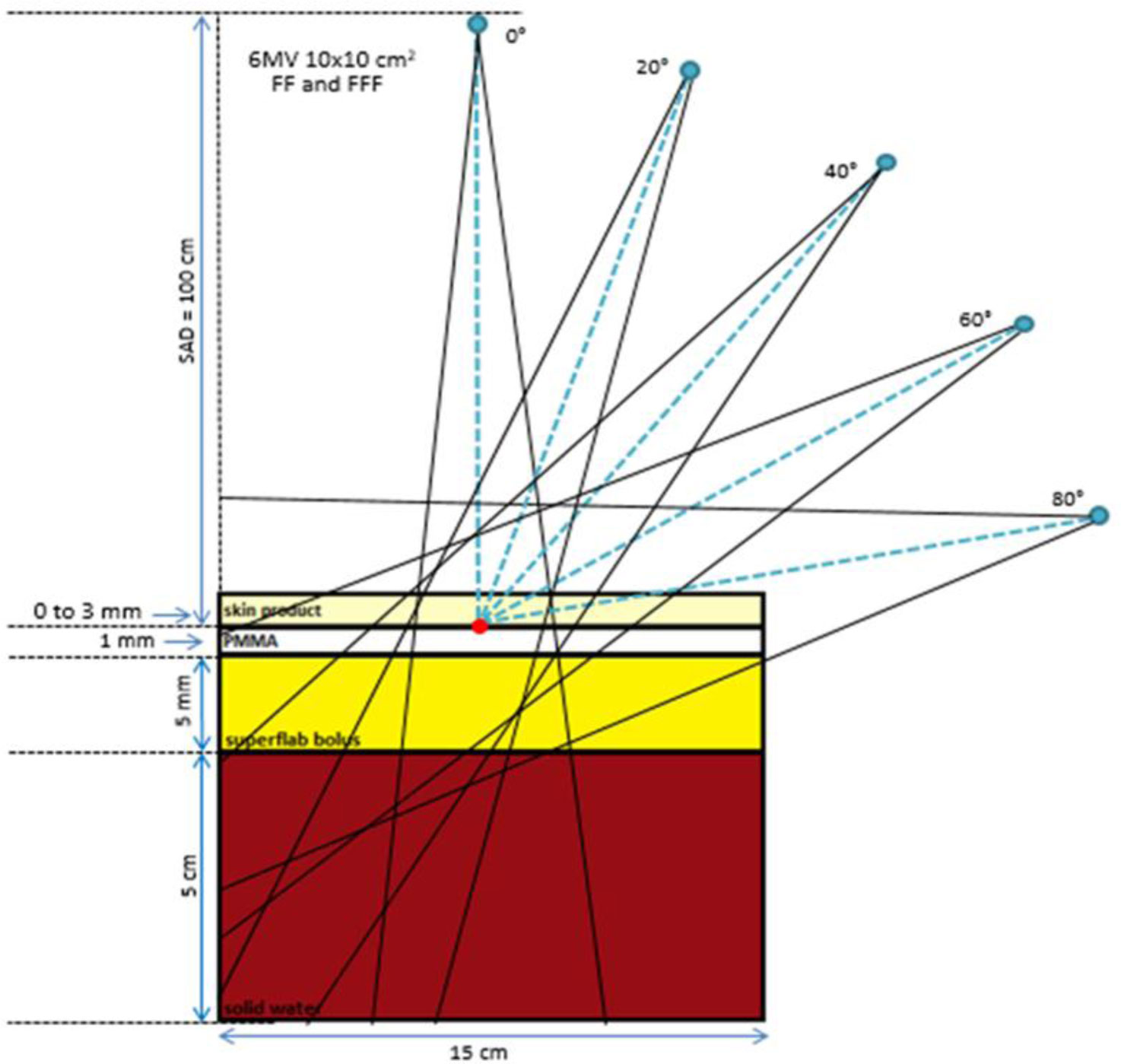
Skin-care cream is commonly applied to relieve skin redness in radiotherapy. However, using cream on the patient under the photon field would increase the skin dose in delivery. The aim of this study is to evaluate the dependences of skin dose enhancement on different beam and cream variables using Monte Carlo simulation. Using a solid water phantom with water-equivalent bolus, PMMA layer and cream layer, we irradiated it by 6 MV photon beams. Skin doses were calculated by varying the beam quality (flattening-filter (FF) and flattening-filter-free (FFF)), beam angle (0°–80°), skin-care cream type (water-based and silicon-based) and cream thickness (0–3 mm) using the EGSnrc Monte Carlo code. The densities of the water- and silicon-based cream were 0.92 and 1.14 g/cm3. The dose enhancement factor (DEF) defined as the ratio of the skin dose with skin-care cream to the skin dose without cream was calculated. It is found that the FF photon beam had higher DEF value than the FFF. For the water-based cream of 3 mm, the DEF for the FF beam was about 22.1% higher than that of the FFF. While for the silicon-based cream with the same thickness, the DEF was 24.2% higher. DEF value also increased with the beam angle. The DEF values were from 1.4 to 2.52 (water-based cream) and 1.42 to 2.68 (silicon-based cream) when the beam angle was increased from 20° to 80° using the 6 MV FF beams. Similarly, for the 6 MV FFF beams, the DEF values increased from 1.29 to 2.07 and 1.30 to 2.18 for the water- and silicon-based cream. These simulation results showed that the skin dose enhancement increased with an increase of beam angle, cream thickness, cream density, and the irradiation of FFF photon beams.
