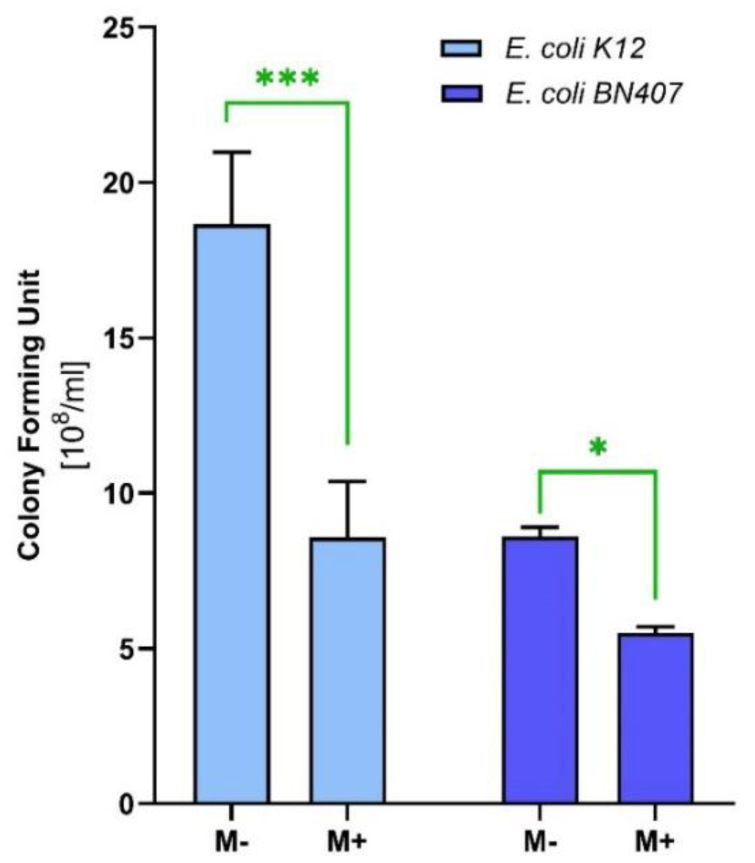
In this investigation, we assessed the antibacterial properties of menthol using Escherichia coli strains, including a tetracycline-resistant (BN407) and a non-resistant reference (K12) strains. The disc-diffusion assay indicated remarkable antibacterial activity of menthol, warranting further exploration. The minimum inhibitory concentration (MIC) for menthol was determined to be 500 µg/ml for both strains. Despite identical MIC values, menthol exhibited different effects on the colony-forming units (CFUs) of the strains, reducing CFUs by 55% in E. coli K12 and 40% in E. coli BN407.
Growth kinetics studies revealed that menthol extended the Lag phase by 50% and decreased the specific growth rate and mean generation time by nearly 50% for both strains. These findings illustrate menthol's significant impact on bacterial replication and adaptation processes. Additionally, menthol disrupted membrane-associated properties, as evidenced by reduced H+-flux through bacterial membranes, affecting both N,N-Dicyclohexylcarbodiimide (DCCD)-sensitive and non-sensitive proton flux rates. This indicates that menthol compromises the proton motive force critical for ATP synthesis and nutrient transport.
In summary, menthol demonstrates potent antibacterial activity, influencing bacterial growth and survival. This activity is supposed to be due to the influence on membrane functionality. These effects are consistent across both tetracycline-resistant and non-resistant E. coli strains.
