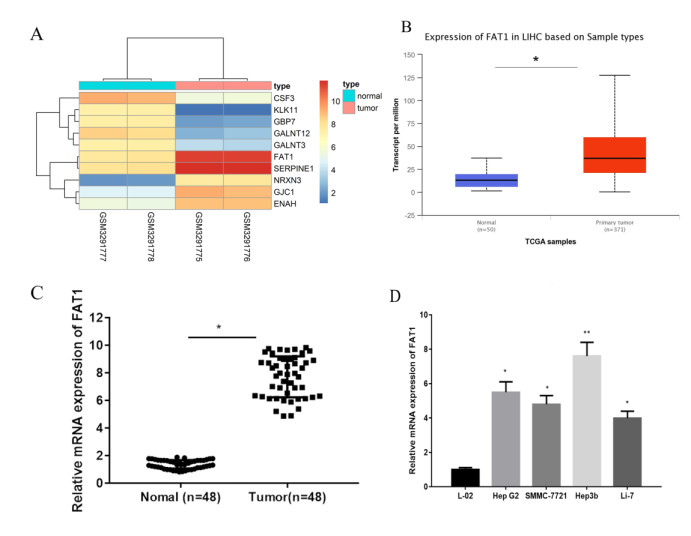
Citation: Jun Xu, Bei Wang, Zhengtao Liu, Mingchun Lai, Mangli Zhang, Shusen Zheng. miR-223-3p regulating the occurrence and development of liver cancer cells by targeting FAT1 gene[J]. Mathematical Biosciences and Engineering, 2020, 17(2): 1534-1547. doi: 10.3934/mbe.2020079
[1] | Li-Bing Wang, Liang Feng, Jing He, Bo Liu, Jian-Guang Sun . MiR-125a-5p inhibits the proliferation and invasion of breast cancer cells and induces apoptosis by targeting GAB2. Mathematical Biosciences and Engineering, 2019, 16(6): 6923-6933. doi: 10.3934/mbe.2019347 |
[2] | Wei Huo, Xiao-Min Zhu, Xin-Yan Pan, Min Du, Zhuo Sun, Zhi-Min Li . MicroRNA-527 inhibits TGF-β/SMAD induced epithelial-mesenchymal transition via downregulating SULF2 expression in non-small-cell lung cancer. Mathematical Biosciences and Engineering, 2019, 16(5): 4607-4621. doi: 10.3934/mbe.2019231 |
[3] | Jie Chen, Jinggui Chen, Bo Sun, Jianghong Wu, Chunyan Du . Integrative analysis of immune microenvironment-related CeRNA regulatory axis in gastric cancer. Mathematical Biosciences and Engineering, 2020, 17(4): 3953-3971. doi: 10.3934/mbe.2020219 |
[4] | Shequn Gu, Jihui Luo, Wenxiu Yao . The regulation of miR-139-5p on the biological characteristics of breast cancer cells by targeting COL11A1. Mathematical Biosciences and Engineering, 2020, 17(2): 1428-1441. doi: 10.3934/mbe.2020073 |
[5] | Guobing Lin, Cunming Zhang, Xuanyu Chen, Junwei Wang, Song Chen, Siyuan Tang, Tianqiang Yu . Identification of circulating miRNAs as novel prognostic biomarkers for bladder cancer. Mathematical Biosciences and Engineering, 2020, 17(1): 834-844. doi: 10.3934/mbe.2020044 |
[6] |
Feng Chen, Xuqing Zhu, Jing Zheng, Tingting Xu, Kuan Wu, Chuhui Ru .
