case) with fertile women over 45 years old (control) intervein with MD [16]. Five studies were randomized trials, randomizing participants to the MD or usual diet [15], or placebo [17], or Central European diet (CED) [18]–[20] (Table 2). The follow-up ranged from 2 months to 1 year, and the intervention spanned from 1 week to 1 year. The intervention designs are diverse, and included education workshops [15], prescription of the MD by a nutritionist [16],[21], oral supplementation with enriched extra virgin olive oil [17], and the provision of pre-packaged main meals according to MD plus dietary advice [18]–[20].
First Author, publication year | Study design | Country | Participants |
Arms | Age (years, mean ± sd) | BMI (kg/m2, mean ± sd) | |
Randomized | Analysed | ||||||
Bihuniak JD, 2016 [21] | Controlled before-and-after study | United States of America | - | 16 | One arm: MD | 77.0±6.8 | 26.1±3.1 |
Rodríguez AS, 2016 [15] | Randomized clinical trial | Spain | 320 | 230 | Arm 1: intervention group MD Arm 2: control group |
53.2±4.3 | 27.6±5.5 27.3±5.3 |
Vignini A, 2017 [17] | Randomized clinical trial | Italy | 60 | 60 | Arm 1: intervention group supplemented with oral extra virgin olive oil (VOO) enriched with vitamins D3, K1 and B6. Arm 2: control group with oral supplementation with VOO |
54.6±3.7 55.6±2.6 |
25.2±2.7 25.9±3.1 |
Bajerska J, 2018 [20] | Randomized clinical trial | Poland | 144 | 130 | Arm 1: Mediterranean diet (MED) Arm 2: Central European diet (CED) |
60.3±4.7 60.8±4.7 |
33.8±5.6 33.6±4.2 |
Duś-Żuchowska M, 2018 [18] | |||||||
Muzsik A, 2019 [19] | |||||||
Lombardo M, 2020 [16] | Case-control study | Italy | 89 | 89 | Arm 1: Menopausal women Arm 2: Fertile women over 45 years of age |
57.1±4.9 48.8±4.0 |
30.6±5.4 29.5±5.0 |
Note: BMI: Body mass index; DRI: Dietary reference intake; CVD: Cardiovascular diseases; NR: Not reported; MD: Mediterranean diet; CED: Central European diet; VOO: Virgin olive oil.
First author, publication year | Intervention length | Follow-up (months) | Recruitment | Randomization | Intervention characteristics | Outcomes |
||||
Anthropo-metric | Dietary | Blood | Blood pressure | Genetic | ||||||
Bihuniak JD, 2016 [21] | 12 weeks | 2 years | Convenience | No | Advised by a dietitian on how to follow a MD, and substitute sources of saturated fat and refined carbohydrates by extra virgin olive oil (3 T/day), walnuts (1.5 oz/day), and fatty fish (3–5 servings/wk) which were provided at 3-week intervals. | Yes | Yes | Yes | No | No |
Rodríguez AS, 2016 [15] | 1 week | 1 year | Recruited in two primary health centres in one province | Yes | Intervention group: receive 3 interactive educational workshops (cardiovascular disease, maintenance of healthy habits and MD). Control group: participants received information pamphlets about MD. |
Yes | Yes | No | No | No |
Vignini A, 2017 [17] | 1 year | 1 year | Recruitment was performed in the ambulatory centre of the local hospital | Yes | Intervention group: oral supplementation with 20 mL/d extra virgin olive oil (VOO) enriched with vitamins D3 (100% RDA), K1 (100% RDA), and B6 (30% RDA). Control group: oral supplementation with 20 mL/d VOO. Standardized MD prescribed for both groups. |
Yes | No | Yes | No | No |
Bajerska J, 2018 [20] | 16 weeks | 16 weeks | Recruited through advertisements | Yes, stratified for BMI | Both groups were advised to follow a hypocaloric diet without added salt, refined fats and sugar; and to maintain the usual level of physical activity. During the intervention period, participants picked up packaged main meals and the other meals were prepared by the participants according to the prescribed instructions. MED group: followed a MD food plan. Olive oil and nuts were consumed every day. The proportion of soluble to insoluble fiber was 20–80%. CED group: followed a CED food plan, with a special focus on high levels of fiber. The proportion of soluble to insoluble fiber was 35–65%. |
Yes | Yes | Yes | Yes | No |
Duś-Żuchowska M, 2018 [18] | No | No | Yes | No | No | |||||
Muzsik A, 2019 [19] | No | No | Yes | No | Yes | |||||
Lombardo M, 2020 [16] | 2 months | 2 months | Convenience, recruited in nutrition clinic | No | All participants were advised to follow a two-month hypocaloric, moderate fat and MD balanced diet individually tailored, with the use of herbs and spices instead of salt, reduction of red meat to less than twice a week and to eat fish at least twice a week. The main sources of daily added fat were 25–35 g of olive oil and 20 g of nuts per day. It was recommended to only performed minimal aerobic training. |
Yes | No | Yes | Yes | No |
Note: NR: Not reported; MD: Mediterranean diet; BMI: Body mass index; CED: Central European diet group; MED: Mediterranean diet group; VOO: Virgin olive oil
The included studies aimed to assess the effect of the MD on various parameters, including the reduction of weight [15],[16],[20] and changes in body composition [15], cardiovascular risk factors [17],[19]–[21], atherosclerosis prevention [18], and bone health [17]. To assess these effects, studies evaluated several outcomes that authors summarize in Table 3 (anthropometric and blood pressure outcomes), Table 4 (dietary outcomes), and Table 5 (blood outcomes). One study also evaluates the association between genetic polymorphism of FADS1 and FADS2 genes and fatty acids concentrations after MD intervention period [19].
In general, MD was modestly efficacious at reducing body weight (ranging from -0.2 to -7.7 kg)[16],[20],[21], with significant changes only in one study [20]. No significant effects on BMI were found. Regarding body composition, one study reported a significant reduction in total fat mass (mean change -6.6 kg) [20], discrepant changes were found in visceral fat between studies (one with significant increase [15] and another with significant decrease [20]). A reduction on fat free mass (ranging from -0.6 to -1.1 kg) [16],[20] was noted, with only in one study achieving significant changes [20]. Waist circumference (ranging from -0.1 to -7.4 cm), SBP (ranging from -9 to -10.2 mmHg) and DBP (ranging from -6.7 to -7 mmHg) decreases after MD intervention [15],[16],[20], however significant changes only found in one study [20] (Table 3).
Three studies evaluated dietary outcomes (Table 4) [15],[20],[21]. One of these studies present MD adherence score from MEDAS-14 questionnaire [22], while the others reported the MD adherence score from MedSD questionnaire [23] and nutritional analysis from multiple 3-day diet records [21] or from daily food records [20]. In both studies [15],[21] MD interventions significantly increased the adherence to the MD, increased the intake of fat, PUFA, MUFA, and decreased the intake of saturated fat, ω6: ω3 ratio and carbohydrates. However, there was discrepancies in the findings related to energy intake. Bihuniak et al. [21] reported that energy intake increased and Bajerska et al. [20] found a significant decrease.
Changes in lipid levels were verified in five studies [16],[17],[19]–[21] (Table 5). It was verified that MD led to a decrease in triglycerides (ranging from -33.9 to -5.8 mg/dL), total cholesterol (ranging from -22.8 to -2.3 mg/dL), LDL (ranging from -28.2 to -4.0 mg/dL), and ω6: ω3 ratio (ranging from -1.5 to -0.2). The effect of MD on HDL, yielded different results, with two studies reporting an increase in HDL values [16],[17] and the other two showing a decrease in HDL values [20],[21]. Bajerska et al. [20] verified a significant increase in homocysteine levels (mean change +0.7 µM, p = 0.002), this could be relevant since some authors correlates homocysteine with cardiovascular problems [24]. MD also showed significant and beneficial impact on C-reactive protein (CRP) (mean change -1.2 mg/L) [18] and on nitric oxide level (NO) (mean change -6.6 nmol NO/mg protein) [17], both indicators of CVD risk, due to the development of atherosclerosis that is associated with inflammation within the vessel walls.
The MD produced similar effects on glycemic control (Table 5), since all of them showed reduction on fasting glucose levels (ranging from -6.4 to -0.8 mg/dL) [16],[17],[20]. Notably, the study from Bajerska et al. showed significant decrease on fasting glucose levels and in homeostatic model assessment of insulin resistance (HOMA2-IR) in women submitted to MD (mean change -0.46, p < 0.0001) [20].