Citation: Silvard Tadevosyan, Naira Sahakyan. Influence of menthol on membrane-associated properties of tetracycline-resistant Escherichia coli[J]. AIMS Biophysics, 2024, 11(3): 329-339. doi: 10.3934/biophy.2024018
[1] | Armenuhi Moghrovyan, Naira Sahakyan . Antimicrobial activity and mechanisms of action of Origanum vulgare L. essential oil: effects on membrane-associated properties. AIMS Biophysics, 2024, 11(4): 508-526. doi: 10.3934/biophy.2024027 |
[2] | Jaouhra Cherif, Anis Raddaoui, Ghofrane Ben Fraj, Asma Laabidi, Nada Souissi . Escherichia coli's response to low-dose ionizing radiation stress. AIMS Biophysics, 2024, 11(2): 130-141. doi: 10.3934/biophy.2024009 |
[3] | Gayane Semerjyan, Inesa Semerjyan, Mikayel Ginovyan, Nikolay Avtandilyan . Characterization and antibacterial/cytotoxic activity of silver nanoparticles synthesized from Dicranum scoparium moss extracts growing in Armenia. AIMS Biophysics, 2025, 12(1): 29-42. doi: 10.3934/biophy.2025003 |
[4] | Abdulrahaman Mahmoud Dogara, Ateeq Ahmed Al-Zahrani, Sarwan W. Bradosty, Saber W. Hamad, Shorsh Hussein Bapir, Talar K. Anwar . Antioxidant, α-glucosidase, antimicrobial activities chemical composition and in silico analysis of eucalyptus camaldulensis dehnh. AIMS Biophysics, 2024, 11(3): 255-280. doi: 10.3934/biophy.2024015 |
[5] | Mikayel Ginovyan, Svetlana Hovhannisyan, Hayarpi Javrushyan, Gohar Sevoyan, Zaruhi Karabekian, Narine Zakaryan, Naira Sahakyan, Nikolay Avtandilyan . Screening revealed the strong cytotoxic activity of Alchemilla smirnovii and Hypericum alpestre ethanol extracts on different cancer cell lines. AIMS Biophysics, 2023, 10(1): 12-22. doi: 10.3934/biophy.2023002 |
[6] | Thi Minh Ngoc Ta, Cynthia Romero-Guido, Thi Hanh Phan, Hai Dang Tran, Hanh Tam Dinh, Yves Waché . Encapsulation of flavours into Yarrowia lipolytica active yeast cells. Fluorescence study of the lipid droplets morphology and steryl/sterol balance during the shock. AIMS Biophysics, 2022, 9(3): 257-270. doi: 10.3934/biophy.2022022 |
[7] | Etimad Alattar, Khitam Alwasife, Eqbal Radwan . Effects of treated water with neodymium magnets (NdFeB) on growth characteristics of pepper (Capsicum annuum). AIMS Biophysics, 2020, 7(4): 267-290. doi: 10.3934/biophy.2020021 |
[8] | Mahmoud Abdallat, Abdallah Barjas Qaswal, Majed Eftaiha, Abdel Rahman Qamar, Qusai Alnajjar, Rawand Sallam, Lara Kollab, Mohammad Masa'deh, Anas Amayreh, Hiba Mihyar, Hesham Aboushakra, Bayan Alkelani, Rawan Owaimer, Mohannad Abd-Alhadi, Salwa Ireiqat, Fahed Turk, Ahmad Daoud, Bashar Darawsheh, Ahmad Hiasat, Majd Alhalaki, Shahem Abdallat, Salsabiela Bani Hamad, Rand Murshidi . A mathematical modeling of the mitochondrial proton leak via quantum tunneling. AIMS Biophysics, 2024, 11(2): 189-233. doi: 10.3934/biophy.2024012 |
[9] | Morteza Vaezi, G. Rezaei Behbehani, Nematollah Gheibi, Alireza Farasat . Thermodynamic, kinetic and docking studies of some unsaturated fatty acids-quercetin derivatives as inhibitors of mushroom tyrosinase. AIMS Biophysics, 2020, 7(4): 393-410. doi: 10.3934/biophy.2020027 |
[10] | James C. Walsh, Christopher N. Angstmann, Anna V. McGann, Bruce I. Henry, Iain G. Duggin, Paul M. G. Curmi . Patterning of the MinD cell division protein in cells of arbitrary shape can be predicted using a heuristic dispersion relation. AIMS Biophysics, 2016, 3(1): 119-145. doi: 10.3934/biophy.2016.1.119 |
In this investigation, we assessed the antibacterial properties of menthol using Escherichia coli strains, including a tetracycline-resistant (BN407) and a non-resistant reference (K12) strains. The disc-diffusion assay indicated remarkable antibacterial activity of menthol, warranting further exploration. The minimum inhibitory concentration (MIC) for menthol was determined to be 500 µg/ml for both strains. Despite identical MIC values, menthol exhibited different effects on the colony-forming units (CFUs) of the strains, reducing CFUs by 55% in E. coli K12 and 40% in E. coli BN407.