RHAMM regulates the growth and migration of lung adenocarcinoma A549 cell line by regulating Cdc2/CyclinB1 and MMP9 genes . Mathematical Biosciences and Engineering, 2020, 17(3): 2150-2163. doi: 10.3934/mbe.2020114 |
[7] | Dongchen Lu, Wei Han, Kai Lu . Identification of key microRNAs involved in tumorigenesis and prognostic microRNAs in breast cancer. Mathematical Biosciences and Engineering, 2020, 17(4): 2923-2935. doi: 10.3934/mbe.2020164 |
[8] | Guoxin Hou, Binbin Song . Gastric cancer patient with c-MET amplification treated with crizotinib after failed multi-line treatment: A case report and literature review. Mathematical Biosciences and Engineering, 2019, 16(5): 5923-5930. doi: 10.3934/mbe.2019296 |
[9] | Ming-Xi Zhu, Tian-Yang Zhao, Yan Li . Insight into the mechanism of DNA methylation and miRNA-mRNA regulatory network in ischemic stroke. Mathematical Biosciences and Engineering, 2023, 20(6): 10264-10283. doi: 10.3934/mbe.2023450 |
[10] | Xu Guo, Yuanming Jing, Haizhou Lou, Qiaonv Lou . Effect and mechanism of long non-coding RNA ZEB2-AS1 in the occurrence and development of colon cancer. Mathematical Biosciences and Engineering, 2019, 16(6): 8109-8120. doi: 10.3934/mbe.2019408 |
Liver cancer is one of the most common causes of cancer-related deaths worldwide [1]. Its five-year survival rate is significantly lower than that of other types of cancers [2], mainly because most patients with liver cancer are in advanced stage at the time of diagnosis [3]. Therefore, it is necessary to identify new biomarkers for early diagnosis of liver cancer [4].
FAT1 is a member of the cadherin superfamily. The cadherin superfamily is a complete set of membrane protein characterized by the presence of cadherin repeat sequences. In addition to 34 tandem cadherin repeat sequences, the products of FAT1 also contain 5 of epidermal growth factor (EGF) like repeat sequences and 1 of laminin AG domain. The gene is highly expressed in many fetal epithelial cells (literature as reference). Its products may work by acting as adhesion molecules or signal transduction receptors, and may play an important role in its development and cell communication. FAT1 is identified as the first fat-like protein in vertebrates [5]. Mice lacking FAT1 show perinatal lethality and kidney and brain defects [6]. In cancer, FAT1 seems to act as a tumor suppressor or carcinogen [7]. FAT1 is down-regulated in invasive breast cancer [8], and up-regulated in leukemia [9]. Also, it can be used as a regulator of carcinogenic pathway in glioma cell lines [10]. In addition, previous studies have shown that FAT1 is a relevant mediator of hypoxia and also of the key tumorigenesis that from growth receptor signal to hepatic cellular cancer, and may be used as a tumor promoter in highly invasive liver cancer [11]. However, the regulatory mechanism of FAT1 on liver cancer is still not particularly clear.
miRNA is a short non-coding RNA, which negatively regulates gene expression mainly by binding to the 3'-UTR of the target gene. Liver cancer and the tumorigenesis of its related miRNA have become a hotspot for research. Previous studies have shown that miRNA may be a candidate prognostic and diagnostic biomarker for liver cancer [12]. In the liver, the expression of miR-223 increases during ischemia and reperfusion injury [13]. miR-223 inhibits inflammation through inhibiting the production of IL-1beta in hepatic macrophages (PMID: 31585800). It has been reported that miR-223 could inhibit cell proliferation in liver cancer [14]. In the serum of patients with chronic hepatitis B virus, the expression of miR-223 was up-regulated, therefore, miR-223 was considered as a biomarker of liver injury [15]. Moreover, miR-223-3p can be used as a new biomarker for HBV-positive liver cancer [16]. These findings suggest the potential role of miR-223-3p in liver pathobiology.
The aim of this study is to investigate the effects of miRNA targeted regulating FAT1 on the occurrence and development of liver cancer cells, and to explore the regulatory mechanism of miR-223-3p and FAT1 in liver cancer, so as to explore new approaches for targeted diagnosis and treatment of liver cancer.
