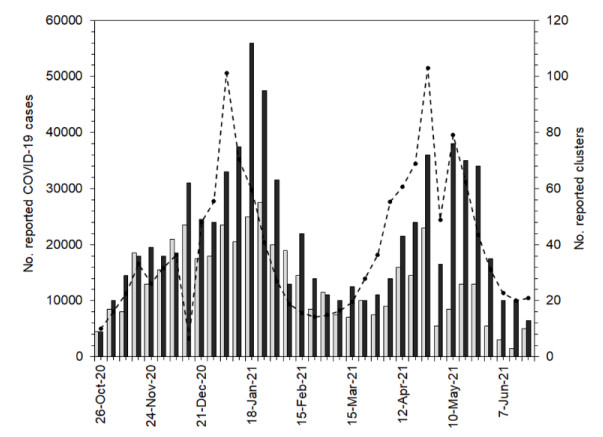
In Japan, a prioritized COVID-19 vaccination program using Pfizer/BioNTech messenger RNA (mRNA) vaccine among healthcare workers commenced on February 17, 2021. As vaccination coverage increases, clusters in healthcare and elderly care facilities including hospitals and nursing homes are expected to be reduced. The present study aimed to explicitly estimate the protective effect of vaccination in reducing cluster incidence in those facilities. A mathematical model was formulated using three pieces of information: (1) the incidence of clusters in facilities from October 26, 2020 to June 27, 2021; (2) the incidence of confirmed COVID-19 cases during the same period; and (3) vaccine doses among healthcare workers from February 17 to June 27, 2021, extracted from the national Vaccination System database. We found that the estimated proportion at risk in healthcare and elderly care facilities declined substantially as the vaccination coverage among healthcare workers increased; the greater risk reduction was observed in healthcare facilities, at 0.10 (95% confidence interval (CI): 0.04–0.16) times that in the pre-vaccination period, while that in elderly care facilities was 0.34 (95% CI: 0.24–0.43) times that in the earlier period. The averted numbers of clusters in healthcare facilities and elderly care facilities were estimated to be 247 (95% CI: 210–301) and 279 (95% CI: 218–354), respectively. Prioritized vaccination among healthcare workers had a marked impact on preventing the incidence of clusters in facilities.
Citation: Misaki Sasanami, Taishi Kayano, Hiroshi Nishiura. The number of COVID-19 clusters in healthcare and elderly care facilities averted by vaccination of healthcare workers in Japan, February–June 2021[J]. Mathematical Biosciences and Engineering, 2022, 19(3): 2762-2773. doi: 10.3934/mbe.2022126
[1] | Yuto Omae, Yohei Kakimoto, Makoto Sasaki, Jun Toyotani, Kazuyuki Hara, Yasuhiro Gon, Hirotaka Takahashi . SIRVVD model-based verification of the effect of first and second doses of COVID-19/SARS-CoV-2 vaccination in Japan. Mathematical Biosciences and Engineering, 2022, 19(1): 1026-1040. doi: 10.3934/mbe.2022047 |
[2] | Yuta Okada, Hiroshi Nishiura . Vaccine-induced reduction of COVID-19 clusters in school settings in Japan during the epidemic wave caused by B.1.1.529 (Omicron) BA.2, 2022. Mathematical Biosciences and Engineering, 2024, 21(9): 7087-7101. doi: 10.3934/mbe.2024312 |
[3] | Pannathon Kreabkhontho, Watchara Teparos, Thitiya Theparod . Potential for eliminating COVID-19 in Thailand through third-dose vaccination: A modeling approach. Mathematical Biosciences and Engineering, 2024, 21(8): 6807-6828. doi: 10.3934/mbe.2024298 |
[4] | Tetsuro Kobayashi, Hiroshi Nishiura . Prioritizing COVID-19 vaccination. Part 2: Real-time comparison between single-dose and double-dose in Japan. Mathematical Biosciences and Engineering, 2022, 19(7): 7410-7424. doi: 10.3934/mbe.2022350 |
[5] | Ayako Suzuki, Hiroshi Nishiura . Transmission dynamics of varicella before, during and after the COVID-19 pandemic in Japan: a modelling study. Mathematical Biosciences and Engineering, 2022, 19(6): 5998-6012. doi: 10.3934/mbe.2022280 |
[6] | Yujie Sheng, Jing-An Cui, Songbai Guo . The modeling and analysis of the COVID-19 pandemic with vaccination and isolation: a case study of Italy. Mathematical Biosciences and Engineering, 2023, 20(3): 5966-5992. doi: 10.3934/mbe.2023258 |
[7] | Beatriz Machado, Liliana Antunes, Constantino Caetano, João F. Pereira, Baltazar Nunes, Paula Patrício, M. Luísa Morgado . The impact of vaccination on the evolution of COVID-19 in Portugal. Mathematical Biosciences and Engineering, 2022, 19(1): 936-952. doi: 10.3934/mbe.2022043 |
[8] | Avinash Shankaranarayanan, Hsiu-Chuan Wei . Mathematical modeling of SARS-nCoV-2 virus in Tamil Nadu, South India. Mathematical Biosciences and Engineering, 2022, 19(11): 11324-11344. doi: 10.3934/mbe.2022527 |
[9] | Sarita Bugalia, Jai Prakash Tripathi, Hao Wang . Estimating the time-dependent effective reproduction number and vaccination rate for COVID-19 in the USA and India. Mathematical Biosciences and Engineering, 2023, 20(3): 4673-4689. doi: 10.3934/mbe.2023216 |
[10] | Fang Wang, Lianying Cao, Xiaoji Song . Mathematical modeling of mutated COVID-19 transmission with quarantine, isolation and vaccination. Mathematical Biosciences and Engineering, 2022, 19(8): 8035-8056. doi: 10.3934/mbe.2022376 |