Growth kinetics studies revealed that menthol extended the Lag phase by 50% and decreased the specific growth rate and mean generation time by nearly 50% for both strains. These findings illustrate menthol's significant impact on bacterial replication and adaptation processes. Additionally, menthol disrupted membrane-associated properties, as evidenced by reduced H+-flux through bacterial membranes, affecting both N,N-Dicyclohexylcarbodiimide (DCCD)-sensitive and non-sensitive proton flux rates. This indicates that menthol compromises the proton motive force critical for ATP synthesis and nutrient transport.
In summary, menthol demonstrates potent antibacterial activity, influencing bacterial growth and survival. This activity is supposed to be due to the influence on membrane functionality. These effects are consistent across both tetracycline-resistant and non-resistant E. coli strains.
essential oil;
compound annual growth rate;
Specific growth rate;
wild type;
standard deviation;
minimal inhibitory concentration;
gas chromatography mass spectrometry
The problem of bacterial resistance against antibiotics is a worldwide challenge for modern medicine and veterinary. The issue is now under the focus of multiple researchers and research groups, although no global solution has been suggested yet. Most antimicrobial substances are of microbial origin (around 97%), and only around 3% of this kind of preparations are the products of plant metabolism [1]. Although different plant species produce a vast number of biologically active substances, they can act as antimicrobials or antibiotic-resistance modifiers among which are several alkaloids, terpenoids, tannins, steroids, coumarins, and flavonoids [1]–[3].
In recent years, resistance has been reported to almost all antibacterial agents produced by microorganisms. However, the complex composition of the plant's biologically active extracts interacts with bacteria by multiple mechanisms instead of a particular single mode of action inhibiting the proliferation of bacterial cells [4]. The influence mechanisms of plant metabolites on bacterial cells are different: one of the most studied is related to triggering the ROS (Reactive Oxygen Species) and overproduction of other reactive species in bacterial cells [5], redox transformations and changes in intracellular pH. These factors are undoubtedly included in the activation or inhibition of other related processes such as proton efflux pump action, changes in ATP-ases activity, deviations in H+/K+ ratio, modulation of the synthesis and activity of different enzymes etc. [3],[6].
The Lamiaceae family plants are among the most applicable plant species in preventive medicine due to their expressed biological activity in vast number of test-systems [7],[8]. The great part of the representatives of this family are essential oil-producing plants, including those of Mentha genus. The essential oil of Mentha genus plants contains menthol as the main component. Menthol can be produced by Mentha piperita, M. arvensis, M. canadensis, M. rotundifolia, and other plants [9], but also can be chemically synthesized. This monoterpenoid metabolite possesses expressed biological activity in different test-systems, among which the most important is the antibacterial activity against vast number of pathogenic and non-pathogenic, gram-positive and gram-negative bacteria, both susceptible and resistant against different antibiotics [10].
Gram-negative bacteria are known for their ability to form biofilms, which contribute to their resistance to antibiotics. Menthol has been shown to inhibit biofilm formation, making the bacteria more susceptible to antimicrobial agents. Researchers also suggest that menthol can enhance the efficacy of certain antibiotics against gram-negative bacteria by weakening the bacterial defenses [11]. This combination can be particularly useful against multidrug-resistant strains. Menthol has been tested against various gram-negative bacteria, including different strains of E. coli, Pseudomonas aeruginosa, and Salmonella typhimurium. The results typically show that menthol expresses a bacteriostatic or bactericidal effect depending on the concentration and exposure time [10], [12]–[14].
Menthol exhibits significant antimicrobial activity against gram-negative bacteria through mechanisms such as membrane disruption, inhibition of biofilm formation, and synergy with antibiotics. Its application in various industries highlights its potential as a natural alternative to synthetic antimicrobials.
Despite some available information on the mechanisms of action of menthol on bacterial cells [15], there is a need to deeply understand the influence mode of this metabolite, especially the action against antibiotic-resistant bacteria.
