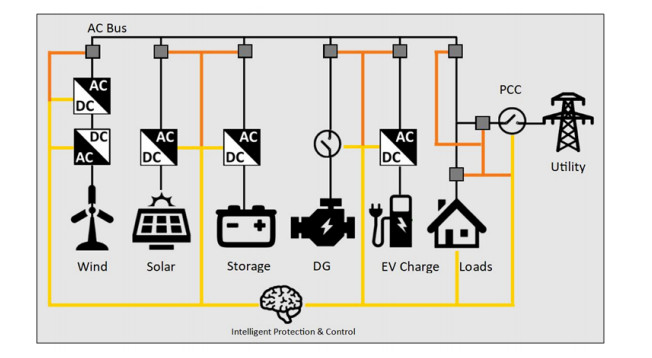
Citation: T S S Senarathna, K T M Udayanga Hemapala. Review of adaptive protection methods for microgrids[J]. AIMS Energy, 2019, 7(5): 557-578. doi: 10.3934/energy.2019.5.557
[1] | Pooja Khandare, Sanjay Deokar, Arati Dixit . Relay Coordination and Optimization techniques using DWT-Differentiation Algorithms for Fault Detection in Microgrid. AIMS Energy, 2020, 8(4): 563-579. doi: 10.3934/energy.2020.4.563 |
[2] | W. E. P. Sampath Ediriweera, N. W. A. Lidula . Design and protection of microgrid clusters: A comprehensive review. AIMS Energy, 2022, 10(3): 375-411. doi: 10.3934/energy.2022020 |
[3] | Tung-Sheng Zhan, Chun-Lien Su, Yih-Der Lee, Jheng-Lun Jiang, Jin-Ting Yu . Adaptive OCR coordination in distribution system with distributed energy resources contribution. AIMS Energy, 2023, 11(6): 1278-1305. doi: 10.3934/energy.2023058 |
[4] | Mohamed G Moh Almihat . An overview of AC and DC microgrid energy management systems. AIMS Energy, 2023, 11(6): 1031-1069. doi: 10.3934/energy.2023049 |
[5] | Tung-Sheng Zhan, Yih-Der Lee, Jheng-Lun Jiang . Optimal OCR coordination in a high penetration distribution power system using a refined immune algorithm with an auto-tuning reproductive mechanism. AIMS Energy, 2024, 12(6): 1225-1263. doi: 10.3934/energy.2024056 |
[6] | Mohammad Aman YAQOBI, Hidehito Matayoshi, Natarajan Prabaharan, Hiroshi Takahashi, Ashraf M. Hemeida, Senjyu Tomonobu . Interconnected standalone DC microgrid fault protection based on Self-Adaptive DC fault current limiter with hybrid solid state circuit breaker. AIMS Energy, 2021, 9(5): 991-1008. doi: 10.3934/energy.2021045 |
[7] | Mohamed G Moh Almihat, MTE Kahn . Centralized control system for islanded minigrid. AIMS Energy, 2023, 11(4): 663-682. doi: 10.3934/energy.2023033 |
[8] | Ahsan Iqbal, Ayesha Ayoub, Asad Waqar, Azhar Ul-Haq, Muhammad Zahid, Syed Haider . Voltage stability enhancement in grid-connected microgrid using enhanced dynamic voltage restorer (EDVR). AIMS Energy, 2021, 9(1): 150-177. doi: 10.3934/energy.2021009 |
[9] | W. E. P. Sampath Ediriweera, N. W. A. Lidula, R. Samarasinghe . Ring connected microgrid clusters for improved resiliency in distribution systems with high solar PV penetration. AIMS Energy, 2024, 12(4): 872-904. doi: 10.3934/energy.2024041 |
[10] | H. H. H. De Silva, D. K. J. S. Jayamaha, N. W. A. Lidula . Review on design and control of solid state transformer based microgrids. AIMS Energy, 2019, 7(6): 901-923. doi: 10.3934/energy.2019.6.901 |
The increase of the world electricity demand calls for the increased generation, but with the limitations faced by grid utility provider and the liberalized market for energy generation allowed the growth of the phenomena called Distributed Generation [1,2]. The concept Microgrid; which occurred concurrently with DG, introduced a new set of benefits which were not possible with the existing radial power system.
DGs installed near the load centers reduced the line losses and increased the reliability along with the power quality. These newly introduced DGs were mainly run on carbon-based fuel. Rising awareness of global warming and depleting natural resources made engineers rethink on adding more and more carbon-based distributed generation. Renewable energy distributed generation became the perfect candidate to become an alternative to the combustion DGs. Choice of location was the main advantage as it allowed the generators placed in most ideal areas where the renewable energy harvest is at maximum (e.g., Maximum solar irradiance, maximum wind speed). Sustainable energy generation became widely applied with technological development in the wind, solar, biomass, and fuel cell energy generation. A mix of renewable and fuel-based DG was often used to achieve the required generation within acceptable economic effectiveness [3,4]. Especially with the rapid development of photovoltaic film technologies and being economically viable through both short-run and medium-run [5], solar energy generation reached nearly 55% of newly installed capacity in 2017 [6]. Wind energy is the most favored micro-turbine Distributed Energy Resource (DER), which accounts for 5.6% of Global Electricity Production [6]. Although not all the DG with micro-turbine are sustainable as some run on natural gas or liquid fuel but provides much cleaner combustion comparatively with their heavy-capacity counterparts. Exploiting the waste heat from electricity generation was also made easier by the microgrids. Combined Heat and Power (CHP) systems were in a position to contribute at a domestic level by reducing the overall fuel usage, carbon emission, and increasing the efficiency of primary energy conversion. The limitations in long-distance heat transfer made CHP systems more valuable to applications like local space heating and hot water systems [7]. Sample structure of a microgrid system is illustrated in Figure 1.
Distributed Storage (DS) is also a key element in microgrid networks, which are usually included when the DG capacity is unable to handle the total load power requirement. In fact, integration of DS allows DG to operate at a steady level where the energy required for the load fluctuations are provided from the storage. The effects due to the intermittency of renewable DGs could be mitigated by using DS techniques. Furthermore, they damp the peak demand surges, improves the fault ride-through capability, and decreases the energy wastage. Batteries, Supercapacitors/Ultracapacitors, and flywheel systems are some DS mainly used with microgrids. Batteries store DC power as chemical energy and should be interfaced with power electronic systems, even in a DC bus for bi-directional power flow. Supercapacitors have high power density while the flywheel systems have faster response during interruptions [8].
Fuel cell technology is a more recent development in DG. Unlike the typical battery storages where the capacity limits the energy, fuel cells can generate energy as long as the fuel is supplied. Hydrogen-rich fuels such as biogas or natural gas encounter an electrochemical process to produce electricity, heat, and water. Due to the nature of the output, they are usually combined with CHP systems. Various researches [9] found that fuel cells have lower Greenhouse gas emission compared to combustion-based distributed generation. Table 1 categorizes the merits and drawbacks of microgrid systems.
Advantages | Disadvantages |
Benefits the environment through the lack of greenhouse gas emission through low/zero-emission generation technologies | Separate control schemes are required to operate within both technical and economical limits |
Through Islanding, microgrids can operate even during utility failures increasing the reliability levels | Use of battery-based energy storage systems can be expensive in both initiation and maintenance |
Can contribute to peak shaving of the grid network by distributed generation during peak hours | Intermittent nature of the renewable energy sources |
Used to electrify remote areas which have difficulties in connecting with the primary grid | Protection Challenges due to the distributed generation |
An ideal solution for the CHP requirements of customers by increased overall energy efficiency | |
The economic advantage of generating own electricity for a lower cost than from the main utility and even by exporting the energy back to the grid |
Voltage and frequency control is a common problem occurring due to the imbalance of electricity generation and consumption. Voltage and frequency variation problem arises due to the mismatch between generation and consumption of both active and reactive power values. Especially in the islanded mode, DGs must participate in active frequency controlling. Inverter interfaced micro sources do not occupy the frequency controlling ability where they are designed to output a constant power when the energy source is available [10]. This lack of inertia can cause fast frequency variations, and even small frequency change can snowball into a more significant problem. Droop control is a popular solution for frequency and voltage control (see Figure 2) but may not be the ideal solution in islanded microgrids, which will require further modifications [11]. Sophisticated power flow analysis methods like Newton trust region method [12] or communication-based approaches such as Multi-Agent Systems (MAS) [13] can be used to overcome power flow problems in islanded mode.