Citation: Megha Sharma, James C. L. Chow. Skin dose enhancement from the application of skin-care creams using FF and FFF photon beams in radiotherapy: A Monte Carlo phantom evaluation[J]. AIMS Bioengineering, 2020, 7(2): 82-90. doi: 10.3934/bioeng.2020008
[1] | Chun He, James C.L. Chow . Gold nanoparticle DNA damage in radiotherapy: A Monte Carlo study. AIMS Bioengineering, 2016, 3(3): 352-361. doi: 10.3934/bioeng.2016.3.352 |
[2] | Dewmini Mututantri-Bastiyange, James C. L. Chow . Imaging dose of cone-beam computed tomography in nanoparticle-enhanced image-guided radiotherapy: A Monte Carlo phantom study. AIMS Bioengineering, 2020, 7(1): 1-11. doi: 10.3934/bioeng.2020001 |
[3] | Moncef ATI, Rachid BOUAMRANE, Djamel ADDI, Fatima Zohra MAROC, Fatima Zohra Mecheret . Monte Carlo dose index estimation in computed tomography. AIMS Bioengineering, 2020, 7(4): 224-235. doi: 10.3934/bioeng.2020019 |
[4] | Mi-Young Yun, Kyoung-Sook Park, Kyung-Ah Yoon, Hwa-Jung Choi . Leaf extracts of Nandina domestica Thunb ameliorate atopic dermatitis in NC/Nga mice treated with 1-chloro-2,4-dinitrobenzene (DNCB). AIMS Bioengineering, 2021, 8(2): 154-164. doi: 10.3934/bioeng.2021014 |
[5] | Gabriella Fabbrocini, Sara Cacciapuoti, Gaia De Fata Salvatores, Claudia Costa, Paolo Chiodini, Maria Antonietta Luciano, Serena Poggi, Silvestro Scotti, Luigi Sparano, Giuseppe Di Leo, Paolo Sommella, Matteo Ferro, Massimiliano Scalvenzi . A new “hub and spoke” teledermoscopy system involving general practitioners and dermatologists for the early detection of cutaneous melanoma: a pilot study. AIMS Bioengineering, 2020, 7(4): 306-320. doi: 10.3934/bioeng.2020025 |
[6] | Marianthi Logotheti, Eleftherios Pilalis, Nikolaos Venizelos, Fragiskos Kolisis, Aristotelis Chatziioannou . Development and validation of a skin fibroblast biomarker profile for schizophrenic patients. AIMS Bioengineering, 2016, 3(4): 552-565. doi: 10.3934/bioeng.2016.4.552 |
[7] | Cong Toai Truong, Kim Hieu Huynh, Van Tu Duong, Huy Hung Nguyen, Le An Pham, Tan Tien Nguyen . Model-free volume and pressure cycled control of automatic bag valve mask ventilator. AIMS Bioengineering, 2021, 8(3): 192-207. doi: 10.3934/bioeng.2021017 |
[8] | Nikolaos Giormezis, Konstantinos Papakonstantinou, Fevronia Kolonitsiou, Eleanna Drougka, Antigoni Foka, Styliani Sarrou, Evangelos D. Anastassiou, Efthimia Petinaki, Iris Spiliopoulou . Biofilm synthesis and its relationship with genetic characteristics in clinical methicillin-resistant staphylococci. AIMS Bioengineering, 2015, 2(4): 375-386. doi: 10.3934/bioeng.2015.4.375 |
[9] | Meiling Sun, Changlei Cui . Transforming lung cancer care: Synergizing artificial intelligence and clinical expertise for precision diagnosis and treatment. AIMS Bioengineering, 2023, 10(3): 331-361. doi: 10.3934/bioeng.2023020 |
[10] | Shital Hajare, Rajendra Rewatkar, K.T.V. Reddy . Design of an iterative method for enhanced early prediction of acute coronary syndrome using XAI analysis. AIMS Bioengineering, 2024, 11(3): 301-322. doi: 10.3934/bioeng.2024016 |