In Japan, a prioritized COVID-19 vaccination program using Pfizer/BioNTech messenger RNA (mRNA) vaccine among healthcare workers commenced on February 17, 2021. As vaccination coverage increases, clusters in healthcare and elderly care facilities including hospitals and nursing homes are expected to be reduced. The present study aimed to explicitly estimate the protective effect of vaccination in reducing cluster incidence in those facilities. A mathematical model was formulated using three pieces of information: (1) the incidence of clusters in facilities from October 26, 2020 to June 27, 2021; (2) the incidence of confirmed COVID-19 cases during the same period; and (3) vaccine doses among healthcare workers from February 17 to June 27, 2021, extracted from the national Vaccination System database. We found that the estimated proportion at risk in healthcare and elderly care facilities declined substantially as the vaccination coverage among healthcare workers increased; the greater risk reduction was observed in healthcare facilities, at 0.10 (95% confidence interval (CI): 0.04–0.16) times that in the pre-vaccination period, while that in elderly care facilities was 0.34 (95% CI: 0.24–0.43) times that in the earlier period. The averted numbers of clusters in healthcare facilities and elderly care facilities were estimated to be 247 (95% CI: 210–301) and 279 (95% CI: 218–354), respectively. Prioritized vaccination among healthcare workers had a marked impact on preventing the incidence of clusters in facilities.
Severe acute respiratory syndrome coronavirus 2 (SARS-CoV-2) has caused a high number of severe cases and deaths across the world. Frontline healthcare workers, among others, have been an obvious inclusion as a high-risk group, frequently contributing to propagating transmission, because their work requires continued patient-facing roles including unavoidable exposure to infected patients for the purpose of professional care [1]. Before vaccinations became available, many countries tried to protect healthcare workers from infection by procuring personal protective equipment to minimize the risk of nosocomial transmission [1]. Other populations of particular concern have been elderly individuals and people with underlying health conditions, because they are at high risk of having a more serious course of infection [2]. This partly explains why care homes, where typically vulnerable older adults reside, have disproportionately experienced large outbreaks [3,4]. On average, care home residents accounted for more than 45% of deaths related to coronavirus disease (COVID-19) in 21 countries before the advent of vaccination [5].
In December 2020, COVID-19 vaccination commenced globally. Many countries began their vaccine rollouts with healthcare workers, the elderly, and residents of care homes taking first priority [6,7,8]. Among the approved vaccines, the Pfizer/BioNTech messenger RNA (mRNA) vaccine BNT162b2 showed a particularly high efficacy during a clinical trial [9], becoming one of the most widely used vaccines in Israel, the United Kingdom, and the United States, among others [6,7,10]. As countries implemented their vaccine rollouts, the direct effect of vaccination (i.e., protection against infection or disease, conferred by vaccination) was demonstrated to be high among various study populations including older people and healthcare workers [6,7,10,11,12]. Moreover, an indirect effect of vaccination, i.e., reduced risk of infection among unvaccinated individuals due to reduced opportunities for infection, was also suggested by household studies and others [13,14,15,16,17].
As in other countries, Japan also struggled to meet the challenges of mitigating the impact of the COVID-19 pandemic and implementing various public health interventions [18,19]. In Japan, a cluster is defined (in the not-legally binding notification rules of Ministry of Health, Labour and Welfare (MHLW)) as five or more confirmed COVID-19 cases that occur at the same place (excluding households) with clear epidemiologic links [18]. During the initial phase of the epidemic, healthcare facilities (such as hospitals) and care facilities (such as nursing homes) were reported to account for approximately half of the incidence of clusters, with each one sometimes involving more than 100 cases [19]. Considering that healthcare and elderly care facilities act as critical source of fatalities for COVID-19, especially in the super-aged nation Japan, it is vital to prevent the emergence of clusters [20]. Regarding the epidemic situation, as of 7 October 2021, Japan has experienced five major waves of COVID-19 cases [21]. During the fourth wave, from around April to June 2021 [21], the proportion of cases infected with the B.1.1.7 variant increased rapidly from March to April 2021, and this variant was estimated to become the dominant SARS-CoV-2 strain by the middle of May 2021 [22].