Two main sets of control methods can be identified as for grid-connected and islanded modes in microgrid operation. In fact, two types of set values and algorithms are vital for all microgrid control and protection tasks, while the detection of islanding should also be integrated into the microgrid control system. However, topological changes such as connection/disconnection of DG or loads, have made microgrid operation more complicated. These topological changes can also be the result of energy storage status and network reconfiguration due to faults or maintenance. Extensive communication infrastructure combined with mathematical algorithms is usually required to cope with these reconfigurations [14].
Power system stability, as identified by CIGRE and IEEE Task Forces, can be categorized into three areas [15]. Rotor angle stability, voltage stability, and frequency stability are these significant classes of system stability. But unlike traditional power systems, microgrids have less synchronous generation, and more Inverter interfaced Generation. The existence of different operating modes also contributes to the stability issues that arise in microgrids. Therefore, microgrid stability problems are mainly classified as Grid-connected issues and Islanded issues. In each of these modes, the stability issues are further segmented into two areas, namely small disturbance stability and transient stability [16]. Microgrid small-signal modeling and comprehensive control methods are required to overcome small disturbance stability issues. Unlike the traditional power systems, microgrids have less inertia prominently due to the lack of rotating mass where the majority of microgrid DGs are PE interfaced. Existence of PE interfaced DG mainly creates problems in maintaining system frequency during transient periods. Since most of the DGs in microgrids are renewable, power oscillations could occur frequently and should be balanced out to create stability. Intermittency of these Renewable DGs and the variations in loads can create significant power imbalances and therefore energy storage methods are vital in islanded microgrid operation.
Excessive use of power electronic inverters and converters, which is common in modern microgrids, can contribute to the increase of harmonic currents. The concepts of DC microgrids and AC/DC hybrid networks further increases the harmonic losses. As per the IEC 61000-3 standards, each source should contribute below the permissible limit of the Voltage Total Harmonic Distortion [17]. Otherwise harmonic compensation methods such as filters should be used. To further mitigate the harmonic current injection by microgrid inverters, harmonic compensation algorithms can be implemented [18].
Modern microgrids can have centralized, decentralized, or hierarchical network topologies where all three requires a robust communication network. Different types of microgrid messages occupy this communication channel, including protection information, control information, and monitoring information. The communication architecture should allow Real-time operation with minimum latency and sufficient bandwidth, reliability with the capability to handle a large number of nodes or agents and have higher security with less vulnerability to cyber-attacks [19].
Unlike the conventional power systems which have a unidirectional power flow, DG integrated microgrids have bi-directional power flow characteristics. Power flow variation creates a whole new problem series with relation to the fault current path concerning the location of the fault. Reconfiguration of the network topology and the states of islanded and grid-connected, further increases the complexity of the protection problem [20]. Fault protection methods must incorporate more than one approach to coping with the issues arising in microgrid networks protection problems are more systematically discussed in the next section.
Microgrids were initially popularized mainly due to their environmental and economic benefits with the addition of renewables. Thus, there is a pressure to live up to its reputation of being economical while creating them technologically feasible. Therefore, when presenting solutions to technical problems, they should also be acceptable in an environmental and commercial aspect [21].
There are two critical levels in the variation of fault current as of the microgrid status changes between grid-connected and islanded modes. During the islanded stage, short circuit capacity for the network is low, as the DGs have a much smaller capacity than the generation available in the utility grid. Due to this significant reduction of fault current [22], if the relay threshold values are selected according to the grid-connected mode, it will not be satisfactory for a fault occurred in the islanded mode. The short circuit current should be increased in this context to match with the settings, or the settings should be adjusted according to the topology. Both of these solutions have been attempted, and the second option is the main focus of this paper. The most common method of increasing the fault current is by adding a fault current source to the network [23].
Conventional distribution systems are radial with unidirectional power flow. Flow direction is from single or multiple sources at one end to the load at the opposite end. With the DG penetration of microgrids, this one-directional flow could change its direction according to the status of local generation and local consumption [24]. Because of this reverse power flow, magnitude, and direction of the fault current may change. This makes conventional protection coordination invalid, and the necessity for a directional protection element arises.
Unnecessary tripping is also identified as selectivity issues or sympathetic tripping. When a fault occurs in a feeder powered by multiple sources; both utility and DG, each can contribute to the fault current. The problem occurs when a healthy portion of the system or a DG is disconnected due to the fault, instead of isolating the faulty feeder [25]. Unnecessary trippings constitute a significant threat to the reliability of the network. Example of this phenomena is illustrated in Figure 3 as relay R1 trips for a fault in the relay R2's protection zone.
Under-Reach, also known as blinding of protection, occurs from the grid fault current reduction due to the addition of DGs. As the fault current contribution decreases with the addition of DGs according to Figure 4a, the grid fault current may not be sufficient to trip the breaker associated with relay R3.
Overreach occurs with a fault occurring downstream of the DG connected bus, during the grid-connected operation. As per the scenario in Figure 4b, Relay R2 will operate for the fault beyond its zone due to the increased fault current when compared with a non-DG scenario.
Loss of Mains (LOM) or Loss of Grid (LOG) scenario can be identified as losing the power from the parent grid. This can either be a disconnection from PCC or a higher level. If it is from PCC, the microgrid becomes islanded and creates problems when DG cannot provide enough capacity to feed the local loads. Frequency stability and voltage dips can occur from the LOM [26]. In the other type of disconnection where a part of unenergized utility is connected with the DG fed microgrid, actions should be taken to either disconnect the DGs or switch to an islanded mode to prevent power from been fed back into the utility. A fast and reliable LOM detection method should be employed in order to cope with these scenarios effectively [27].
Integration of a DG to a microgrid could increase the short circuit level associated with all of the network buses. The level of this increment will depend on the location, size, and type of the interconnecting DG [28]. Furthermore, the type of these DGs also contributes to this variation in a much diverse context. Synchronous DGs can contribute up to 5 times its rated current while PE interfaced DGs can only contribute up to 1.5 to 2 times to the short circuit capacity of the network [29]. Moreover, this value of short circuit current is pre-defined in the inverter control system [30]; therefore, different types of PE interfaces have different short circuit levels. Induction generator based DGs also fall behind when providing short circuit current when compared to synchronous type DGs [31]. Different types of renewable DG source addition could further increase the complexity of varying short circuit level. Two types of most commonly used wind energy generation techniques, synchronous generator (SG) and doubly-fed induction generators (DFIG) has different symmetrical short circuit (SSC) characteristics with SG having about twice the value of DFIG for maximum short circuit capacity [32].
Adaptive protection, as it says in the name, is an online protection system that changes the fault response according to the system's state. There are numerous techniques of applying the adaptive protection to a microgrid system, but the simplest and the classic approach is to have two sets of relay-set-values each for islanded and grid-connected modes. Relay settings would be updated with the change of microgrid status and usually includes the shifting of relay characteristic curve to cope with the change of fault current [33]. There are several other variants of adaptive protection schemes which are discussed further in the rest of the review.
Adaptive protection is one of the less complicated approaches with reasonable implementation cost, which makes it a favorable solution. Adaptive protection method requires to have prior knowledge on microgrid configurations in order to perform power flow and short circuit calculations to identify the ideal relay settings for each possible setup. It may also depend on a communication method in order to update the settings at each network reconfiguration. Implementing an adaptive protection scheme can require some upgrade in protection infrastructures such as microprocessor-based relays, communication channels and software programs for appropriate control.
Differential protection is mainly used in the islanded mode, where the fault current is lower and difficult to detect using conventional methods. The differential method cannot be used as a complete protection solution. It is more suited to detect downstream earth faults while some other techniques should be adopted to identify further faults like upstream ground faults, Line-to-Line (L-L) faults, and symmetrical faults [34]. This method also employs communication links for the differential operation.
Voltage-based protection utilizes the voltage measurements to initiate protection measures to various faults. Al-Nasseri et al. [35] have developed a voltage-based protection method applicable to both internal and external faults. They perform abc-dq transformations on voltage waveform to identify the short circuit fault type and location. A further developed scheme of voltage-based protection by using digital data acquisition was presented by Wang et al. [36]. They have only tested the system for a laboratory-scale system. This method mainly eliminates the complications caused by different short circuit levels in microgrids but can create maloperation in any voltage disturbances.