Skin-care cream is commonly applied to relieve skin redness in radiotherapy. However, using cream on the patient under the photon field would increase the skin dose in delivery. The aim of this study is to evaluate the dependences of skin dose enhancement on different beam and cream variables using Monte Carlo simulation. Using a solid water phantom with water-equivalent bolus, PMMA layer and cream layer, we irradiated it by 6 MV photon beams. Skin doses were calculated by varying the beam quality (flattening-filter (FF) and flattening-filter-free (FFF)), beam angle (0°–80°), skin-care cream type (water-based and silicon-based) and cream thickness (0–3 mm) using the EGSnrc Monte Carlo code. The densities of the water- and silicon-based cream were 0.92 and 1.14 g/cm3. The dose enhancement factor (DEF) defined as the ratio of the skin dose with skin-care cream to the skin dose without cream was calculated. It is found that the FF photon beam had higher DEF value than the FFF. For the water-based cream of 3 mm, the DEF for the FF beam was about 22.1% higher than that of the FFF. While for the silicon-based cream with the same thickness, the DEF was 24.2% higher. DEF value also increased with the beam angle. The DEF values were from 1.4 to 2.52 (water-based cream) and 1.42 to 2.68 (silicon-based cream) when the beam angle was increased from 20° to 80° using the 6 MV FF beams. Similarly, for the 6 MV FFF beams, the DEF values increased from 1.29 to 2.07 and 1.30 to 2.18 for the water- and silicon-based cream. These simulation results showed that the skin dose enhancement increased with an increase of beam angle, cream thickness, cream density, and the irradiation of FFF photon beams.
External radiotherapy is the most popular option in cancer therapy [1] among chemotherapy and surgery. However, in radiotherapy, when the photon beam is directed to the tumour inside the patient body, the beam would inevitably irradiate through the patient skin on the way to the tumour. Therefore, patient going through radiotherapy may experience some level of side effects on the skin. These side effects often involve the epidermis. Skin reactions and side effects in radiotherapy vary among patients and tend to vary according to any pre-existing factors [2]. Irradiation of the skin can cause multitude of complications such as discomfort, moist desquamation, dry desquamation, irritation, and pain. To date, different skin care recommendations and practices amid radiotherapy are made by different cancer centres and hospitals in the world [3]–[5].
For common skin side effect such as redness caused by radiotherapy, skin-care (water- or silicone-based) creams are suggested applying onto the patient's skin to treat the symptom [6],[7]. However, for external radiotherapy using megavoltage (MV) photon beams, increase of skin dose happens when there is a material on top of the skin under the photon field. This material can be from an immobilization device such as a thermoplastic mask [8], a piece of bolus to reduce or alter the dose in delivery [9], or a layer of skin-care cream for skin redness relief [10]. This increase of skin dose on the patient surface is due to the depth dose characteristic of the MV photon beam. When a layer of material is placed on the patient skin under irradiation, the location of the maximum depth dose for the MV beam will shift towards the patient surface. This results in an increase of dose at the patient skin under the photon field. It is found that such skin dose enhancement can be affected by the material thickness, material composition (density), beam angle and beam quality [11]–[14]. In this study, we will focus on the skin dose enhancement from the application of skin-care creams in radiotherapy.