Forty-eight tumor tissues and corresponding adjacent tissues of patients with liver cancer treated in our hospital from March 2018 to October 2018 were collected. All patients received no chemotherapy or radiotherapy before operation. During the operation, liver cancer tissues and matched samples of adjacent non-cancer tissues that were 2 cm away from the edge of cancer lesions were obtained from patients, which were diagnosed and confirmed by experienced pathologists. Tumor tissues were quickly put into RNA preservation solution after isolation. The samples were obtained with the approval and consent of the ethics committee of our hospital. All patients signed the informed consent.
The microarray data of liver cancer chip, GSE117361, from GEO database (https://www.ncbi.nlm.nih.gov/geo/) was obtained. With empirical Bayesian method, “limma” package based on Bio-conductor of R language was used to select important differentially expressed genes. Differentially expressed genes were annotated by “annotate” package. The expression level of differentially expressed gene, FAT1, in liver cancer samples was retrieved in the tumor database (http://ualcan.path.uab.edu/cgi-bin/ualcan-res.pl) included in TCGA. The targeted prediction websites, microRNA, mirDIP, miRWalk, DIANA and Targetscan, were used to predict the upstream regulatory, miRNA, of FAT1 respectively. Take their intersections and predict the targeted binding sites of FAT1 and miRNA with DIANA.
Normal human hepatocyte, L-02, was purchased from the China Center for Type Culture Collection, and human liver cancer cell lines, Hep G2, SMMC-7721, Hep 3b and Li-7, were purchased from the Cell Resource Center of the Institute of Basic Medical Sciences of CAMS. L-02, Hep G2 and Hep 3b cell lines were cultured in MEM-EBSS: Minimum Essential Medium (MEM Eagles with Earle's Balanced Salts, purchased from Hyclone Company, USA) containing 10% of FBS, while SMMC-7721 and Li-7 cell lines were cultured in RPMI 1640 (w/o Hepes) medium (Hyclone Company, USA). Cells were incubated in a humid incubator (37 ℃, 5% CO2).
When the cells growing into logarithmic phase, liver cancer cell lines were grouped into sh-NC, sh-FAT1, NC mimic miR-223-3p mimic NC mimic+oe-NC, miR-223-3p mimic+oe-FAT1 group. miR-223-3p mimic and NC mimic were transiently transfected into liver cancer cells of each group with Lipofectamine® 2000 reagent, respectively, then cultured in corresponding mediums with 5% CO2 at 37 ℃. After 6 hours, replace the mediums and continuous culture them for 48 hours for subsequently experiments. Expression vector of FAT1 was constructed by lentivirus packaging. FAT1 cDNA (oe-FAT1) and shRNA sequence (sh-FAT1) with restriction enzyme sites of KpnI and XhoI and their corresponding control sequences (designed by BLOCK-iTTM RNAi Designer website) were synthesized and linked to Lentivirus Expression Vector, pLVX-IRES-neo, with T4 ligase. Transfect the receptor cells, and culture them to proliferate at 37 ℃ for 24 hours, then extract the plasmids and make a sequence analysis. Then the packaging plasmids and virus particles were dripped into liver cancer cells for invasion, and the silencing efficiency of different shRNA sequences were detected by qRT-PCR.
Trizol (Thermo Fisher Scientific, Waltham, MA, USA) was used to extract the total RNA from tissue samples and cells. cDNA of miRNA was synthesized with qScript microRNA cDNA synthesis kit (Quantabio, Beverly, MA) to detect the expression of miR-223-3p. 1 μg of the total RNA of FAT1 was used to synthesize cDNA with cDNA synthesis kit (Thermo Fisher Scientific, Waltham, MA, USA). Quantitative PCR was carried out with miScript SYBR Green PCR Kit (Qiagen, Hilden, Germany) under the following conditions of thermal cycler: 95 ℃ for 2 min, 95 ℃ for 5 s, and 60 ℃ for 30 s. Repeat this cycle for 40 times. The primers of miR-223-3p, U6, FAT1 and GAPDH were purchased from GeneCopoeia (GeneCopoeia Inc., Guangzhou, China).