This method depends heavily on the network configuration, and may not be viable with reconfiguring networks. It also falls short when detecting high impedance faults. When the microgrid network becomes more extensive, calculating the park transformation becomes complex and can increase the fault detection time if enough processing power is not available.
The classic overcurrent protection should be modified to match with the existence of DG and varying fault levels for it to be applicable in the microgrids. It is usually integrated with a directional feature. Zamani et al. [37] have developed a digital relay design that is functional in both islanded and grid-connected modes. Lotfi-fard et al. [38] have presented a protection scheme based on symmetrical current components. An algorithm has been implemented to identify faulty conditions based on zero, positive, and negative components of the current in this context. Furthermore, the identifying of non-faulty transients such as induction motor starting or transformer energizing to prevent maloperation of the relays has also been included in this research.
The main drawback of this approach is the requirement for infrastructure upgrade, including communication channels. In case of a communication failure, adequate backup protection must be included in order to satisfy the safe operation.
Impedance-based fault location techniques have been applied to identify the fault location of radial systems without DG presence. In recent years, this method was modified to include microgrid scenarios with DG penetration by different studies, including the works of Nunes and Bretas [39]. A communication assisted impedance-based protection method is presented by Elkhatib et al. [40]. Relays are equipped with impedance element and directional element to identify the occurrence and direction of the fault. According to the implemented pilot protection logic, the relays relevant to the fault will receive a permissive signal while others will get a blocking signal.
Distance protection is usually applied for the protection of transmission lines and it measures the voltage and current at the relay point to calculate the impedance and utilizes to detect faults in its defined protection zone. The works of Dewadasa et al. [41] present a distance protection method based on mho characteristics. However, it reports about the unnecessary operation concerning the coordination rules and requires further modifications with a directional feature.
Communication between relays is not required in the distance protection which is a main advantage of the method. This method may have some inaccuracies when considering the current harmonics and transients. The resistance of the fault and shorter distribution lines could create errors in the measured admittance.
This method is based on Stockwell transform (S-transform) which is a time-frequency transform technique used to perform a differential energy-based analysis. Kar et al. [42] used data from both ends of the faulty feeder and subject it to the S-transform to obtain the differential energy. This differential energy is used to identify fault patterns of the microgrid by comparing with pre-identified patterns for different fault scenarios. The trip signal is issued according to the threshold level and the simulation results of the above study show that healthy and faulty occasions are easily distinguishable. The main drawback of this type of protection is that it requires more data (frequency, time) to formulate differential energy contours and to make a decision. Gururani et al. [43] used Hilbert-Huang transform instead of the s-transform to get the differential energy and found that it has better performance in varying conditions due to instantaneous extraction.
Multiagent systems operate using distributed agents which could be either software or hardware, also known as Intelligent Electronic Devices (IED), working collectively to achieve protection of the microgrid. This is a more decentralized approach with different layers in the system. Cintuglu et al. [44] proposed a multi-agent-based protection system developed for overcurrent and frequency problems. It utilizes the IEC 61850 GOOSE communication protocol and makes use of an adaptive technique to overcome frequency and overcurrent selectivity problems. The main issue with the multi-agent system is when the number of agents increase, the complexity and the cost of communication protocol also increases.
Wide area protection schemes employ supervisory control and data acquisition (SCADA) and Intelligent Electronic Devices (IED) to collect measured values in a wider area and perform protective actions based on the data. As reviewed by Luo et al. [45], wide-area protection technology is being evolved with the integration of other protection schemes such as adaptive and multi-agent. Wide area protection method requires a backup protection method in case of a communication failure.
As presented above, it is clear that none of the methods qualify to provide overall protection for a given network (see Table 2). It is common to use a combined approach in designing microgrid protection methods in order to achieve better performance. Adaptive protection is a favorable choice to use in combination with other methods to provide better protection performance.
Protection Scheme | Method | Features |
Adaptive | Relay setting changes according to network state | Online system Requires network upgrades Prior knowledge on configurations Communication requirement Fault calculations for relay settings |
Differential | Comparison of input and output current of a zone | Immune to the current flow direction and magnitude variations Problems due to unbalances and transients Depends on the communication channel |
Current-based | Current symmetrical component | Problems with unsymmetrical loads due to single-phase DGs Depends on the communication |
Voltage-based | Voltage measurements a b c to d-q transformation | HIF cannot be detected Poor accuracy in grid-connected and varying networks Voltage drops can create errors |
Impedance-based (incl. distance protection) | Measured impedance with threshold values | Accuracy affected by harmonics and transients Errors due to fault impedance Not effective with short-range lines |
Differential energy | Time-frequency transformation with threshold | Applicable to most fault types Long computational durations Pre-calculated threshold values |
Multi-agent protection | Hardware and software agents at critical points | Become expensive and complex with larger systems |
Wide-area protection | IED with fault zone matrix | Not validated for comprehensive systems |
The concepts of adaptive protection were among the electrical researchers focus from as early as the 1960s. Digital relaying concepts were also developed in this era [46], which enabled the conceptualization of adaptive protection. The adaptation of relay settings according to the system conditions, was initially proposed by Tomas Liacco [47] in 1967. As this was before the introduction of microgrids, the purpose of the early version of adaptive protection was mainly to improve the essential protection characteristics: sensitivity, selectivity, and speed. Adaptive protection schemes evolved through the years, and by 1989, it was proposed for transmission protection [48]. From there on the concept of adaptive protection has evolved and utilized in different methodologies and combined with other protection schemes.
Nikkhajoei et al. [49] have presented a method that includes intentional islanding. They are willing to apply the same protection strategy to both grid-connected and islanded operation. They included a static switch at the Point of Common Coupling (PCC) which opens on detection of a fault and enable fault clearing techniques. In this way, they will not have high fault currents. This method is not feasible in modern microgrids as the DG will not always provide the load requirement.
Fault Current Limiters (FCL) are employed in fault protection of microgrids due to their dynamic impedance characteristics. Najy et al. [50] have formulated an optimization problem for the optimum value for the FCL for both grid-connected and islanded modes so that the relay settings can stay the same. Optimal OCR settings were found and tested for both radial and meshed systems and obtained satisfactory results. The major shortcoming of this scheme is that they mainly focused on Conventional Synchronous Generator (CSG) type DGs. As mentioned in section 3.6, Synchronous type DGs have the highest fault current contribution when compared with PE interfaced DG and Induction type DGs. This method needs a problem reformulation to be applicable with PE interfaced DGs and microgrids including energy storage.
Lu et al. [51] focus on using FCL with Inverter dominant microgrids with a method called Virtual-impedance-based fault current limiter. The proposed system is to implement the FCL capabilities inside the inverter control loop rather than using additional hardware. The proposed method is validated only in Software and Hardware-in-loop simulations. Specially designed DG interfaced with controllable inverters will be required to implement this method in a practical network.
There have been studies on increasing the fault current as well. Van Overbeeke [52] used a Fault Current Source (FCS) to inject additional fault current in the islanded operation to match with the grid-connected operation. The researcher suggests flywheel, battery, or super-capacitor as the FCS. These types of energy storage methods require significant capital investment and may not provide the fastest fault clearing if they fail to match grid-connected short circuit levels.
Brahma and Girgis [53] take a zoned approach to face protection problems. They focus their method on a microgrid with high DG penetration for each zone to have a balance between generation and load. Each area should have a frequency controlling generator and circuit breakers with remote operating capabilities at the interconnections of zones. It requires synchronized current measurements at each DG and utility and the direction of current flow between zones. This method also performs off-line power flow and short-circuit analysis for all possible scenarios and recalculated after each reconfiguration. This method further integrates auto reclosing to segregate temporary faults, and the simulation results prove its accuracy increases with more DG integration. However, drawbacks of this system are not feasible on low DG microgrids, dependability on communication channels and the expenses of phasor measurement units. It also assumes zero fault impedance and may provide maloperation with a fault having a resistance.