In 3D-conformal radiotherapy which used to be popular, a uniform beam profile was a must to provide homogeneous dose distribution at the tumour. This can be achieved using a flattening filter (FF) equipped inside the head of a medical linear accelerator. However, with the advance of intensity modulated radiotherapy, dose conformity at the target can be obtained better by the intensity modulated beams generated by the multileaf collimator [15]–[17]. Because of that, the FF is no longer needed and can be removed from the linear accelerator. One advantage of removing the FF is a huge increase of the beam output from hundreds to thousands of monitor unit per minute. Such increase of beam output can shorten the treatment time to increase the patient throughput in the cancer centre, and decrease the dose uncertainty due to the internal organ motion such as breathing during the delivery [18]. However, removing the FF also changes the quality (photon energy distribution) of the MV photon beam. Since FF absorbs most of the low-energy photons in the MV beam, removing the filter will lead to a large number of low-energy photons in the beam. It is found that such increase of low-energy photons in the flattening-filter-free (FFF) beam would weaken the beam hardening effect and increase the patient skin dose in the delivery [13],[14],[19]. This increase of skin dose to the patient would likely introduce additional side effect in radiotherapy. Therefore, it is worthwhile to study the dependence of skin dose enhancement from the skin-care cream on the photon beam quality. Moreover, since the external contour of the patient is rounded, the patient surface is usually not perfectly perpendicular to the central beam axis. Since it is found that the skin dose of the patient can be varied with the beam obliquity [13],[20], it is worthwhile to determine the relationship between the skin dose enhancement and beam obliquity.
Monte Carlo simulation is a computational method relying on repeated random sampling of a probability density function to obtain numerical result from a problem. Monte Carlo simulation is often used to solve physical and mathematical problems when it is very difficult to find out the absolute solution [21]. In radiotherapy, Monte Carlo simulation is the benchmark to predict radiation dose in a heterogeneous medium [22]. In this study, the skin dose enhancement was predicted by Monte Carlo simulation (EGSnrc-based codes) using a phantom [23].
This study was to conceptualize the impact of skin-care creams on cancer patients who undergo radiotherapy using the new FFF photon beams. The study focused on manipulating several skin-care cream and photon beam variables, namely, cream thickness, cream type, FFF and FF, and beam angles. The aim of this study is to determine the dependences of skin dose enhancement on the above variables using Monte Carlo simulation.
To determine the skin dose enhancement due to skin-care creams using the FFF and FF photon beams, surface doses were calculated and compared using a phantom with a single beam geometry. To compare the skin dose with and without using the skin-care cream, a phantom for Monte Carlo simulation was used as shown in Figure 1. The phantom contained a solid water slab with thickness equal to 5 cm. A 5 mm layer of water-equivalent bolus was placed on top of the solid water slab to mimic the skin. The polymethyl methacrylate (PMMA) layer on the bolus was the skin dose calculation point and a layer of skin-care cream was added on the PMMA. The cream thickness was in the range of 0–3 mm. Two kinds of skin-care creams were used, namely, water-based and silicon-based cream. The water-based cream contains H, O, C and P, and the density is equal to 0.92 g/cm3. The silicon-based cream contains C, H, N, O, Si, Ca and Na and the density is equal to 1.14 g/cm3. These kinds of creams are frequently used for patient care in radiotherapy [6],[7]. The creams were simulated based on their compositions using the EGSnrc-based PEGS4 program [23].
For the MV photon beams, a 6 MV flattening-filter (FF) and FFF beam based on a Varian TrueBEAM linear accelerator were used in the simulation. The field size of beams was equal to 10 × 10 cm2. The source-to-axis distance was equal to 100 cm and the isocenter was located at the interface between the PMMA and skin-care cream layer (red spot in Figure 1). Monte Carlo simulations using the EGSnrc-based DOSXYZnrc code [24] were carried out to predict skin doses at the PMMA layer with beam angles set to 0°, 20°, 40°, 60° and 80°. Phase-space files containing particle information of type, coordinate, orientation and energy for the 6 MV FF and 6 MV FFF photon beams, generated by the Varian Monte Carlo study team, were used in this study [25,26]. Each phase-space file contained 1 × 109 particles and verifications of the Monte Carlo model can be found elsewhere [13],[14]. Monte Carlo verification by comparing the simulated and measured results was shown elsewhere [5],[6].
Based on the beam and phantom geometry in Figure 1, the skin doses were determined with variations of the photon beam quality (FF and FFF), beam angle (0°–80°), cream thickness (0–3 mm) and cream type (water-based and silicon-based).
