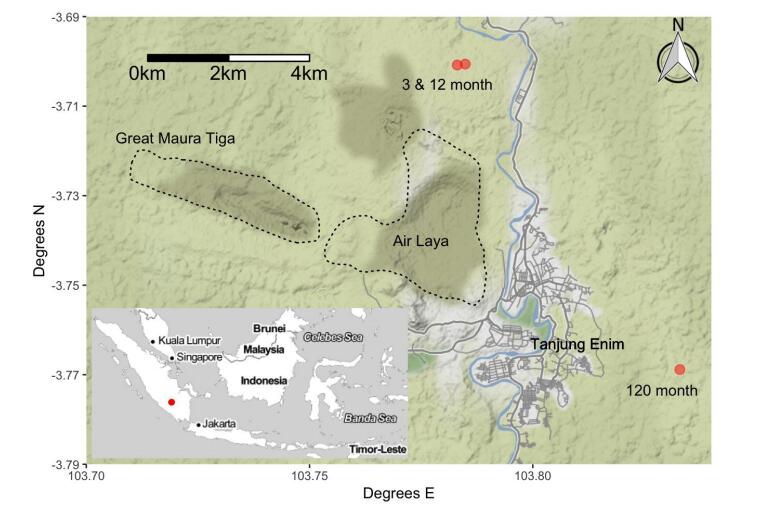
Effector CD8+ cells lyse human immunodeficiency viruses (HIV)-infected CD4+ cells by recognizing a viral peptide presented by human leukocyte antigens (HLA) on the CD4+ cell surface, which plays an irreplaceable role in within-host HIV clearance. Using a semi-saturated lysing efficiency of a CD8+ cell, we discuss a model that captures HIV dynamics with different magnitudes of lysing rate induced by different HLA alleles. With the aid of local stability analysis and bifurcation plots, exponential interactions among CD4+ cells, HIV, and CD8+ cells were investigated. The system exhibited unexpectedly complex behaviors that were both mathematically and biologically interesting, for example monostability, periodic oscillations, and bistability. The CD8+ cell lysing rate, the CD8+ cell count, and the saturation effect were combined to determine the HIV kinetics. For a given CD8+ cell count, a low CD8+ cell lysing rate and a high saturation effect led to monostability to a high viral titre, and a low CD8+ cell lysing rate and a low saturation effect triggered periodic oscillations; this explained why patients with a non-protective HLA allele were always associated with a high viral titer and exhibited bad infection control. On the other hand, a high CD8+ cell lysing rate led to bistability and monostability to a low viral titer; this explained why protective HLA alleles are not always associated with good HIV infection outcomes. These mathematical results explain how differences in HLA alleles determine the variability in viral infection.
Citation: Shilian Xu. Saturated lysing efficiency of CD8+ cells induced monostable, bistable and oscillatory HIV kinetics[J]. Mathematical Biosciences and Engineering, 2024, 21(10): 7373-7393. doi: 10.3934/mbe.2024324
[1] | Lei Wang, Huan Du, Jiajun Wu, Wei Gao, Linna Suo, Dan Wei, Liang Jin, Jianli Ding, Jianzhi Xie, Zhizhuang An . Characteristics of soil erosion in different land-use patterns under natural rainfall. AIMS Environmental Science, 2022, 9(3): 309-324. doi: 10.3934/environsci.2022021 |
[2] | Thierry Woignier, Florence Clostre, Philippe Cattan, Magalie Lesueur-Jannoyer . Pollution of soils and ecosystems by a permanent toxic organochlorine pesticide: chlordecone—numerical simulation of allophane nanoclay microstructure and calculation of its transport properties. AIMS Environmental Science, 2015, 2(3): 494-510. doi: 10.3934/environsci.2015.3.494 |
[3] | Jerry R. Miller, John P. Gannon, Kyle Corcoran . Concentrations, mobility, and potential ecological risks of selected metals within compost amended, reclaimed coal mine soils, tropical South Sumatra, Indonesia. AIMS Environmental Science, 2019, 6(4): 298-325. doi: 10.3934/environsci.2019.4.298 |
[4] | Oltion Marko, Joana Gjipalaj, Dritan Profka, Neritan Shkodrani . Soil erosion estimation using Erosion Potential Method in the Vjosa River Basin, Albania. AIMS Environmental Science, 2023, 10(1): 191-205. doi: 10.3934/environsci.2023011 |
[5] | Lawrence H. Tanner, Megan M. Vandewarker . Significance of vegetation cover differences on albedo and soil carbon on a basaltic sandplain in southern Iceland. AIMS Environmental Science, 2019, 6(6): 435-444. doi: 10.3934/environsci.2019.6.435 |
[6] | Ndakara Ofudjaye Emmanuel, Ofuoko Ukaro Albert . Characterizing plant biomass and soil parameters under exotic trees within rainforest environment in southern Nigeria. AIMS Environmental Science, 2020, 7(6): 611-626. doi: 10.3934/environsci.2020039 |
[7] | Amanda Swearingen, Jessica Price, Janet Silbernagel, Randy Swaty, Nicholas Miller . State-and-transition simulation modeling to compare outcomes of alternative management scenarios under two natural disturbance regimes in a forested landscape in northeastern Wisconsin, USA. AIMS Environmental Science, 2015, 2(3): 737-763. doi: 10.3934/environsci.2015.3.737 |
[8] | Katie Lewis, Jamie Foster, Frank Hons, Thomas Boutton . Initial aggregate formation and soil carbon storage from lipid-extracted algae amendment. AIMS Environmental Science, 2017, 4(6): 743-762. doi: 10.3934/environsci.2017.6.743 |
[9] | Jorge Sierra, Antoine Richard . Modeling the temporal dynamics of chlordecone in the profile of tropical polluted soils as affected by land use change. AIMS Environmental Science, 2021, 8(4): 304-320. doi: 10.3934/environsci.2021020 |
[10] | Gene T. Señeris . Nabaoy River Watershed potential impact to flooding using Geographic Information System remote sensing. AIMS Environmental Science, 2022, 9(3): 381-402. doi: 10.3934/environsci.2022024 |
Effector CD8+ cells lyse human immunodeficiency viruses (HIV)-infected CD4+ cells by recognizing a viral peptide presented by human leukocyte antigens (HLA) on the CD4+ cell surface, which plays an irreplaceable role in within-host HIV clearance. Using a semi-saturated lysing efficiency of a CD8+ cell, we discuss a model that captures HIV dynamics with different magnitudes of lysing rate induced by different HLA alleles. With the aid of local stability analysis and bifurcation plots, exponential interactions among CD4+ cells, HIV, and CD8+ cells were investigated. The system exhibited unexpectedly complex behaviors that were both mathematically and biologically interesting, for example monostability, periodic oscillations, and bistability. The CD8+ cell lysing rate, the CD8+ cell count, and the saturation effect were combined to determine the HIV kinetics. For a given CD8+ cell count, a low CD8+ cell lysing rate and a high saturation effect led to monostability to a high viral titre, and a low CD8+ cell lysing rate and a low saturation effect triggered periodic oscillations; this explained why patients with a non-protective HLA allele were always associated with a high viral titer and exhibited bad infection control. On the other hand, a high CD8+ cell lysing rate led to bistability and monostability to a low viral titer; this explained why protective HLA alleles are not always associated with good HIV infection outcomes. These mathematical results explain how differences in HLA alleles determine the variability in viral infection.
Approximately 40 percent of global electricity is produced by burning coal [1]. Surface or opencast mining, where large parcels of land are disturbed to extract coal, often leads to environmental impacts, including deforestation, decreased biodiversity, degradation of soil health, and decreased water quality [2,3,4,5,6]. An unavoidable consequence of mining, particularly opencast coal mining, is the generation of large volumes of particulate waste. In fact, the annual production of solid mine wastes (from all mine types) is estimated at several thousand million tonnes per year, which is equivalent to the amount of Earth materials moved by global geological processes [7]. A particularly prevalent problem within coal mining areas is the erosion of mine spoils and the transport of metal contaminated sediments to adjacent streams and rivers. Coal gangue (the geological units which contain or are immediately adjacent to the coal) and overburden often contain significant quantities of sulfide minerals, such as pyrite. Once exposed to oxygenated waters, the oxidation of these sulfides can produce acid mine drainage (AMD) [8] during which metal cations and hydronium ions (H+) are released to the environment [8,9]. A potential effect of sulfide oxidation is the lowering of pH of both surface and soil waters, and an increase in both metal concentrations and solubility. When combined with the impacts of lowered pH, the mobile metals often degrade local surface and groundwater quality, and may inhibit plant growth on the mine spoils [9,10]. Even where pits are backfilled with materials from adjacent pits, small depressions and pit lakes may be filled with rainwater and runoff, leading to the long-term (century to millennial scale) generation of acid mine waters. Thus, if improperly managed these mine wastes may continue to degrade local water resources well after mining has ceased.
The environmental threats posed by both active and historic coal mining operations has led to the implementation of widespread reclamation programs at opencast mine sites. The nature of these programs varies widely, but they can be subdivided into two broad categories. In areas of historic opencast mining, the landscape is often characterized by piles of mine wastes interspersed with large depressions, many of which are filled with water. These mine wastes tend to be composed of coal gangue, overburden, or a combination of the two. Reclamation in these areas is dominated by the direct afforestation of the mining wastes in an attempt to stabilize the piles, limit the erosion and offsite migration of sediments and pollutants, and improve visual aesthetics [4,11,12]. Previous studies have shown that reclaimed mine spoils developed in these areas contain a wide range of metal concentrations, and can reach toxic levels [13,14,15,16]. The potential threat depends on the type and geochemical composition of the mine spoils (gangue vs overburden), the chemical speciation and bioavailability of the metals, the local soil properties (e.g., texture and organic matter content), and a host of other factors [12,17,18,19].
More recent opencast mining operations rely on more proactive reclamation programs that involve the stockpiling of "topsoils" and overburden, followed by its replacement once mining has ended. In these areas, topsoils serve as the media for afforestation, although they are significantly different from the soils that existed prior to its removal. The metal concentrations in these topsoils is highly variable, but often controlled by the pre-mining concentrations in the soils, the migration of metals from overburden or coal gangue into the soils, or the wet and dry deposition of atmospheric pollutants and mining dust [16,17].
Regardless of the applied methods, there is general agreement that reclamation is a complex and difficult process with clear limits on what can be achieved, particularly with regards to the long-term recovery of the biodiversity of the site [20]. Thus, the development of sound reclamation strategies requires a detailed, multi-disciplinary understanding of the physical, geochemical and biological processes that are associated with the reclamation effort. To date, the overwhelming majority of research on reclamation has focused on humid-temperate climates [21,22,23,24]. It is apparent, however, that reclamation strategies need to be tailored for specific climatic conditions. Key processes that control, for example, soil and water geochemistry (including sorption-desorption, precipitation-dissolution reactions), surface runoff and infiltration (e.g., soil type, temperature, precipitation), hydrology (e.g., discharge volumes and regimes) and ecology (e.g., plant and animal assemblages) differ between climatic regimes. As a result, reclamation techniques need to be designed for a region's physical, chemical, and biological characteristics [25] to most effectively limit acid mine drainage and the off-site migration of pollutants, and to provide for an effective media for species establishment, vegetation growth, and the promotion of microbial processes [26,27,28].