In 2009 Oudalov et al. [54] proposed an adaptive protection scheme with digital relays to check and update its parameters through advanced communication infrastructure. They have performed an off-line short circuit analysis including DERs status and recorded it in an event table with the pre-calculated relay settings for each status. Whenever a fault is triggered, the controller takes real-time values and estimates the corresponding status on the event table and uploads the relevant settings to the on-field devices. Furthermore, they have included a 'follow-me' type hardware interlock function to improve the selectivity of the system. In contrast, the major drawbacks of this method include directional numerical overcurrent relays, and due to the use of a look-up table, the entire microgrid configuration should be known beforehand. Also, whenever a different topology or deployment has been made, the whole calculations, including short-circuit and relay parameters, should be repeated.
An adaptive protection method based on the system impedance is developed by Han et al. [55] in 2010. System impedance is calculated using measured voltage and current fault component, which is obtained from the Fourier filter algorithm. Through the impedance comparison, relevant relay settings are updated. It also identifies an applicability issue where the non-fault component extraction is limited up to two cycles within the occurrence of the fault. Therefore, it is only suitable in quick fault clearance and not suitable as a backup protection method.
In 2011, Dang et al. [56] have presented an adaptive protection method with selectivity and reliability improvements. Protection Devices (PDs) perform mode discrimination through comparing zero sequence impedance angle, and voltage dq0 transformation is used to identify the fault in the islanded mode while the traditional method of overcurrent protection is kept at the grid connection mode. Further protection coordination is performed by detecting the zero-sequence current flowing through each PD. They have not performed a simulation as only the demonstrated the strategy.
Same year Ustun et al. [57] utilized a Central Protection Unit (CPU) with an intensive communication system to identify the microgrid connection/disconnection (with utility) and DGs connection/disconnection. Here DGs should be able to report its status, rated current and fault contribution, while the interrupt-based algorithm updates the relay settings. All the DGs and relays need to be connected to the CPU through the communication channel, and the CPU should be powerful with faster data acquisition and multi-threaded processing to keep up with the triggers in providing the output settings.
Multi-agent-based adaptive protection with the use of distributed intelligence was proposed into an MV level application by Kauhaniemi and Voima [58] in 2012. It employs the IEDs located in new smart grid networks. As this is in the concept stage, not much application details are discussed. It admits the vulnerability of the system through communication failure and suggests a system to monitor telecommunication links and predefined backup protection settings. Its main goal is to create the largest possible island in a fault situation and provides a base for smart-grid functionalities such as self-healing.
Power Line Communication (PLC) was the primary communication method used for power system applications before the popularization of wireless technologies. Now there are many wireless protocols used in power system applications, namely ZigBee, WLAN, cellular communication, and WiMAX. In 2013 Ustun et al. [59] have developed a WiMAX (Worldwide Interoperability for Microwave Access, Inc) based adaptive protection scheme. It utilizes the bi-directional communication capability between CPC and relay/DG to gather data on fault current direction, DG status/contribution, and to update relay settings. Use of WiMAX reduces the infrastructure implementation and maintenance cost relative to fiber optics network. They also performed a simulation to verify the communication broadcast latency does not exceed 40 ms (2 cycles of the 50Hz system) as their primary focus of the study was on the communication architecture.
In 2014 Kauhaniemi et al. [60] studied the directional and distance protection methods and proposed a hybrid adaptive protection method based on the results of the study. They found that distance protection can perform well under the islanded mode, where directional fails to perform without modification. Their proposed method allows selectivity operation in network changes.
Lin et al. [61] proposed an adaptive distance protection method that has better selectivity and sensitivity features comparatively with adaptive overcurrent and adaptive differential protection methods. PMU integrated into modern IED are used for the state estimation, and the control center executes the adapted decision through on-field-devices. Mho characteristics-based distance protection is integrated with three protection zones, and the simulation suggests it has better performance among overcurrent and differential protection methods.
In 2016 Sitharthan et al. [62], have utilized Electronically Coupled Distributed Energy Resources (EC-DER) such as wind, solar, fuel cells, and battery to develop an adaptive protection scheme. They have developed algorithms for each microgrid status separately and then combined it to achieve adaptive protection. A fault is identified using negative sequence current and occupy the Microgrid Common Medium (MCM) as the communication method. PSCAD simulation represents the reduction of operating time for the grid-connected and islanded operation. Furthermore, the ride-through capability for temporary faults was added using an Auto Recloser Module (ARM).
Research | Method | Features | Remarks | ||
Fault Type | Communication | Voltage Level | |||
[53] | PMU based adaptive | All | Yes | LV | Zoned Approach, Accurate only for High DG Penetration, Calculation only |
[54] | Numerical Directional Overcurrent with Central control | All | Yes | LV | Offline analysis-based event table, Directional Interlock, Simulation by calculations |
[55] | Fault component extraction (Impedance based) | L-G L-L-L-G | No | LV | Works with IIDG only, not suitable as backup protection, Simulation only |
[58] | IED based Multi-agent | All | Yes | MV | Decentralized communication, only conceptual design |
[59] | Microgrid CPU with WiMAX | - | Yes | LV | Focused on communication latency, Centralized |
[60] | Directional & Distance adaptive | L-L-L | Yes | MV/LV | Changing the protection method between directional and distance |
[61] | PMU based adaptive distance | All | Yes | MV | Event table-based, Tested on an actual system |
[62] | EC-DER embedded protection | All (Simulated for L-L-G) | Yes | LV | Only for low fault impedances, Auto recloser function, Simulation only |
Main areas of Computational Intelligence (CI) methods can be identified as Data mining, Machine Learning, and Nature-Inspired Algorithms (NIA). Authors have used techniques from all these categories to derive adaptive protection solutions.
There are three significant areas where CI can be useful, namely the current operating topology identification, calculation of the protection device settings, and rule-based application of the settings mentioned above. In most of the adaptive protection schemes, the possible topology configurations are pre-determined, and identification of these different topologies is done manually. Data mining and Neural network approaches can drastically increase the efficiency and reduce the calculation burden on network topology identification phase.
Data mining and analytics more commonly called as 'Big Data' can play a vital role in modern microgrids. Microgrids can produce data on generation, transmission, distribution, and consumption. These data are usually measured using installed sensors and smart meters in the microgrid network. The amount of data collected by a group of 1 million smart meters through the course of a year can be as high as 35.04 billion [63]. This vast amount of data undergoes data mining procedures to produce meaningful data records. These data records lay the foundation in applying CI for microgrid protection.
Decision tree (DT) based microgrid adaptive protection system was developed by Mishra et al. [64]. In this method, current signals are subjected to wavelet transformation, and the results during different transients are used to train the DT model. Two DTs are used one for identifying the existence and the other for the classification of the fault. System was extensively trained for faults occurring at different operating modes. The final accuracy of fault detection DT was 97% while the fault classification accuracy was at 85%.
In 2018, Tang et al. [65] have utilized DT methods to achieve adaptive overcurrent protection. They use Simple Binary DT to pinpoint the system condition and ANN model to train and apply adaptive relay settings. Data mining and simple initial calculation are done using IEDs embedded in the network, and other computations are carried out by a centralized controller. Simulation results were taken for a couple of systems, and it showed zero violations in relay settings during different operating modes.
Hengwei et al. [66] have developed an adaptive overcurrent protection system based on synchronous phase measurement techniques and ANN. Two ANN models were created as one for identifying the faulty conditions, and the other was trained to locate the fault. The estimated fault location was compared with the on-field measurement results and a decision was confirmed and performed. All the details on the actions were recorded for future AI training purposes. This method requires extensive processing power for the operation, and they have only included an algorithm level simulation which needs more support before validation.
A Fuzzy-based adaptive protection system was developed by Chaitanya et al. [67]. Rather than only detecting the fault, they further consider fault classification and fault phase detection. For the utility faults, microgrid enters the islanded mode and continue operation while during a fault in the microgrid, the protection scheme identifies and isolates the relevant phase(s) without losing the grid connection. Fuzzy Based Fault Identifiers aided with current sequential and phasor measurements from all microgrid terminals. The technique is validated by simulating and faster operating times are observed even during dynamic operating conditions.
Lin et al. [68] have presented a protection system that utilizes machine learning extensively. It tackles the microgrid uncertain elements with Pearson correlation coefficients and the rule-based adaptiveness is achieved by a hybrid system. ANN model was developed to identify the presence of the fault and a Support Vector Machine (SVM) is used to identify the fault location. The error percentages of the SVM stays below 1% in the simulated case studies. However, the ANN model accuracy drops to 77.5% and 80.5% in some lines.