The dose enhancement factor (DEF) was calculated using the formula,
The dependences of DEF on the water- and silicon-based cream thickness are shown in Figure 2a,b, respectively. The beam angle was equal to zero in Figure 2 and both 6 MV FF and FFF photon beams were used. At this beam angle, the beam central axis (i.e., broken lines in Figure 1) was perpendicular to the phantom surface. For other beam angles, the dependences of DEF on the water- and silicon-based cream thickness for the 6 MV FF photon beams are shown in Figure 3a,b, while similar dependences of DEF on the cream thickness for the 6 MV FFF beams are shown in Figure 3c,d.
For the dependence of DEF on the cream thickness with the beam angle equal to zero using the water-based cream (Figure 2a), it is found that the DEF value increased with an increase of the cream thickness. This result can also be found in Figure 2b using the silicon-based cream. This is because the cream thickness on the phantom surface is related to the shift distance of the maximum depth dose of the photon beam. The closer the maximum depth dose to the phantom surface, the higher the surface or skin dose. Therefore, it is seen that increasing the cream thickness on the patient skin would also increase the skin dose. On the other hand, the DEF values for the 6 MV FF beams were higher than those of the FFF beams with the same thicknesses of water- (Figure 2a) and silicon-based cream (Figure 2b). This is because the Dair value in Eq 1 for the 6 MV FFF beam was higher than that of the 6 MV FF beam. This higher Dair value is due to the fact that the FFF beam contained more low-energy photons, which would attenuate and deposit energy at the surface [13],[14]. For the water-based cream, the maximum DEF value of the 6 MV FF beam was about 22.1% higher than the FFF beam. While for the silicon-based cream, the maximum DEF value of the FF beam was about 24.2% higher than the FFF beam. This shows that the increase of skin dose enhancement was more significant in the FF beams rather than the FFF.
Comparing the DEF values between the water- and silicon-based cream using the same beam angle and quality as shown in Figure 2a,b, it is seen that the DEF value for the silicon-based cream was higher than that of the water-based. For the maximum DEF at cream thickness equal to 3 mm, the silicon-based cream was 5.2% higher than the water-based for the FFF beams and 7.0% for the FF. The higher skin dose enhancement for the silicon-based cream is due to its higher density of 1.14 g/cm3 compared to 0.92 g/cm3 for the water-based cream. The skin dose enhancement also increased more for the FFF photon beam compared to the FF. As the texture of silicon-based cream looked “thicker” or more protective when applied on the patient skin, it would actually increase skin dose more than the water-based type.
When the beam angle was increased from 0° to 80°, the phantom surface dose increased. For the dependences of DEF on the water- and silicon-based cream thickness varying with the beam angle as shown in Figure 3a,b, the DEF value was found increased with an increase of beam angle at the same cream thickness using the 6 MV FF beams. The maximum DEF values (cream thickness = 3 mm) for beam angles equal to 20°, 40°, 60° and 80° were 1.40, 1.89, 2.28 and 2.52 for the water-based cream using the 6 MV FF beams. While for the silicon-based cream using the same photon beam and cream thickness, the maximum DEF values for beam angles of 20°, 40°, 60° and 80° were 1.42, 1.95, 2.41 and 2.68, respectively. It can be seen that the DEF values for the silicon-based cream were slightly higher than those of the water-based. This result agreed well with that from the beam angle equal to zero (Figure 2). When the 6 MV FFF photon beams were used as shown in Figure 3c,d, the maximum DEF values for the beam angles of 20°, 40°, 60° and 80° were 1.29, 1.66, 1.92 and 2.07 for the water-based cream, and 1.30, 1.71, 2.01 and 2.18 for the silicon-based cream. Similar to the results from the FF photon beams in Figure 3a,b, the silicon-based cream had higher DEF values than those for the water-based. Comparing Figure 3a,b to Figure 3c,d, it is seen that the FF photon beams had higher DEF values for oblique beams than the FFF. Therefore, the FFF photon beams had a lower skin dose enhancement compared to the FF beams. Moreover, the skin dose enhancement increased with an increase of beam angle.