Unfortunately, few studies have quantitatively examined the reclamation of opencast coal mines in tropical areas. This is in spite of the fact that there has been an exponential growth in coal production in tropical areas of southeast Asia since the late 20th century. In Indonesia, for example, coal production has increased by approximately 460% since 2000, reaching 489 Mt in 2013 [29]. Indonesia is now the leading exporter of thermal (steam) coal in the world, accounting for about 20% of the international market [30] and is the fourth largest producer of coal world-wide. Given that it hosts 31 billion Mt of coal reserves, it is unlikely that coal mining will significantly decrease anytime soon. In fact, it is predicted that peak coal output in Indonesia will not be reached until 2026 [30].
Given the recent increases in coal production, and the remaining coal reserves, considerable concern exists with regards to the potential impacts of coal mining activities on terrestrial and aquatic ecosystems as well as potable drinking water resources in southeast Asia in general, and Indonesia in particular (see, for example, [31]). In response to this concern, a suite of international and interdisciplinary investigations was initiated in 2014 to examine key issues associated with acid mine drainage and opencast mine land reclamation at the PT Bukit Asam Tanjung Enim (PTBA-TE) coal mine. The mine is located approximately 165 km west of Palembang in the tropical region of South Sumatra, Indonesia (Figure 1).
Reclamation of post-mine land by PTBA has been carried out in accordance with the provisions of Indonesian Law No. 4 of 2009 and Regulation of Minister No. 18 of 2008, which govern the reclamation and rehabilitation of post-mine lands. Reclamation addresses surface landscaping (e.g., the development of water flow schemes and the application of topsoil), the control of erosion and acid mine drainage, and the revegetation of reclaimed areas [32]. A key provision in the regulations is that the reclaimed land can be handed over by the mine to local communities when the areal coverage of revegetation exceeds more than 80% of the total post-mined land area [33].
In addition to the regulations described above, the Government of Muara Enim Regency has issued additional regulations, referred to as the "reclamation plus" strategy, that apply to the post-mined management of coal mining lands in the area. The intent is for reclamation to not only address the total area of revegetated land, but to design revegetation schemes that will ultimately provide economic value to the local communities in the form of non-woody products (fruits, oils, and latex) as well as recreational opportunities [34]. Reclamation plus also includes the development of an urban forest. The overall objectives, then, are to provide a forest-based recreation area for economic purposes as well as to conserve typical germplasm (species) usually found in the area of Muara Enim Regency [34].
During the past two decades, PT Bukit Asam (PTBA) has reclaimed multiple areas encompassing 100s of hectares of terrain at the Tanjung Enim coal mine in pursuit of the standards detailed above. Reclamation methods (described in more detail below) have varied, but typically involved the backfilling and capping of previously mined areas with "topsoil", followed by the revegetation of the sites. As part of the revegetation strategy, decomposed organic material (compost) derived from oil palms and/or local municipal solid wastes (MSWC) were added as a soil amendment (Figure 2a). Compost increases the organic matter (OM) content of the soil and improves overall soil quality [35,36]. In some cases, dissolved metals can also be sorbed by organic matter in the soil, thereby decreasing metal solubility and mobility (defined here as the transfer of metals between soil horizons and/or ecological compartments). However, depending on the physiochemical environment, the dissolution of organic matter in the soil by infiltrating waters can form dissolved organic carbon (DOC). DOC tends to be geochemically stable, and may bond with hydrophobic metal ions, forming soluble and highly mobile organic complexes [37,38,39,40]. Thus, the addition of compost can either increase or decrease metal mobility. Although metal mobility is governed by a host of factors, including soil texture and composition, metal concentrations and speciation, site hydrology, and compost composition as well as its methods and rates of application may have either a positive or negative influence on the potential contamination of soil waters located both on- and offsite. Studies conducted to date have primarily focused on the potential effects of different types of soil amendments on metal mobility, rather than the methods of application [41].
The primary purpose of this study was to evaluate the potential risks posed by selected major and trace metals contained within reclaimed soils at the PTBA-TE mine. Inherent in this objective is an assessment of the role of compost, and differing compost application methods, on metal mobility. Specific research questions included the following: (1) Are metal concentrations in the reclaimed soils high enough to be of ecological concern? (2) Are metals contained within the reclaimed soils redistributed between soil horizons by infiltrating waters following emplacement? (3) Do concentrations of selected metals within subsurface waters exceed existing drinking water and aquatic life standards? (4) Does applying, and/or the method of applying compost to revegetated sites influence the mobility of metals within compost-amended reclaimed soils?
The PTBA-TE coal mine is located 165 km west of Palembang, Indonesia (3°44'51"S 103°48'2"E) (Figure 1). Climatically, the region is characterized by a humid, tropical regime with semi-stable annual air temperatures [42]. Daily temperatures range from 20 to 30 ℃; annual precipitation ranges from 2820 mm to 3832 mm [42]; average annual precipitation is 3, 085 mm. The climate exhibits distinct dry and wet seasons; rainfall is highest from November to March and driest from May to October. It rains, on average, 181 days per year.
The PTBA-TE mine covers an area of 66, 414 ha. Mining began in 1919, but was re-developed in the 1980s [42]. Production dramatically increased near the turn of the 20th century. The mined strata contain an estimated 6.36 billion tons of coal, 1.59 billion tons of which are thought to be mineable. The capacity of the mine in 2015 was 20 M tons per year.
The coal deposits at PTBA-TE are mid- to late Miocene in age and were deposited in the Tertiary South Sumatra sedimentary basin [42]. The basin was locally affected by igneous intrusions that aided in the formation of semi-anthracite to sub-bituminous rank coal deposits. Three coal seams, two of which are locally separated by claystones (shale) and other fine-grained units, are mined at PTBA-TE. They have a combined thickness of approximately 40 m and are overlain by about 100 m of interbedded shale, siltstone, and sandstone that must be removed to mine the coal. The ratio of waste to coal is approximately 4:1. The waste rocks are dominated by clay/mudstones containing bentonite, siltstones and lesser quantities of sandstone [42,43,44].
This study was carried out at three sites that had been mined and reclaimed over the past 10 years. The selection of sites was based on the ability to collect and analyze backfill soils and soil waters from reclaimed plots of differing age and that had been subjected to different compost application methods (Figures 2 and 3). The reclaimed sites are referred to herein as Agrotourism Tupak (AT), Agroforestry Tupak – Blok 1 (AF), and Banko Barat 3 (BB). The Agrotourism Tupak reclamation area was constructed three months prior to data/sample collection. In addition to serving as a site for this study, the plot was used to examine the relationship between compost application methods, soil health, and rates of plant growth [45]. More specifically, Red Jabon saplings (Anthocephalus macrophyllus) were planted in soils that were amended with compost using three different strategies or treatments. The utilized compost consisted of a mixture of 14-week-old composted fruit from oil palm trees (Elaeis guineensis). In the first treatment, between 3 to 5 km of compost was mixed with soil and used as backfill when planting each individual sapling. The saplings were planted in a hand-dug hole that was approximately 40 cm in diameter and 40 cm deep. For the second treatment, a 5 cm thick layer of compost was spread over the reclaimed soil surface, after saplings were planted. The third method served as a control; saplings were planted in the reclaimed topsoil without any addition of compost.
In contrast to Agrotourism Tupak, soils at Agroforestry Tupak – Blok 1 were amended with a 5 to 10 cm thick layer of 14-week-old compost that was combined with small amounts of municipal solid waste (MSWC) (Figure 2). Agroforestry Tupak – Blok 1 was started in 2016 and vegetated with a mixture of white paperbark (Melaleuca leucadendra), matoa (Pometia pinnata), and pineapple (Ananas comosus), among other species. It was approximately 12-months-old at the time of sample/data collection. The backfill used at both the Agrotourism Tupak and Agroforestry Tupak – Blok 1 sites was reportedly composed of topsoils that had been removed and set aside during the onset of mining.
The Banko Barat 3 site was reclaimed around 2005, vegetated primarily with species of the genus Acacia, and was roughly 120-months-old at the time of data collection. Information pertaining to the nature of the backfill soil and compost that was used were not fully documented. However, it is assumed that the backfill soil consisted of stockpiled topsoil, and that compost was applied as a layer of material over the reclaimed surface.
A total of 15 monitoring wells were installed at the three sites to sample subsurface water. At Agrotourism Tupak, three wells were installed at the control sites, three at the mixed compost/fill sites, and three across the compost layer sites. Each well was installed as close as possible to an individual sapling or tree without disturbing its root system. Three wells were also installed at the Agroforestry Tupak – Blok 1 site (12 months old) and the 120-month-old Banko Barat 3 (BB) site. Wells were installed in boreholes augured to a depth of ~60 cm at a ~45° angle from vertical. Angled installation was used to increase the probability that the well screen intersected vertical soil fractures observed during soil profile characterization. These vertical fractures were likely to enhance the migration of water transporting major and trace metals through the soils by macropore flow [46,47]. Wells were constructed of 38.1 mm schedule 40 PVC pipe that was screened along the bottom 30 cm of the pipe. The screen was constructed by cutting slots partially through the pipe with a hand saw at multiple locations.
The wells were installed in July, during the dry season. At the time of installation, all 15 wells were dry. However, after precipitation events most wells produced water, allowing for the collection of samples. Samples were collected from the wells following approximately five precipitation events, all during the rainy season. Not all precipitation events produced groundwater levels that reached the wells, particularly at the Banko Barat 3 site (where samples were collected during only 1 event). Samples were pulled from wells with a bailer and filtered immediately using a 0.45 μm syringe filter. Filtered samples were frozen and shipped to Aberystwyth University for analysis of major (Al, Fe, Mn) and selected trace metals (Ni, Pb, Zn). The analyses were carried out using an Agilent 7700 Series Inductively Coupled Plasma Mass Spectrometer (ICP-MS; Agilent, Santa Clara, CA).
The reclaimed soils at the study sites were described in several hand-dug pits excavated to a depth of ~60 cm. These descriptions provided information on soil horizonation, color, soil structure, and texture, among other parameters (e.g., the presences and orientation of macropores).
Sediment samples were collected at a depth of ~5 cm below the surface of the upper mineral horizon at each well installation site, and near the bottom of auger holes used for the installation of monitoring wells (vertical depth of ~42 cm below the ground surface). A total of 30 soil samples were collected and shipped to the U.S. where they were analyzed at Western Carolina University (WCU) for selected major (Al, Fe, Mn) and trace metal (Ni, Pb, Zn) concentrations, soil carbon content, and grain size distribution.
The sediment samples were analyzed for selected major and trace metals including (i.e., Al, Fe, Mn, Ni, Pb, and Zn) following a procedure modified from the Nevada Bureau of Mines and Geology in Reno, Nevada [48]. Selection of the elements was based on previously collected geochemical data that demonstrated that these elements exhibited relatively high concentrations in mine pit waters [49]. All 6 elements are commonly elevated in areas plagued by AMD at coal mines [50].