Relay setting calculation by NIA has been the focus for a large number of recent researches. It allows much faster calculation along with more optimized results. In the case of adaptive protection, relay settings for each configuration can be easily calculated by NIA methods. Srivastava et al. [69] used a Hybrid algorithm based on Particle Swarm Optimization (PSO) and Gravitational Search Algorithm to calculate relay settings for a reconfigurable network. Two operating cases were studied and found the optimal relay settings given by the algorithm. The hybrid algorithm provided a better total operating time of relays when compared with other algorithms. The drawback of this method is unlike previous machine learning methods, calculations for each case should be done manually.
This paper presents a comprehensive review of microgrid adaptive protection researches. Due to the challenging nature of microgrid protection, there are various solutions suggested by literature each having its own merits and demerits. Due to the dynamic and uncertain nature of microgrid network, an adaptive solution is an ideal scheme. Adaptive protection is more favored to be combined with, in order to create a complete protection solution. These protection solutions can be either centralized or decentralized, and the existence of communication links is common but not compulsory.
This paper has reviewed the development of adaptive protection schemes throughout the past decade, followed by the introduction of microgrid protection problems and various solutions. The main observations of these protection methods are that none of them have evolved into a mainstream solution due to the short life cycle of each scheme. The rapid technological advancement of related technologies is the main reason for this.
Most recent developments have been focused on using CI methods. These CI methods have made the protection system operation simple and also allows implementing much more sophisticated features. Most of these novel protection methods eliminate the necessity of classic relays. They obtain network data through IEDs, and a machine learning model replaces the relay function and capable of directly controlling the circuit breakers for accurate fault clearances. The pinnacle of these continuing researches would be an intelligent all-in-one circuit breaker which single-handedly performs data mining, processing and executing fault clearing tasks.
The authors gratefully acknowledge the support provided by the National Research Council Sri Lanka; Grant number 17-065 and Senate Research Committee University of Moratuwa; Grant number SRC/TP/2017/03.
The author declares that there is no conflict of interests in this paper.
[1] | Jenkins N (2008) Embedded Generation. 1st Eds. London: The Institution of Engineering and Technology. |
[2] | Hatziargyriou N, Asano H, Iravani R, et al. (2007) Microgrids. IEEE Power and Energy Mag 5: 78–94. |
[3] |
Ahmed M, Amin U, Aftab S, et al. (2015) Integration of renewable energy resources in microgrid. Energy Power Eng 7: 12–29. doi: 10.4236/epe.2015.71002
![]() |
[4] |
Tanrioven M (2005) Reliability and cost–benefits of adding alternate power sources to an independent micro-grid community. J Power Sources 150: 136–149. doi: 10.1016/j.jpowsour.2005.02.071
![]() |
[5] |
Baker E, Fowlie M, Lemoine D, et al. (2013) The economics of solar electricity. Annu Rev Resour Econ 5: 387–426. doi: 10.1146/annurev-resource-091912-151843
![]() |
[6] | Renewables Global Status Report-REN21 2018. Available from: http://www.ren21.net/status-of-renewables/global-status-report. |
[7] | Lasseter R, Akhil A, Marnay C, et al. (2002) Integration of distributed energy resources. The CERTS Microgrid Concept. Lawrence Berkeley National Lab. (LBNL), Berkeley, CA, United States. |
[8] | Kroposki B, Lasseter R, Ise T, et al. (2008) Making microgrids work. IEEE Power Energy Mag 6: 40–53. |
[9] | Elgowainy A, Wang MQ (2008) Fuel cycle comparison of distributed power generation technologies. Argonne National Lab. (ANL), Argonne, IL, United States. |
[10] |
Lopes JAP, Moreira CL, Madureira AG (2006) Defining control strategies for microGrids islanded operation. IEEE Trans Power Syst 21: 916–924. doi: 10.1109/TPWRS.2006.873018
![]() |
[11] |
Mohamed Y, El-Saadany EF (2008) Adaptive decentralized droop controller to preserve power sharing stability of paralleled inverters in distributed generation microgrids.IEEE Trans Power Electron 23: 2806–2816. doi: 10.1109/TPEL.2008.2005100
![]() |
[12] |
Abdelaziz MMA, Farag HE, El-Saadany EF, et al. (2013) A novel and generalized three-phase power flow algorithm for islanded microgrids using a Newton trust region method.IEEE Trans Power Syst 28: 190–201. doi: 10.1109/TPWRS.2012.2195785
![]() |
[13] | Chamorro HR, Diaz NL (2013) Hierarchical power flow control in low voltage microgrids. 2013 North American Power Symposium (NAPS). 1–5. |
[14] |
Erseghe T, Tomasin S, Vigato A (2013) Topology estimation for smart micro grids via powerline communications.IEEE Transactions on Signal Processing 61: 3368–3377. doi: 10.1109/TSP.2013.2259826
![]() |
[15] |
Kundur P, Paserba J, Ajjarapu V, et al. (2004) Definition and classification of power system stability IEEE/CIGRE joint task force on stability terms and definitions.IEEE Transactions on Power Systems 19: 1387–1401. doi: 10.1109/TPWRS.2004.825981
![]() |
[16] |
Shuai Z, Sun Y, Shen ZJ, et al. (2016) Microgrid stability: Classification and a review. Renewable Sustainable Energy Rev 58: 167–179. doi: 10.1016/j.rser.2015.12.201
![]() |
[17] | International Electrotechnical Commission (2008) Electromagnetic compatibility (EMC). Part 3–6: IEC TR 61000-3-6:2008 Limits-Assessment of emission limits for the connection of distorting installations to MV, HV and EHV power systems. Geneva: IEC. |
[18] | Micallef A, Apap M, Spiteri-Staines C, et al. (2015) Mitigation of harmonics in grid-connected and islanded microgrids via virtual admittances and impedances.IEEE Trans Smart Grid: 1–11. |
[19] |
Marzal S, Salas R, González-Medina R, et al. (2018) Current challenges and future trends in the field of communication architectures for microgrids. Renewable Sustainable Energy Rev 82: 3610–3622. doi: 10.1016/j.rser.2017.10.101
![]() |
[20] | Zeineldin HH, El-saadany EF, Salama MMA (2006) Distributed Generation Micro-Grid Operation: Control and Protection. 2006 Power Systems Conference: Advanced Metering, Protection, Control, Communication, and Distributed Resources. Clemson, SC, USA: IEEE. 105–111. |
[21] | Asanol H, Bandol S (2007) Economic analysis of microgrids. 2007 Power Conversion Conference-Nagoya. Nagoya, Japan: IEEE. 654–658. |
[22] | Maqbool U, Khan UA (2017) Fault current analysis for grid-connected and Islanded microgrid modes. 2017 13th International Conference on Emerging Technologies (ICET). Islamabad: IEEE. 1–5. |
[23] | Overbeeke FV (2009) Fault current source to ensure the fault level in inverter-dominated networks. Proc. 20th International Conference and Exhibition on Electricity Distribution. CIRED. 1–4. |
[24] | Chatterjee S, Agarwal M, Sen D (2015) The challenges of protection for Microgrid. International Advanced Research Journal in Science, Engineering and Technology (IARJSET) 2. 155–158. |
[25] | Choudhary NK, Mohanty SR, Singh RK (2014) A review on Microgrid protection. 2014 International Electrical Engineering Congress (iEECON). Chonburi, Thailand: IEEE. 1–4. |
[26] | Buque C, Chowdhury S, Chowdhury SP (2013) Modelling and simulation of reverse power relay for loss of mains protection of distributed generation in microgrids. 2013 IEEE Power & Energy Society General Meeting. Vancouver, BC: IEEE. 1–5. |