The dependences of DEF on various skin-care cream and beam variables were evaluated using Monte Carlo simulation. It is found that the DEF value increased with an increase of the cream thickness. Moreover, the higher density silicon-based cream had a higher DEF value compared to the water-based cream. For the beam quality, the FF photon beam had a higher DEF value than the FFF beam containing more low-energy photons. The DEF value also increased with an increase of beam angle. It is concluded that when skin-care cream is applied to treat skin redness, lower skin dose enhancement can be achieved by using thinner layer of cream, the FFF photon beam and a small beam angle. These dosimetric results predicted by Monte Carlo simulation provide important information for the radiation staff, when setting up patient care policy to avoid skin side effect in radiotherapy. Future work includes using clinical CT image set to study the true skin dose (entrance and exit dose) for patients in breast radiotherapy.
[1] |
Delaney G, Jacob S, Featherstone C, et al. (2005) The role of radiotherapy in cancer treatment: estimating optimal utilization from a review of evidence-based clinical guidelines. Cancer 104: 1129-1137. doi: 10.1002/cncr.21324
![]() |
[2] |
Naylor W, Mallett J (2001) Management of acute radiotherapy induced skin reactions: a literature review. Eur J Oncol Nurs 5: 221-233. doi: 10.1054/ejon.2001.0145
![]() |
[3] |
Baumann BC, Verginadis II, Zeng C, et al. (2018) Assessing the validity of clinician advice that patients avoid use of topical agents before daily radiotherapy treatments. JAMA Oncol 4: 1742-1748. doi: 10.1001/jamaoncol.2018.4292
![]() |
[4] |
Harris R, Probst H, Beardmore C, et al. (2012) Radiotherapy skin care: A survey of practice in the UK. Radiography 18: 21-27. doi: 10.1016/j.radi.2011.10.040
![]() |
[5] |
Morley L, Cashell A, Sperduti A, et al. (2014) Evaluating the relevance of dosimetric considerations to patient teaching regarding skin care during radiation therapy. J Radiother Pract 13: 294-301. doi: 10.1017/S1460396913000241
![]() |
[6] |
Tse K, Morley L, Cashell A, et al. (2016) Dosimetric impacts on skin toxicity for patients using topical agents and dressings radiotherapy. J Radiother in Pract 15: 314-321. doi: 10.1017/S1460396916000285
![]() |
[7] |
Hadley SW, Kelly R, Lam K (2005) Effects of immobilization mask material on surface dose. J Appl Clin Med Phys 6: 1-7. doi: 10.1120/jacmp.v6i1.1957
![]() |
[8] |
Khan Y, Villarreal-Barajas JE, Udowicz M, et al. (2013) Clinical and dosimetric implications of air gaps between bolus and skin surface during radiation therapy. J Cancer Ther 4: 1251-1255. doi: 10.4236/jct.2013.47147
![]() |
[9] |
Løkkevik E, Skovlund E, Reitan JB, et al. (1996) Skin treatment with bepanthen cream versus no cream during radiotherapy: a randomized controlled trial. Acta Oncol 35: 1021-1026. doi: 10.3109/02841869609100721
![]() |
[10] |
Chow JCL, Grigorov GN (2008) Surface dosimetry for oblique tangential photon beams: A Monte Carlo simulation study. Med Phys 35: 70-76. doi: 10.1118/1.2818956
![]() |
[11] |
Chow JCL, Grigorov GN, Barnett RB (2006) Study on surface dose generated in prostate intensity-modulated radiation therapy treatment. Med Dosim 31: 249-258. doi: 10.1016/j.meddos.2005.07.002
![]() |
[12] |
Chow JCL, Owrangi AM (2016) A surface energy spectral study on the bone heterogeneity and beam obliquity using the flattened and unflattened photon beams. Rep Pract Oncol Radiother 21: 63-70. doi: 10.1016/j.rpor.2015.11.001
![]() |
[13] |