Forward primer of miR-223-3p: 5′-GCAGAGTGTCAGTTTGTCAAAT-3′
U6 RT
Forward primer: 5′-CTCGCTTCGGCAGCACA-3′
Reverse primer: 5′-GTGCAGGGTCCGAGGT-3′
FAT1
Forward primer: 5′-GTG TTT GTT CTC TGC CGT AAG-3′
Reverse primer: 5′-TAGGCTTCT GGA TGG AGT CG-3′
GAPDH
Forward primer: 5′-GGAGCGAGATCCCTCCAAAAT-3′
Reverse primer: 5′-GGCTGTTGTCATACTTCTCATGG-3′
The result was presented as the comparison between the difference of the relative expression of the target gene mRNA of the control group and experimental group with 2-ΔΔCt value. The assay was repeated 3 times.
CCK-8 kit (Dojindo Molecular Technologies, Inc., Kumamoto, Japan) was used to detect the proliferation of cells. Liver cancer cells, Hep G2, were inoculated into a 24-well plate with a density of 6 × 105 cells/well, then incubated in 10% CCK-8 at 37 ℃ for color conversion. OD value was measured 24, 48 and 72 hours after transfection respectively to calculate the proliferation rate. The assay was repeated three times.
When the cells grew and about 70-80% of the cells emerged in the culture pore, the tip of the 200 μL of pipette was used to gently scrape the single layer that across the center of the pore. The pore was washed twice with medium to remove the separated cells. After adding fresh medium, the cells regrew for 24 hours. Then photograph under a microscope and calculate the migration rate of liver cancer cells.
The twenty-four -well Transwell(with a pore size of 8 μm,BD Biosciences)was used to detect the Transwell invasion. About 2 × 104 cells were added to the upper chamber, and the Matrigel matrix (Corning, Corning, NY) was used to coat the top chamber. Durbeco's modified Eagle medium (DMEM) containing 10% of fetal bovine serum (FBS) was filled into the lower chamber. After incubation at 37 ℃ for 24 hours, the cells that did not pass through the membrane were removed by cotton swab applicator, and the cells on the lower surface of the membrane were stained with crystal violet.
After transfection for 48 hours, the cells were washed 3 times with cold PBS, and lysed to proteins on ice for 10 min with whole protein lysate, then the proteins were quantitated by a BCA quantification kit. Add 10μL of loading buffer to the proteins and boil at 95 ℃ for 10 min, then perform SDS-PAGE at 100 V. After electrophoresis, the proteins were transferred to the NC membrane at 100 mA for 120 min. Block the membrane with blocking solution (5% BSA/TBST) for 60 min. Add primary antibodies, including FAT1 (ab190242, 1:2000, Abcam company, China), E-cadherin (ab15148, 1:500, Abcam company, China), Vimentin (ab137321, 1:500, Abcam company, China), GAPDH (ab9485, 1:2500, Abcam company, China), and incubate them at 4 ℃ overnight. After the primary antibodies incubated, the membrane was washed with 1×TBST solution at room temperature with a shaking table for 5 min×3 times, and the horseradish peroxidase-labeled secondary antibody, goat anti-rabbit IgG, was added to make a hybridization at room temperature for 120 min. TBST was used to wash the membrane 3 times. 20 min after each time, carry out the luminescence reaction with an ECL kit, and observe the western blot, then analyze the image. Analyze the band gray value with Quantity One software, and detect the relative expression of FAT1, which was expressed by the ratio of the gray value of the target band to the internal reference band. The assay was repeated 3 times.