[27] | Crolla P, Roscoe AJ, Dysko A, et al. (2011) Methodology for testing loss of mains detection algorithms for microgrids and distributed generation using real-time power hardware-in-the-loop based technique. 8th International Conference on Power Electronics-ECCE Asia. Jeju, Korea (South): IEEE. 833–838. |
[28] | Uqaili MA, Sahito AA, Halepoto IA, et al. (2014) Impact of distributed generation on network short circuit level. 2014 4th International Conference on Wireless Communications, Vehicular Technology, Information Theory and Aerospace & Electronic Systems (VITAE). Aalborg, Denmark: IEEE. 1–5. |
[29] |
Brearley BJ, Prabu RR (2017) A review on issues and approaches for microgrid protection.Renewable Sustainable Energy Rev 67: 988–997. doi: 10.1016/j.rser.2016.09.047
![]() |
[30] | Plet CA, Graovac M, Green TC, et al. (2010) Fault response of grid-connected inverter dominated networks. IEEE PES General Meeting. Minneapolis, MN: IEEE. 1–8. |
[31] |
Freitas W, Vieira JCM, Morelato A, et al. (2006) Comparative analysis between synchronous and induction machines for distributed generation applications.IEEE Trans Power Syst 21: 301–311. doi: 10.1109/TPWRS.2005.860931
![]() |
[32] |
Nazir MS, Wu Q, Li M, et al. (2017) Symmetrical short circuit parameter differences of double fed induction generator and synchronous generator based wind turbine.Indones J Electr Eng Comput Sci 6: 268. doi: 10.11591/ijeecs.v6.i2.pp268-277
![]() |
[33] |
Che L, Khodayar ME, Shahidehpour M (2014) Adaptive protection system for microgrids: Protection practices of a functional microgrid system. IEEE Electrification Mag 2: 66–80. doi: 10.1109/MELE.2013.2297031
![]() |
[34] |
Akhtar Z, Saqib MA (2016) Microgrids formed by renewable energy integration into power grids pose electrical protection challenges. Renewable Energy 99: 148–157. doi: 10.1016/j.renene.2016.06.053
![]() |
[35] | Al-Nasseri H, Redfern MA, Li F (2006) A voltage based protection for micro-grids containing power electronic converters. 2006 IEEE Power Engineering Society General Meeting. Montreal, Que., Canada: IEEE. |
[36] | Wang X-p, Li Y, Yu Y-y (2011) Research on the relay protection system for a small laboratory-scale microgrid system. 2011 6th IEEE Conference on Industrial Electronics and Applications. Beijing, China: IEEE. 2712–2716. |
[37] |
Zamani MA, Sidhu TS, Yazdani A (2011) A protection strategy and Microprocessor-Based relay for Low-Voltage microgrids.IEEE Trans Power Delivery 26: 1873–1883. doi: 10.1109/TPWRD.2011.2120628
![]() |
[38] |
Lotfi-fard S, Faiz J, Iravani R (2007) Improved overcurrent protection using symmetrical components.IEEE Trans Power Delivery 22: 843–850. doi: 10.1109/TPWRD.2007.893591
![]() |
[39] | Nunes JUN, Bretas AS (2011) A impedance-based fault location technique for unbalanced distributed generation systems. 2011 IEEE Trondheim PowerTech. Trondheim: IEEE. 1–7. |
[40] | Elkhatib ME, Ellis A (2017) Communication-assisted impedance-based microgrid protection scheme. 2017 IEEE Power & Energy Society General Meeting. Chicago, IL: IEEE. 1–5. |
[41] | Dewadasa JM, Ghosh A, Ledwich G (2008) Distance protection solution for a converter controlled microgrid.Fifteenth National Power Systems Conference (NPSC), ⅡT Bombay, 586–591. |
[42] |
Kar S, Samantaray SR (2014) Time-frequency transform-based differential scheme for microgrid protection. IET Gener, Transm Distrib 8: 310–320. doi: 10.1049/iet-gtd.2013.0180
![]() |
[43] |
Gururani A, Mohanty SR, Mohanta JC (2016) Microgrid protection using Hilbert–Huang transform based-differential scheme. IET Gener, Transm Distrib 10: 3707–3716. doi: 10.1049/iet-gtd.2015.1563
![]() |
[44] |
Cintuglu MH, Ma T, Mohammed OA (2017) Protection of autonomous microgrids using Agent-Based distributed communication.IEEE Trans Power Delivery 32: 351-360. doi: 10.1109/TPWRD.2016.2551368
![]() |
[45] |
Luo L, Tai N, Yang G (2012) Wide-area protection research in the smart grid. Energy Proc 16: 1601–1606. doi: 10.1016/j.egypro.2012.01.249
![]() |
[46] | Rockefeller G (1969) Fault protection with a digital computer.IEEE Trans Power Appar Syst PAS-88: 438–464. |
[47] |
Dy Liacco T (1967) The adaptive reliability control system.IEEE Trans Power Appar Syst PAS-86: 517–531. doi: 10.1109/TPAS.1967.291728
![]() |
[48] |
Kumar JA, Venkata SS, Damborg MJ (1989) Adaptive transmission protection: concepts and computational issues. IEEE Trans Power Delivery 4: 177–185. doi: 10.1109/61.19203
![]() |
[49] | Nikkhajoei H, Lasseter RH (2007) Microgrid protection. 2007 IEEE Power Engineering Society General Meeting. Tampa, FL, USA: IEEE. 1–6. |
[50] | Najy WKA, Zeineldin HH, Woon WL (2013) Optimal protection coordination for microgrids with Grid-Connected and islanded capability.IEEE Trans Ind Electron 60: 1668–1677. |
[51] |
Lu X, Wang J, Guerrero JM, et al. (2018) Virtual-Impedance-Based fault current limiters for inverter dominated AC microgrids.IEEE Trans Smart Grid 9: 1599–1612. doi: 10.1109/TSG.2016.2594811
![]() |
[52] | Van Overbeeke F (2009) Fault current source to ensure the fault level in inverter-dominated networks. IET Conference Publications. Prague, Czech Republic: IET. 369–369. |
[53] | Brahma SM, Girgis AA (2004) Development of adaptive protection scheme for distribution systems with high penetration of distributed generation.IEEE Trans Power Delivery 19: 56–63. |
[54] | Oudalov A, Fidigatti A (2009) Adaptive network protection in microgrids. 24 Int J Distrib Energy Resour 5: 201–225. |
[55] | Han Y, Hu X, Zhang D (2010) Study of adaptive fault current algorithm for microgrid dominated by inverter based distributed generators. The 2nd International Symposium on Power Electronics for Distributed Generation Systems. Hefei, China: IEEE. 852–854. |
[56] | Dang K, He X, Bi D, et al. (2011) An adaptive protection method for the inverter dominated microgrid. 2011 International Conference on Electrical Machines and Systems. Beijing, China: IEEE. 1–5. |
[57] | Ustun TS, Ozansoy C, Zayegh A (2011) A microgrid protection system with central protection unit and extensive communication. 2011 10th International Conference on Environment and Electrical Engineering. Rome, Italy: IEEE. 1–4. |
[58] | Kauhaniemi K, Voima S (2012) Adaptive relay protection concept for smart grids.Renewable Efficient Energy Ⅱ Conference, Vaasa, Finland. |
[59] | Ustun TS, Khan RH, Hadbah A, et al. (2013) An adaptive microgrid protection scheme based on a wide-area smart grid communications network. 2013 IEEE Latin-America Conference on Communications. Santiago, Chile: IEEE. 1–5. |
[60] | Kauhaniemi K, Voima S, Laaksonen H (2014) Adaptive protection scheme for smart grids. 12th IET International Conference on Developments in Power System Protection (DPSP 2014). Copenhagen, Denmark: IET. 1–6. |
[61] | Lin H, Guerrero JM, Vasquez JC, et al. (2015) Adaptive distance protection for microgrids. IECON 2015-41st Annual Conference of the IEEE Industrial Electronics Society. Yokohama: IEEE. 725–730. |
[62] |
Sitharthan R, Geethanjali M, Karpaga Senthil Pandy T (2016) Adaptive protection scheme for smart microgrid with electronically coupled distributed generations. Alexandria Eng J 55: 2539–2550. doi: 10.1016/j.aej.2016.06.025
![]() |
[63] |
Zhang Y, Huang T, Bompard EF (2018) Big data analytics in smart grids: a review. Energy Inf 1: 8. doi: 10.1186/s42162-018-0007-5
![]() |
[64] |
Mishra DP, Samantaray SR, Joos G (2016) A combined wavelet and data-mining based intelligent protection scheme for microgrid.IEEE Trans Smart Grid 7: 2295–2304. doi: 10.1109/TSG.2015.2487501
![]() |
[65] |
Tang WJ, Yang HT (2018) Data Mining and Neural Networks Based Self-Adaptive Protection Strategies for Distribution Systems with DGs and FCLs. Energies 11: 426. doi: 10.3390/en11020426
![]() |