First Author, publication year | Body Composition |
Blood Pressure |
||||||||||||||
Weight (kg) |
Body Mass Index (Kg/m2) |
Total fat mass (kg) |
Visceral fat (kg) |
Fat-free mass (kg) |
Waist circumference (cm) |
SBP (mmHg) |
DBP (mmHg) |
|||||||||
Baseline | Mean change | Baseline | Mean change | Baseline | Mean change | Baseline | Mean change | Baseline | Mean change | Baseline | Mean change | Baseline | Mean change | Baseline | Mean change | |
Bihuniak JD, 2016 [21] | 65.5±9.5 | -0.2# | 26.1±3.1 | -0.5# | NR | NR | NR | NR | NR | NR | NR | NR | NR | NR | NR | NR |
Rodríguez AS, 2016 [15] | IG NR | NR | 27.6±5.5 | +0.1 | 24.8±9.9 | +0.2 | 7.8±3.0 | +0.2* | NR | NR | 92.9±13.3 | -0.1 | NR | NR | NR | NR |
CG NR | 27.3±5.3 | +0.5* | 24.8±9.2 | +1.3* | 7.7±2.8 | +0.5* | 93.4±13.6 | +1.6* | ||||||||
Vignini A, 2017 [17] | PlaVOO NR |
NR | 25.2±2.7 | -0.2# | NR | NR | NR | NR | NR | NR | NR | NR | NR | NR | NR | NR |
VitVOO NR |
25.9±3.1 | -1.7# | ||||||||||||||
Bajerska J, 2018 [20] | MED 87.0±NR | −7.7* | NR | NR | 40.4±NR | −6.6* | 1.1±NR | −0.3* | 46.6±NR | −1.1* | 105.0±NR | −7.4* | 140.9±NR | −10.2* | 87.0±NR | −6.7* |
CED 85.3±NR | −7.5 | 39.5±NR | −6.7 | 1.1±NR | −0.3 | 45.8±NR | −0.8* | 105.4±NR | −7.5 | 142.1±NR | −10.4 | 86.9±NR | −8.1 | |||
Lombardo M, 2020 [16] | MEN 80.8±16.2 |
-3.7 | 30.6±5.4 | -0.9 | 33.4±11.1 | -2.4 | NR | NR | 45.1±5.4 | -0.6 | 97.5±15.3 | -3.1 | 133.8±17.2 | -9 | 85.2±13.0 | -7 |
REG 77.0±14.1 |
-3.1 | 29.5±5.0 | -0.8 | 29.9±9.7 | -2.2 | 44.9±5.3 | -0.8 | 92.2±11.5 | -3.2 | 124.6±13.3 | -6.8 | 80.6±12.8 | -3.3 |
Note: Values are reported as: mean ± standard deviation, unless otherwise specified. NR: Not reported; NO: Values are not an outcome; TEI: Total energy intake; PUFA: Polyunsaturated fatty acids; MUFA: Monounsaturated fatty acids; VitVO: Vitaminized virgin olive oil group; PlaVOO: Placebo virgin olive oil group; MED: Group with mediterranean diet; CED: Group with central european diet; IG: Intervention group; CG: Control group; MEN: Menopausal women group; REG: Not menopausal; SBP: Systolic blood pressure; DBP: Diastolic blood pressure; #: mean change was computed as the difference in means between baseline and follow-up using data from the publication; *p < 0.05.
First Author, publication year | Dietary |
|||||||||||||||||
Med Diet adherence |
Energy (kcal/d) |
Fat (%TEI) |
Saturated fat (%TEI) |
PUFA (%TEI) |
MUFA (%TEI) |
ω6: ω3 ratio |
Carbohydrates (%TEI) |
Fiber (g) |
||||||||||
Baseline | Mean change | Baseline | Mean change | Baseline | Mean change | Baseline | Mean change | Baseline | Mean change | Baseline | Mean change | Baseline | Mean change | Baseline | Mean change | Baseline | Mean change | |
Bihuniak JD, 2016 [21] | 32.3±4.3 | +9.0#* | 1679±231 | +124# | 31.4±5.6 | +9.3#* | 10.3±2.4 | -0.7# | NR | NR | NR | NR | 8.2±5.2 | -3.7#* | 51.5±7.2 | -7.0#* | 20.3±7.4 | +1# |
Rodríguez AS, 2016 [15] | IG 7.06±2.02 | +2.31#* | NR | NR | NR | NR | NR | NR | NR | NR | NR | NR | NR | NR | NR | NR | NR | NR |
CG 6.96±2.15 | +0.15 | |||||||||||||||||
Bajerska J, 2018 [20] | MED NR | NR | 1912±NR | -574* | 36.1±NR | +0.3* | 16.9±NR | -8.4* | 5.2±NR | +3.8* | 14.0±NR | +5.0 | NR | NR | 47.5±NR | -2.5* | 21.0±NR | +11.7* |
CED NR | NR | 1936±NR | -574 | 35.9±NR | -8.5 | 17.4±NR | -8.6 | 5.2±NR | +3.9 | 13.2±NR | -3.9 | 48.6±NR | +5.7 | 21.7±NR | +17.6 |
Note: Values are reported as: mean ± standard deviation, unless otherwise specified. NR: not reported; TEI: Total energy intake; PUFA: Polyunsaturated fatty acids; MUFA: Monounsaturated fatty acids; MED: Group with mediterranean diet; CED: Group with central european diet; IG: Intervention group; CG: Control group; #: mean change was computed as the difference in means between baseline and follow-up using data from the publication; *p < 0.05.
First author, publication year | Blood |
|||||||||||||||||
ω6: ω3 ratio |
Triglycerides (mg/dL) |
Total cholesterol (mg/dL) |
HDL (mg/dL) |
LDL (mg/dL) |
Fasting glucose (mg/dL) |
Homocysteine (µM) |
CRP (mg/L) |
NO (nmol NO/ mg protein) |
||||||||||
Baseline | Mean change | Baseline | Mean change | Baseline | Mean change | Baseline | Mean change | Baseline | Mean change | Baseline | Mean change | Baseline | Mean change | Baseline | Mean change | Baseline | Mean change | |
Bihuniak JD, 2016 [21] | 10.4±1.9 | -1.5#* | 99.8±34.8 | -12.5 #* | 205.5±31.9 | -2.3# | 68.1±13.0 | -4.0#* | 117.6±25.6 | -4.0# | NR | NR | NR | NR | NR | NR | NR | NR |
Vignini A, 2017 [17] | NR | NR | VitVO 125.2±46.9 | -5.8# | 219.6±34.3 | -15.3# | 58.6±14.1 | +7.1#* | 133.1±33.7 | -5.5# | 82.5±9.0 | -0.8# | NR | NR | NR | NR | 43.8±4.9 | -6.6#* |
PlaVO 127.2±44.3 | -12.0# | 218.3±33.5 | -12.7# | 57.6±15.3 | +6.8#* | 132.4±32.4 | -3.9# | 83.5±9.0 | -2.0# | 41.9±4.2 | +0.7# | |||||||
Bajerska J, 2018 [20] | MED NR | NR | 157.4±NR | -33.9* | 235.8±NR | -15.5* | 55.3±NR | -0.1 | 149.7±NR | -9.4 | 98.0±NR | -6.4* | 11.5±NR | +0.7* | NR | NR | NR | NR |
CED NR | NR | 164.1±NR | -38.8 | 226.4±NR | -11.2 | 53.1±NR | -2.0 | 143.1±NR | -4.9 | 98.1±NR | -5.4 | 10.9±NR | +0.8 | NR | NR | NR | NR | |
Duś-Żuchowska M, 2018 [18] | NR | NR | NR | NR | NR | NR | NR | NR | NR | NR | NR | NR | NR | NR | MED 4.5±4.5 | -1.2* | NR | NR |
CED 4.5±4.4 | -0.9* | |||||||||||||||||
Muzsik A, 2019 [19] | MED 2.5±0.7∥ | -0.2 | NR | NR | NR | NR | NR | NR | NR | NR | NR | NR | NR | NR | NR | NR | NR | NR |
CED 2.4±0.7∥ | +0.01 | |||||||||||||||||
Lombardo M, 2020 [16] | NR | NR | MEN 108.3±65.2 | -22.9 | 219.6±41.7 | -22.8 | 58.3±12.6 | +6.0 | 143.6±36.2 | -28.2* | 92.7±14.0 | -2.5 | NR | NR | NR | NR | NR | NR |
REG 92.0±39.0 | +8.2 | 202.9±35.5 | -9.3 | 58.9±16.4 | +2.2 | 130.3±29.4 | -7.7 | 92.4±9.4 | -2.2 |
Note: Values are reported as: mean ± standard deviation, unless otherwise specified. NR: Not reported; HDL: High density lipoprotein; LDL: Low density lipoprotein; CRP: C-reactive protein; NO: Nitric oxide level; MED: Mediterranean diet group; CED: Central european diet group; MEN: Menopausal women group; REG: Not menopausal; VitVO: Vitaminized virgin olive oil group; PlaVO: Placebo virgin olive oil group; IG: Intervention group; CG: Control group; ∥: values obtained in red blood cells; #: mean change was computed as the difference in means between baseline and follow-up using data from the publication; *p < 0.05.
The five randomized studies included exhibited degrees of bias of low concern [17], some concerns [18]–[20], and one with high concern [15] (Figure 2). The domain with poorest performance in the analysis was the bias related to deviations from intended interventions.
The two non-randomized studies showed a serious risk of bias [16],[21], mainly due to confounding variables (e.g., physical activity level), deviations from intended interventions, and missing data (Figure 3).
In 2020, the United Nations estimated that, worldwide, 985 million women were 50 years old or older, and that number is projected to increase to 1.65 billion by 2050 [25]. Therefore, women are living longer, and the phase of their life lived in menopause is increasing as well. In that context, the immediate and long-term impacts of estrogen depletion and aging on health pose a significant challenge to health care systems around the world.
Optimizing diet is increasingly recognized as being crucial in strategies aimed to promote women's health during the menopause period [4]. The MD, characterized by the high intake of plan-based foods, is considered by several authors to be a possible explanation for improved bone metabolism, enhanced muscle performance, and reduced oxidative stress, inflammation, and insulin resistance [4],[26]. Therefore, gaining insights into the impacts associated with adhering to the MD on menopausal women's health is essential for the appropriate and specific design of public health interventions.
This systematic review, addressing the effectiveness of MD-based interventions conducted among menopausal women, suggests that adhering to the MD can have beneficial impacts during the climacteric phase of life, including the reduction of weight, blood pressure, blood ω6: ω3 ratio, triglycerides, total cholesterol, and LDL levels.