Chow JCL, Owrangi AM (2014) Dosimetric dependences of bone heterogeneity and beam angle on the unflattened and flattened photon beams: A Monte Carlo comparison. Radiat Phys Chem 101: 46-52. doi: 10.1016/j.radphyschem.2014.03.041
![]() |
[14] |
Bortfeld T (2006) IMRT: a review and preview. Phys Med Biol 51: R363. doi: 10.1088/0031-9155/51/13/R21
![]() |
[15] | Jia J, Hui L, Chow JCL (2015) A leaf sequencing algorithm for multileaf collimator in intensity modulated radiotherapy. Rep Radiother Oncol 2: e4922. |
[16] |
Chow JCL, Grigorov GN, Yazdani N (2006) SWIMRT: A graphical user interface using sliding window algorithm to construct a fluence map machine file. J Appl Clin Med Phys 7: 69-85. doi: 10.1120/jacmp.v7i2.2231
![]() |
[17] |
Sharma SD (2011) Unflattened photon beams from the standard flattening filter free accelerators for radiotherapy: advantages, limitations and challenges. J Med Phys 36: 123-125. doi: 10.4103/0971-6203.83464
![]() |
[18] |
Chow JCL, Owrangi AM (2019) Mucosal dosimetry on unflattened photon beams: A Monte Carlo phantom study. Biomed Phys Eng Express 5: 015007. doi: 10.1088/2057-1976/aaeaaa
![]() |
[19] |
Chow JCL, Jiang R, Leung MKK (2011) Dosimetry of oblique tangential photon beams calculated by superposition/convolution algorithms: a Monte Carlo evaluation. J Appl Clin Med Phys 12: 108-121. doi: 10.1120/jacmp.v12i1.3424
![]() |
[20] |
Chow JCL (2018) Recent progress in Monte Carlo simulation of gold nanoparticle radiosensitization. AIMS Biophys 5: 231-244. doi: 10.3934/biophy.2018.4.231
![]() |
[21] |
Rogers DWO (2006) Fifty years of Monte Carlo simulations for medical physics. Phys Med Biol 51: R287. doi: 10.1088/0031-9155/51/13/R17
![]() |
[22] | Kawrakow I, Mainegra-Hing E, Rogers DWO, et al. (2000) The EGSnrc code system: Monte Carlo simulation of electron and photon transport. NRCC Report PIRS-701 Ottawa: NRC, Available from: https://nrc-cnrc.github.io/EGSnrc/doc/pirs701-egsnrc.pdf. |
[23] | Walters B, Kawrakow I, Rogers DWO (2005) DOSXYZnrc users manual. NRCC Report PIRS-794revB Ottawa: NRC, Available from: https://nrc-cnrc.github.io/EGSnrc/doc/pirs794-dosxyznrc.pdf. |
[24] |
Constantin M, Perl J, LoSasso T, et al. (2011) Modeling the TrueBeam linac using a CAD to Geant4 geometry implementation: dose and IAEA-compliant phase space calculations. Med Phys 38: 4018-4024. doi: 10.1118/1.3598439
![]() |
[25] |
Sardari D, Maleki R, Samavat H, et al. (2010) Measurement of depth-dose of linear accelerator and simulation by use of GEANT4 computer code. Rep Pract Oncol Radiother 15: 64-68. doi: 10.1016/j.rpor.2010.03.001
![]() |
1. | James C. L. Chow, Depth Dose Enhancement on Flattening-Filter-Free Photon Beam: A Monte Carlo Study in Nanoparticle-Enhanced Radiotherapy, 2020, 10, 2076-3417, 7052, 10.3390/app10207052 | |
2. | James Chun Lam Chow, 2021, 9780128231524, 193, 10.1016/B978-0-12-823152-4.00001-6 | |
3. | Armando Spina, James C. L. Chow, Dosimetric Impact on the Flattening Filter and Addition of Gold Nanoparticles in Radiotherapy: A Monte Carlo Study on Depth Dose Using the 6 and 10 MV FFF Photon Beams, 2022, 15, 1996-1944, 7194, 10.3390/ma15207194 | |
4. | Afia Sadiq, James C. L. Chow, Evaluation of Dosimetric Effect of Bone Scatter on Nanoparticle-Enhanced Orthovoltage Radiotherapy: A Monte Carlo Phantom Study, 2022, 12, 2079-4991, 2991, 10.3390/nano12172991 | |
5. | Hikari Sato, Ryo Saga, Fumio Komai, Yumiko Sato, Hideki Obara, Masahiko Aoki, Yoichiro Hosokawa, Fundamental evaluation of dose and dose distribution under applied topical agents and dressings used for skin care in radiotherapy, 2023, 16, 16878507, 100616, 10.1016/j.jrras.2023.100616 | |
6. | James C. L. Chow, 2024, Chapter 7, 978-981-97-0220-6, 177, 10.1007/978-981-97-0221-3_7 |