[66] | Hengwei L, Guerrero JM, Chenxi J, et al. (2016) Adaptive overcurrent protection for microgrids in extensive distribution systems. IECON 2016-42nd Annual Conference of the IEEE Industrial Electronics Society. Florence, Italy: IEEE. 4042–4047. |
[67] | Chaitanya BK, Soni AK, Yadav A (2018) Communication assisted fuzzy based adaptive protective relaying scheme for microgrid.J Power Technol 98: 57–69. |
[68] |
Lin H, Sun K, Tan Z-H, et al. (2019) Adaptive protection combined with machine learning for microgrids. IET Gener, Transm Distrib 13: 770–779. doi: 10.1049/iet-gtd.2018.6230
![]() |
[69] |
Srivastava A, Tripathi JM, Mohanty SR, et al. (2016) Optimal over-current relay coordination with distributed generation using hybrid particle swarm optimization–gravitational search algorithm.Electric Power Components Systems 44: 506–517. doi: 10.1080/15325008.2015.1117539
![]() |
1. | Muhammad Usama, Hazlie Mokhlis, Mahmoud Moghavvemi, Nurulafiqah Nadzirah Mansor, Majed A. Alotaibi, Munir Azam Muhammad, Abdullah Akram Bajwa, A Comprehensive Review on Protection Strategies to Mitigate the Impact of Renewable Energy Sources on Interconnected Distribution Networks, 2021, 9, 2169-3536, 35740, 10.1109/ACCESS.2021.3061919 | |
2. | Hammad Khalid, Abdulfetah Shobole, Existing Developments in Adaptive Smart Grid Protection: A Review, 2021, 191, 03787796, 106901, 10.1016/j.epsr.2020.106901 | |
3. | Jalal Sahebkar Farkhani, Mohammad Zareein, Arsalan Najafi, Rui Melicio, Eduardo M. G. Rodrigues, The Power System and Microgrid Protection—A Review, 2020, 10, 2076-3417, 8271, 10.3390/app10228271 | |
4. | M.K Perera, R.U.I Disanayaka, E.M.C.S Kumara, W.M.C.S.B Walisundara, H.V.V Priyadarshana, E.M.A.G.N.C Ekanayake, K.T.M.U Hemapala, 2020, Multi Agent Based Energy Management System for Microgrids, 978-1-7281-6664-3, 1, 10.1109/PIICON49524.2020.9113021 | |
5. | Daniel Alcala-Gonzalez, Eva Maria García del Toro, María Isabel Más-López, Santiago Pindado, Effect of Distributed Photovoltaic Generation on Short-Circuit Currents and Fault Detection in Distribution Networks: A Practical Case Study, 2021, 11, 2076-3417, 405, 10.3390/app11010405 | |
6. | Thiramuni Sisitha Sameera Senarathna, Kullappu Thantrige Manjula Udayanga Hemapala, Optimized Adaptive Overcurrent Protection Using Hybridized Nature-Inspired Algorithm and Clustering in Microgrids, 2020, 13, 1996-1073, 3324, 10.3390/en13133324 | |
7. | Ernesto Vázquez, Héctor Esponda, Manuel A. Andrade, 2021, Chapter 18, 978-3-030-59749-8, 489, 10.1007/978-3-030-59750-4_18 | |
8. | Swetalina Sarangi, Binod Kumar Sahu, Pravat Kumar Rout, Review of distributed generator integrated AC microgrid protection: issues, strategies, and future trends , 2021, 0363-907X, 10.1002/er.6689 | |
9. | Mostafa Bakkar, Santiago Bogarra, Felipe Córcoles, Ahmed Aboelhassan, Shuo Wang, Javier Iglesias, Artificial Intelligence-Based Protection for Smart Grids, 2022, 15, 1996-1073, 4933, 10.3390/en15134933 | |
10. | Daisy Nkele Molokomme, Adeiza James Onumanyi, Adnan M. Abu-Mahfouz, Edge Intelligence in Smart Grids: A Survey on Architectures, Offloading Models, Cyber Security Measures, and Challenges, 2022, 11, 2224-2708, 47, 10.3390/jsan11030047 | |
11. | Wael Al Hanaineh, Jose Matas, Jorge El Mariachet, Peilin Xie, Mostafa Bakkar, Josep. M. Guerrero, A THD-Based Fault Protection Method Using MSOGI-FLL Grid Voltage Estimator, 2023, 23, 1424-8220, 980, 10.3390/s23020980 | |
12. | Tao Bai, Junkai Song, Research on the control problem of actuator anti-saturation of supercavitating vehicle, 2021, 19, 1551-0018, 394, 10.3934/mbe.2022020 | |
13. | Muhammad Waseem Altaf, Mohammad Taufiqul Arif, Shama Naz Islam, Md. Enamul Haque, Microgrid Protection Challenges and Mitigation Approaches–A Comprehensive Review, 2022, 10, 2169-3536, 38895, 10.1109/ACCESS.2022.3165011 | |
14. | Abdulfetah Abdela Shobole, Mohammed Wadi, Multiagent systems application for the smart grid protection, 2021, 149, 13640321, 111352, 10.1016/j.rser.2021.111352 | |
15. | Anestis Anastasiadis, Ioannis Oikonomou, Dimitrios Barkas, Georgios A. Vokas, Constantinos Psomopoulos, 2022, 2437, 0094-243X, 020067, 10.1063/5.0093304 | |
16. | Muntathir Al Talaq, Mohammad Al-Muhaini, Optimal Coordination of Time Delay Overcurrent Relays for Power Systems with Integrated Renewable Energy Sources, 2022, 15, 1996-1073, 6749, 10.3390/en15186749 | |
17. | Jorge I.D. Cisneros-Saldana, Smrutirekha Samal, Hemkesh Singh, Miroslav Begovic, S. R. Samantaray, 2022, Microgrid Protection with Penetration of DERs - A Comprehensive Review, 978-1-6654-7902-8, 1, 10.1109/TPEC54980.2022.9750716 | |
18. | Shazia Baloch, Mannan Saeed Muhammad, An Intelligent Data Mining-Based Fault Detection and Classification Strategy for Microgrid, 2021, 9, 2169-3536, 22470, 10.1109/ACCESS.2021.3056534 | |
19. | Khalfan Al Kharusi, Abdelsalam El Haffar, Mostefa Mesbah, Fault Detection and Classification in Transmission Lines Connected to Inverter-Based Generators Using Machine Learning, 2022, 15, 1996-1073, 5475, 10.3390/en15155475 | |
20. | Wael Al Hanaineh, Jose Matas, Josep M. Guerrero, A Comparative Study of Smart THD-Based Fault Protection Techniques for Distribution Networks, 2023, 23, 1424-8220, 4874, 10.3390/s23104874 | |
21. | Moein Choobineh, James Stoupis, 2024, An Adaptive Overcurrent Protection Technique for Microgrids, 979-8-3503-7220-5, 1, 10.1109/PECI61370.2024.10525249 | |
22. | Mohammadreza Aminian, Mehdi Jafari Shahbazzadeh, Mahdiyeh Eslami, Distance optimization and directional overcurrent relay coordination using edge-powered biogeography-genetic algorithms, 2024, 13, 2192-113X, 10.1186/s13677-024-00672-2 | |
23. | Regin Bose Kannaian, Belwin Brearley Joseph, Raja Prabu Ramachandran, An Adaptive Centralized Protection and Relay Coordination Algorithm for Microgrid, 2023, 16, 1996-1073, 4820, 10.3390/en16124820 | |
24. | Armando J Taveras-Cruz, Javier Aquino Espinal, Deyslen Mariano-Hernández, David González Polanco, Elvin Arnaldo Jiménez Matos, Fabio Andrade, Iván Jhonson Mateo, Miguel Aybar-Mejía, Carlos R. Baier, Jesús C. Hernandez, 2024, Differential adaptive protection proposal to interconnect isolated microgrid to the primary electric grid: A case study in the Pedernales province, 979-8-3503-6161-2, 78, 10.1109/icSmartGrid61824.2024.10578079 | |
25. | Ahmed N. Sheta, Gabr M. Abdulsalam, Bishoy E. Sedhom, Abdelfattah A. Eladl, Comparative framework for AC-microgrid protection schemes: challenges, solutions, real applications, and future trends, 2023, 8, 2367-2617, 10.1186/s41601-023-00296-9 | |
26. | Eatmad W. Nahas, Hossam A. Abd El-Ghany, Diaa-Eldin A. Mansour, Samir M. Dawoud, Ahmed M. Azmy, 2023, Advanced Protection Scheme based on IoT for Grid-Connected DC Microgrids, 979-8-3503-5846-9, 1, 10.1109/MEPCON58725.2023.10462449 | |
27. | Mostafa Bakkar, Santiago Bogarra, Felipe Córcoles, Javier Iglesias, Wael Al Hanaineh, Multi-Layer Smart Fault Protection for Secure Smart Grids, 2023, 14, 1949-3053, 3125, 10.1109/TSG.2022.3229848 | |
28. | Waleed M. Hamanah, Md Ismail Hossain, Md Shafiullah, M. A. Abido, AC Microgrid Protection Schemes: A Comprehensive Review, 2023, 11, 2169-3536, 76842, 10.1109/ACCESS.2023.3298306 | |
29. | Amandeep Gill, Priyanka Chandani, Murugan R, 2023, Deployment of AI in Micro Grids: A Survey, 979-8-3503-5776-9, 1390, 10.1109/PEEIC59336.2023.10450747 | |
30. | Armando J. Taveras-Cruz, Deyslen Mariano-Hernández, Elvin Jiménez-Matos, Miguel Aybar-Mejia, Patricio A. Mendoza-Araya, Angel Molina-García, Adaptive protection based on multi-agent systems for AC microgrids: A review, 2025, 377, 03062619, 124673, 10.1016/j.apenergy.2024.124673 | |