Menopause has been associated with an increase in body weight and changes in body composition such as an increase in fat mass and a reduction in fat-free mass. That shift is attributed to the reduction of basal metabolic rate, diminished physical activity, and the loss of estrogen's influences on lipoprotein lipase activity and lipolysis [27],[28]. Moreover, changes in body composition reflect the significant decline in muscle mass, with a sharp annual decline after the age of 50 years, ranging between 0.6% and 2%, most prominently in the first 3 years after the onset of permanent amenorrhea [29],[30]. Those changes can be critical for the risk of insulin resistance, diabetes, and cardiovascular diseases. The studies included in this systematic review suggest that following the MD is associated with reduced body weight but cannot reduce BMI and can exert conflicting impacts on body composition (e.g., total and visceral fat levels). The observed discrepancies in results regarding BMI may be partially explained by the reduction in women's height, often linked to worsened bone condition in the spine, such that osteoporotic vertebral fractures are common in the postmenopause period but are seldom clinically identified [31]. Other authors have found that the MD can result in greater weight loss than a low-fat diet [32] and that a higher adherence to the diet is associated with increased likelihood of maintained weight loss [33] and reduced abdominal adiposity, particularly visceral fat levels [34],[35]. However, the particularities of menopausal women can be complex, and total energy intake and physical activity level can be important confounders that should be more rigorously controlled in future studies.
The accelerated loss of fat-free mass, also reported to occur during menopause, increases the risk of sarcopenia, a condition that can lead to functional impairment and physical disabilities [36]. The accelerated loss of fat-free mass reflects a decline in both muscle mass and bone mass, the latter of which intensifies after menopause [37]. The results of this review generally show the reduction of fat-free mass in menopausal women following a MD-based dietary intervention. Moreover, although no clinical trials were found that examined adherence to the MD and sarcopenia, a systematic review of observational studies has shown no evidence of the MD's positive effect on sarcopenia other than its general positive role in improving muscle mass and muscle function [38].
During the reproductive period, the production of oestrogens exerts a protective effect on endothelial function and lipid metabolism, with a decrease in LDL and the ratio of total cholesterol and HDL. Estrogen, along with changes in the lipid profile, contributes to improved vasodilation and reduced levels of homocysteine and fibrinogen [39]. Conversely, the drop in estrogen levels in the bloodstream may potentially contribute to an increase in blood pressure through various mechanisms, such as influencing the arterial wall, activating the renin–angiotensin system, and stimulating the sympathetic nervous system [40]. Those physiological changes are associated with and elevated risk of cardiovascular diseases and the higher prevalence of metabolic syndrome [4]. The results of this review suggest that menopausal women who follow the MD may experience benefits such as reduced blood pressure, triglycerides, total cholesterol, and LDL levels. Those results align with the findings of other authors [32],[41],[42] and can be partly explained by components of the MD such as a high prevalence of unsaturated fats in sources of fiber and protein, a scarcity of saturated fats, and a richness of fruits, vegetables, whole grains, nuts, and legumes, all of which contribute to the dietary pattern [41]. Other authors have also found that supplementation of fish oil rich in omega-3 significantly improves endothelial function and reduces pro-inflammatory markers among patients with diabetes due to its antioxidant and anti-inflammatory properties [43].
Increased blood pressure with aging can be also explained by increased sensitivity to salt with aging, which contributes to the development of age-related cardiovascular disease [44]. The MD, however, makes no prescriptions regarding salt intake, and Viroli et al. [45] did not detect any differences in sodium intake between a lower versus higher adherence to the MD. Another recent systematic review concluded that among individuals more than 65 years old, high potassium intake (i.e., >3510 mg/d) and a low sodium: potassium ratio (i.e., <1) have a beneficial effect on reducing the risk of cardiovascular disease. That positive outcome is largely because the potassium present in fruits and vegetables can mitigate the harmful effects of excess sodium intake [46].
A systematic review encompassing all meta-analyses and randomised controlled trials that compared the MD with a control diet on the treatment of type 2 diabetes and prediabetic states found that MD was associated with better glycemic control and improved cardiovascular risk factors than control diets, including those characterized by lower fat content [47]. That association was attributed to components of the MD that are considered to be anti-inflammatory and antioxidative (e.g., fiber, vitamins, and minerals, as well as antioxidants and polyphenols), coupled with a lower intake of proinflammatory foods and nutrients (e.g., saturated and trans fatty acids, refined sugars, and starches). Those explanations align with the findings of the present review, which all showed a reduction in fasting glucose levels among menopausal women [16],[17],[20].
It may be surprising that the present systematic review focused on the effectiveness of dietary interventions but only three studies assessed dietary outcomes [15],[20],[21], primarily to control adherence to the intervention. That point is significant because most health-related outcomes can be directly influenced by differences in the daily total energy and nutritional intake of participants. Two of the studies evaluated adherence to the MD using two different scoring systems [15],[21], which could also be viewed as a limitation. After all, using a score to estimate a dietary pattern is limited by subjectivity, which can cause considerable variability in the interpretation of the results [48].
Another limitation of the systematic review was that most studies did not include assessments of physical activity or total energy intake, both of which could be significant confounders affecting the outcomes. Thus, the interpretation of the results should be approached with caution. Beyond that, the relatively short duration of interventions (e.g., 1 week) could be insufficient to achieve meaningful results.
In conclusion, this systematic review suggests that adhering to a MD can have beneficial impacts on menopausal women's health, including the reduction of weight, blood pressure, blood ω6: ω3 ratio, triglycerides, total cholesterol, and LDL levels. Those findings appear to be relevant in the context of public health interventions to aimed to enhancing the quality of life for menopausal women.
The authors declare they have not used Artificial Intelligence (AI) tools in the creation of this article.
[1] | Alzheimer's Association.2023 Alzheimer's disease facts and figures. Alzheimers Dement (2023) 19: 1598-1695. https://doi.org/10.1002/alz.13016 |
[2] |
Diogo VS, Ferreira HA, Prata D, et al. (2022) Early diagnosis of Alzheimer's disease using machine learning: A multi-diagnostic, generalizable approach. Alz Res Therapy 14: 107. https://doi.org/10.1186/s13195-022-01047-y ![]() |
[3] |
Jakob-Roetne R, Jacobsen H (2009) Alzheimer's disease: From pathology to therapeutic approaches. Angew Chem Int Ed Engl 48: 3030-3059. https://doi.org/10.1002/anie.200802808 ![]() |
[4] |
Ferrari-Souza JP, Bellaver B, Ferreira PCL, et al. (2023) APOEϵ4 potentiates amyloid β effects on longitudinal tau pathology. Nat Aging 3: 1210-1218. https://doi.org/10.1038/s43587-023-00490-2 ![]() |
[5] |
Saravanan KM, Kannan M, Meera P, et al. (2022) E3 ligases: A potential multi-drug target for different types of cancers and neurological disorders. Future Med Chem 14: 187-201. https://doi.org/10.4155/fmc-2021-0157 ![]() |
[6] |
Moore KBE, Hung TJ, Fortin JS (2023) Hyperphosphorylated tau (p-tau) and drug discovery in the context of Alzheimer's disease and related tauopathies. Drug Discov Today 28: 103487. https://doi.org/10.1016/j.drudis.2023.103487 ![]() |
[7] |
Saravanan KM, Zhang H, Zhang H, et al. (2020) On the conformational dynamics of β-amyloid forming peptides: A computational perspective. Front Bioeng Biotechnol 8: 532. https://doi.org/10.3389/fbioe.2020.00532 ![]() |
[8] |
Agnello L, Lo Sasso B, Vidali M, et al. (2021) Neurogranin as a reliable biomarker for synaptic dysfunction in Alzheimer's disease. Diagnostics 11: 2339. https://doi.org/10.3390/diagnostics11122339 ![]() |