The antibacterial activity of menthol (Carl Roth, Germany) was determined by the agar diffusion method [16], using cellulose disks (6 mm in diameter). Tetracycline-resistant E. coli BN407 and E. coli K12 (reference strain) were applied as test-organisms.
The test was performed using a solid peptone nutrient medium (peptone -2%, glucose -0.2%, NaCl -0.5%, K2HPO4 -0.2%, agar -1.7%, pH = 7.5). 31.25–1000 µg/mL of menthol were applied in the test. Ethanol (96%) and the antibiotic solution (tetracycline (15 µg/mL)) were used as negative controls, kanamycin (15 µg/mL)—as positive control. Plates were incubated for 24 h at 37 °C, and then antimicrobial activity of menthol was evaluated by the measurement of growth inhibition zone diameter (mm). Data were expressed in minimal inhibitory concentration (MIC) values.
Escherichia coli K12 (Wt, Keio collection) and E. coli BN407 containing plasmid ColV-K30 with iucC: lacZ fusion [17] received from Dr. J. Imlay [18] and kindly provided by Dr. Prof. Oktyabrskiy O.N. (Perm, RF).
Monoclonal cells from a single colony were transferred to the new liquid medium without agar and grown aerobically overnight at 37 °C and 150 rpm rotary shaking conditions. A total of 1.5% of the culture was inoculated in 200 mL liquid media and grown in the same conditions, aerobically. Mid-logarithmic stage cells were used for further experiments.
The effect of menthol on specific growth rate (µ), generation succeeding factor (G) of bacteria and the cell doubling time was determined by growing bacteria in the liquid media and measuring the density of a solution by DEN-1 McFarland Densitometer (Biosan, Latvia) as described. The specific growth rate (SGR) was calculated as the ratio of the logarithmic difference of doubled optical reading and doubling time when the bacteria growth curve was linear. The cell doubling time was calculated as the ln2/µ ratio [19].
Determination of the colony-forming units (CFU) was carried out under the influence of menthol by the Koch's method [20]. The test was performed using a solid peptone nutrient medium (the medium composition is described above). The control version was without menthol supplementation and the experimental version contained menthol at a concentration of 125 µg/mL as this was the lowest acting concentration in a broth-dilution assay.
Late logarithmic or early stationary growth phase cells were harvested by centrifugation (Sorvall LYNX 6000 Superspeed Centrifuge, Thermo Scientific, USA) at 3500 g for 10 min; then, the pellet was washed twice with distilled water. Pellet was resuspended in 150 mM Tris–HCl experimental assay (containing 0.4 mM MgSO4, 1 mM NaCl, and 1 mM KCl). H+ flux rate by whole cells was determined as described [21] upon the addition of glucose (2 g/L) and menthol (125 µg/mL), by registering the pH value changes using a pH-meter (Hanna Instruments /ORP/pH/TDS/EC Meter – HI5522-01, Italy) equipped with a selective H+ electrode (HI11310) [19]. The proton flux rate was calculated as the negative logarithm of proton concentration in millimolar value. In whole cells incubated with ATPase inhibitor (0.1 mM N, N′-dicyclohexylcarbodiimide (DCCD)), the same principle was applied. Results were expressed in mmol H+/min per 108 cells in 1 unit of volume (mL).
Chemicals: DL-Menthol (≥ 99 %, synth., CAS No. 89-78-1, Carl Roth GmbH Co. KG (Karlsruhe, Germany), ethanol (POCH S. A., Lot no. 1156/11/21, Gliwice, Poland), kanamycin sulfate(#066M4019V, Sigma-Aldrich, Taufkirchen, Germany), tetracyclin (Lot no. 60-54-8, Sigma-Aldrich, Taufkirchen, Germany), dimethyl sulfoxide (DMSO) (#BCCJ0028, Sigma-Aldrich, Taufkirchen, Germany), DCCD (CAS No. 538–75–0, Sigma-Aldrich, Taufkirchen, Germany).