Japan's vaccine rollout began on February 17, 2021, and healthcare workers were prioritized as the first group to be vaccinated, as they were considered to be at a higher risk of infection compared to the general population [23]. The vast majority of them received BNT162b2, because it was initially the only available vaccine. Healthcare workers eligible for vaccination were defined as: 1) staff members working for hospitals, clinics, pharmacies, and agencies that provide home-visit nursing services; 2) staff members working for local authorities who are responsible for COVID-19 response; and 3) ambulance crews and personnel of the Japan Coast Guard or Japan Self-Defense Forces [24]. It was also advised that extra doses every day (i.e., residual doses of vaccines at the end of the day) should be distributed to staff and patients at other medical facilities or the general population (prioritizing older adults), especially for people who could receive the vaccine on that day [25,26]. As vaccination coverage increases, the expectation has been that clusters in facilities including healthcare and elderly care facilities, where healthcare workers are working, would be reduced. Figure 1 shows the number of confirmed COVID-19 cases in Japan from the third to the fourth waves, and the reported number of clusters during the pre- and post-vaccination period. Unfortunately, trivial calculations that compare the incidence of infection between vaccinated and unvaccinated do not permit an explicit computation of the risk of clustering in those high-risk facilities. The present study aimed to explicitly estimate the protective effect of vaccination in reducing cluster incidence in those facilities.
We analyzed the weekly data on incidence of clusters from October 26, 2020, to June 27, 2021, which included both pre- and post-vaccination periods. Throughout this article, a cluster is defined as the emergence of five or more confirmed cases in the same facility, regardless of symptoms, diagnosed in a short period of time and considered as linked from each other, which is in common with the governmental definition in Introduction [20]. Although the notification is not legally binding, the occurrence of clusters has been regularly reported by local prefectures and distributed via news media. The media-based cluster data of the abovementioned period were systematically collected across Japan. The dataset of the incidence of confirmed COVID-19 cases for the same period was obtained from the website of MHLW. Confirmatory diagnosis was mostly made by real time polymerase chain reaction (rt-PCR), and all cases were mandated to be notified to the government following implementation of the Infectious Disease Control Law. Distributed vaccine doses among healthcare workers were recorded through the Vaccination System (V-SYS), and the data from February 17 to June 27, 2021, were extracted from the website of Prime Minister's Office of Japan. Original case count data and vaccination data were aggregated weekly.
Here we formulate a mathematical model that is used to estimate the averted risk of clustering that could be attributed to the vaccination. We handled clusters in healthcare and elderly care facilities independently.
We assumed that clusters in each facility occurred proportionally to the incidence of COVID-19 cases, which would be deemed reasonable considering the force of infection [27]; this proportionate relationship is modified by vaccination as follows:
E(nt)={kct−xwithoutvaccination(1−εt)kct−xwithvaccination | (1) |
where nt represents the number of clusters either in healthcare or elderly care facilities in week t, and E(.) represents the expected number. ct−x is the number of confirmed COVID-19 cases in week t−x, i.e., x representing the time lag between COVID-19 cases for the entire population and clusters in facilities. It must be noted that we handle not the number of cases but the number of clusters. Here we specifically considered the time lag x, because a published study suggested that transmission in the facilities is likely to take place at a later part of the local transmission chain that may follow persistent community transmission [19]. k is a scaling parameter that adjusts the proportionality between COVID-19 incidence and clusters. εt is the fraction protected by vaccination, which we consider to be proportional to the protected fraction of the entire population, i.e.,
εt=ω(q1(∑a−1i=0v1,t−iF1,i+∑t−x+ai=a(v1,t−i−v2,t−i+a)F1,i)+q2∑t−xi=0v2,t−iF2,i+a) | (2) |
where ω is a factor of adjustment that converts the fraction protected into the protective effect of vaccination in reducing clusters. v1,t−i and v2,t−i represent the proportion of healthcare workers who received the first and second dose in week t−i (i.e., vaccination coverage of the first and second doses) while i indicates the elapsed number of weeks since the first dose. a represents a fixed number of weeks between doses, recommended to be 3 weeks for BNT162b2 in Japan [28]. q1 and q2 are the maximum effectiveness resulting from the first and second dose of the vaccination. F1,i and F2,i are cumulative distribution functions of building up vaccine-induced immunity following the first and second doses in week i. In the right-hand side of equation (2), the first summation represents immune fraction due to first-dose vaccines in those who are waiting for the second dose due to the time lag of a weeks, the second summation represents the immune fraction of those who received only the first dose, and the third summation is the immune fraction of those who received the second dose.