[9] |
Pelucchi S, Gardoni F, Di Luca M, et al. (2022) Chapter 28 - Synaptic dysfunction in early phases of Alzheimer's Disease. Handbook of Clinical Neurology.Elsevier 417-438. https://doi.org/10.1016/B978-0-12-819410-2.00022-9 ![]() |
[10] |
Saunders T, Gunn C, Blennow K, et al. (2023) Neurogranin in Alzheimer's disease and ageing: A human post-mortem study. Neurobiol Dis 177: 105991. https://doi.org/10.1016/j.nbd.2023.105991 ![]() |
[11] |
Rani S, Dhar SB, Khajuria A, et al. (2023) Advanced overview of biomarkers and techniques for early diagnosis of Alzheimer's disease. Cell Mol Neurobiol 43: 2491-2523. https://doi.org/10.1007/s10571-023-01330-y ![]() |
[12] |
Abraham WC, Jones OD, Glanzman DL (2019) Is plasticity of synapses the mechanism of long-term memory storage?. npj Sci Learn 4: 9. https://doi.org/10.1038/s41539-019-0048-y ![]() |
[13] |
Xue M, Sun FR, Ou YN, et al. (2020) Association of cerebrospinal fluid neurogranin levels with cognition and neurodegeneration in Alzheimer's disease. Aging 12: 9365-9379. https://doi.org/10.18632/aging.103211 ![]() |
[14] |
Toader C, Dobrin N, Brehar FM, et al. (2023) From recognition to remedy: The significance of biomarkers in neurodegenerative disease pathology. Int J Mol Sci 24: 16119. https://doi.org/10.3390/ijms242216119 ![]() |
[15] |
Scheltens P, De Strooper B, Kivipelto M, et al. (2021) Alzheimer's disease. Lancet 397: 1577-1590. https://doi.org/10.1016/S0140-6736(20)32205-4 ![]() |
[16] |
Cardoso BR, Roberts BR, Malpas CB, et al. (2019) Supranutritional sodium selenate supplementation delivers selenium to the central nervous system: Results from a randomized controlled pilot trial in Alzheimer's disease. Neurotherapeutics 16: 192-202. https://doi.org/10.1007/s13311-018-0662-z ![]() |
[17] |
DeTure MA, Dickson DW (2019) The neuropathological diagnosis of Alzheimer's disease. Mol Neurodegener 14: 32. https://doi.org/10.1186/s13024-019-0333-5 ![]() |
[18] | Zhang XX, Tian Y, Wang ZT, et al. (2021) The Epidemiology of Alzheimer's disease modifiable risk factors and prevention. J Prev Alzheimers Dis 8: 313-321. https://doi.org/10.14283/jpad.2021.15 |
[19] |
Dubois B, von Arnim CAF, Burnie N, et al. (2023) Biomarkers in Alzheimer's disease: Role in early and differential diagnosis and recognition of atypical variants. Alz Res Therapy 15: 175. https://doi.org/10.1186/s13195-023-01314-6 ![]() |
[20] |
Jellinger KA (2022) Recent update on the heterogeneity of the Alzheimer's disease spectrum. J Neural Transm 129: 1-24. https://doi.org/10.1007/s00702-021-02449-2 ![]() |
[21] |
Dubois B, Villain N, Frisoni GB, et al. (2021) Clinical diagnosis of Alzheimer's disease: Recommendations of the International Working Group. Lancet Neurol 20: 484-496. https://doi.org/10.1016/S1474-4422(21)00066-1 ![]() |
[22] |
Camporesi E, Nilsson J, Brinkmalm A, et al. (2020) Fluid biomarkers for synaptic dysfunction and loss. Biomark Insights 15: 1177271920950319. https://doi.org/10.1177/1177271920950319 ![]() |
[23] |
Liu W, Lin H, He X, et al. (2020) Neurogranin as a cognitive biomarker in cerebrospinal fluid and blood exosomes for Alzheimer's disease and mild cognitive impairment. Transl Psychiatry 10: 125. https://doi.org/10.1038/s41398-020-0801-2 ![]() |
[24] |
Hindley N, Sanchez Avila A, Henstridge C (2023) Bringing synapses into focus: Recent advances in synaptic imaging and mass-spectrometry for studying synaptopathy. Front Synaptic Neurosci 15: 1130198. https://doi.org/10.3389/fnsyn.2023.1130198 ![]() |
[25] |
Mravinacová S, Alanko V, Bergström S, et al. (2024) CSF protein ratios with enhanced potential to reflect Alzheimer's disease pathology and neurodegeneration. Mol Neurodegener 19: 15. https://doi.org/10.1186/s13024-024-00705-z ![]() |
[26] | Milos T, Vuic B, Balic N, et al. (2024) Cerebrospinal fluid in the differential diagnosis of Alzheimer's disease: An update of the literature. Expert Rev Neurother 1–17. https://doi.org/10.1080/14737175.2024.2400683 |
[27] |
Pereira JB, Janelidze S, Ossenkoppele R, et al. (2021) Untangling the association of amyloid-β and tau with synaptic and axonal loss in Alzheimer's disease. Brain 144: 310-324. https://doi.org/10.1093/brain/awaa395 ![]() |
[28] |
Kester MI, Teunissen CE, Crimmins DL, et al. (2015) Neurogranin as a cerebrospinal fluid biomarker for synaptic loss in symptomatic Alzheimer disease. JAMA Neurol 72: 1275-1280. https://doi.org/10.1001/jamaneurol.2015.1867 ![]() |
[29] |
Willemse EAJ, Sieben A, Somers C, et al. (2021) Neurogranin as biomarker in CSF is non-specific to Alzheimer's disease dementia. Neurobiol Aging 108: 99-109. https://doi.org/10.1016/j.neurobiolaging.2021.08.002 ![]() |
[30] |
van Dyck CH, Swanson CJ, Aisen P, et al. (2023) Lecanemab in early Alzheimer's disease. N Engl J Med 388: 9-21. https://doi.org/10.1056/NEJMoa2212948 ![]() |
[31] |
Bergström S, Remnestål J, Yousef J, et al. (2021) Multi-cohort profiling reveals elevated CSF levels of brain-enriched proteins in Alzheimer's disease. Ann Clin Transl Neurol 8: 1456-1470. https://doi.org/10.1002/acn3.51402 ![]() |
[32] |
Remnestål J, Just D, Mitsios N, et al. (2016) CSF profiling of the human brain enriched proteome reveals associations of neuromodulin and neurogranin to Alzheimer's disease. Proteomics Clin Appl 10: 1242-1253. https://doi.org/10.1002/prca.201500150 ![]() |
[33] |
Sandelius Å, Portelius E, Källén Å, et al. (2019) Elevated CSF GAP-43 is Alzheimer's disease specific and associated with tau and amyloid pathology. Alzheimers Dement 15: 55-64. https://doi.org/10.1016/j.jalz.2018.08.006 ![]() |
[34] |
McGrowder DA, Miller F, Vaz K, et al. (2021) Cerebrospinal fluid biomarkers of Alzheimer's disease: Current evidence and future perspectives. Brain Sci 11: 215. https://doi.org/10.3390/brainsci11020215 ![]() |
[35] |
Remnestål J, Bergström S, Olofsson J, et al. (2021) Association of CSF proteins with tau and amyloid β levels in asymptomatic 70-year-olds. Alz Res Therapy 13: 54. https://doi.org/10.1186/s13195-021-00789-5 ![]() |
[36] |
Rosenberg A, Öhlund-Wistbacka U, Hall A, et al. (2022) β-amyloid, tau, neurodegeneration classification and eligibility for anti-amyloid treatment in a memory clinic population. Neurology 99: e2102-e2113. https://doi.org/10.1212/WNL.0000000000201043 ![]() |
[37] |
Cummings J (2019) The national institute on aging—Alzheimer's association framework on Alzheimer's disease: Application to clinical trials. Alzheimers Dement 15: 172-178. https://doi.org/10.1016/j.jalz.2018.05.006 ![]() |
[38] |
Tahami Monfared AA, Byrnes MJ, White LA, et al. (2022) Alzheimer's disease: Epidemiology and clinical progression. Neurol Ther 11: 553-569. https://doi.org/10.1007/s40120-022-00338-8 ![]() |
[39] |
Xiang Y, Xin J, Le W, et al. (2020) Neurogranin: A potential biomarker of neurological and mental diseases. Front Aging Neurosci 12: 584743. https://doi.org/10.3389/fnagi.2020.584743 ![]() |
[40] |
van der Flier WM, de Vugt ME, Smets EMA, et al. (2023) Towards a future where Alzheimer's disease pathology is stopped before the onset of dementia. Nat Aging 3: 494-505. https://doi.org/10.1038/s43587-023-00404-2 ![]() |
[41] | Rasmussen J, Langerman H (2019) Alzheimer's disease–why we need early diagnosis. Degener Neurol Neuromuscul Dis 123–130. https://doi.org/10.2147/DNND.S228939 |
[42] |
Liang Y, Kang X, Zhang H, et al. (2023) Knockdown and inhibition of hippocampal GPR17 attenuates lipopolysaccharide-induced cognitive impairment in mice. J Neuroinflammation 20. https://doi.org/271.10.1186/s12974-023-02958-9 ![]() |
[43] |
Schindler SE, Bollinger JG, Ovod V, et al. (2019) High-precision plasma β-amyloid 42/40 predicts current and future brain amyloidosis. Neurology 93: e1647-e1659. https://doi.org/10.1212/WNL.0000000000008081 ![]() |
[44] |
Klyucherev TO, Olszewski P, Shalimova AA, et al. (2022) Advances in the development of new biomarkers for Alzheimer's disease. Transl Neurodegener 11: 25. https://doi.org/10.1186/s40035-022-00296-z ![]() |
[45] |
Janelidze S, Mattsson N, Palmqvist S, et al. (2020) Plasma P-tau181 in Alzheimer's disease: Relationship to other biomarkers, differential diagnosis, neuropathology and longitudinal progression to Alzheimer's dementia. Nat Med 26: 379-386. https://doi.org/10.1038/s41591-020-0755-1 ![]() |
[46] |
O'Bryant SE, Petersen M, Hall J, et al. (2022) Characterization of mild cognitive impairment and dementia among community-dwelling Mexican Americans and non-hispanic whites. J Alzheimers Dis 90: 905-915. https://doi.org/10.3233/JAD-220300 ![]() |
[47] |
Pohlan J, Kress W, Hermann K-G, et al. (2020) Computed tomography thermography for ablation zone prediction in microwave ablation and cryoablation: Advantages and challenges in an ex vivo porcine liver model. J Comput Assist Tomogr 44: 744-749. https://doi.org/10.1097/RCT.0000000000001081 ![]() |
[48] |
Agnello L, Gambino CM, Lo Sasso B, et al. (2021) Neurogranin as a novel biomarker in Alzheimer's disease. Lab Med 52: 188-196. https://doi.org/10.1093/labmed/lmaa062 ![]() |