All data presented represent averaged results of 3 independent biological replicates. The standard deviation of the data was determined according to the student's t-test, and it was less than 5%. p values were calculated by two-tail unpaired t-test using GraphPad Prism 8.0.2 (263) data analyzing tool. Graph presentations were carried out by GraphPad and Microsoft Excel 10 programs.
Our aim is to study some mechanisms of action of menthol on antibiotic-resistant bacterial cell membrane.
Our investigation showed that according to the disc-diffusion assay, menthol suppresses the growth of both tested bacterial strains.
According to our research data, the MIC values of menthol against both tested bacteria were 500 µg/ml. The action was evaluated as bacteriostatic at the tested concentrations. Further investigations revealed that despite the same MIC values against tetracycline-resistant E. coli BN407 and the reference E. coli K12 strains, the influence of menthol on CFU formation differs (Figure 1). This can be explained by the low sensitivity of the disk-diffusion test as well as the volatile nature of the tested metabolite.
The CFU ability reduced in both strains under the influence of menthol. In the case of E. coli K 12, it decreased by almost 55 %, while in the case of E. coli BN407, the ability of CFU decreased by almost 40% (Figure 1).
According to obtained data, the growth parameters of both bacterial strains changed under the influence of menthol (Figure 2). The Lag phase duration of both reference and tested antibiotic-resistant strain was extended by 50% (Figure 2a). Furthermore, the specific growth rate was decreased by almost 50% in case of both strains (Figure 3b). The same tendency was observed with the mean generation time (g), which was decreased by 50% in both E. coli strains (Figure 2b).
Menthol influences also membrane-associated properties of both bacterial strains (Figure 3). Both DCCD-sensitive and non-sensitive proton flux rates were significantly decreased under the influence of this metabolite in both tested bacterial strains (Figure 3). The DCCD-non-sensitive proton flux rate decreased by 43% in the antibiotic-resistant strain. Moreover, in the E.coli K12 strain, this parameter value decreased by 23% under the influence of menthol. The DCCD-sensitive proton flux rate increased under the influence of menthol in E.coli BN407 strain almost twice, and in the reference strain, the change was not very significant (approximately 12%).
The negative controls had no any effect on growth parameters of bacteria or proton flux rates through their membranes. The positive control, kanamycin, influences the RNA synthesis [22]. This feature allowed us to not consider this antibiotic in the tests revealing the influence on membrane-associated properties of bacteria.
Plants can be applied as sources for valuable metabolites both for pharmaceutical and other branches of industry [23],[24]. As mentioned, one of the most studied plant family is the Lamiaceae, which is known as a plant family widely applicable in preventive medicine, food and cosmetic industry and produces a vast number of biologically active metabolites [25],[8]. Menthol is among the biologically active substances produced by several members of this family [9]. It has been shown in our and other investigations that there is a strong antibacterial effect of menthol-bearing plants against several bacteria [26],[16], including both the tetracycline-resistant E. coli BN407 and the non-resistant E. coli K12 strains. The disk-diffusion assay showed the antibacterial effect, although the method's limitations suggest further evaluation of the results. Moreover, the MIC values obtained indicated that a concentration of 500 µg/mL of menthol was sufficient to inhibit the growth of both bacterial strains. This consistency in MIC values across strains, despite their differing resistance profiles, underscores possibly the same mechanism of action of menthol in both strains. However, the MIC value alone does not fully encapsulate the antimicrobial efficacy or the differential impact. While the MIC values were identical for both strains, the CFU assay demonstrated varied sensitivity to menthol in the tested bacterial strains. In the case of the non-resistant E. coli K12 strain, menthol reduced CFU by approximately 55% compared to a 40% reduction in the tetracycline-resistant E. coli BN407 strain. This discrepancy highlights that although the disk-diffusion assay and MIC values indicate a strong antibacterial effect, they may not fully reflect the differential impacts observed in other aspects of bacterial growth and survival. The CFU assay, which measures the ability of bacteria to survive and proliferate after exposure to antibacterial agents, provides insight into the bactericidal or bacteriostatic nature of the compound. The action of this metabolite was bacteriostatic in both strains.