Sediment samples were air dried prior to analysis, after which 0.2 to 0.5 g of sediment was subsampled and placed in a 125 mL, acid washed high density polyethylene bottle. Three (3) mL of aqua regia (1:4 hydrochloric to nitric acid) was added to the bottles; the lids were then placed on the bottles and tightened. The bottles were subsequently placed in a hot water bath at a temperature of 90 to 95 ℃ for one hour before being allowed to cool. After digestion, the sample solution was transferred to a nitric acid-washed 100 mL volumetric flask and brought to volume using ultra-pure water. The samples were then analyzed by means of a Perkin Elmer Optima 4100DV Inductively Coupled Plasma Optical Emission Spectrometer (ICP-OES). Calibration was performed using in-house and National Institute of Standards and Technology (NIST) standards. Reagent blanks and analyte concentrations for the working standards were plotted against blank-subtracted integrated peak areas. A regression line was fitted to the calibration points and the equation of the line was used to quantify unknown sample concentrations. Deviation of standards from the regression line was used to estimate analytical accuracy, and replicate analyses were used to determine analytical precision. Both accuracy and precision of the soil and water analyses were typically within +/- 5%.
The analysis of soil samples for total carbon content was initiated by drying the materials at 100 ℃ for ~24 hours. The soils were then ground to a powder and homogenized using a mortar and pestle. Following homogenization, ~25 mg of powdered sediment was combined with ~20 to 30 mg of tungsten trioxide (WO3). Samples were then analyzed for total carbon content using the Vario EL III Elemental Analyzer (Elementar, Mt. Laurel, NJ) at WCU.
The grain size distribution of the < 2 mm soil samples was measured using a Mastersizer 2000 particle size analyzer (Malvern Instruments, Malvern, UK). Prior to analysis, ~5 g of sediment was combined in a 50 mL beaker with 5 mL of pyrophosphate and ~30 to 40 mL of deionized water. The mixture was stirred and left to sit overnight.
A Kruskal-Wallis analysis was used to determine how plot ages and compost treatment types correlated with (1) major and trace metal concentrations in subsurface water samples, (2) percent carbon in the soil, and (3) percent silt and clay in the soil. Pair-wise correlation analysis was conducted for determining the strength and relationship between metal concentrations analyzed from subsurface water samples. Metal concentration data were not normally distributed; therefore, the Spearman method was used [51] in addition to pair-wise comparisons.
Enrichment factors (EF) were calculated to assess whether selected metals, including Al, Fe, Mn, Ni, Pb, and Zn within the surface and subsurface (~0.5 m depth) soil materials at the three reclaimed sites were enriched in comparison to background values. Enrichment factors have been calculated in different ways, but generally take the form of:
EF=MS/MBG | (1) |
where MS is the concentration of the metal of interest in the sample, and MBG is the concentration of the metal of concern in the background sediments.
While the concept of background is simple, defining background concentrations has proven problematic, and is particularly difficult in mining areas where the catchment is likely to be underlain by mineralized rocks characterized by metal concentrations that may be similar to anthropogenic source(s). Moreover, metal concentrations within background materials can be highly variable, changing as a function of the amount of chemically reactive, fine-grained (<63 μm) sediment within the analyzed soil [52,53], or the lithologic composition of the deposits [54,55,56]. Sand-sized sediments or rock fragments may, for example, possess mafic (e.g., biotite, augite and amphiboles) or sulfide (e.g., pyrite, galena, and sphalerite) minerals that contain the trace metal(s) of interest.
In light of the above, recent studies have argued for the calculation of a local enrichment factor, in which background is determined (1) using the concentration of metals in soils or sediments from the immediate area of analysis that are devoid of anthropogenic inputs, and (2) where MBG in Equation 1 is empirically predicted using a background function, such that:
MBG=f(Mprop) | (2) |
where Mprop represents a property of the soil or sediment, such as fine-grained particle content, organic matter content, or a conservative reference element (e.g., Al, Fe, Ti, etc.) [54,56,57](see; [56], for a more detailed discussion). The most commonly used background function relates grain size to metal concentration, reflecting the commonly observed influence of fine-grained clay minerals, Fe and Mn oxyhydroxides and organic matter on the sorption of hydrophobic contaminants. In order to be applied, a statistically significant relationship is required between metal concentration and some measure of grain size, such as the proportion of < 63 μm particles in the samples.
In the case of the reclaimed soils at PTBA-TE, only Mn exhibited a weak, but statistically significant correlation with the amount of fine (<63 μm) sediment in the samples, inhibiting the use of grain size-metal concentration relationships as a background function (Table 1). An alternative to grain size is the normalization of the metal of interest by a conservative element from an undisturbed sample location. Unfortunately, metal concentration data from local soils that were not impacted by coal mining or other anthropogenic activities were not available at the time of this analysis. Thus, we calculated EFs, using Equation 1, on the basis of (1) global averages in metal concentrations within soils in general, and (2) soils data collected from northern Sumatra (Banda Aceh, Aceh Province). The global soils background data were based on the dataset compiled by Buonicore [58]. The dataset does not include concentrations for Al or Fe. While global or regional average concentrations are commonly used, the approach is plagued by a high, but unquantifiable amount of uncertainty, related in part to differences in the geological units in which the soils are developed. Similarly, while the soils are both 'volcanic" in nature, the unimpacted tsunami soils sampled and analyzed by Chaerun et al. [59] from northern Sumatra are likely to differ lithologically from the reclaimed soils at PTBA-TE. Thus, the results shown herein provide only general insights into the metal content of the reclaimed soils.
Carbon | Silt+clay | Al | Fe | Mn | Ni | Pb | Zn | |
Carbon | 1.00 | |||||||
Silt/clay | 0.08 | 1.00 | ||||||
Al | 0.19 | -0.09 | 1.00 | |||||
Fe | -0.49* | -0.02 | 0.28 | 1.00 | ||||
Mn | 0.42* | 0.39* | 0.23 | 0.23 | 1.00 | |||
Ni | 0.14 | 0.18 | 0.05 | 0.60* | 0.73* | 1.00 | ||
Pb | -0.02 | -0.06 | 0.17 | 0.63* | 0.49* | 0.78* | 1.00 | |
Zn | 0.37 | 0.34 | -0.03 | 0.23 | 0.66* | 0.70* | 0.57* | 1.00 |
To assess the environmental risks posed by metals in the reclaimed soils, Risk Quotients (RQ) were also calculated for the soil samples in the study. As applied here, a RQ was defined as:
RQ=(EECsoil/LOAEC) | (3) |
where EECsoil is the estimated exposure concentration in the reclaimed soils, and LOAEC is the lowest-observed-adverse-effect concentration for the metal of interest. Thus, the RQ is a ratio that expresses metal exposure to a previously defined toxicity end-point concentration. Here, EECsoil was taken as the concentration observed in the reclaimed soil samples from the three study sites. The LOAEC was based on three different approaches. First, it was defined using total critical soil concentrations provided by Kabata-Pendias and Pendias [60]. These concentrations were argued to represent thresholds above which plant toxicity is considered possible and have been used by other investigators to define phytotoxicity, including in relation to coal mine spoils (e.g., [12,61]). Second, the LOAEC was based on contaminant threshold values defined for Swedish and Finnish soils [62]. This threshold represents a concentration above which soils must be further assessed to determine their potential "harmful" effects on the environment. The use of both the total critical soil concentration and the threshold criteria for Swedish and Finnish soils assumes that the proportion of available metals in the reclaimed soils and the reference sites is similar. This assumption cannot be evaluated given the available data, but the standards used to generate the RQ values represent relatively conservative threshold concentrations in comparison to those used in many other developed countries. Third, RQTEC, was defined on the consensus threshold-effect concentrations (TEC) put forth in MacDonald et al. [63]. The TEC represents the concentration at which impacts to aquatic biota may begin to occur. The assumption is that the soils may be transported offsite by wind or water to local aquatic ecosystems, potentially impacting aquatic biota. Adverse effect criteria were not available to calculate RQFindland or RQTEC for As, Fe, and Mn. Similarly, LOAEC data for Al and Fe were not provided in [60], preventing the calculation of RQKKP for these two elements.
With regards to soil waters, risk quotients were calculated on the basis of two criteria: the World Health Organization drinking water standards (RQWHOdw), and the USEPA freshwater chronic aquatic life criteria (RQaquatic). Use of these parameters provide insights into the potential risks for both human and ecosystem health.
When RQs are less than 1, the risks are considered negligible; values greater than one suggest an impact may be possible. It is important to note that RQs greater than 1 are not statistical probabilities; rather the approach (as used here) represents a simple method to evaluate the degree to which the utilized adverse effect guidelines are exceeded.
All three study sites had been reclaimed by backfilling the area with "topsoil" that had previously been extracted from and stockpiled at the mine. The surface was then contoured and revegetated. The existing surface soils at Agrotourism Tupak (3 month) and Agroforestry Tupak – Blok 1 (12 month) sites were similar. As expected of recently emplaced fill, weathering profiles had yet to develop in the surface materials. Rather, it was characterized by a heterogeneous mixture of fine-grained sediments consisting of dark brown clay- to loamy clay-sized material containing small clasts (typically < 2 cm in diameter) that were disseminated throughout (Figure 3). The clasts primarily consisted of small fragments of sandstone, siltstones, and iron nodules. The soil matrix was locally mottled, and broke into coarse blocky ped-like structures. The surfaces of the "peds" were covered by thin, continuous Fe and, to a lesser degree, Mn coatings. Longer vertical fractures that extended from the surface to about 20 cm in depth occurred at multiple locations within described profiles. The surfaces of the fractures were also coated with Fe oxyhydroxides, and where compost had been applied, organic matter (Figure 3c).
Metal sulfide minerals are an especially common component of coal mine seams, coal gangue, and coal mine spoils, and often lead to the generation of AMD [15]. AMD is a significant problem plaguing pit waters at the PTBA-TE mine. Both active and passive AMD treatment processes are being used to improve the quality of pit waters prior to their release to the local drainage system. However, there was no evidence, such as the precipitation of Fe oxyhydroxides on the soil surface or in small channels draining the two sites that AMD had been produced. The lack of AMD is supported by measurements of soil pH; the pH of reclaimed soils at Agrotourism Tupak (3 month in age) and Agroforestry Tupak – Blok 1 (12 months in age) averaged 6.0, well above the mean pH of 4.1 found in soils from the control site [64]. The qualitative microscopic/petrographic examination of the silt- and sand-sized soil fractions also failed to identify sulfide minerals.