[49] |
Nashine S, Kenney MC (2024) Effects of Humanin G (HNG) on angiogenesis and neurodegeneration markers in Age-related Macular Degeneration (AMD). Mitochondrion 74: 101818. https://doi.org/10.1016/j.mito.2023.11.001 ![]() |
[50] | Vontell RT, Gober R, Dallmeier J, et al. (2024) Association of region-specific hippocampal reduction of neurogranin with inflammasome proteins in post mortem brains of Alzheimer's disease. Alzheimers Dement 10: e12444. https://doi.org/10.1002/trc2.12444 |
[51] |
Li L, Lai M, Cole S, et al. (2020) Neurogranin stimulates Ca2+/calmodulin-dependent kinase II by suppressing calcineurin activity at specific calcium spike frequencies. PLoS Comput Biol 16: e1006991. https://doi.org/10.1371/journal.pcbi.1006991 ![]() |
[52] |
Kvartsberg H, Lashley T, Murray CE, et al. (2019) The intact postsynaptic protein neurogranin is reduced in brain tissue from patients with familial and sporadic Alzheimer's disease. Acta Neuropathol 137: 89-102. https://doi.org/10.1007/s00401-018-1910-3 ![]() |
[53] |
Svirsky SE, Henchir J, Li Y, et al. (2024) Temporal-specific sex and injury-dependent changes on neurogranin-associated synaptic signaling after controlled cortical impact in rats. Mol Neurobiol 61: 7256-7268. https://doi.org/10.1007/s12035-024-04043-5 ![]() |
[54] |
Zhong L, Gerges NZ (2020) Neurogranin regulates metaplasticity. Front Mol Neurosci 12: 322. https://doi.org/10.3389/fnmol.2019.00322 ![]() |
[55] |
Rueda-García V, Rondón-Barragán IS (2024) Molecular characterization of neurogranin (NRGN) gene from Red‑Bellied Pacu (Piaractus brachypomus). Mol Neurobiol 61: 2620-2630. https://doi.org/10.1007/s12035-023-03700-5 ![]() |
[56] |
Casaletto KB, Elahi FM, Bettcher BM, et al. (2017) Neurogranin, a synaptic protein, is associated with memory independent of Alzheimer biomarkers. Neurology 89: 1782-1788. https://doi.org/10.1212/WNL.0000000000004569 ![]() |
[57] |
Vasung L, Abaci Turk E, Ferradal SL, et al. (2019) Exploring early human brain development with structural and physiological neuroimaging. Neuroimage 187: 226-254. https://doi.org/10.1016/j.neuroimage.2018.07.041 ![]() |
[58] |
Subramanian L, Calcagnotto ME, Paredes MF (2020) Cortical malformations: Lessons in human brain development. Front Cell Neurosci 13: 576. https://doi.org/10.3389/fncel.2019.00576 ![]() |
[59] |
Bick J, Nelson CA (2016) Early adverse experiences and the developing brain. Neuropsychopharmacology 41: 177-196. https://doi.org/10.1038/npp.2015.252 ![]() |
[60] |
Lista S, Santos-Lozano A, Emanuele E, et al. (2024) Monitoring synaptic pathology in Alzheimer's disease through fluid and PET imaging biomarkers: a comprehensive review and future perspectives. Mol Psychiatry 29: 847-857. https://doi.org/10.1038/s41380-023-02376-6 ![]() |
[61] |
Svirsky S, Henchir J, Li Y, et al. (2019) Neurogranin protein expression is reduced after controlled cortical impact in rats. J Neurotrauma 37: 939-949. https://doi.org/10.1089/neu.2019.6759 ![]() |
[62] |
Garrido-García A, de Andrés R, Jiménez-Pompa A, et al. (2019) Neurogranin expression is regulated by synaptic activity and promotes synaptogenesis in cultured hippocampal neurons. Mol Neurobiol 56: 7321-7337. https://doi.org/10.1007/s12035-019-1593-3 ![]() |
[63] |
Hwang H, Szucs MJ, Ding LJ, et al. (2021) Neurogranin, encoded by the schizophrenia risk gene NRGN, bidirectionally modulates synaptic plasticity via calmodulin-dependent regulation of the neuronal phosphoproteome. Biol Psychiatry 89: 256-269. https://doi.org/10.1016/j.biopsych.2020.07.014 ![]() |
[64] |
Cheriyan VT, Alfaidi M, Jorgensen AN, et al. (2020) Neurogranin regulates eNOS function and endothelial activation. Redox Biol 34: 101487. https://doi.org/10.1016/j.redox.2020.101487 ![]() |
[65] |
Lin H, Zhang J, Dai Y, et al. (2023) Neurogranin as an important regulator in swimming training to improve the spatial memory dysfunction of mice with chronic cerebral hypoperfusion. J Sport Heal Sci 12: 116-129. https://doi.org/10.1016/j.jshs.2022.01.008 ![]() |
[66] |
Gribaudo S, Saraulli D, Nato G, et al. (2021) Neurogranin regulates adult-born olfactory granule cell spine density and odor-reward associative memory in mice. Int J Mol Sci 22: 4269. https://doi.org/10.3390/ijms22084269 ![]() |
[67] |
Cardozo LP, de Lima BQI, Maciel MAE, et al. (2019) Synaptic elimination in neurological disorders. Curr Neuropharmacol 17: 1071-1095. https://doi.org/10.2174/1570159X17666190603170511 ![]() |
[68] |
Ordyan M, Bartol T, Kennedy M, et al. (2020) Interactions between calmodulin and neurogranin govern the dynamics of CaMKII as a leaky integrator. PLoS Comput Biol 16: e1008015. https://doi.org/10.1371/journal.pcbi.1008015 ![]() |
[69] |
Putkey JA, Hoffman L, Berka V, et al. (2024) Neurogranin modulates the rate of association between calmodulin and target peptides. Biophys J 123: 1676-1689. https://doi.org/10.1016/j.bpj.2024.05.010 ![]() |
[70] |
O'Day DH (2020) Calmodulin binding proteins and Alzheimer's disease: Biomarkers, regulatory enzymes and receptors that are regulated by calmodulin. Int J Mol Sci 21: 7344. https://doi.org/10.3390/ijms21197344 ![]() |
[71] |
Moradi F, Copeland EN, Baranowski RW, et al. (2020) Calmodulin-binding proteins in muscle: A minireview on nuclear receptor interacting protein, neurogranin, and growth-associated protein 43. Int J Mol Sci 21: 1016. https://doi.org/10.3390/ijms21031016 ![]() |
[72] |
Dulewicz M, Kulczyńska-Przybik A, Słowik A, et al. (2021) Neurogranin and neuronal pentraxin receptor as synaptic dysfunction biomarkers in Alzheimer's disease. J Clin Med 10: 4575. https://doi.org/10.3390/jcm10194575 ![]() |
[73] |
Poejo J, Salazar J, Mata AM, et al. (2021) The relevance of amyloid β-calmodulin complexation in neurons and brain degeneration in Alzheimer's disease. Int J Mol Sci 22: 4976. https://doi.org/10.3390/ijms22094976 ![]() |
[74] |
Kumar V, Chichili VPR, Zhong L, et al. (2013) Structural basis for the interaction of unstructured neuron specific substrates neuromodulin and neurogranin with calmodulin. Sci Rep 3: 1392. https://doi.org/10.1038/srep01392 ![]() |
[75] |
Szklarczyk D, Kirsch R, Koutrouli M, et al. (2023) The STRING database in 2023: Protein–protein association networks and functional enrichment analyses for any sequenced genome of interest. Nucleic Acids Res 51: D638-D646. https://doi.org/10.1093/nar/gkac1000 ![]() |
[76] |
Nazir FH, Camporesi E, Brinkmalm G, et al. (2021) Molecular forms of neurogranin in cerebrospinal fluid. J Neurochem 157: 816-833. https://doi.org/10.1111/jnc.15252 ![]() |
[77] | Joglekar AP A cell-type centric view of alternative splicing in the mammalian brain (2022). Available from: https://research.weill.cornell.edu/about-us/news-updates/cell-type-centric-view-alternative-splicing-mammalian-brain |
[78] |
Beghi S, Furmanik M, Jaminon A, et al. (2022) Calcium signalling in heart and vessels: Role of calmodulin and downstream calmodulin-dependent protein kinases. Int J Mol Sci 23: 16139. https://doi.org/10.3390/ijms232416139 ![]() |
[79] |
O'Day DH (2022) Calmodulin binding domains in critical risk proteins involved in neurodegeneration. Curr Issues Mol Biol 44: 5802-5814. https://doi.org/10.3390/cimb44110394 ![]() |
[80] |
Zhang H, Jiang X, Ma L, et al. (2022) Role of Aβ in Alzheimer's-related synaptic dysfunction. Front Cell Dev Biol 10: 964075. https://doi.org/10.3389/fcell.2022.964075 ![]() |
[81] |
Abi-Dargham A, Moeller SJ, Ali F, et al. (2023) Candidate biomarkers in psychiatric disorders: State of the field. World Psychiatry 22: 236-262. https://doi.org/10.1002/wps.21078 ![]() |
[82] |
Blennow K, Diaz-Lucena D, Zetterberg H, et al. (2019) CSF neurogranin as a neuronal damage marker in CJD: A comparative study with AD. J Neurol Neurosurg Psychiatry 90: 846-853. https://doi.org/10.1136/jnnp-2018-320155 ![]() |
[83] |
Rabbito A, Dulewicz M, Kulczyńska-Przybik A, et al. (2020) Biochemical markers in Alzheimer's disease. Int J Mol Sci 21: 1989. https://doi.org/10.3390/ijms21061989 ![]() |
[84] |
Mavroudis IA, Petridis F, Chatzikonstantinou S, et al. (2020) A meta-analysis on CSF neurogranin levels for the diagnosis of Alzheimer's disease and mild cognitive impairment. Aging Clin Exp Res 32: 1639-1646. https://doi.org/10.1007/s40520-019-01326-z ![]() |
[85] |
Siddappaji KK, Gopal S (2021) Molecular mechanisms in Alzheimer's disease and the impact of physical exercise with advancements in therapeutic approaches. AIMS Neurosci 8: 357-389. https://doi.org/10.3934/Neuroscience.2021020 ![]() |
[86] |
Vaughn MN, Winston CN, Levin N, et al. (2022) Developing biomarkers of mild traumatic brain injury: Promise and progress of CNS-derived exosomes. Front Neurol 12: 698206. https://doi.org/10.3389/fneur.2021.698206 ![]() |
[87] |
Kivisäkk P, Carlyle BC, Sweeney T, et al. (2022) Increased levels of the synaptic proteins PSD-95, SNAP-25, and neurogranin in the cerebrospinal fluid of patients with Alzheimer's disease. Alzheimers Res Ther 14: 58. https://doi.org/10.1186/s13195-022-01002-x ![]() |