The growth kinetics of both E. coli strains under the influence of menthol at MIC were significantly altered, as shown in Figure 3. Menthol extended the Lag phase duration by 50% in both strains. This phase is critical as it represents the period of adaptation where bacteria adjust to new conditions before commencing exponential growth [27]. An extended Lag phase indicates that menthol disrupts bacterial adaptation processes, delaying the onset of active growth.
Moreover, the specific growth rate, which measures the rate at which bacteria reproduce during the exponential phase, was reduced by nearly 50% in both strains. This significant decrease points to menthol's capacity to impede bacterial replication efficiently, regardless of the strain's resistance profile.
The mean generation time (g), which is the time required for the bacterial population to double, also saw a substantial increase by almost 50%. This prolongation suggests that menthol significantly slows the bacterial cell division process, affecting both resistant and non-resistant strains similarly.
Menthol's influence extends beyond just inhibiting bacterial growth and CFU formation; it also affects membrane-associated properties, as evidenced by the H+-flux assays in Figure 3. The membrane potential and proton gradients are critical for various cellular processes, including ATP production and nutrient transport [28],[29].
The H+-flux through the bacterial membranes, both DCCD-sensitive and non-sensitive, was markedly decreased under the influence of menthol. DCCD is an inhibitor of ATP synthase, and by assessing the proton flux in the presence and absence of this inhibitor, we can infer the impact on energy production pathways [30],[31]. The reduction in proton fluxes indicates that menthol disrupts the proton motive force, which is essential for ATP synthesis and other cellular processes [32]. This disruption could be a key factor in the bacteriostatic or bactericidal activity of menthol. By impairing the bacterial cell membrane's function, menthol likely compromises the cells' energy generation and nutrient uptake, leading to the observed reductions in growth rate and CFU formation.
The observed differences in the impact of menthol on the tetracycline-resistant E. coli BN407 and the reference E. coli K12 highlight the complexity of antimicrobial action. The greater reduction in CFU and growth rate for the non-resistant strain suggests that resistance mechanisms might afford some level of protection, albeit not enough to counteract the effects of menthol completely. One hypothesis is that the structural or functional attributes conferred by antibiotic resistance mechanisms in E. coli BN407 might mitigate some of the disruptive effects of menthol on cell membranes. This could account for the slightly less pronounced decrease in CFU and growth rate compared to the non-resistant strain.
Menthol's ability to perturb membrane functions and proton gradients aligns with the known mechanisms of action of various essential oils and their components, which often involve disrupting membrane integrity and function. This disruption can lead to increased permeability, leakage of cellular contents, and eventual cell death, which corroborates our observations of decreased CFU and altered growth kinetics.
Menthol exhibits potent antibacterial activity against both tetracycline-resistant and non-resistant E. coli strains, as evidenced by its consistent MIC values and significant impacts on CFU formation, growth kinetics, and membrane-associated properties. The nuanced differences in how it affects resistant and non-resistant strains underline the complexity of its action and potential for use against bacterial pathogens.
Our findings underscore the importance of utilizing multiple assays to fully understand the antibacterial potential and mechanisms of action of compounds like menthol. While disk-diffusion and MIC provide initial insights, assays such as CFU, growth kinetics, and membrane-associated properties offer deeper understanding and reveal the compound's broader impacts on bacterial physiology. Future studies could explore the exact molecular interactions between menthol and bacterial membranes, and its potential synergistic effects with other antibiotics, to enhance its efficacy and broaden its application in antimicrobial treatments.
The authors declare they have not used Artificial Intelligence (AI) tools in the creation of this article.