Because the precise number of frontline healthcare workers in Japan was unknown, we calculated the vaccination coverage among healthcare workers by taking the number of those who had received at least one dose by the last week of our analysis as a denominator, assuming that all frontline healthcare workers would have been vaccinated by then. This assumption was deemed reasonable as the proxy denominator exceeded 4,800,000, which was the estimated number of healthcare workers in the most recent census data [23].
q1, q2, F1,i, and F2,i were derived based on a cohort study conducted in the United Kingdom regarding vaccine effectiveness among healthcare workers who were vaccinated with BNT162b2 when the B.1.1.7 (alpha) variant was the dominant variant in circulation in the country [6]. This published study shared similar epidemiological situations with the present study because the alpha variant was common in Japan from April, and healthcare workers were nearly all fully vaccinated and were exposed at rates similar to the overall population, with an increasing incidence of cases. Accordingly, q1 and q2, i.e., the maximum effectiveness resulting from the first and second doses of the vaccination, were assumed to be 0.71 and 0.86, respectively [6]. F1,i and F2,i were dealt with as logistic functions of weeks since vaccination. These were estimated by fitting logistic models to the datasets from the published cohort study in the United Kingdom [6], and optimal parameter estimates were derived by means of the maximum likelihood method. While we also employed a cumulative Weibull distribution, the logistic function was chosen based on the lowest value of Akaike Information Criterion (AIC). Although the Moderna vaccine, mRNA-1273, was also used beginning May 24, 2021, for healthcare workers and the elderly in Japan, we regarded this impact on our estimate as negligible, because it accounted for only 0.49% (50,989/10,454,415) of the total doses distributed among healthcare workers during the study period.
The parameters k and ω were estimated based on the maximum likelihood method, assuming that the weekly number of clusters follows a Poisson distribution, i.e.,
L(k,ω;D)=∏tE(nt)ntexp(−E(nt))nt! | (3) |
where D represents the observed data. The 95% confidence intervals (CI) were computed via a parametric bootstrapping method using 1,000 samples from a Hessian matrix.
As part of the sensitivity analysis, we examined the impact of varying time lag x on the resulting number of clusters averted. Altering the lag x from 0 to 3, the best fit model for E(nt) was selected according to AIC.
Following the estimation, the numbers of clusters averted in healthcare and elderly care facilities during the study period were then calculated as:
Avertednumberofclusters=∑Tt=1εtkct−x | (4) |
In equation (4), we consider the model without vaccination in equation (1) as a counterfactual scenario. T represents the last week of the study period, and 95% CI for the total averted number of clusters were also computed by a bootstrap method.
From October 26, 2020, to June 27, 2021, a total of 682,702 confirmed COVID-19 cases and 6,775 clusters (i.e., 6,775 groups of cases, each containing five or more confirmed cases) were reported in Japan. Of these clusters, 13.8% (935/6775) and 22.3% (1509/6775) occurred in healthcare and elderly care facilities, respectively. By June 27, 2021, approximately 6 million (5,977,868) of the first doses and 5 million (4,986,483) of the second doses of vaccines were administered among healthcare workers, surpassing the total of 4.8 million persons identified as healthcare workers in the most recent census data.
Figure 2 shows the reported number of clusters in healthcare and elderly care facilities overlaid with the model predictions. In both facilities, the best fit to cluster data was obtained with the lag x = 2 weeks. The results of sensitivity analysis using different x weeks are available in Supplementary Table S1. The scaling factor of the number of clusters, k, and the factor of adjustment from the protected fraction to the population-level protection in the facility, ω, were estimated to be 0.0017 (95% CI: 0.0016–0.0019) and 1.20 (95% CI: 1.13–1.28) for clusters in healthcare facilities, respectively. In elderly care facilities, these estimates were 0.0027 (95% CI: 0.0025–0.0028) and 0.89 (95% CI: 0.76–1.02), respectively. The bootstrap-based uncertainty bounds contained the observed incidence of clusters within the 95% CI.
Figure 3 shows the relative reduction in the number of clusters owing to vaccination, as calculated from (1−εt). The time-dependent proportion of facilities at risk of clustering declined substantially as the vaccination coverage increased. A greater risk reduction was seen in healthcare facilities; e.g., at the end of observation period, the risk was as small as 0.10 (95% CI: 0.044–0.16) times that of the pre-vaccination period, while that in elderly care facilities at the end of the observation period was 0.34 (95% CI: 0.24–0.43) times that of the pre-vaccination period.
The total averted number of clusters in healthcare and elderly care facilities were estimated to be 247 (95% CI: 210–301) and 279 (95% CI: 218–354), respectively, during the study period (Figure 4). As we assumed that the incidence curve of clusters should have been proportional to the incidence of confirmed cases with a fixed delay, the cluster incidence of counterfactual scenario was similar to the epidemic curve of the fourth wave (dashed line in Figure 1), and in the absence of vaccination, the peak height of cluster incidence during the fourth wave could have been similar to that of the third wave.
The present study evaluated the impact of COVID-19 vaccination among healthcare workers on the incidence of clusters in healthcare and elderly care facilities in Japan. We employed a simple approach, imposing assumptions that the incidence of clusters (i.e., the risk of cluster emergence) was proportional to the incidence of confirmed cases, and moreover that the hazard of that proportionality is relatively reduced by the vaccinated immune fraction of the population. We have shown that the numbers of clusters in healthcare and elderly care facilities were reduced to a factor of 0.10 and 0.34 at the end of observation period, and in these facilities, the total of 247 and 279 clusters, respectively, were estimated to have been averted. Without employing the model, one cannot purify this type of total effectiveness (i.e., the sum of direct and indirect effect in reducing the number of clusters) with counterfactual scenarios. The reduced risk of clustering in high-risk facilities by prioritized vaccination among healthcare workers was demonstrated, implying the existence of both direct and indirect impact on clustering.