The primary difference in the nature of the soils within and between 3 and 12 month-old reclaimed sites was the extent to which partially decomposed organic matter could be seen within the profiles. Where compost had been applied over the surface, a layer of partially decomposed, dark colored organic matter ranging from about 2 to 10 cm in thickness overlaid deeper mineral layers (Figure 3b). The contact between the organic and mineral layer was consistently abrupt. However, pieces of organic matter and partially decomposed plant fragments occurred within the upper 10 cm of the mineral horizon (Figure 3a). At Agrotoursim Tupak, compost was also mixed within the soil matrix adjacent to planted trees as an alternative treatment (Figure 4). At these sites, the mineral layers extended to the ground surface, but fragments of plant debris and organic matter were visible in augured cuttings through the entire 40 cm of the examined soil profile. Organic fragments were not present at control sites at Agrotourism Tupak where compost had not been applied.
The soils at Banko Barat 3 (120 months in age) differed significantly from those at the two younger sites (Figure 3d). Undecomposed plant debris (litter) from dense vegetation that covered the surface extended to a depth of 2–4 cm below the ground surface. Beneath the undecomposed litter, the soil profiles consisted of a single homogeneous, massive layer of reddish brown, sandy clay loam (Figure 3d). Moreover, the profiles lacked visible organic matter. As was the case at the other two sites, evidence of the generation of AMD was absent.
Quantitative particle size analysis revealed only minor differences in the amount of fine-grained (<63 μm; silt plus clay) particles in samples between compost treatments at the Agrotoursim Tupak site, and between the three reclamation sites of differing age (Table 2). However, silt and clay content consistently increased with depth (i.e., between top- and subsoil samples) at all sampling plots (Table 2).
Research plot | Site age (months) | Compost treatment | % Silt+Clay topsoil | % Silt+Clay subsoil |
AT | 3 | Control | 79.8, 81.8 [8.10] | 90.3, 88.6 [5.28] |
AT | 3 | Mixed | 71.9, 77.5 [13.48] | 85.6, 78.6 [13.90] |
AT | 3 | Surface layer | 74.5, 78.5 [7.40] | 83.9, 85.1 [11.27] |
AF | 12 | Surface layer | 69.8, 77.7 [19.40] | 86.9, 88.3 [6.23] |
BB | 120 | Surface layer | 75.8, 71.9 [13.63] | 72.4, 70.9 [4.52] |
The mean and median C content of the reclaimed mineral soils varied systematically within sites and between the three sites (Table 3). At all sites, the surface soils consistently exhibited higher percentages of C than the subsoils; differences in the mean content of the surface and subsurface soils, for example, ranged from about 0.03% at the Agroforestry Tupak sites to 1.78% at the Agrotourism Tupak mixed sites (Table 3). Between sites, the plots at Agrotourism Tupak that were amended with compost exhibited similar C contents, and consistently possessed at least 1% more C than the control sites (Table 3). Soils with the highest mean and median C contents were found at the Agroforestry Tupak site, where compost had been applied at the surface approximately 12 months before sampling; the mean and median C content of these soils was more than 2% higher than those found at the other study plots, and is presumed to reflect the addition of a much thicker lay of compost over the surface of the soils (Figure 3). Soils with the lowest C contents were found at the Banko Barat 3 plots; the average C content was 1.77% C in topsoil, and 0.62% C in subsoils (Table 3). These lower mean values were about half of that measured at the other sites that received compost, and more than 1% lower than those measured at the Agrotourism Tupak control sites. Differences in the median C values were even more pronounced (Table 3).
Research plot |
Site age (M=months) | Compost treatment |
% Carbon topsoil |
% Carbon subsoil |
AT | 3 | Control | 3.11, 2.62 [1.01] | 2.39, 1.95 [0.89] |
AT | 3 | Mixed | 4.23, 4.23 [1.25] | 2.19, 2.45 [1.32] |
AT | 3 | Surface layer | 4.54, 4.27 [1.41] | 1.80, 3.88 [3.64] |
AF | 12 | Surface layer | 6.51, 6.34 [2.74] | 5.11, 6.31 [5.31] |
BB | 120 | Surface layer | 2.04, 1.77 [0.87] | 0.48, 0.62 [0.31] |
The carbon content and field descriptions of the soils at the three sites suggests that the parent material for soil formation – the reclaimed topsoil, was likely similar for the three- and 12-month-old sites (Agroforestry Tupak and Agrotourism Tupak). This inference is based on similarities in C content, clast abundance and composition, color, structure, and texture. Observations for the same metrics differed, however, at the 10-year-old sites (Banko Barat-3). These differences suggest that the topsoil used at the three- and 12-month-old sites had a different source or treatment than that used at the 10-year-old site.
A commonly asked question with regards to reclaimed coal mine soils is whether their metal contents are high enough to pose a significant threat to the environment. Multiple approaches have been used to address this question; one of the most common methods is to compare the concentrations within the reclaimed soil (general the upper soil layers that serve as the medium for plant growth) to reference or background values. Many studies quantify these comparisons through the calculation of various indices, such as an enrichment factor [54,65,66,67], the Geo-accumulation index [68,69,70], or a risk quotient [71,72]. In this study, enrichment factors (EF) and risk quotients (RQ) were calculated to help assess environmental impact at the PTBA-TE mine.
Soils with an EF of 1 are assumed to exhibit a concentration for the metal of interest that is equivalent to that of the background (i.e., no enrichment). Soils with an EF of less than 1 are therefore depleted in the element, and those above 1 are enriched in the element. Enrichment factors based on these data (EFavesoil) averaged between 1.1 to 1.3 for Mn, Pb, and Zn within the reclaimed mine soils; values for Ni were slightly depleted, averaging 0.6 (Table 4). The maximum observed values were calculated for Mn (2.1) and Zn (2.5); Mn EFavesoil values > 2.0 occurred in 27% of the samples; 7% of the reclaimed soil samples exceeded 2 for Zn. The mean EFAceh for Mn, Pb, and Zn based on coastal soils from Aceh Province that were not impacted by the December 26, 2004 tsunami ranged between 1.2 and 1.6; the mean EFAceh values for Al, Fe, and Ni were very low, ranging between 0.1 and 0.6 (Table 4). For comparison, Acevedo-Figueroa et al. [73] suggest that soils with EF values < 3 exhibit only minor enrichment. Given that the calculated EF were based on soils from different regions that likely differ in their lithologic composition, and possessed a wide range of metal concentrations (particularly for Mn and Zn) (Table 5) [74], it appears that metal enrichment in the examined reclaimed soils at the PTBA-TE was negligible.
Soils | Al | Fe | Mn | Ni | Pb | Zn |
EFavesoil | --- | --- | 1.1 (0.1–2.1) | 0.6 (0.01–0.8) | 0.9 (0.6–1.3) | 1.3 (0.4–2.5) |
EFAceh | 0.1 (0.1–0.3) | 0.6 (0.3–1.0) | 1.6 (0.1–3.0) | 0.2 (0.01–0.3) | 1.2 (0.7–1.6) | 1.3 (0.4–2.4) |
RQKPP | --- | --- | 1.6 (0.09–3.0) | 0.1 (0.0–0.2) | 1.2 (0.7–1.6) | 0.3 (0.1–0.6) |
RQTEC | --- | --- | --- | 0.4 (0.0–0.7) | 0.5 (0.3–0.7) | 0.6 (0.2–1.2) |
RQFinland | --- | --- | --- | 0.2 (0.01–0.30) | 0.3 (0.2–0.4) | 0.4 (0.2–0.7) |
Soil waters | Al | Fe | Mn | Ni | Pb | Zn |
RQWHOdw | 0.04 (0.00–0.87) |
0.02 (0.01–0.23) |
3.82 (0.00–29.49) |
0.16 (0.01–1.80) |
0.33 (0.00–10.66) |
0.00 (0.00–0.02) |
RQaquatic | 0.01 (0.00–0.23) |
0.01 (0.00–0.07) |
--- | 0.06 (0.00–0.69) |
1.33 (0.01–42.64) |
0.03 (0.00–0.44) |
An alternative to the use of EFs is to assess the environmental risks posed by metals in the reclaimed soils using a Risk Quotient (RQ). RQs have been widely used in environmental assessments to obtain a first approximation of the potential impacts of pollutants on ecological systems (e.g., [71,72]). Table 4 shows that with the exception of Mn and Pb, the RQs calculated using all three adverse effect concentrations were generally well below 1. About 80% of the RQKKP values for Mn and Pb exceeded 1. The maximum values were 3.0 and 1.6 for Mn and Pb respectively. It is important to recognize, however, that the three LOAECs used here to calculate enrichment factors differ considerably (>100%) (Table 5). These differences are likely to be related, in part, to variations in the bioavailable fraction of the metals within the soils that can be readily accumulated in biota, which in turn depends on metal speciation and the physicochemical characteristics of the soil environment among other factors. Moreover, a range of metal concentrations were given for the LOAECKKP (Table 5); the mid-point of each range was used to calculate RQKKP herein. Had the minimum values been used, the number of samples that exhibited RQKKP values greater than 1 would have been negligible. In light of above, there is no evidence to suggest that the reclaimed soils pose a significant health risk.
Background - guideline | Al (mg/kg) | Fe (mg/kg) | Mn (mg/kg) | Ni (mg/kg) | Pb (mg/kg) | Zn (mg/kg) | |
Upper contin. crust | 41001 | 39001 | 10002 | 471 | 171 | 671 | |
Average soil3 | --- | --- | 560 | 19 | 19 | 60 | |
Aceh Province sumatra4 | 43387 | 34169 | 423 | 50 | 13.7 | 62 | |
Plant effects4 | --- | --- | 300–500 | 10–100 | 30–300 | 100–400 | |
TEC5 | --- | --- | --- | 22.7 | 35.8 | 121 | |
Finland guidelines6 | --- | --- | --- | 50 | 60 | 200 | |
Topsoil plot | Compost treatment | Al (%) | Fe (%) | Mn (mg/kg) | Ni (mg/kg) | Pb (mg/kg) | Zn (mg/kg) |
AT | Control | 0.3540 (0.051) | 2.699 (0.6480) | 741.0 (125.8) | 9.930 (3.130) | 19.53 (0.720) | 93.54 (40.13) |
AT | Mixed | 0.2668 (0.080) | 1.393 (0.2470) | 421.6 (169.9) | 5.770 (2.710) | 12.57 (4.840) | 56.41 (18.06) |
AT | Surface | 0.3630 (0.070) | 2.139 (0.2990) | 696.7 (257.2) | 11.64 (1.570) | 20.49 (2.340) | 86.82 (23.97) |
AF | Surface | 0.9903 (0.275) | 2.047 (0.5323) | 684.5 (206.1) | 8.7 (4.870) | 15.87 (3.210) | 84.45 (12.77) |
BB | Surface | --- | --- | 155.3 | 2.610 (0.370) | 15.35 (0.980) | 41.33 (10.19) |
Subsoil plot | Compost treatment | Al (%) | Fe (%) | Mn (mg/kg) | Ni (mg/kg) | Pb (mg/kg) | Zn (mg/kg) |
AT | Control | 0.321 (0.124) | 2.259 (0.0277) | 748.5 (311.3) | 14.74 (2.110) | 20.87 (5.090) | 81.61 (36.48) |
AT | Mixed | 0.363 (0.046) | 2.138 (0.3044) | 828.5 (204.5) | 10.18 (2.620) | 19.64 (2.370) | 84.74 (10.01) |
AT | Surface | 0.327 (0.097) | 2.220 (0.1906) | 792.2 (38.67) | --- | --- | --- |
AF | Surface | 0.367 (0.177) | 2.091 (0.5392) | 867.0 (204.7) | 6.90 (3.350) | 18.33 (2.850) | 67.95 (14.18) |
BB | Surface | --- | --- | 85.7 (152.3) | 0.58 (6.560) | 18.54 (5.910) | 52.98 (17.36) |
1Average composition of upper continental curst (Rudnick and Gao, 2003); 2Crustal Rocks, Barbalance [85]; 3Buonicore [59], average concentrations found in soils; Chaerun [60], average from un-impacted tsunami soils; 5Kabata-Pendias and Pendias [61], critical level at which metals effect plants, mid-point of concentration range used to calculate RQs; 6Consensus based threshold effect concentration of metals in sediments for aquatic organisms63 |
Metal concentrations within the Agrotourism Tupak and Agroforestry Tupak – Bloc 1 sites varied within a similar range of concentrations (Table 5). These similarities are presumable related to use of geochemically similar topsoil for site reclamation. However, Al concentrations at the Banko Barat 3 site were higher than those observed at the two younger sites, whereas mean concentrations of Mn, Ni, and Zn were lower (Table 5). Field descriptions of the soil profiles showed that the soil parent materials between Banko Barat 3 and the other two sites not only varied in C content, but in color, clast abundance and composition, soil structure, and, to a lesser degree, texture. These differences, including the link between Al concentration and clay mineralogy, suggest that the topsoils used at the Banko Barat 3 site differed from those used approximately 9 years later at the other two sites. Thus, the observed differences in trace metal concentrations are likely to reflect, in part, the geochemical characteristics of the parent materials used for reclamation, a conclusion also reached by Pietrzykowski et al. [17].