[1] |
Seukep AJ, Mbuntcha HG, Zeuko'o EM, et al. (2023) Established antibacterial drugs from plants. Adv Bot Res 106: 81-149. https://doi.org/10.1016/bs.abr.2022.08.005 ![]() |
[2] |
Vaou N, Stavropoulou E, Voidarou C, et al. (2021) Advances in medicinal plant antimicrobial activity: a review study on challenges and future perspectives. Microorganisms 9: 2041. https://doi.org/10.3390/microorganisms9102041 ![]() |
[3] |
Ginovyan M, Babayan A, Shirvanyan A, et al. (2023) The action mechanisms, anti-cancer and antibiotic-modulation potential of Vaccinium myrtillus L. extract. Discov Med 35: 590-611. https://doi.org/10.24976/Discov.Med.202335177.59 ![]() |
[4] |
Vaou N, Stavropoulou E, Voidarou CC, et al. (2022) Interactions between medical plant-derived bioactive compounds: focus on antimicrobial combination effects. Antibiotics (Basel) 11: 1014. https://doi.org/10.3390/antibiotics11081014 ![]() |
[5] |
Imlay JA (2019) Where in the world do bacteria experience oxidative stress?. Environ Microbiol 21: 521-530. https://doi.org/10.1111/1462-2920.14445 ![]() |
[6] |
Seukep AJ, Kuete V, Nahar L, et al. (2020) Plant-derived secondary metabolites as the main source of efflux pump inhibitors and methods for identification. J Pharm Anal 10: 277-290. https://doi.org/10.1016/j.jpha.2019.11.002 ![]() |
[7] | Sahakyan N, Petrosyan M, Trchounian A (2019) Some Lamiaceae family plant essential oil chemical composition and their potential as antimicrobial agents against antibiotic-resistant bacteria. Proceedings of the YSU B: Chemical and Biological Sciences 53: 23-28. https://doi.org/10.46991/PYSU:B/2019.53.1.023 |
[8] |
Sahakyan N (2023) Lamiaceae family plants: one of the potentially richest sources of antimicrobials. Pharm Chem J 57: 507-5014. https://doi.org/10.1007/s11094-023-02921-1 ![]() |
[9] | Salehi B, Stojanović-Radić Z, Matejić J, et al. (2018) Plants of genus Mentha: from farm to food factory. Plants (Basel) 7: 70. https://doi.org/10.3390/plants7030070 |
[10] |
Landau E, Shapira R (2012) Effects of subinhibitory concentrations of menthol on adaptation, morphological, and gene expression changes in enterohemorrhagic Escherichia coli. Appl Environ Microbiol 78: 5361-5367. https://doi.org/10.1128/AEM.00894-12 ![]() |
[11] |
Nazzaro F, Fratianni F, De Martino L, et al. (2013) Effect of essential oils on pathogenic bacteria. Pharmaceuticals (Basel) 6: 1451-1474. https://doi.org/10.3390/ph6121451 ![]() |
[12] |
Fernández-Billón M, Llambías-Cabot AE, Jordana-Lluch E, et al. (2023) Mechanisms of antibiotic resistance in Pseudomonas aeruginosa biofilms. Biofilm 5: 100129. https://doi.org/10.1016/j.bioflm.2023.100129 ![]() |
[13] |
Husain FM, Ahmad I, Khan MS, et al. (2015) Sub-MICs of Mentha piperita essential oil and menthol inhibits AHL mediated quorum sensing and biofilm of gram-negative bacteria. Front Microbiol 6: 420. https://doi.org/10.3389/fmicb.2015.00420 ![]() |
[14] |
Ferreira de Melo AN, Targino de Souza Pedrosa G, Tayse da Cruz Almeida E, et al. (2020) Successive exposure to Mentha piperita L. essential oil affects the culturability and induces membrane repair in a persister epidemic Salmonella Typhimurium PT4. Microb Pathog 149: 104264. https://doi.org/10.1016/j.micpath.2020.104264 ![]() |
[15] |
Trombetta D, Castelli F, Sarpietro MG, et al. (2005) Mechanisms of antibacterial action of three monoterpenes. Antimicrob Agents Ch 49: 2474-2478. https://doi.org/10.1128/aac.49.6.2474-2478.2005 ![]() |
[16] |
Tadevosyan S, Shirvanyan A, Markosyan A, et al. (2023) Chemical composition and antibacterial activity of essential oil of Mentha arvensis L. harvested at high altitude Armenian flora. Proceedings of the YSU B: Chemical and Biological Sciences 57: 230-237. https://doi.org/10.46991/PYSU:B/2023.57.3.230 ![]() |