HepG2 cells were inoculated into a 24-well plate with a density of 6×105 cells/well and incubated for 24 hours. Subsequently, the cells were co-transfected with 0.8 μg of pGL3-FAT1-3'UTR or pGL3-FAT1-3'UTR Mut plasmid or 0.08 ng of phRL-SV40 control vector (Promega Corporation, Madison, WI, USA), and then with 100nM of miR-223-3p or inactive control RNA using Lipofectamine 2000 (Invitrogen; Thermo Fisher Science, Inc). Twenty-four hours after transfection, the activity of Renilla luciferase and firefly luciferase were detected by dual-luciferase assay (Promega Corporation). The assay was repeated three times.
All data was processed using SPSS 22.0 statistical software. The measurement data was expressed in the form of mean ± standard deviation. The comparison between the two groups was performed by t test. The comparison among multiple groups was analyzed by one-way ANOVA. P < 0.05 indicates that the difference is statistically significant.
GEO database was used to analyze the differentially expressed genes in liver cancer chip, GSE117361. As shown in Figure 1A, FAT1 was highly expressed in liver cancer tissues. Meanwhile, the expression of FAT1 in clinical samples of liver cancer tissues was retrieved from TCGA database. As shown in Figure 1B, the expression of FAT1 was significantly up-regulated. Furthermore, the expression of FAT1 in the samples of liver cancer tissues and corresponding adjacent tissues was detected by qRT-PCR. As shown in Figure 1C, compared with that of adjacent tissues, the expression of mRNA of FAT1 in liver cancer tissues was significantly up-regulated. Then qRT-PCR was used to detect the expression of FAT1 in normal human liver cell, L-02, and human liver cancer cell lines, Hep G2, SMMC-7721, Hep 3b and Li-7. As shown in Figure 1D, compared with that of L-02, the expression of FAT1 in Hep G2, SMMC-7721, Hep 3b and Li-7 were significantly up-regulated (P < 0.05), especially in Hep 3B (P < 0.01).
Then qRT-PCR was used to detect the interference efficiency of FAT1. As shown in Figure 2A, compared with that of sh-NC group, the expression of mRNA of FAT1 in FAT1-shRNA1 group and FAT1-shRNA2 group were significantly down-regulated (P < 0.05), especially in FAT1-shRNA3 group (P < 0.01). Therefore, FAT1-shRNA3 group was selected as sh-FAT1 group in subsequent experiments for interference transfection. CCK-8 method was used to detect the cell viability of sh-NC group and sh-FAT1 group. As shown in Figure 2B, compared with that of sh-NC group, the Hep 3b cells viability in sh-FAT1 group significantly decreased (P < 0.05).
Wound healing assay was used to detect the migration ability of Hep 3b cells. As shown in Figure 3A, compared with that of sh-NC group, the migration rate of Hep 3B cells in sh-FAT1 group was significantly decreased (P < 0.05). Transwell invasion assay was used to detect the invasion ability of cells in sh-NC group and sh-FAT1 group. As shown in Figure 3B, compared with that of sh-NC group, the number of invasive cells in sh-FAT1 group decreased significantly (P < 0.05). Western blot was used to detect the expression of EMT-related markers, E-cadherin and Vimentin, in liver cancer cells of each group. As shown in Figure 3C, compared with that of sh-NC group, the expression of E-cadherin (epithelial marker) of sh-FAT1 group was significantly up-regulated, while the expression of Vimentin (interstitial marker) was significantly down-regulated (all P < 0.05).
Targeted prediction websites, microRNA, mirDIP, miRWalk, DIANA and Targetscan, were used to predict the upstream regulatory, miRNA, of FAT1. Take the intersections and predict the targeted binding sites of miRNA and FAT1 with DIANA. As shown in Figure 4A, B, miR-223-3p may targeted bind to the 3'UTR of FAT1. Then the targeted relationship was validated with dual-luciferase. As shown in Figure 4C, compared with that of FAT1-wt and NC co-transfection group, the luciferase activity of FAT1-wt and miR-223-3p co-transfection group significantly decreased (P < 0.05), indicating that there was a targeted regulation relationship between miR-223-3p and FAT1. Western blot was used to detect the expression of FAT1 in NC mimic group and miR-223-3p mimic group after transfection. As shown in Figure 4D, compared with that of NC mimic group, the expression of FAT1 in miR-223-3p mimic group was significantly down-regulated (P < 0.05).