[88] |
Kent SA, Spires-Jones TL, Durrant CS (2020) The physiological roles of tau and Aβ: Implications for Alzheimer's disease pathology and therapeutics. Acta Neuropathol 140: 417-447. https://doi.org/10.1007/s00401-020-02196-w ![]() |
[89] | Bouwman FH, Frisoni GB, Johnson SC, et al. (2022) Clinical application of CSF biomarkers for Alzheimer's disease: From rationale to ratios. Alzheimers Dement 14: e12314. https://doi.org/10.1002/dad2.12314 |
[90] |
Saunders TS, Gadd DA, Spires-Jones TL, et al. (2022) Associations between cerebrospinal fluid markers and cognition in ageing and dementia: A systematic review. Eur J Neurosci 56: 5650-5713. https://doi.org/10.1111/ejn.15656 ![]() |
[91] |
Lepeta K, Lourenco MV, Schweitzer BC, et al. (2016) Synaptopathies: Synaptic dysfunction in neurological disorders–A review from students to students. J Neurochem 138: 785-805. https://doi.org/10.1111/jnc.13713 ![]() |
1. | Leticia Gómez-Sánchez, Marta Gómez-Sánchez, Luis García-Ortiz, Cristina Agudo-Conde, Cristina Lugones-Sánchez, Susana Gonzalez-Sánchez, Emiliano Rodríguez-Sánchez, Manuel A. Gómez-Marcos, The Relationship between the Mediterranean Diet and Vascular Stiffness, Metabolic Syndrome, and Its Components in People over 65 Years of Age, 2024, 16, 2072-6643, 3464, 10.3390/nu16203464 | |
2. | Chen Zhu, Shalini Arunogiri, Elizabeth H. X. Thomas, Qi Li, Jayashri Kulkarni, Caroline Gurvich, The development and evaluation of a fact sheet resource for women managing menopausal-related cognitive complaints, 2024, 31, 1530-0374, 987, 10.1097/GME.0000000000002434 | |
3. | Tamlyn J. Watermeyer, Sarah Gregory, Emmi Leetham, Chinedu T. Udeh-Momoh, Graciela Muniz-Terrera, Hormone replacement therapy, menopausal age and lifestyle variables are associated with better cognitive performance at follow-up but not cognition over time in older-adult women irrespective of APOE4 carrier status and co-morbidities, 2025, 3, 2813-3919, 10.3389/frdem.2024.1496051 | |
4. | Deeksha Malhan, Müge Yalçin, Susanne Liedtke, Rayka Grötsch, Christina Enzmann, Monika Rau, Angela Relógio, A prospective study to investigate circadian rhythms as health indicator in women’s aging, 2025, 3, 2948-1716, 10.1038/s44294-025-00057-z | |
5. | Hazem Ayesh, Samar A. Nasser, Keith C. Ferdinand, Barbara Gisella Carranza Leon, Sex-Specific Factors Influencing Obesity in Women: Bridging the Gap Between Science and Clinical Practice, 2025, 136, 0009-7330, 594, 10.1161/CIRCRESAHA.124.325535 |
First Author, publication year | Study design | Country | Participants |
Arms | Age (years, mean ± sd) | BMI (kg/m2, mean ± sd) | |
Randomized | Analysed | ||||||
Bihuniak JD, 2016 [21] | Controlled before-and-after study | United States of America | - | 16 | One arm: MD | 77.0±6.8 | 26.1±3.1 |
Rodríguez AS, 2016 [15] | Randomized clinical trial | Spain | 320 | 230 | Arm 1: intervention group MD Arm 2: control group |
53.2±4.3 | 27.6±5.5 27.3±5.3 |
Vignini A, 2017 [17] | Randomized clinical trial | Italy | 60 | 60 | Arm 1: intervention group supplemented with oral extra virgin olive oil (VOO) enriched with vitamins D3, K1 and B6. Arm 2: control group with oral supplementation with VOO |
54.6±3.7 55.6±2.6 |
25.2±2.7 25.9±3.1 |
Bajerska J, 2018 [20] | Randomized clinical trial | Poland | 144 | 130 | Arm 1: Mediterranean diet (MED) Arm 2: Central European diet (CED) |
60.3±4.7 60.8±4.7 |
33.8±5.6 33.6±4.2 |
Duś-Żuchowska M, 2018 [18] | |||||||
Muzsik A, 2019 [19] | |||||||
Lombardo M, 2020 [16] | Case-control study | Italy | 89 | 89 | Arm 1: Menopausal women Arm 2: Fertile women over 45 years of age |
57.1±4.9 48.8±4.0 |
30.6±5.4 29.5±5.0 |
Note: BMI: Body mass index; DRI: Dietary reference intake; CVD: Cardiovascular diseases; NR: Not reported; MD: Mediterranean diet; CED: Central European diet; VOO: Virgin olive oil.
First author, publication year | Intervention length | Follow-up (months) | Recruitment | Randomization | Intervention characteristics | Outcomes |
||||
Anthropo-metric | Dietary | Blood | Blood pressure | Genetic | ||||||
Bihuniak JD, 2016 [21] | 12 weeks | 2 years | Convenience | No | Advised by a dietitian on how to follow a MD, and substitute sources of saturated fat and refined carbohydrates by extra virgin olive oil (3 T/day), walnuts (1.5 oz/day), and fatty fish (3–5 servings/wk) which were provided at 3-week intervals. | Yes | Yes | Yes | No | No |
Rodríguez AS, 2016 [15] | 1 week | 1 year | Recruited in two primary health centres in one province | Yes | Intervention group: receive 3 interactive educational workshops (cardiovascular disease, maintenance of healthy habits and MD). Control group: participants received information pamphlets about MD. |
Yes | Yes | No | No | No |
Vignini A, 2017 [17] | 1 year | 1 year | Recruitment was performed in the ambulatory centre of the local hospital | Yes | Intervention group: oral supplementation with 20 mL/d extra virgin olive oil (VOO) enriched with vitamins D3 (100% RDA), K1 (100% RDA), and B6 (30% RDA). Control group: oral supplementation with 20 mL/d VOO. Standardized MD prescribed for both groups. |
Yes | No | Yes | No | No |
Bajerska J, 2018 [20] | 16 weeks | 16 weeks | Recruited through advertisements | Yes, stratified for BMI | Both groups were advised to follow a hypocaloric diet without added salt, refined fats and sugar; and to maintain the usual level of physical activity. During the intervention period, participants picked up packaged main meals and the other meals were prepared by the participants according to the prescribed instructions. MED group: followed a MD food plan. Olive oil and nuts were consumed every day. The proportion of soluble to insoluble fiber was 20–80%. CED group: followed a CED food plan, with a special focus on high levels of fiber. The proportion of soluble to insoluble fiber was 35–65%. |
Yes | Yes | Yes | Yes | No |
Duś-Żuchowska M, 2018 [18] | No | No | Yes | No | No | |||||
Muzsik A, 2019 [19] | No | No | Yes | No | Yes | |||||
Lombardo M, 2020 [16] | 2 months | 2 months | Convenience, recruited in nutrition clinic | No | All participants were advised to follow a two-month hypocaloric, moderate fat and MD balanced diet individually tailored, with the use of herbs and spices instead of salt, reduction of red meat to less than twice a week and to eat fish at least twice a week. The main sources of daily added fat were 25–35 g of olive oil and 20 g of nuts per day. It was recommended to only performed minimal aerobic training. |
Yes | No | Yes | Yes | No |
Note: NR: Not reported; MD: Mediterranean diet; BMI: Body mass index; CED: Central European diet group; MED: Mediterranean diet group; VOO: Virgin olive oil
First Author, publication year | Body Composition |
Blood Pressure |
||||||||||||||
Weight (kg) |
Body Mass Index (Kg/m2) |
Total fat mass (kg) |
Visceral fat (kg) |
Fat-free mass (kg) |
Waist circumference (cm) |
SBP (mmHg) |
DBP (mmHg) |
|||||||||
Baseline | Mean change | Baseline | Mean change | Baseline | Mean change | Baseline | Mean change | Baseline | Mean change | Baseline | Mean change | Baseline | Mean change | Baseline | Mean change | |
Bihuniak JD, 2016 [21] | 65.5±9.5 | -0.2# | 26.1±3.1 | -0.5# | NR | NR | NR | NR | NR | NR | NR | NR | NR | NR | NR | NR |
Rodríguez AS, 2016 [15] | IG NR | NR | 27.6±5.5 | +0.1 | 24.8±9.9 | +0.2 | 7.8±3.0 | +0.2* | NR | NR | 92.9±13.3 | -0.1 | NR | NR | NR | NR |
CG NR | 27.3±5.3 | +0.5* | 24.8±9.2 | +1.3* | 7.7±2.8 | +0.5* | 93.4±13.6 | +1.6* | ||||||||
Vignini A, 2017 [17] | PlaVOO NR |
NR | 25.2±2.7 | -0.2# | NR | NR | NR | NR | NR | NR | NR | NR | NR | NR | NR | NR |
VitVOO NR |
25.9±3.1 | -1.7# | ||||||||||||||
Bajerska J, 2018 [20] | MED 87.0±NR | −7.7* | NR | NR | 40.4±NR | −6.6* | 1.1±NR | −0.3* | 46.6±NR | −1.1* | 105.0±NR | −7.4* | 140.9±NR | −10.2* | 87.0±NR | −6.7* |
CED 85.3±NR | −7.5 | 39.5±NR | −6.7 | 1.1±NR | −0.3 | 45.8±NR | −0.8* | 105.4±NR | −7.5 | 142.1±NR | −10.4 | 86.9±NR | −8.1 | |||
Lombardo M, 2020 [16] | MEN 80.8±16.2 |
-3.7 | 30.6±5.4 | -0.9 | 33.4±11.1 | -2.4 | NR | NR | 45.1±5.4 | -0.6 | 97.5±15.3 | -3.1 | 133.8±17.2 | -9 | 85.2±13.0 | -7 |
REG 77.0±14.1 |
-3.1 | 29.5±5.0 | -0.8 | 29.9±9.7 | -2.2 | 44.9±5.3 | -0.8 | 92.2±11.5 | -3.2 | 124.6±13.3 | -6.8 | 80.6±12.8 | -3.3 |
Note: Values are reported as: mean ± standard deviation, unless otherwise specified. NR: Not reported; NO: Values are not an outcome; TEI: Total energy intake; PUFA: Polyunsaturated fatty acids; MUFA: Monounsaturated fatty acids; VitVO: Vitaminized virgin olive oil group; PlaVOO: Placebo virgin olive oil group; MED: Group with mediterranean diet; CED: Group with central european diet; IG: Intervention group; CG: Control group; MEN: Menopausal women group; REG: Not menopausal; SBP: Systolic blood pressure; DBP: Diastolic blood pressure; #: mean change was computed as the difference in means between baseline and follow-up using data from the publication; *p < 0.05.