[17] |
Lorenzo V, Wee S, Herrero M, et al. (1987) Operator sequences of the aerobactin operon of plasmid ColV-K30 binding the ferric uptake regulation (fur) repressor. J Bacteriol 169: 2624-2630. https://doi.org/10.1128/jb.169.6.2624-2630.1987 ![]() |
[18] |
Maringanti S, Imlay J (1999) An intracellular iron chelator pleiotropically suppresses enzymatic and growth defects of superoxide dismutase-deficient Escherichia coli. J Bacteriol 181: 3792-3802. https://doi.org/10.1128/jb.181.12.3792-3802.1999 ![]() |
[19] |
Hovhannisyan Z, Timotina M, Manoyan J, et al. (2022) Ribes nigrum L. extract-mediated green synthesis and antibacterial action mechanisms of silver nanoparticles. Antibiotics 11: 1415. https://doi.org/10.3390/antibiotics11101415 ![]() |
[20] |
Sieuwerts S, De Bok FAM, Mols E, et al. (2008) A simple and fast method for determining colony forming units. Lett Appl Microbiol 47: 275-278. https://doi.org/10.1111/j.1472-765X.2008.02417.x ![]() |
[21] |
Vanyan L, Trchounian K (2022) HyfF subunit of hydrogenase 4 is crucial for regulating FOF1 dependent proton/potassium fluxes during fermentation of various concentrations of glucose. Jf Bioenerg Biomembr 54: 69-79. https://doi.org/10.1007/s10863-022-09930-x ![]() |
[22] |
Chang Y, Sun W, Murchie AIH, et al. (2023) Genome-wide identification of kanamycin B binding RNA in Escherichia coli. BMC Genomics 24: 120. https://doi.org/10.1186/s12864-023-09234-3 ![]() |
[23] |
Mohaddab M, El Goumi Y, Gallo M, et al. (2022) Biotechnology and in vitro culture as an alternative system for secondary metabolite production. Molecules 27: 8093. https://doi.org/10.3390/molecules27228093 ![]() |
[24] |
Chaachouay N, Zidane L (2024) Plant-derived natural products: a source for drug discovery and development. Drug Drug Candidates 3: 184-207. https://doi.org/10.3390/ddc3010011 ![]() |
[25] |
Uritu CM, Mihai CT, Stanciu GD, et al. (2018) Medicinal plants of the family Lamiaceae in pain therapy: a review. Pain Res Manag 2018: 7801543. https://doi.org/10.1155/2018/7801543 ![]() |
[26] |
Piran P, Kafil HS, Ghanbarzadeh S, et al. (2017) Formulation of menthol-loaded nanostructured lipid carriers to enhance its antimicrobial activity for food preservation. Adv Pharm Bull 7: 261-268. https://doi.org/10.15171/apb.2017.031 ![]() |
[27] |
Rolfe MD, Rice CJ, Lucchini S, et al. (2012) Lag phase is a distinct growth phase that prepares bacteria for exponential growth and involves transient metal accumulation. J Bacteriol 194: 686-701. https://doi.org/10.1128/JB.06112-11 ![]() |
[28] |
Benarroch JM, Asally M (2020) The microbiologist's guide to membrane potential dynamics. Trends Microbiol 28: 304-314. https://doi.org/10.1016/j.tim.2019.12.008 ![]() |
[29] |
Lane N (2017) Proton gradients at the origin of life. Bioessays 39: 1600217. https://doi.org/10.1002/bies.201600217 ![]() |
[30] |
Sapra R, Bagramyan K, Adams MW (2003) A simple energy-conserving system: proton reduction coupled to proton translocation. Proc Natl Acad Sci USA 100: 7545-7550. https://doi.org/10.1073/pnas.1331436100 ![]() |
[31] |
Toei M, Noji H (2013) Single-molecule analysis of F0F1-ATP synthase inhibited by N,N-dicyclohexylcarbodiimide. J Biol Chem 288: 25717-25726. https://doi.org/10.1074/jbc.M113.482455 ![]() |
[32] |
Rieger B, Arroum T, Borowski MT, et al. (2021) Mitochondrial F1 FO ATP synthase determines the local proton motive force at cristae rims. EMBO Rep 22: e52727. https://doi.org/10.15252/embr.202152727 ![]() |