qRT-PCR was used to detect the expression of miR-223-3p in the samples of liver cancer tissues and adjacent tissues. As shown in Figure 5A, compared with that of adjacent tissues, the expression of miR-223-3p in the samples of liver cancer tissues was significantly down-regulated. Then qRT-PCR was used to detect the expression of miR-223-3p in normal human liver cells, L-02, and human liver cancer cell lines, Hep G2, SMMC-7721, Hep 3b and Li-7. As shown in Figure 5B, compared with that of L-02, the expression of miR-223-3p in Hep G2, SMMC-7721, Hep 3b and Li-7 were significantly down-regulated (P < 0.05), especially in Hep 3b (P < 0.01).
CCK-8 method was used to detect the cell viability of NC mimic group, miR-223-3p mimic group, NC mimic+oe-NC group and miR-223-3p mimic+oe-FAT1 group. As shown in Figure 6, compared with that of NC mimic group, the Hep 3b cells viability in miR-223-3p mimic group significantly decreased (P < 0.05); compared with that of miR-223-3p mimic group, the Hep 3b cells viability in miR-223-3p mimic+oe-FAT1 group significantly improved (P < 0.05).
Wound healing assay was used to detect the migration ability of cells in NC mimic group, miR-223-3p mimic group, NC mimic+oe-NC group and miR-223-3p mimic+oe-FAT1 group. As shown in Figure 7A, compared with that of NC mimic group, the cell migration ability of Hep 3b in miR-223-3p mimic group significantly decreased (P < 0.05); compared with that of miR-223-3p mimic group, the cell migration ability of Hep 3b in miR-223-3p mimic+oe-FAT1 group significantly improved (P < 0.05). Transwell invasion assay was used to detect the cell invasion ability of each group. As shown in Figure 7B, compared with that of NC mimic group, the cell invasion ability of Hep 3b in miR-223-3p mimic group significantly decreased (P < 0.05); compared with that of miR-223-3p mimic group, the cell invasion ability of Hep 3b in miR-223-3p mimic+oe-FAT1 group significantly improved (P < 0.05)t. Western blot was used to detect the expression of EMT-related markers, E-cadherin and Vimentin, in liver cancer cells of each group. As shown in Figure 7C, compared with that of NC mimic group, the expression of E-cadherin (epithelial marker) of miR-223-3p mimic group was significantly up-regulated, while the expression of Vimentin (interstitial marker) was significantly down-regulated (all P < 0.05); compared with that of miR-223-3p mimic group, EMT in miR-223-3p mimic+oe-FAT1 group was inhibited.
The specific function of FAT1 in cancer development and progression is still under study. FAT1 is considered as both an oncogene and an anti-oncogene [8,9,10]. It has been proved that FAT1 is highly expressed in HCC cells and tissues, indicating that FAT1 can act as a carcinogen in HCC [11], which is consistent with the results of our study. In addition, FAT1 seems to inhibit the progression of agammaessive phenotypes in breast cancer [17]. In non-malignant vascular smooth muscle cells, FAT1 knockdown leads to the decrease of migration activity [18]. Moreover, FAT1 can be used as a regulator of inflammatory pathway of cancer cells [19]. Its expression in liver cancer has been reported, and it is considered to be a relevant mediator of the key tumorigenesis that from growth receptor signal to HCC [11]. Besides, FAT1 expression is associated with higher tumor stage and mitotic activity in human HCC. Some studies have found that FAT1 affects the epithelial-mesenchymal transition (EMT) of glioblastoma [20] and esophageal squamous cell carcinoma [21], while the effect on EMT in liver cancer has not been reported. In this study, we silenced the expression of FAT1 and found that silencing FAT1 inhibited the proliferation, migration and invasion ability and EMT of liver cancer cells. Moreover, from the perspective of targeted regulation of miRNA, we looked for the mechanism of FAT1 in liver cancer in the upstream.