The most important conclusion of the present study is that vaccination among healthcare professionals yielded a substantial reduction of clusters in healthcare and elderly care facilities. The great reduction in these facilities indicates that not only infections but also a substantial number of severe cases and deaths were avoided, because the susceptible people in the facilities represent a group at high risk for severe illness, involving elderly people and patients with underlying comorbidities [2]. In the present study, the data source relied on an arbitrary definition of cluster that must have involved five or more confirmed COVID-19 cases in Japan [18]. What follows from this definition is that the total number of infections prevented would be even greater than what was estimated here.
The estimated risk reduction reflects both the direct and indirect effectiveness of vaccination. Vaccination averted clusters that could have started from healthcare workers as well as other individuals to whom healthcare professionals could have passed infection. It is worth noting that our model assumed a substantial vaccine effectiveness of BNT162b2 primarily against infection with the B.1.1.7 variant, and the modeled protected fraction well explained the fast decline of clusters during the later stages of the fourth wave. Considering that vaccine effectiveness can differ by variant [29], such a protective effect could also vary accordingly. However, even if the effect is lower, the prioritized vaccination of healthcare workers would be still justified, considering that healthcare professionals play a maintenance role in transmission [1]. In this sense, averting infection in healthcare workers would have an immense impact on preventing facility clusters that involve vulnerable populations at high risk of severe illness and death.
We estimated that there were two weeks of lag between reports of COVID-19 cases and reports of clusters in the facilities. Aside from the aforementioned reason, that is, that clusters probably occur following persistent community transmissions [19], differences in reporting systems may also contribute to this lag. In Japan, confirmed COVID-19 cases must be reported upon diagnosis [18]; however, it is not mandatory to report the incidence of clusters, and this feature could have acted as a source of reporting delay. A cluster has been defined as a series of local transmissions involving five or more confirmed cases, so by the time at least five cases are associated with a facility, their emergence would not result in notification and would therefore go unrecognized.
The present study has several limitations. First, all clusters are not mandated to be notified in Japan, and ascertainment rate may differ according to the epidemic situation (e.g., the reporting rate may be low in the presence of a surge of COVID-19 cases due to overstretched contact tracing capacity). However, the cluster data were collected in a systematic manner across Japan, and the data reliability was improved compared to the early stages of COVID-19 epidemics when efficient and accurate data collection system was still not established. Second, the study did not account for the effectiveness of vaccination among older people. Vaccination among the elderly (aged 65 years and older) began April 12, 2021 in Japan [30]. Although this age group is more likely to be in healthcare and elderly care facilities, there were only 3,104,059 persons out of 35,768,503 (8.7%) of them who were deemed "fully vaccinated", i.e., two weeks following their second vaccination dose, and clearly not all of them were associated with the facilities. We therefore believe that the protective effects brought from the vaccination of the older people did not affect the study results substantially. Third, it should be noted that, by definition by the Japanese government, "healthcare workers" included non-medical staff working in healthcare and elderly care facilities who could contact not only the patients or elderly but also their family members, the food, or fomite, to name a few. Whether or not such non-medical staff should be included in "healthcare workers" and hence in the prioritized group of vaccination might be controversial. However, because they are likely to be part of the transmission chain in these facilities, they were categorized as "healthcare workers". Although the reduced risk attributable to their vaccination might be lower compared to that brought by medical staff, who provide constant patient-facing care, their vaccination should still contribute to prevention of clustering in the facilities. Therefore, we handled them as part of "healthcare workers" in this analysis. Lastly, we assumed that the averted clusters were fully attributable to vaccination; however, there may exist other factors that have contributed to the reduced risk of COVID-19 infection, e.g., seasonal variations in temperature.
Despite the abovementioned limitations, this study presents a novel yet simple approach to evaluating vaccine effectiveness in preventing clusters. This approach could be applied in resource-limited settings. Moreover, the present study endorses the prioritization policy of vaccination among healthcare workers that has been implemented in many countries.
This modeling study quantified the effectiveness of vaccination among healthcare workers in reducing the incidence of clusters in healthcare and elderly care facilities. The estimated impact appears to have been especially substantial because people in such facilities represent those at higher risk of severe illness and death. The present study supports the prioritization of vaccination among healthcare workers that has been implemented in many countries.