In combination, the indices presented above provide little evidence that the reclaimed soils are significantly enriched in the 6 metals examined herein, or present significant ecological threats to either terrestrial or aquatic ecosystems. Previous studies have shown reclaimed mine soils possess highly variable concentrations of trace metals [17], but which can occur at levels that are toxic to biota [13,14]. However, the occurrence of toxic levels of bioavailable trace metals is not always the case. Concentrations often depend on the geochemistry of the parent materials used for reclamation. The highest concentrations are often associated with coal mine gangue and mine spoils, followed by overburden dumps, but even soils formed in these materials may contain metal concentrations that pose only limited threats to the environment [12,15,16,17,75]. Pietrzykowski et al. [17], for example, examined four coal mining areas in Poland and found that trace metal concentrations within reclaimed soils developed on mine spoils possessed "no direct threat" to the environment. Similarly, Tang et al. [75], working in the semi-humid coal mining region near Huainan, China, found that while the concentrations of some trace metals in reclaimed coal gangue soils were elevated, they were below the Environmental Quality Standards for Soils in China. Maiti [12] examined the concentrations and bioavailability of trace metals in reclaimed soils on overburden dumps within the tropical, Central Coalfields of India, and also found that they were below the toxic limits. Thus, the relatively low concentrations of trace metals within the reclaimed area of the PTBA-TE mine is not surprising, particularly given that the parent material was composed of stockpiled topsoils that appear to contain minimal quantities of sulfide minerals.
The total concentration of a toxic trace metal within the soil matrix is not always a good predictor of its ecologic impacts, because those impacts are closely linked to the bioavailability and chemical mobility of the metal within the soils and soil waters. Mobility is dependent on the geochemical behavior of a metal in the environment [15], and controls the transport of metals from soils to other environmental compartments (e.g., water, sediments, and biota)[14]. Previous studies of the interactions between water and mine tailings have found that total metal concentrations within the tailings materials decreases through time as the tailings weather [76,77,78]. The decline in concentration is presumably related to the migration of metals out of the tailings materials. Dang et al. [15] showed, however, that the trace metal contents of fresh and weathered coal mine spoils in south Wales (UK), were similar, indicating that the mobility of metals released during the weathering process was negligible. The differences in metal mobility observed between these study sites is not only dependent on the total concentration of the metals within the soil, but the mineralogy and stability of the host minerals, the physical and chemical characteristics of the soil (e.g., composition, texture, permeability, pH, Eh, etc.) and the chemical form or speciation of the metals in the soil matrix [15]. Metal migration is most prevalent in soil layers that are associated with frequent fluctuations in groundwater levels [79], which promotes the migration of soil waters, the decomposition of organic matter, and alterations in pH, redox, and other physio-chemical parameters. Redox sensitive metals, such as Fe and Mn, are particularly prone to migration (in comparison, for example, to Pb) given that both changes in pH and redox may lead to the dissolution and/or re-precipitation of Mn and Fe oxyhydroxides. The mobility of both Mn and Fe is increased by reducing conditions.
The reclaimed soils within PTBA-TE mining area were initially characterized as a heterogenous mixture of materials derived from the previous stockpiling of topsoil. The soils within the three study plots were subject to significant, but unquantified degrees of particle weathering and fluctuations in groundwater levels. During the dry season, for example, none of the installed wells contained water. However, during the wet season several wells produced water, which allowed for the collection of samples. In addition, weak and discontinuous gleying of the soil material (a common indicator of water table fluctuations) was observed below about 20 cm in depth in some soil pits from the two younger sites.
Hypothetically, metal concentrations within the initial, heterogenous mixture of reclaimed soils would be random, and dependent (as argued above) on the composition of the soil matrix. Through time, weathering, plant growth, and soil development will alter and spatially re-organize the composition of the soil matrix within mine reclaimed areas, leading in many instances to overall improvements in soil quality [80]. Thus, spatial variations, including the development of systematic spatial trends in metal concentrations, often reflect both metal mobility and source [81]. Figure 5 compares the concentrations of the 6 metals analyzed from the reclaimed sites at the PTBA-TE mine, and shows that there were no systematic trends in metal concentrations between surface and subsurface soil layers at any of the three sites. Rather, at some sampling locations (plots), concentrations were higher at the surface, and in others, concentrations were lower. The potential exception is Fe. Fe concentrations in the control plots (those that did not receive compost) at the Agrotourism Tupak site were consistently lower in the subsurface soils than the surface soils.
The lack of a well-defined trend in concentrations as a function of depth suggests that the downward transfer of metals through the soil matrix was limited. However, the lack of observed variations may also be related to the relatively coarse nature of the applied sampling scheme. Unfortunately, a more detailed analysis of metal mobility, and the factors that control mobility (e.g., soil and soil water pH, Eh, and mineralogical composition), cannot be conducted at the current time given the available data. Nonetheless, there are several lines of evidence that suggest mobility has been limited. First, the spatial extent of metal migration is controlled, in part, by the hydraulic properties of the soil matrix. It is more prevalent in well-sorted, coarse-grained, and permeable soils, especially sand layers [82]. The fine-grained nature of the reclaimed soils at all three of the study sites at the PTBA-TE mine are characterized by fine-grained particles with a low intrinsic permeability (on the order of 10-6–10-1 cm/s). The hydraulically 'tight', finer grain sediments are also characterized a relatively high surface area, which increases the frictional resistance to flow [83] and time available for elemental sorption to the soil matrix.
Second, the concentration of metals in soil waters and their movement through the soil matrix is dependent, in part, on their sorption to particulate matter, particularly clay minerals, organic matter, and Mn and Fe oxyhydroxides, all of which are predominately associated with the fine-grained soil fraction. Given the fine-grained nature of the reclaimed soils at the PTBA-TE mine (Table 2), the sorption potential of the soil matrix is likely to be exceptionally high, thereby limiting metal migration. Interestingly, only Mn concentrations positively correlated with the amount of fine-grained (<63 μm) material in the samples (Table 1). This lack of correlation is not clearly understood, but may reflect the abundance of silt- and clay-sized sediments in the samples, and the relatively minor variations (within a range of about 15–20%) of fine sediment in the parent materials. Alternatively, statistically significant relationships between Mn, Ni, Pb, and Zn suggest that the latter three trace metals may be bound to Mn oxyhydroxides (which are effective trace metal scavengers) [84]. Mn oxyhydroxides were observed to coat ped-like fracture surfaces and mineral grains of all sizes within the soils. Such coatings would degrade statistical metal-particle size relationships. All four elements were also weakly correlated to the total C content of the soils, suggesting that a portion of the metals may also be bound to organic matter. Distinct differences, however, in the concentration of these metals between control and compost treated plots at the Agrotourism Tupak site were lacking (Table 3), indicating that organic matter derived from the compost was unlikely to play a highly significant role in controlling overall metal migration.
The third factor that was likely to limit metal mobility is a lack of significant AMD within the reclaimed study areas. Metal mobility in coal mine spoils is often linked to sulfide mineral oxidation, soil water pH, and the formation of Fe oxyhydroxides [85]. Oxidation not only releases metal cations, but reduces surface and soil water pH, thereby fostering metal solubility and migration. The pH values in recently reclaimed soils (those at the Agrotourism and Agroforestry Tupak-Blok 1 sites) that received compost were in the range of 6, suggesting that the solubility of Mn and Fe as well as the other metal cations, should be relatively low. However, the pH in the soils from the control sites and older (10- to 18-year old) reclaimed areas declined to approximately 4–4.5 [64], potentially leading to an increase in metal solubility and mobility through time. These lower values of pH are typical of soils in the area as a result of its tropical climatic conditions.
Dissolved metals are readily available for bioaccumulation and possess a relatively high potential to reach surface- or groundwater bodies, thereby contaminating both potable and ecological water resources. Thus, an alternative approach to the assessment of both metal mobility and bioavailability is the analysis of dissolved metal concentrations in subsurface soil waters.
Dissolved metal concentrations were determined for soil waters collected from the three PTBA-TE study sites following five precipitation events that occurred during the rainy season (Figure 6). Dissolved concentrations for all 6 metals were highly variable and were not directly correlated to the concentrations measured in the soil matrix (Figure 7). Thus, factors in addition to total soil concentration appear to have controlled their dissolved concentrations in the soil water.