First Author, publication year | Dietary |
|||||||||||||||||
Med Diet adherence |
Energy (kcal/d) |
Fat (%TEI) |
Saturated fat (%TEI) |
PUFA (%TEI) |
MUFA (%TEI) |
ω6: ω3 ratio |
Carbohydrates (%TEI) |
Fiber (g) |
||||||||||
Baseline | Mean change | Baseline | Mean change | Baseline | Mean change | Baseline | Mean change | Baseline | Mean change | Baseline | Mean change | Baseline | Mean change | Baseline | Mean change | Baseline | Mean change | |
Bihuniak JD, 2016 [21] | 32.3±4.3 | +9.0#* | 1679±231 | +124# | 31.4±5.6 | +9.3#* | 10.3±2.4 | -0.7# | NR | NR | NR | NR | 8.2±5.2 | -3.7#* | 51.5±7.2 | -7.0#* | 20.3±7.4 | +1# |
Rodríguez AS, 2016 [15] | IG 7.06±2.02 | +2.31#* | NR | NR | NR | NR | NR | NR | NR | NR | NR | NR | NR | NR | NR | NR | NR | NR |
CG 6.96±2.15 | +0.15 | |||||||||||||||||
Bajerska J, 2018 [20] | MED NR | NR | 1912±NR | -574* | 36.1±NR | +0.3* | 16.9±NR | -8.4* | 5.2±NR | +3.8* | 14.0±NR | +5.0 | NR | NR | 47.5±NR | -2.5* | 21.0±NR | +11.7* |
CED NR | NR | 1936±NR | -574 | 35.9±NR | -8.5 | 17.4±NR | -8.6 | 5.2±NR | +3.9 | 13.2±NR | -3.9 | 48.6±NR | +5.7 | 21.7±NR | +17.6 |
Note: Values are reported as: mean ± standard deviation, unless otherwise specified. NR: not reported; TEI: Total energy intake; PUFA: Polyunsaturated fatty acids; MUFA: Monounsaturated fatty acids; MED: Group with mediterranean diet; CED: Group with central european diet; IG: Intervention group; CG: Control group; #: mean change was computed as the difference in means between baseline and follow-up using data from the publication; *p < 0.05.
First author, publication year | Blood |
|||||||||||||||||
ω6: ω3 ratio |
Triglycerides (mg/dL) |
Total cholesterol (mg/dL) |
HDL (mg/dL) |
LDL (mg/dL) |
Fasting glucose (mg/dL) |
Homocysteine (µM) |
CRP (mg/L) |
NO (nmol NO/ mg protein) |
||||||||||
Baseline | Mean change | Baseline | Mean change | Baseline | Mean change | Baseline | Mean change | Baseline | Mean change | Baseline | Mean change | Baseline | Mean change | Baseline | Mean change | Baseline | Mean change | |
Bihuniak JD, 2016 [21] | 10.4±1.9 | -1.5#* | 99.8±34.8 | -12.5 #* | 205.5±31.9 | -2.3# | 68.1±13.0 | -4.0#* | 117.6±25.6 | -4.0# | NR | NR | NR | NR | NR | NR | NR | NR |
Vignini A, 2017 [17] | NR | NR | VitVO 125.2±46.9 | -5.8# | 219.6±34.3 | -15.3# | 58.6±14.1 | +7.1#* | 133.1±33.7 | -5.5# | 82.5±9.0 | -0.8# | NR | NR | NR | NR | 43.8±4.9 | -6.6#* |
PlaVO 127.2±44.3 | -12.0# | 218.3±33.5 | -12.7# | 57.6±15.3 | +6.8#* | 132.4±32.4 | -3.9# | 83.5±9.0 | -2.0# | 41.9±4.2 | +0.7# | |||||||
Bajerska J, 2018 [20] | MED NR | NR | 157.4±NR | -33.9* | 235.8±NR | -15.5* | 55.3±NR | -0.1 | 149.7±NR | -9.4 | 98.0±NR | -6.4* | 11.5±NR | +0.7* | NR | NR | NR | NR |
CED NR | NR | 164.1±NR | -38.8 | 226.4±NR | -11.2 | 53.1±NR | -2.0 | 143.1±NR | -4.9 | 98.1±NR | -5.4 | 10.9±NR | +0.8 | NR | NR | NR | NR | |
Duś-Żuchowska M, 2018 [18] | NR | NR | NR | NR | NR | NR | NR | NR | NR | NR | NR | NR | NR | NR | MED 4.5±4.5 | -1.2* | NR | NR |
CED 4.5±4.4 | -0.9* | |||||||||||||||||
Muzsik A, 2019 [19] | MED 2.5±0.7∥ | -0.2 | NR | NR | NR | NR | NR | NR | NR | NR | NR | NR | NR | NR | NR | NR | NR | NR |
CED 2.4±0.7∥ | +0.01 | |||||||||||||||||
Lombardo M, 2020 [16] | NR | NR | MEN 108.3±65.2 | -22.9 | 219.6±41.7 | -22.8 | 58.3±12.6 | +6.0 | 143.6±36.2 | -28.2* | 92.7±14.0 | -2.5 | NR | NR | NR | NR | NR | NR |
REG 92.0±39.0 | +8.2 | 202.9±35.5 | -9.3 | 58.9±16.4 | +2.2 | 130.3±29.4 | -7.7 | 92.4±9.4 | -2.2 |
Note: Values are reported as: mean ± standard deviation, unless otherwise specified. NR: Not reported; HDL: High density lipoprotein; LDL: Low density lipoprotein; CRP: C-reactive protein; NO: Nitric oxide level; MED: Mediterranean diet group; CED: Central european diet group; MEN: Menopausal women group; REG: Not menopausal; VitVO: Vitaminized virgin olive oil group; PlaVO: Placebo virgin olive oil group; IG: Intervention group; CG: Control group; ∥: values obtained in red blood cells; #: mean change was computed as the difference in means between baseline and follow-up using data from the publication; *p < 0.05.