H. N. received funding from Health and Labour Sciences Research Grants (19HA1003, 20CA2024, 20HA2007, and 21HB1002); the Japan Agency for Medical Research and Development (AMED; JP20fk0108140 and JP20fk0108535); the Japan Society for the Promotion of Science (JSPS) KAKENHI (17H04701 and 21H03198); the Japan Science and Technology Agency (JST) CREST program (JPMJCR1413); and the SICORP program (JPMJSC20U3 and JPMJSC2105). T.K. received funding from the JSPS KAKENHI (21K10495). The funders played no role in the study design, data collection and analysis, decision to publish, or preparation of the manuscript. We thank John Daniel from Edanz (https://jp.edanz.com/ac) for editing a draft of this manuscript.
All authors declare no conflicts of interest in this paper.
[1] |
L. H. Nguyen, D. A. Drew, M. S. Graham, A. D. Joshi, C. G. Guo, W. Ma, et al., Risk of COVID-19 among front-line health-care workers and the general community: A prospective cohort study, Lancet Public Health, 5 (2020), e475–e483. doi: 10.1016/S2468-2667(20)30164-X. doi: 10.1016/S2468-2667(20)30164-X
![]() |
[2] |
R. E. Jordan, P. Adab, K. K. Cheng, Covid-19: Risk factors for severe disease and death, BMJ, 368 (2020), 1–2. doi: 10.1136/bmj.m1198. doi: 10.1136/bmj.m1198
![]() |
[3] |
T. M. Mcmichael, S. Clark, S. Pogosjans, M. Kay, J. Lewis, A. Baer, COVID-19 in a long-term care facility—King County, Washington, MMWR. Morb. Mortal. Wkly. Rep., 69 (2020), 339–342. doi: 10.15585/mmwr.mm6912e1. doi: 10.15585/mmwr.mm6912e1
![]() |
[4] |
S. N. Ladhani, J. Y. Chow, R. Janarthanan, J. Fok, E. Crawley-Boevey, A. Vusirikala, et al., Investigation of SARS-CoV-2 outbreaks in six care homes in London, April 2020, EClinicalMedicine, 26 (2020), 100533. doi: 10.1016/j.eclinm.2020.100533. doi: 10.1016/j.eclinm.2020.100533
![]() |
[5] | A. Comas-herrera, J. Zalakaín, E. Lemmon, C. Litwin, A. T. Hsu, A. E. Schmidt, et al., Mortality associated with COVID-19 in care homes: International evidence, Int. Long-Term Care Policy Netw., (2020), 1–30. Available from: https://ltccovid.org/wp-content/uploads/2020/10/Mortality-associated-with-COVID-among-people-living-in-care-homes-14-October-2020-4.pdf. |
[6] |
V. J. Hall, S. Foulkes, A. Saei, N. Andrews, B. Oguti, A. Charlett, et al., COVID-19 vaccine coverage in health-care workers in England and effectiveness of BNT162b2 mRNA vaccine against infection (SIREN): A prospective, multicentre, cohort study, Lancet., 397 (2021), 1725–1735. doi: 10.1016/S0140-6736(21)00790-X. doi: 10.1016/S0140-6736(21)00790-X
![]() |
[7] |
M. G. Thompson, J. L. Burgess, A. L. Naleway, H. L. Tyner, S. K. Yoon, J. Meece, et al., Interim estimates of vaccine effectiveness of BNT162b2 and mRNA-1273 COVID-19 vaccines in preventing SARS-CoV-2 infection among health care personnel, first responders, and other essential and frontline workers—Eight U.S. locations, December 2020–March, MMWR. Morb. Mortal. Wkly. Rep., 70 (2021), 495–500. doi: 10.15585/mmwr.mm7013e3. doi: 10.15585/mmwr.mm7013e3
![]() |
[8] |
E. J. Haas, F. J. Angulo, J. M. McLaughlin, E. Anis, S. R. Singer, F. Khan, et al., Impact and effectiveness of mRNA BNT162b2 vaccine against SARS-CoV-2 infections and COVID-19 cases, hospitalisations, and deaths following a nationwide vaccination campaign in Israel: An observational study using national surveillance data, Lancet, 397 (2021), 1819–1829. doi: 10.1016/S0140-6736(21)00947-8. doi: 10.1016/S0140-6736(21)00947-8
![]() |
[9] |
F. P. Polack, S. J. Thomas, N. Kitchin, J. Absalon, A. Gurtman, S. Lockhart, et al., Safety and efficacy of the BNT162b2 mRNA Covid-19 vaccine, N. Engl. J. Med., 383 (2020), 2603–2615. doi: 10.1056/nejmoa2034577. doi: 10.1056/nejmoa2034577
![]() |
[10] |
N. Dagan, N. Barda, E. Kepten, O. Miron, S. Perchik, M. A. Katz, et al., BNT162b2 mRNA Covid-19 vaccine in a nationwide mass vaccination setting, N. Engl. J. Med., 384 (2021), 1412–1423. doi: 10.1056/nejmoa2101765. doi: 10.1056/nejmoa2101765
![]() |
[11] |