Spatially, Figure 6 shows that there were no statistically significant differences (P < 0.05) in dissolved Mn or Zn concentrations between the compost treatments at the Agrotourism Tupak site, or between the three sites of differing age. The inability to distinguish differences between compost treated and untreated sites suggests that DOC did not significantly influence Mn or Zn concentrations in the reclaimed soil waters. In contrast, the control plots at Agrotourism Tupak exhibited the lowest concentrations of Al, Fe, and Pb. With regards to Pb, dissolved concentrations were consistently below detection at the control plots. Lead concentrations were higher at plots treated with a layer of compost, particularly plots at Agroforestry Tupak -Bloc 1 and Banko Barat 3. However, differences in concentration were relatively minor. In addition, the maximum concentrations of all 6 elements were measured at the Agroforestry Tupak – Bloc 1 sites, the soils of which also possessed the highest C contents. These data suggest that the dissolution of organic matter and the formation of DOC may have increased the solubility of Al, Fe, and Pb within the reclaimed soils. Interestingly, the dissolved concentrations of Ni within soil waters from the control plots at Agrotourism Tupak were higher than the compost treated plots at the site. However, they were lower than those measured at the Agroforestry Tupak – Bloc 1 site. The observed differences in Ni concentrations between compost treatments is harder to explain, but the higher concentrations at Agroforestry Tupak-Bloc 1 may indicate that more than 3 months are required for the weathering of the compost and the generation of DOC. The relatively high concentrations of Al, Fe, and Pb at Banko Barat 3 may also be associated with the production of DOC (in part from litter which overlies the ground surface), and the observed decreases in soil pH through time, both of which would enhance metal solubility. Unfortunately, we were unable to analyze the water samples for DOC to more directly assess the relationships between dissolved metal and DOC concentrations, and its role in controlling metal mobility.
Regardless of the influence of compost on metal mobility, the metal concentrations measured in soil waters from the three plots were generally low. For illustration, risk quotients were calculated for soil waters on the basis of two criteria: the World Health Organization drinking water standards (RQWHOdw), and the USEPA freshwater chronic aquatic life criteria (RQaquatic). The latter represents a conservative view of contamination as these criteria are typically much lower than those associated with acute aquatic impact guidelines. The RQs for Al, Fe, Ni, and Zn were consistently below 1, indicating limited risk. Approximately 5% and 7% of the 56 total samples analyzed for Pb exhibited RQWHOdw and RQaquatic values that exceeded 1, respectively. All of these samples were collected from the Agroforestry Tupak – Blok 1 site, where compost had been added as a surface layer. Values of RQWHOdw greater than 1 were most frequently observed for Mn. At the Agrotourism Tupak site, high Mn RQ values were event specific. During one event, 93% of the RQWHOdw values for Mn were greater than 1; whereas 25% were greater than 1 during a second event. None of the samples collected during the other three events exhibited values greater than 1. There were no significant differences in RQWHOdw for Mn between three types of compost treatment. At the Agroforestry Tupak – Blok 1 site, about 75% of the collected samples exhibited RQWHOdw values for Mn that were greater than 1. Only three water samples were collected at the Banko Barat 3 site, as several remained dry throughout the study. However, in contrast to the other sites, none of these water samples exceed RQWHOdw values of 1 for Mn.
The data presented above show that with the possible exception of Mn, the dissolved metal concentrations in soil waters were low and posed little ecological risk to the environment. Moreover, while the dissolved Mn concentrations in soil waters at Agrotourism Tupak and Agroforestry Tupak – Blok 1 sites were frequently above the drinking water standard, the level of exceedance was typically limited (the mean RQWHOdw was 3.82).
A primary goal of the mine land reclamation program at the PT Bukit Asam-Tanjung Enim (PTBA-TE) mine, located in South Sumatra, Indonesia is to produce a vegetated surface that protects adjacent water resources from metal pollutants and that limits the fine-grained inputs of sediment associated with soil erosion to local drainage systems. An additional local requirement is the use of vegetation that will be of economic benefit once the reclaimed areas have been turned over to the local community. During this study, an analysis was undertaken to determine the potential risks posed by selected major and trace metals (Al, Fe, Mn, Ni, Pb, Zn) contained with the topsoils used in the reclamation of three sites. Metal concentrations measured in both the soil matrix and soil waters were low, and calculated enrichment factors and risk quotients suggest that the risks posed by metals in reclaimed soils and soil water were limited.
A question of particular interest was whether organic matter (OM), added as compost to reclaimed soils to improve soil health, influenced metal bioavailability by either scavenging dissolved metals from the subsurface waters, or increasing dissolved metal concentrations in soil waters by producing dissolved organic carbon (DOC) that formed mobile organo-metallic complexes. The analyses were carried out by analyzing the concentrations of metals in the soil matrix and soil waters at sites of differing age and compost treatments. Spatial differences in dissolved soil water concentrations between compost treated and untreated plots suggest that the influence of OM on metal mobility varied as a function of the examined metal. Dissolved Mn and Zn concentrations lacked statistically significant differences (P < 0.05) between control and compost treated sites at the Agrotourism Tupak site, which had been reclaimed approximately 3 months prior to data collection. These similarities in concentrations between plots in which compost was and was not applied suggest that DOC did not significantly influence Mn or Zn concentrations in the soil water. In contrast, control plots at Agrotourism Tupak exhibited the lowest dissolved soil water concentrations of Al, Fe, and Pb. Lead concentrations were particularly low; none of the water samples collected during 5 precipitation events exhibiting detectable concentrations. Higher concentrations of these three elements were measured at the Agroforesty Tupak – Blok 1 site (reclaimed about 12 months prior to data collection). In fact, the maximum concentrations of all of these elements were measured at this site, which was treated with a thick surface layer of compost and possessed the highest soil C contents. These data suggest that the dissolution of OM, and the formation of DOC, may have increased the solubility of Al, Fe, and Pb within the reclaimed soils.
The downward mobility of the metals was examined through the analysis of vertical trends in metal concentrations within the soil matrix. None of the metals exhibited systematic spatial variations in concentration between surface and subsurface soils, suggesting metal migration through the upper 40–60 cm of the soil profile was limited. Metal mobility was likely reduced by multiple factors, including (1) relatively low metal concentrations and a paucity of acid mine generating sulfide minerals in the topsoil used for mine reclamation, and (2) the fine-grained nature of the reclaimed soils, which limited the rate of subsurface water flow and increased the sorption potential of the soil matrix. While differences in metal concentrations within the soil matrix existed between relatively young (<1-year-old) and old (~10-year-old) sites, they appear to reflect differences in the soil utilized for reclamation.
The authors like to thank our collaborates for their assistance in the collection of both field and laboratory data. Special thanks go to Dr. Bill Perkins of Aberystwyth University, UK for analyzing the water samples, and Joseph Brazelton for helping in the collection of soil samples. This investigation could not have been undertaken without the support of PT Bukit Asam and staff at the Tanjung Enim mine, including Amarudin, Adi Arti Ellataria, and Arief Juniarto, who provided value insights into the sites reclamation programs, aided in the installation of monitoring wells, and collected soil waters during the rainy season. Financial support for this study was provided by the Global Innovative Initiative, and the Whitmire Endowment at Western Carolina University. Their support is highly appreciated.
The authors declare no conflicts of interest.
[1] |
L. Bekker, C. Beyrer, N. Mgodi, S. R. Lewin, S. Delany-Moretlwe, B. Taiwo, et al., HIV infection, Nat. Rev. Dis. Primers, 9 (2023), 42. https://doi.org/10.1038/s41572-023-00452-3 doi: 10.1038/s41572-023-00452-3