First Author, publication year | Study design | Country | Participants |
Arms | Age (years, mean ± sd) | BMI (kg/m2, mean ± sd) | |
Randomized | Analysed | ||||||
Bihuniak JD, 2016 [21] | Controlled before-and-after study | United States of America | - | 16 | One arm: MD | 77.0±6.8 | 26.1±3.1 |
Rodríguez AS, 2016 [15] | Randomized clinical trial | Spain | 320 | 230 | Arm 1: intervention group MD Arm 2: control group |
53.2±4.3 | 27.6±5.5 27.3±5.3 |
Vignini A, 2017 [17] | Randomized clinical trial | Italy | 60 | 60 | Arm 1: intervention group supplemented with oral extra virgin olive oil (VOO) enriched with vitamins D3, K1 and B6. Arm 2: control group with oral supplementation with VOO |
54.6±3.7 55.6±2.6 |
25.2±2.7 25.9±3.1 |
Bajerska J, 2018 [20] | Randomized clinical trial | Poland | 144 | 130 | Arm 1: Mediterranean diet (MED) Arm 2: Central European diet (CED) |
60.3±4.7 60.8±4.7 |
33.8±5.6 33.6±4.2 |
Duś-Żuchowska M, 2018 [18] | |||||||
Muzsik A, 2019 [19] | |||||||
Lombardo M, 2020 [16] | Case-control study | Italy | 89 | 89 | Arm 1: Menopausal women Arm 2: Fertile women over 45 years of age |
57.1±4.9 48.8±4.0 |
30.6±5.4 29.5±5.0 |
First author, publication year | Intervention length | Follow-up (months) | Recruitment | Randomization | Intervention characteristics | Outcomes |
||||
Anthropo-metric | Dietary | Blood | Blood pressure | Genetic | ||||||
Bihuniak JD, 2016 [21] | 12 weeks | 2 years | Convenience | No | Advised by a dietitian on how to follow a MD, and substitute sources of saturated fat and refined carbohydrates by extra virgin olive oil (3 T/day), walnuts (1.5 oz/day), and fatty fish (3–5 servings/wk) which were provided at 3-week intervals. | Yes | Yes | Yes | No | No |
Rodríguez AS, 2016 [15] | 1 week | 1 year | Recruited in two primary health centres in one province | Yes | Intervention group: receive 3 interactive educational workshops (cardiovascular disease, maintenance of healthy habits and MD). Control group: participants received information pamphlets about MD. |
Yes | Yes | No | No | No |
Vignini A, 2017 [17] | 1 year | 1 year | Recruitment was performed in the ambulatory centre of the local hospital | Yes | Intervention group: oral supplementation with 20 mL/d extra virgin olive oil (VOO) enriched with vitamins D3 (100% RDA), K1 (100% RDA), and B6 (30% RDA). Control group: oral supplementation with 20 mL/d VOO. Standardized MD prescribed for both groups. |
Yes | No | Yes | No | No |
Bajerska J, 2018 [20] | 16 weeks | 16 weeks | Recruited through advertisements | Yes, stratified for BMI | Both groups were advised to follow a hypocaloric diet without added salt, refined fats and sugar; and to maintain the usual level of physical activity. During the intervention period, participants picked up packaged main meals and the other meals were prepared by the participants according to the prescribed instructions. MED group: followed a MD food plan. Olive oil and nuts were consumed every day. The proportion of soluble to insoluble fiber was 20–80%. CED group: followed a CED food plan, with a special focus on high levels of fiber. The proportion of soluble to insoluble fiber was 35–65%. |
Yes | Yes | Yes | Yes | No |
Duś-Żuchowska M, 2018 [18] | No | No | Yes | No | No | |||||
Muzsik A, 2019 [19] | No | No | Yes | No | Yes | |||||
Lombardo M, 2020 [16] | 2 months | 2 months | Convenience, recruited in nutrition clinic | No | All participants were advised to follow a two-month hypocaloric, moderate fat and MD balanced diet individually tailored, with the use of herbs and spices instead of salt, reduction of red meat to less than twice a week and to eat fish at least twice a week. The main sources of daily added fat were 25–35 g of olive oil and 20 g of nuts per day. It was recommended to only performed minimal aerobic training. |
Yes | No | Yes | Yes | No |
First Author, publication year | Body Composition |
Blood Pressure |
||||||||||||||
Weight (kg) |
Body Mass Index (Kg/m2) |
Total fat mass (kg) |
Visceral fat (kg) |
Fat-free mass (kg) |
Waist circumference (cm) |
SBP (mmHg) |
DBP (mmHg) |
|||||||||
Baseline | Mean change | Baseline | Mean change | Baseline | Mean change | Baseline | Mean change | Baseline | Mean change | Baseline | Mean change | Baseline | Mean change | Baseline | Mean change | |
Bihuniak JD, 2016 [21] | 65.5±9.5 | -0.2# | 26.1±3.1 | -0.5# | NR | NR | NR | NR | NR | NR | NR | NR | NR | NR | NR | NR |
Rodríguez AS, 2016 [15] | IG NR | NR | 27.6±5.5 | +0.1 | 24.8±9.9 | +0.2 | 7.8±3.0 | +0.2* | NR | NR | 92.9±13.3 | -0.1 | NR | NR | NR | NR |
CG NR | 27.3±5.3 | +0.5* | 24.8±9.2 | +1.3* | 7.7±2.8 | +0.5* | 93.4±13.6 | +1.6* | ||||||||
Vignini A, 2017 [17] | PlaVOO NR |
NR | 25.2±2.7 | -0.2# | NR | NR | NR | NR | NR | NR | NR | NR | NR | NR | NR | NR |
VitVOO NR |
25.9±3.1 | -1.7# | ||||||||||||||
Bajerska J, 2018 [20] | MED 87.0±NR | −7.7* | NR | NR | 40.4±NR | −6.6* | 1.1±NR | −0.3* | 46.6±NR | −1.1* | 105.0±NR | −7.4* | 140.9±NR | −10.2* | 87.0±NR | −6.7* |
CED 85.3±NR | −7.5 | 39.5±NR | −6.7 | 1.1±NR | −0.3 | 45.8±NR | −0.8* | 105.4±NR | −7.5 | 142.1±NR | −10.4 | 86.9±NR | −8.1 | |||
Lombardo M, 2020 [16] | MEN 80.8±16.2 |
-3.7 | 30.6±5.4 | -0.9 | 33.4±11.1 | -2.4 | NR | NR | 45.1±5.4 | -0.6 | 97.5±15.3 | -3.1 | 133.8±17.2 | -9 | 85.2±13.0 | -7 |
REG 77.0±14.1 |
-3.1 | 29.5±5.0 | -0.8 | 29.9±9.7 | -2.2 | 44.9±5.3 | -0.8 | 92.2±11.5 | -3.2 | 124.6±13.3 | -6.8 | 80.6±12.8 | -3.3 |
First Author, publication year | Dietary |
|||||||||||||||||
Med Diet adherence |
Energy (kcal/d) |
Fat (%TEI) |
Saturated fat (%TEI) |
PUFA (%TEI) |
MUFA (%TEI) |
ω6: ω3 ratio |
Carbohydrates (%TEI) |
Fiber (g) |
||||||||||
Baseline | Mean change | Baseline | Mean change | Baseline | Mean change | Baseline | Mean change | Baseline | Mean change | Baseline | Mean change | Baseline | Mean change | Baseline | Mean change | Baseline | Mean change | |
Bihuniak JD, 2016 [21] | 32.3±4.3 | +9.0#* | 1679±231 | +124# | 31.4±5.6 | +9.3#* | 10.3±2.4 | -0.7# | NR | NR | NR | NR | 8.2±5.2 | -3.7#* | 51.5±7.2 | -7.0#* | 20.3±7.4 | +1# |
Rodríguez AS, 2016 [15] | IG 7.06±2.02 | +2.31#* | NR | NR | NR | NR | NR | NR | NR | NR | NR | NR | NR | NR | NR | NR | NR | NR |
CG 6.96±2.15 | +0.15 | |||||||||||||||||
Bajerska J, 2018 [20] | MED NR | NR | 1912±NR | -574* | 36.1±NR | +0.3* | 16.9±NR | -8.4* | 5.2±NR | +3.8* | 14.0±NR | +5.0 | NR | NR | 47.5±NR | -2.5* | 21.0±NR | +11.7* |
CED NR | NR | 1936±NR | -574 | 35.9±NR | -8.5 | 17.4±NR | -8.6 | 5.2±NR | +3.9 | 13.2±NR | -3.9 | 48.6±NR | +5.7 | 21.7±NR | +17.6 |
First author, publication year | Blood |
|||||||||||||||||
ω6: ω3 ratio |
Triglycerides (mg/dL) |
Total cholesterol (mg/dL) |
HDL (mg/dL) |
LDL (mg/dL) |
Fasting glucose (mg/dL) |
Homocysteine (µM) |
CRP (mg/L) |
NO (nmol NO/ mg protein) |
||||||||||
Baseline | Mean change | Baseline | Mean change | Baseline | Mean change | Baseline | Mean change | Baseline | Mean change | Baseline | Mean change | Baseline | Mean change | Baseline | Mean change | Baseline | Mean change | |
Bihuniak JD, 2016 [21] | 10.4±1.9 | -1.5#* | 99.8±34.8 | -12.5 #* | 205.5±31.9 | -2.3# | 68.1±13.0 | -4.0#* | 117.6±25.6 | -4.0# | NR | NR | NR | NR | NR | NR | NR | NR |
Vignini A, 2017 [17] | NR | NR | VitVO 125.2±46.9 | -5.8# | 219.6±34.3 | -15.3# | 58.6±14.1 | +7.1#* | 133.1±33.7 | -5.5# | 82.5±9.0 | -0.8# | NR | NR | NR | NR | 43.8±4.9 | -6.6#* |
PlaVO 127.2±44.3 | -12.0# | 218.3±33.5 | -12.7# | 57.6±15.3 | +6.8#* | 132.4±32.4 | -3.9# | 83.5±9.0 | -2.0# | 41.9±4.2 | +0.7# | |||||||
Bajerska J, 2018 [20] | MED NR | NR | 157.4±NR | -33.9* | 235.8±NR | -15.5* | 55.3±NR | -0.1 | 149.7±NR | -9.4 | 98.0±NR | -6.4* | 11.5±NR | +0.7* | NR | NR | NR | NR |
CED NR | NR | 164.1±NR | -38.8 | 226.4±NR | -11.2 | 53.1±NR | -2.0 | 143.1±NR | -4.9 | 98.1±NR | -5.4 | 10.9±NR | +0.8 | NR | NR | NR | NR | |
Duś-Żuchowska M, 2018 [18] | NR | NR | NR | NR | NR | NR | NR | NR | NR | NR | NR | NR | NR | NR | MED 4.5±4.5 | -1.2* | NR | NR |
CED 4.5±4.4 | -0.9* | |||||||||||||||||
Muzsik A, 2019 [19] | MED 2.5±0.7∥ | -0.2 | NR | NR | NR | NR | NR | NR | NR | NR | NR | NR | NR | NR | NR | NR | NR | NR |
CED 2.4±0.7∥ | +0.01 | |||||||||||||||||
Lombardo M, 2020 [16] | NR | NR | MEN 108.3±65.2 | -22.9 | 219.6±41.7 | -22.8 | 58.3±12.6 | +6.0 | 143.6±36.2 | -28.2* | 92.7±14.0 | -2.5 | NR | NR | NR | NR | NR | NR |
REG 92.0±39.0 | +8.2 | 202.9±35.5 | -9.3 | 58.9±16.4 | +2.2 | 130.3±29.4 | -7.7 | 92.4±9.4 | -2.2 |