J.L. Bernal, N. Andrews, C. Gower, C. Robertson, J. Stowe, E. Tessier, et al., Effectiveness of the Pfizer-BioNTech and Oxford-AstraZeneca vaccines on covid-19 related symptoms, hospital admissions, and mortality in older adults in England: Test negative case-control study, BMJ, 373 (2021). doi: 10.1136/bmj.n1088. doi: 10.1136/bmj.n1088
![]() |
[12] |
A. Britton, K. M. J. Slifka, C. Edens, S. A. Nanduri, S. M. Bart, N. Shang, et al., Effectiveness of the Pfizer-BioNTech COVID-19 vaccine among residents of two skilled nursing facilities experiencing COVID-19 outbreaks—Connecticut, December 2020-February 2021, MMWR Recomm. Reports, 70 (2021), 396–401. doi: 10.15585/mmwr.mm7011e3. doi: 10.15585/mmwr.mm7011e3
![]() |
[13] |
R. J. Harris, J. A. Hall, A. Zaidi, N. J. Andrews, J. K. Dunbar, G. Dabrera, Effect of vaccination on household transmission of SARS-CoV-2 in England, N. Engl. J. Med., 385 (2021), 759–760. doi: 10.1056/nejmc2107717. doi: 10.1056/nejmc2107717
![]() |
[14] | J. Salo, M. Hägg, M. Kortelainen, T. Leino, T. Saxell, M. Siikanen, et al., The indirect effect of mRNA-based Covid-19 vaccination on unvaccinated household members, unpublished work. doi: 10.1101/2021.05.27.21257896. |
[15] |
A. S. V. Shah, C. Gribben, J. Bishop, P. Hanlon, D. Caldwell, R. Wood, et al., Effect of vaccination on transmission of SARS-CoV-2, N. Engl. J. Med., 385 (2021), 1718–1720. doi: 10.1056/NEJMc2106757. doi: 10.1056/NEJMc2106757
![]() |
[16] |
O. Milman, I. Yelin, N. Aharony, R. Katz, E. Herzel, A. Ben-Tov, et al., Community-level evidence for SARS-CoV-2 vaccine protection of unvaccinated individuals, Nat. Med., 27 (2021), 1367–1369. doi: 10.1038/s41591-021-01407-5. doi: 10.1038/s41591-021-01407-5
![]() |
[17] | Public Health England, Direct and indirect impact of the vaccination programme on COVID-19 infections and mortality, 2021. Available from: https://assets.publishing.service.gov.uk/government/uploads/system/uploads/attachment_data/file/997495/Impact_of_COVID-19_vaccine_on_infection_and_mortality.pdf |
[18] | Ministry of Health Labour and Welfare, Infection prevention and healthcare system development. Available from: https://www.mhlw.go.jp/stf/covid-19/kansenkakudaiboushi-iryouteikyou.html. |
[19] |
Y. Furuse, E. Sando, N. Tsuchiya, R. Miyahara, I. Yasuda, Y.K. Ko, et al., Clusters of coronavirus disease in communities, Japan, January–April 2020, Emerg. Infect. Dis., 26 (2020), 13–16. doi: 10.3201/eid2609.202272. doi: 10.3201/eid2609.202272
![]() |
[20] | National Institute of Infectious Diseases, COVID-19 cluster prevention measures, 2020. Available from: https://www.niid.go.jp/niid/ja/typhi-m/iasr-reference/2523-related-articles/related-articles-485/9756-485r03.html. |
[21] | Ministry of Health Labour and Welfare, Visualizing the data: information on COVID-19 infections. Available from: https://covid19.mhlw.go.jp/en/. |
[22] | National Institute of Infectious Diseases, About new concerning variant of SARS-CoV-2 that may have different infectiousness, transmissibility, and antigenicity (9th report), 2021. Available from: https://www.niid.go.jp/niid/ja/2019-ncov/2484-idsc/10434-covid19-43.html. |
[23] | Prime Minister's Office of Japan, About COVID-19 vaccine rollout schedule. Available from: https://www.kantei.go.jp/jp/headline/kansensho/vaccine_supply.html. |
[24] | Ministry of Health Labour and Welfare, About vaccination of healthcare workers. Available from: https://www.mhlw.go.jp/stf/seisakunitsuite/bunya/vaccine_iryoujuujisha.html. |
[25] | Ministry of Health Labour and Welfare, Effective distribution of vaccines for healthcare workers (amended), 2021. Available from: https://www.mhlw.go.jp/content/000786364.pdf. |
[26] | Ministry of Health Labour and Welfare, About questions and answers (Q & A) regarding "Effective distribution of vaccines for healthcare workers (amended)", 2021. Available from: https://www.mhlw.go.jp/content/000793279.pdf. |
[27] |
S. Bagchi, J. Mak, Q. Li, E. Sheriff, E. Mungai, A. Anttila, et al., Rates of COVID-19 among residents and staff members in nursing homes—United States, May 25–November 22, 2020, MMWR. Morb. Mortal. Wkly. Rep., 70 (2021), 52–55. doi: 10.15585/mmwr.mm7002e2. doi: 10.15585/mmwr.mm7002e2