![]() |
[2] |
S. G. Deeks, J. Overbaugh, A. Phillips, S. Buchbinder, HIV infection, Nat. Rev. Dis. Primers, 1 (2015), 15035. https://doi.org/10.1038/nrdp.2015.35 doi: 10.1038/nrdp.2015.35
![]() |
[3] |
R. T. Gandhi, R. Bedimo, J. F. Hoy, R. J. Landovitz, D. M. Smith, E. F. Eaton, et al., Antiretroviral drugs for treatment and prevention of HIV Infection in Adults: 2022 recommendations of the International Antiviral Society–USA Panel, JAMA, 329 (2023), 63–84. https://doi.org/10.1001/jama.2022.22246 doi: 10.1001/jama.2022.22246
![]() |
[4] | V. Montessori, N. Press, M. Harris, L. Akagi, J. S. G. Montaner, Adverse effects of antiretroviral therapy for HIV infection, CMAJ, 170 (2004), 229–238. |
[5] |
C. A. Dendrou, J. Petersen, J. Rossjohn, L. Fugger, HLA variation and disease, Nat. Rev. Immunol., 18 (2018), 325–339. https://doi.org/10.1038/nri.2017.143 doi: 10.1038/nri.2017.143
![]() |
[6] |
S. Medhasi, N. Chantratita, Human leukocyte antigen (HLA) system: Genetics and association with bacterial and viral infections, J. Immunol. Res., 2022 (2022), 9710376. https://doi.org/10.1155/2022/9710376 doi: 10.1155/2022/9710376
![]() |
[7] |
Y. M. Mosaad, Clinical role of human leukocyte antigen in health and disease, Scand. J. Immunol., 82 (2015), 283–306. https://doi.org/10.1111/sji.12329 doi: 10.1111/sji.12329
![]() |
[8] |
M. Borrell, I. Fernández, F. Etcheverrry, A. Ugarte, M. Plana, L. Leal, et al., High rates of long-term progression in HIV-1-positive elite controllers, J. Int. AIDS Soc., 24 (2021), e25675. https://doi.org/10.1002/jia2.25675 doi: 10.1002/jia2.25675
![]() |
[9] |
B. A. Woldemeskel, A. K. Kwaa, J. N. Blankson, Viral reservoirs in elite controllers of HIV-1 infection: Implications for HIV cure strategies, eBioMedicine, 62 (2020), 103118. https://doi.org/10.1016/j.ebiom.2020.103118 doi: 10.1016/j.ebiom.2020.103118
![]() |
[10] |
D. R. Collins, G. D. Gaiha, B. D. Walker, CD8+ T cells in HIV control, cure and prevention, Nat. Rev. Immunol., 20 (2020), 471–482. https://doi.org/10.1038/s41577-020-0274-9 doi: 10.1038/s41577-020-0274-9
![]() |
[11] |
B. Monel, A. McKeon, P. Lamothe-Molina, P. Jani, J. Boucau, Y. Pacheco, et al., HIV controllers exhibit effective CD8(+) T cell recognition of HIV-1-infected non-activated CD4(+) T cells, Cell Rep., 27 (2019), 142–153. https://doi.org/10.1016/j.celrep.2019.03.016 doi: 10.1016/j.celrep.2019.03.016
![]() |
[12] |
S. A. Migueles, D. Mendozaa, M. G. Zimmermana, K. M. Martinsa, S. A. Toulmina, E. P. Kelly, et al., CD8+ T-cell cytotoxic capacity associated with human immunodeficiency virus-1 control can be mediated through various epitopes and human leukocyte antigen types, eBioMedicine, 2 (2015), 46–58. http://dx.doi.org/10.1016/j.ebiom.2014.12.009 doi: 10.1016/j.ebiom.2014.12.009
![]() |
[13] |
A. R. Hersperger, J. N. Martin, L. Y. Shin, P. M. Sheth, C. M. Kovacs, G. L. Cosma, et al., Increased HIV-specific CD8+ T-cell cytotoxic potential in HIV elite controllers is associated with T-bet expression, Blood, 117 (2011), 3799–3808. https://doi.org/10.1182/blood-2010-12-322727 doi: 10.1182/blood-2010-12-322727
![]() |
[14] | A. L. Hill, Mathematical models of HIV latency, in Current Topics in Microbiology and Immunology, Springer, 417 (2018), 131–156. https://doi.org/10.1007/82_2017_77 |
[15] | S. Xu, Modelling the Interaction of Influenza Virus and its Antibody, Ph.D thesis, Monash University, 2022. |
[16] |
P. Cao, Z. Wang, A. W. Yan, J. McVernon, J. Xu, J. M. Heffernan, et al., On the role of CD8+ T cells in determining recovery time from influenza virus infection, Front. Immunol., 7 (2016), 611. https://doi.org/10.3389/fimmu.2016.00611 doi: 10.3389/fimmu.2016.00611
![]() |
[17] |
S. M. Ciupe, R. M. Ribeiro, P. W. Nelson, A. S. Perelson, Modeling the mechanisms of acute hepatitis B virus infection, J. Theor. Biol., 247 (2007), 23–35. https://doi.org/10.1016/j.jtbi.2007.02.017 doi: 10.1016/j.jtbi.2007.02.017
![]() |
[18] |
A. Amoddeo, A mathematical model and numerical simulation for SARS-CoV-2 dynamics, Sci. Rep., 13 (2023), 4575. https://doi.org/10.1038/s41598-023-31733-2 doi: 10.1038/s41598-023-31733-2
![]() |
[19] |
V. V. Ganusov, D. L. Barber, R. J. De Boer, Killing of targets by CD8+ T cells in the mouse spleen follows the law of mass action, PLOS ONE, 6 (2011), e15959. https://doi.org/10.1371/journal.pone.0015959 doi: 10.1371/journal.pone.0015959
![]() |
[20] |
M. A. Myers, A. P. Smith, L. C. Lane, D. J. Moquin, R. Aogo, S. Woolard, et al., Dynamically linking influenza virus infection kinetics, lung injury, inflammation, and disease severity, eLife, 10 (2021), e68864. https://doi.org/10.7554/eLife.68864 doi: 10.7554/eLife.68864
![]() |
[21] |
S. Xu, Modelling role of protective and nonprotective HLA allele inducing different HIV infection outcomes, Bull. Math. Biol., 86 (2024), 107. https://doi.org/10.1007/s11538-024-01334-9 doi: 10.1007/s11538-024-01334-9
![]() |
[22] |
C. L. Althaus, R. J. De Boer, Implications of CTL-mediated killing of HIV-infected cells during the non-productive stage of infection, PLOS ONE, 6 (2011), e16468. https://doi.org/10.1371/journal.pone.0016468 doi: 10.1371/journal.pone.0016468
![]() |
[23] |
D. Burg, L. Rong, A. U. Neumann, H. Dahari, Mathematical modeling of viral kinetics under immune control during primary HIV-1 infection, J. Theor. Biol., 259 (2009), 751–759. https://doi.org/10.1016/j.jtbi.2009.04.010 doi: 10.1016/j.jtbi.2009.04.010
![]() |
[24] |
R. Malka, B. Wolach, R. Gavrieli, E. Shochat, V. Rom-Kedar, Evidence for bistable bacteria-neutrophil interaction and its clinical implications, J. Clin. Invest., 122 (2012), 3002–3011. https://doi.org/10.1172/JCI59832 doi: 10.1172/JCI59832
![]() |
[25] |
N. Frenkel, R. S. Dover, E. Titon, Y. Shai, V. Rom-Kedar, Bistable bacterial growth dynamics in the presence of antimicrobial agents, Antibiotics, 10 (2021), 87. https://doi.org/10.3390/antibiotics10010087 doi: 10.3390/antibiotics10010087
![]() |
[26] |
S. Xu, J. Yang, C. Yin, X. Zhao, The dominance of bacterial genotypes leads to susceptibility variations under sublethal antibiotic pressure, Future Microbiol., 13 (2018), 165–185. https://doi.org/10.2217/fmb-2017-0070 doi: 10.2217/fmb-2017-0070
![]() |
[27] |
S. Wang, H. Li, F. Xu, Monotonic and nonmonotonic immune responses in viral infection systems, Discrete Contin. Dyn. Syst. B, 27 (2022), 141–165. https://doi.org/10.3934/dcdsb.2021035 doi: 10.3934/dcdsb.2021035
![]() |
[28] |
Q. Song, S. Wang, F. Xu, Robustness and bistability in a cytokine-enhanced viral infection model, Appl. Math. Lett., 158 (2024), 109215. https://doi.org/10.1016/j.aml.2024.109215 doi: 10.1016/j.aml.2024.109215
![]() |
[29] |
S. Wang, T. Wang, F. Xu, L. Rong, Bistability of an HIV model with immune impairment, SIAM J. Appl. Dyn. Syst., 23 (2024), 1108–1132. https://doi.org/10.1137/23M1596004 doi: 10.1137/23M1596004
![]() |
[30] |
R. Luo, M. J. Piovoso, J. Martinez-Picado, R. Zurakowski, HIV model parameter estimates from interruption trial data including drug efficacy and reservoir dynamics, PLOS ONE, 7 (2012), e40198. https://doi.org/10.1371/journal.pone.0040198 doi: 10.1371/journal.pone.0040198
![]() |
[31] |
H. Mohri, A. S. Perelson, K. Tung, R. M. Ribeiro, B. Ramratnam, M. Markowitz, et al., Increased turnover of T lymphocytes in HIV-1 infection and its reduction by antiretroviral therapy, J. Exp. Med., 194 (2001), 1277–1288. https://doi.org/10.1084/jem.194.9.1277 doi: 10.1084/jem.194.9.1277
![]() |
[32] |
G. Doitsh, M. Cavrois, K. G. Lassen, O. Zepeda, Z. Yang, M. L. Santiago, et al., Abortive HIV infection mediates CD4 T cell depletion and inflammation in human lymphoid tissue, Cell, 143 (2010), 789–801. https://doi.org/10.1016/j.cell.2010.11.001 doi: 10.1016/j.cell.2010.11.001
![]() |
[33] |
M. Markowitz, M. Louie, A. Hurley, E. Sun, M. Di Mascio, A. S. Perelson, et al., A novel antiviral intervention results in more accurate assessment of human immunodeficiency virus type 1 replication dynamics and T-cell decay in vivo, J. Virol., 77 (2003), 5037–5038. https://doi.org/10.1128/jvi.77.8.5037-5038.2003 doi: 10.1128/jvi.77.8.5037-5038.2003
![]() |
[34] |
H. Y. Chen, M. Di Mascio, A. S. Perelson, D. D. Ho, L. Zhang, Determination of virus burst size in vivo using a single-cycle SIV in rhesus macaques, PNAS, 104 (2007), 19079–19084. https://doi.org/10.1073/pnas.0707449104 doi: 10.1073/pnas.0707449104
![]() |
[35] | B. Ramratnam, S. Bonhoeffer, J. Binley, A. Hurley, L. Zhang, J. E. Mittler, et al., Rapid production and clearance of HIV-1 and hepatitis C virus assessed by large volume plasma apheresis, Lancet, 354 (1999), 1782–1785. |
[36] |
M. Helleberg, G. Kronborg, H. Ullum, L. P. Ryder, N. Obel, J. Gerstoft, Course and clinical significance of CD8+ T-cell counts in a large cohort of HIV-infected individuals, J. Infect. Dis., 211 (2015), 1726–1734. https://doi.org/10.1093/infdis/jiu669 doi: 10.1093/infdis/jiu669
![]() |
[37] | R. S. Sauls, C. McCausland, B. N. Taylor, Histology, T-Cell Lymphocyte, StatPearls Publishing, 2024. |