31. | Deepali Mishra, Preeti Jain, 2022, A Reliable Protection Scheme for Smartgrid Based On Wavelet Transform and Ensemble Classifier, 978-1-6654-6109-2, 1, 10.1109/ASSIC55218.2022.10088302 | |
32. | Amr S. Mohamed, Deepa Kundur, Mohsen Khalaf, A Probabilistic Approach to Adaptive Protection in the Smart Grid, 2024, 2378-962X, 10.1145/3656347 | |
33. | Hussain A. Alhaiz, Ahmed S. Alsafran, Ali H. Almarhoon, Single-Phase Microgrid Power Quality Enhancement Strategies: A Comprehensive Review, 2023, 16, 1996-1073, 5576, 10.3390/en16145576 | |
34. | Hinal Shah, Jaydeep Chakravorty, Nilesh G. Chothani, Protection challenges and mitigation techniques of power grid integrated to renewable energy sources: A review, 2023, 45, 1556-7036, 4195, 10.1080/15567036.2023.2203111 | |
35. | Muntathir Al Talaq, Mohammad Al-Muhaini, 2024, 9780323917803, 81, 10.1016/B978-0-323-91780-3.00004-0 |
Advantages | Disadvantages |
Benefits the environment through the lack of greenhouse gas emission through low/zero-emission generation technologies | Separate control schemes are required to operate within both technical and economical limits |
Through Islanding, microgrids can operate even during utility failures increasing the reliability levels | Use of battery-based energy storage systems can be expensive in both initiation and maintenance |
Can contribute to peak shaving of the grid network by distributed generation during peak hours | Intermittent nature of the renewable energy sources |
Used to electrify remote areas which have difficulties in connecting with the primary grid | Protection Challenges due to the distributed generation |
An ideal solution for the CHP requirements of customers by increased overall energy efficiency | |
The economic advantage of generating own electricity for a lower cost than from the main utility and even by exporting the energy back to the grid |
Protection Scheme | Method | Features |
Adaptive | Relay setting changes according to network state | Online system Requires network upgrades Prior knowledge on configurations Communication requirement Fault calculations for relay settings |
Differential | Comparison of input and output current of a zone | Immune to the current flow direction and magnitude variations Problems due to unbalances and transients Depends on the communication channel |
Current-based | Current symmetrical component | Problems with unsymmetrical loads due to single-phase DGs Depends on the communication |
Voltage-based | Voltage measurements a b c to d-q transformation | HIF cannot be detected Poor accuracy in grid-connected and varying networks Voltage drops can create errors |
Impedance-based (incl. distance protection) | Measured impedance with threshold values | Accuracy affected by harmonics and transients Errors due to fault impedance Not effective with short-range lines |
Differential energy | Time-frequency transformation with threshold | Applicable to most fault types Long computational durations Pre-calculated threshold values |
Multi-agent protection | Hardware and software agents at critical points | Become expensive and complex with larger systems |
Wide-area protection | IED with fault zone matrix | Not validated for comprehensive systems |
Research | Method | Features | Remarks | ||
Fault Type | Communication | Voltage Level | |||
[53] | PMU based adaptive | All | Yes | LV | Zoned Approach, Accurate only for High DG Penetration, Calculation only |
[54] | Numerical Directional Overcurrent with Central control | All | Yes | LV | Offline analysis-based event table, Directional Interlock, Simulation by calculations |
[55] | Fault component extraction (Impedance based) | L-G L-L-L-G | No | LV | Works with IIDG only, not suitable as backup protection, Simulation only |
[58] | IED based Multi-agent | All | Yes | MV | Decentralized communication, only conceptual design |
[59] | Microgrid CPU with WiMAX | - | Yes | LV | Focused on communication latency, Centralized |
[60] | Directional & Distance adaptive | L-L-L | Yes | MV/LV | Changing the protection method between directional and distance |
[61] | PMU based adaptive distance | All | Yes | MV | Event table-based, Tested on an actual system |
[62] | EC-DER embedded protection | All (Simulated for L-L-G) | Yes | LV | Only for low fault impedances, Auto recloser function, Simulation only |
Advantages | Disadvantages |
Benefits the environment through the lack of greenhouse gas emission through low/zero-emission generation technologies | Separate control schemes are required to operate within both technical and economical limits |
Through Islanding, microgrids can operate even during utility failures increasing the reliability levels | Use of battery-based energy storage systems can be expensive in both initiation and maintenance |
Can contribute to peak shaving of the grid network by distributed generation during peak hours | Intermittent nature of the renewable energy sources |
Used to electrify remote areas which have difficulties in connecting with the primary grid | Protection Challenges due to the distributed generation |
An ideal solution for the CHP requirements of customers by increased overall energy efficiency | |
The economic advantage of generating own electricity for a lower cost than from the main utility and even by exporting the energy back to the grid |
Protection Scheme | Method | Features |
Adaptive | Relay setting changes according to network state | Online system Requires network upgrades Prior knowledge on configurations Communication requirement Fault calculations for relay settings |
Differential | Comparison of input and output current of a zone | Immune to the current flow direction and magnitude variations Problems due to unbalances and transients Depends on the communication channel |
Current-based | Current symmetrical component | Problems with unsymmetrical loads due to single-phase DGs Depends on the communication |
Voltage-based | Voltage measurements a b c to d-q transformation | HIF cannot be detected Poor accuracy in grid-connected and varying networks Voltage drops can create errors |
Impedance-based (incl. distance protection) | Measured impedance with threshold values | Accuracy affected by harmonics and transients Errors due to fault impedance Not effective with short-range lines |
Differential energy | Time-frequency transformation with threshold | Applicable to most fault types Long computational durations Pre-calculated threshold values |
Multi-agent protection | Hardware and software agents at critical points | Become expensive and complex with larger systems |
Wide-area protection | IED with fault zone matrix | Not validated for comprehensive systems |
Research | Method | Features | Remarks | ||
Fault Type | Communication | Voltage Level | |||
[53] | PMU based adaptive | All | Yes | LV | Zoned Approach, Accurate only for High DG Penetration, Calculation only |
[54] | Numerical Directional Overcurrent with Central control | All | Yes | LV | Offline analysis-based event table, Directional Interlock, Simulation by calculations |
[55] | Fault component extraction (Impedance based) | L-G L-L-L-G | No | LV | Works with IIDG only, not suitable as backup protection, Simulation only |
[58] | IED based Multi-agent | All | Yes | MV | Decentralized communication, only conceptual design |
[59] | Microgrid CPU with WiMAX | - | Yes | LV | Focused on communication latency, Centralized |
[60] | Directional & Distance adaptive | L-L-L | Yes | MV/LV | Changing the protection method between directional and distance |
[61] | PMU based adaptive distance | All | Yes | MV | Event table-based, Tested on an actual system |
[62] | EC-DER embedded protection | All (Simulated for L-L-G) | Yes | LV | Only for low fault impedances, Auto recloser function, Simulation only |