![]() |
[28] | Ministry of Health Labour and Welfare, COVID-19 Vaccines. Available from: https://www.mhlw.go.jp/stf/covid-19/vaccine.html. |
[29] |
A. Sheikh, J. McMenamin, B. Taylor, C. Robertson, SARS-CoV-2 Delta VOC in Scotland: demographics, risk of hospital admission, and vaccine effectiveness, Lancet, 397 (2021), 2461–2462. doi: 10.1016/S0140-6736(21)01358-1. doi: 10.1016/S0140-6736(21)01358-1
![]() |
[30] | Prime Minister's Office of Japan, About COVID-19 vaccination. Available from: https://www.kantei.go.jp/jp/headline/kansensho/vaccine.html. |
1. | Kana Kazawa, Tatsuhiko Kubo, Masahiro Akishita, Shinya Ishii, Geriatric care facilities' concerns regarding hospital admission of infected residents with dementia or transport for admission in the COVID ‐19 pandemic , 2022, 22, 1444-1586, 1050, 10.1111/ggi.14499 | |
2. | Kana Kazawa, Tatsuhiko Kubo, Masahiro Akishita, Shinya Ishii, Experiences with COVID ‐19 cluster infections in geriatric care facilities , 2022, 22, 1444-1586, 537, 10.1111/ggi.14412 | |
3. | Adam L Gordon, Wilco P Achterberg, Johannes J M van Delden, Mandatory vaccination against COVID-19 for health and social care workers caring for older people, 2022, 51, 0002-0729, 10.1093/ageing/afac097 | |
4. | Misaki Sasanami, Marie Fujimoto, Taishi Kayano, Katsuma Hayashi, Hiroshi Nishiura, Projecting the COVID-19 immune landscape in Japan in the presence of waning immunity and booster vaccination, 2023, 559, 00225193, 111384, 10.1016/j.jtbi.2022.111384 | |
5. | Misaki Sasanami, Taishi Kayano, Hiroshi Nishiura, Monitoring the COVID-19 immune landscape in Japan, 2022, 122, 12019712, 300, 10.1016/j.ijid.2022.06.005 | |
6. | Jonas Crèvecoeur, Niel Hens, Thomas Neyens, Ynke Larivière, Bruno Verhasselt, Hanna Masson, Heidi Theeten, Change in COVID19 outbreak pattern following vaccination in long-term care facilities in Flanders, Belgium, 2022, 40, 0264410X, 6218, 10.1016/j.vaccine.2022.09.028 | |
7. | Kai-Ming Chow, Umberto Maggiore, Frank J.M.F. Dor, Ethical Issues in Kidney Transplant and Donation During COVID-19 Pandemic, 2022, 42, 02709295, 151272, 10.1016/j.semnephrol.2022.07.006 | |
8. | Minami Ueda, Katsuma Hayashi, Hiroshi Nishiura, Identifying High-Risk Events for COVID-19 Transmission: Estimating the Risk of Clustering Using Nationwide Data, 2023, 15, 1999-4915, 456, 10.3390/v15020456 | |
9. | Takashi IMANO, Toshihiro KAWAZOE, Kohei MAKITA, A Qualitative Study on the Effect of Coronavirus Disease 2019 on Animal-Assisted Intervention Activities Using Modified Grounded Theory Approach, 2023, 27, 1343-2583, 108, 10.2743/jve.27.108 | |
10. | Li Lv, Xu-Dong Wu, Huan-Jun Yan, Shuang-Ying Zhao, Xiao-Dong Zhang, Ke-Lei Zhu, The disparity in hesitancy toward COVID-19 vaccination between older individuals in nursing homes and those in the community in Taizhou, China, 2023, 23, 1471-2318, 10.1186/s12877-023-04518-5 | |
11. | Li Lv, Xiao-Qing Lin, Yan Chen, Chenqian Ying, Eva Jiang, Mindan Jin, Tao-Hsin Tung, Jiansheng Zhu, The Disparity in Hesitancy Toward COVID-19 Vaccination between Older Adults in Care Homes and Those in the Community in Taizhou, China, 2022, 1556-5068, 10.2139/ssrn.4139623 | |
12. | Moid Sandhu, David Silvera-Tawil, Paulo Borges, Qing Zhang, Brano Kusy, Internet of robotic things for independent living: Critical analysis and future directions, 2024, 25, 25426605, 101120, 10.1016/j.iot.2024.101120 | |
13. | Yuta Okada, Hiroshi Nishiura, Vaccine-induced reduction of COVID-19 clusters in school settings in Japan during the epidemic wave caused by B.1.1.529 (Omicron) BA.2, 2022, 2024, 21, 1551-0018, 7087, 10.3934/mbe.2024312 | |
14. | Junpei Tanabe, Hungu Jung, Yuji Iwamoto, Shinya Ishii, Investigation of the factors contributing to symptoms worsening in elderly care‐facility residents with dementia: Difficulties encountered in dealing with COVID‐19 clusters, 2025, 1444-1586, 10.1111/ggi.15057 |