[38] | S. Ruan, J. Wei, On the zeros of transcendental functions with applications to stability of delay differential equations with two delays, Dyn. Contin. Discrete and Impulsive Syst. Ser. A, 10 (2003), 863–874. |
1. | Defritus Aldrin Punuf, Junun Sartohadi, M Anggri Setiawan, Community-based management of small reservoirs in an erosion-landslide-drought area in the dry tropical region of Kupang Regency , 2025, 12, 2502-2458, 7337, 10.15243/jdmlm.2025.122.7337 | |
2. | Edwin Maulana, Junun Sartohadi, Muhammad Anggri Setiawan, Landscape design for gully erosion control on the upper slopes of Mount Sumbing, Central Java, Indonesia , 2025, 12, 2502-2458, 7037, 10.15243/jdmlm.2025.122.7037 | |
3. | Edwin Maulana, Junun Sartohadi, Muhammad Anggri Setiawan, Understanding gully erosion development through geomorphological approach, 2025, 18015395, 10.17221/92/2024-SWR |
Carbon | Silt+clay | Al | Fe | Mn | Ni | Pb | Zn | |
Carbon | 1.00 | |||||||
Silt/clay | 0.08 | 1.00 | ||||||
Al | 0.19 | -0.09 | 1.00 | |||||
Fe | -0.49* | -0.02 | 0.28 | 1.00 | ||||
Mn | 0.42* | 0.39* | 0.23 | 0.23 | 1.00 | |||
Ni | 0.14 | 0.18 | 0.05 | 0.60* | 0.73* | 1.00 | ||
Pb | -0.02 | -0.06 | 0.17 | 0.63* | 0.49* | 0.78* | 1.00 | |
Zn | 0.37 | 0.34 | -0.03 | 0.23 | 0.66* | 0.70* | 0.57* | 1.00 |
Research plot | Site age (months) | Compost treatment | % Silt+Clay topsoil | % Silt+Clay subsoil |
AT | 3 | Control | 79.8, 81.8 [8.10] | 90.3, 88.6 [5.28] |
AT | 3 | Mixed | 71.9, 77.5 [13.48] | 85.6, 78.6 [13.90] |
AT | 3 | Surface layer | 74.5, 78.5 [7.40] | 83.9, 85.1 [11.27] |
AF | 12 | Surface layer | 69.8, 77.7 [19.40] | 86.9, 88.3 [6.23] |
BB | 120 | Surface layer | 75.8, 71.9 [13.63] | 72.4, 70.9 [4.52] |
Research plot |
Site age (M=months) | Compost treatment |
% Carbon topsoil |
% Carbon subsoil |
AT | 3 | Control | 3.11, 2.62 [1.01] | 2.39, 1.95 [0.89] |
AT | 3 | Mixed | 4.23, 4.23 [1.25] | 2.19, 2.45 [1.32] |
AT | 3 | Surface layer | 4.54, 4.27 [1.41] | 1.80, 3.88 [3.64] |
AF | 12 | Surface layer | 6.51, 6.34 [2.74] | 5.11, 6.31 [5.31] |
BB | 120 | Surface layer | 2.04, 1.77 [0.87] | 0.48, 0.62 [0.31] |
Soils | Al | Fe | Mn | Ni | Pb | Zn |
EFavesoil | --- | --- | 1.1 (0.1–2.1) | 0.6 (0.01–0.8) | 0.9 (0.6–1.3) | 1.3 (0.4–2.5) |
EFAceh | 0.1 (0.1–0.3) | 0.6 (0.3–1.0) | 1.6 (0.1–3.0) | 0.2 (0.01–0.3) | 1.2 (0.7–1.6) | 1.3 (0.4–2.4) |
RQKPP | --- | --- | 1.6 (0.09–3.0) | 0.1 (0.0–0.2) | 1.2 (0.7–1.6) | 0.3 (0.1–0.6) |
RQTEC | --- | --- | --- | 0.4 (0.0–0.7) | 0.5 (0.3–0.7) | 0.6 (0.2–1.2) |
RQFinland | --- | --- | --- | 0.2 (0.01–0.30) | 0.3 (0.2–0.4) | 0.4 (0.2–0.7) |
Soil waters | Al | Fe | Mn | Ni | Pb | Zn |
RQWHOdw | 0.04 (0.00–0.87) |
0.02 (0.01–0.23) |
3.82 (0.00–29.49) |
0.16 (0.01–1.80) |
0.33 (0.00–10.66) |
0.00 (0.00–0.02) |
RQaquatic | 0.01 (0.00–0.23) |
0.01 (0.00–0.07) |
--- | 0.06 (0.00–0.69) |
1.33 (0.01–42.64) |
0.03 (0.00–0.44) |
Background - guideline | Al (mg/kg) | Fe (mg/kg) | Mn (mg/kg) | Ni (mg/kg) | Pb (mg/kg) | Zn (mg/kg) | |
Upper contin. crust | 41001 | 39001 | 10002 | 471 | 171 | 671 | |
Average soil3 | --- | --- | 560 | 19 | 19 | 60 | |
Aceh Province sumatra4 | 43387 | 34169 | 423 | 50 | 13.7 | 62 | |
Plant effects4 | --- | --- | 300–500 | 10–100 | 30–300 | 100–400 | |
TEC5 | --- | --- | --- | 22.7 | 35.8 | 121 | |
Finland guidelines6 | --- | --- | --- | 50 | 60 | 200 | |
Topsoil plot | Compost treatment | Al (%) | Fe (%) | Mn (mg/kg) | Ni (mg/kg) | Pb (mg/kg) | Zn (mg/kg) |
AT | Control | 0.3540 (0.051) | 2.699 (0.6480) | 741.0 (125.8) | 9.930 (3.130) | 19.53 (0.720) | 93.54 (40.13) |
AT | Mixed | 0.2668 (0.080) | 1.393 (0.2470) | 421.6 (169.9) | 5.770 (2.710) | 12.57 (4.840) | 56.41 (18.06) |
AT | Surface | 0.3630 (0.070) | 2.139 (0.2990) | 696.7 (257.2) | 11.64 (1.570) | 20.49 (2.340) | 86.82 (23.97) |
AF | Surface | 0.9903 (0.275) | 2.047 (0.5323) | 684.5 (206.1) | 8.7 (4.870) | 15.87 (3.210) | 84.45 (12.77) |
BB | Surface | --- | --- | 155.3 | 2.610 (0.370) | 15.35 (0.980) | 41.33 (10.19) |
Subsoil plot | Compost treatment | Al (%) | Fe (%) | Mn (mg/kg) | Ni (mg/kg) | Pb (mg/kg) | Zn (mg/kg) |
AT | Control | 0.321 (0.124) | 2.259 (0.0277) | 748.5 (311.3) | 14.74 (2.110) | 20.87 (5.090) | 81.61 (36.48) |
AT | Mixed | 0.363 (0.046) | 2.138 (0.3044) | 828.5 (204.5) | 10.18 (2.620) | 19.64 (2.370) | 84.74 (10.01) |
AT | Surface | 0.327 (0.097) | 2.220 (0.1906) | 792.2 (38.67) | --- | --- | --- |
AF | Surface | 0.367 (0.177) | 2.091 (0.5392) | 867.0 (204.7) | 6.90 (3.350) | 18.33 (2.850) | 67.95 (14.18) |
BB | Surface | --- | --- | 85.7 (152.3) | 0.58 (6.560) | 18.54 (5.910) | 52.98 (17.36) |
1Average composition of upper continental curst (Rudnick and Gao, 2003); 2Crustal Rocks, Barbalance [85]; 3Buonicore [59], average concentrations found in soils; Chaerun [60], average from un-impacted tsunami soils; 5Kabata-Pendias and Pendias [61], critical level at which metals effect plants, mid-point of concentration range used to calculate RQs; 6Consensus based threshold effect concentration of metals in sediments for aquatic organisms63 |
Carbon | Silt+clay | Al | Fe | Mn | Ni | Pb | Zn | |
Carbon | 1.00 | |||||||
Silt/clay | 0.08 | 1.00 | ||||||
Al | 0.19 | -0.09 | 1.00 | |||||
Fe | -0.49* | -0.02 | 0.28 | 1.00 | ||||
Mn | 0.42* | 0.39* | 0.23 | 0.23 | 1.00 | |||
Ni | 0.14 | 0.18 | 0.05 | 0.60* | 0.73* | 1.00 | ||
Pb | -0.02 | -0.06 | 0.17 | 0.63* | 0.49* | 0.78* | 1.00 | |
Zn | 0.37 | 0.34 | -0.03 | 0.23 | 0.66* | 0.70* | 0.57* | 1.00 |
Research plot | Site age (months) | Compost treatment | % Silt+Clay topsoil | % Silt+Clay subsoil |
AT | 3 | Control | 79.8, 81.8 [8.10] | 90.3, 88.6 [5.28] |
AT | 3 | Mixed | 71.9, 77.5 [13.48] | 85.6, 78.6 [13.90] |
AT | 3 | Surface layer | 74.5, 78.5 [7.40] | 83.9, 85.1 [11.27] |
AF | 12 | Surface layer | 69.8, 77.7 [19.40] | 86.9, 88.3 [6.23] |
BB | 120 | Surface layer | 75.8, 71.9 [13.63] | 72.4, 70.9 [4.52] |
Research plot |
Site age (M=months) | Compost treatment |
% Carbon topsoil |
% Carbon subsoil |
AT | 3 | Control | 3.11, 2.62 [1.01] | 2.39, 1.95 [0.89] |
AT | 3 | Mixed | 4.23, 4.23 [1.25] | 2.19, 2.45 [1.32] |
AT | 3 | Surface layer | 4.54, 4.27 [1.41] | 1.80, 3.88 [3.64] |
AF | 12 | Surface layer | 6.51, 6.34 [2.74] | 5.11, 6.31 [5.31] |
BB | 120 | Surface layer | 2.04, 1.77 [0.87] | 0.48, 0.62 [0.31] |
Soils | Al | Fe | Mn | Ni | Pb | Zn |
EFavesoil | --- | --- | 1.1 (0.1–2.1) | 0.6 (0.01–0.8) | 0.9 (0.6–1.3) | 1.3 (0.4–2.5) |
EFAceh | 0.1 (0.1–0.3) | 0.6 (0.3–1.0) | 1.6 (0.1–3.0) | 0.2 (0.01–0.3) | 1.2 (0.7–1.6) | 1.3 (0.4–2.4) |
RQKPP | --- | --- | 1.6 (0.09–3.0) | 0.1 (0.0–0.2) | 1.2 (0.7–1.6) | 0.3 (0.1–0.6) |
RQTEC | --- | --- | --- | 0.4 (0.0–0.7) | 0.5 (0.3–0.7) | 0.6 (0.2–1.2) |
RQFinland | --- | --- | --- | 0.2 (0.01–0.30) | 0.3 (0.2–0.4) | 0.4 (0.2–0.7) |
Soil waters | Al | Fe | Mn | Ni | Pb | Zn |
RQWHOdw | 0.04 (0.00–0.87) |
0.02 (0.01–0.23) |
3.82 (0.00–29.49) |
0.16 (0.01–1.80) |
0.33 (0.00–10.66) |
0.00 (0.00–0.02) |
RQaquatic | 0.01 (0.00–0.23) |
0.01 (0.00–0.07) |
--- | 0.06 (0.00–0.69) |
1.33 (0.01–42.64) |
0.03 (0.00–0.44) |
Background - guideline | Al (mg/kg) | Fe (mg/kg) | Mn (mg/kg) | Ni (mg/kg) | Pb (mg/kg) | Zn (mg/kg) | |
Upper contin. crust | 41001 | 39001 | 10002 | 471 | 171 | 671 | |
Average soil3 | --- | --- | 560 | 19 | 19 | 60 | |
Aceh Province sumatra4 | 43387 | 34169 | 423 | 50 | 13.7 | 62 | |
Plant effects4 | --- | --- | 300–500 | 10–100 | 30–300 | 100–400 | |
TEC5 | --- | --- | --- | 22.7 | 35.8 | 121 | |
Finland guidelines6 | --- | --- | --- | 50 | 60 | 200 | |
Topsoil plot | Compost treatment | Al (%) | Fe (%) | Mn (mg/kg) | Ni (mg/kg) | Pb (mg/kg) | Zn (mg/kg) |
AT | Control | 0.3540 (0.051) | 2.699 (0.6480) | 741.0 (125.8) | 9.930 (3.130) | 19.53 (0.720) | 93.54 (40.13) |
AT | Mixed | 0.2668 (0.080) | 1.393 (0.2470) | 421.6 (169.9) | 5.770 (2.710) | 12.57 (4.840) | 56.41 (18.06) |
AT | Surface | 0.3630 (0.070) | 2.139 (0.2990) | 696.7 (257.2) | 11.64 (1.570) | 20.49 (2.340) | 86.82 (23.97) |
AF | Surface | 0.9903 (0.275) | 2.047 (0.5323) | 684.5 (206.1) | 8.7 (4.870) | 15.87 (3.210) | 84.45 (12.77) |
BB | Surface | --- | --- | 155.3 | 2.610 (0.370) | 15.35 (0.980) | 41.33 (10.19) |
Subsoil plot | Compost treatment | Al (%) | Fe (%) | Mn (mg/kg) | Ni (mg/kg) | Pb (mg/kg) | Zn (mg/kg) |
AT | Control | 0.321 (0.124) | 2.259 (0.0277) | 748.5 (311.3) | 14.74 (2.110) | 20.87 (5.090) | 81.61 (36.48) |
AT | Mixed | 0.363 (0.046) | 2.138 (0.3044) | 828.5 (204.5) | 10.18 (2.620) | 19.64 (2.370) | 84.74 (10.01) |
AT | Surface | 0.327 (0.097) | 2.220 (0.1906) | 792.2 (38.67) | --- | --- | --- |
AF | Surface | 0.367 (0.177) | 2.091 (0.5392) | 867.0 (204.7) | 6.90 (3.350) | 18.33 (2.850) | 67.95 (14.18) |
BB | Surface | --- | --- | 85.7 (152.3) | 0.58 (6.560) | 18.54 (5.910) | 52.98 (17.36) |
1Average composition of upper continental curst (Rudnick and Gao, 2003); 2Crustal Rocks, Barbalance [85]; 3Buonicore [59], average concentrations found in soils; Chaerun [60], average from un-impacted tsunami soils; 5Kabata-Pendias and Pendias [61], critical level at which metals effect plants, mid-point of concentration range used to calculate RQs; 6Consensus based threshold effect concentration of metals in sediments for aquatic organisms63 |