miRNA is associated with a variety of liver diseases, including acute liver injury, nonalcoholic fatty liver disease and nonalcoholic fatty hepatitis, hepatitis B virus/hepatocellular carcinoma infection and liver cancer [12]. miRNA can play a important role in signal transduction pathway of tumor by degrading or inhibiting targeted mRNAs translation (PMID: 27120794, 25889562). Therefore, we dig up the upstream regulatory miRNA of FAT1. It was found that miR-223-3p might regulate the expression of FAT1. miR-223-3p has potential as a new non-invasive biomarker for early diagnosis of hepatitis B virus (HBV) associated hepatocellular carcinoma (HCC) [22]. In this study, we used several targeted prediction websites and discovered the targeted binding relationship between miR-223-3p and 3'-UTR of FAT1, which was validated by luciferase assay. At the same time, the study showed that miR-223-3p was lowly expressed in liver cancer cells, which was negatively correlated with the trend of FAT1 protein expression, suggesting that in liver cancer, miR-223-3p may regulate the occurrence and development of liver cancer through targeted down-regulating the expression of FAT1 (Figure S1). Therefore, we used the miR-223-3p mimic group to overexpress miR-223-3p, but the up-regulation of miR-223-3p expression inhibited the expression of FAT1 and the effect of FAT1 on the proliferation, migration and invasion of liver cancer cells, and regulated the expression of EMT markers. These results suggest that miR-223-3p can targeted down-regulate the expression of FAT1, thus inhibit the occurrence and development of liver cancer cells [23].
In conclusion, this study identified the targeted regulation of miR-223-3p on FAT1 in liver cancer cells and found its effect on the biological behavior of liver cancer cells. Although the regulation axis of miR-223-3p / FAT1 has been explored in this study, the related signaling pathways involved in the downstream of FAT1 protein and the reasons for the low expression of miR-223-3p in HCC are still unknown. Its in-depth molecular mechanism needs further study in the future. But overall, this study may provide a new insight into the mechanism of the occurrence and development of liver cancer, and provide a new way of its diagnosis and treatment.
This research was supported by Zhejiang Provincial Natural Science Foundation of China under Grant No. LQ19H030011
The authors have no conflict of interest to declare.
[1] | Y. A. Ghouri, I. Mian, B. Blechacz, Cancer review: Cholangiocarcinoma, J. Carcinog., 14 (2015). |
[2] | J. Tejeda-Maldonado, I. García-Juárez, J. Aguirre-Valadez, A. González-Aguirre, M. Vilatobá-Chapa, A. Armengol-Alonso, Diagnosis and treatment of hepatocellular carcinoma: An update, World J. Hepatol., 7 (2015), 362-376. |
[3] | A. Kinoshita, H. Onoda, N. Fushiya, K. Koike, H. Nishino, H. Tajiri, Staging systems for hepatocellular carcinoma: Current status and future perspectives, World J. Hepatol.,7 (2015), 406-424. |
[4] | T. Kimhofer, H. Fye, S. Taylor-Robinson, E. Holmes, Proteomic and metabonomic biomarkers for hepatocellular carcinoma: A comprehensive review, Br. J. Cancer, 112 (2015), 1141-1156. |
[5] | J. Dunnea, A. M. Hanby, R. Poulsom, T. A. Jones, Molecular cloning and tissue expression of FAT, the human homologue of the Drosophila fat gene that is located on chromosome 4q34-q35 and encodes a putative adhesion molecule, Genomics, 30 (1995), 207-223. |
[6] | L. Ciani, A. Patel, N. D. Allen, C. ffrench-Constant, Mice lacking the giant protocadherin mFAT1 exhibit renal slit junction abnormalities and a partially penetrant cyclopia and anophthalmia phenotype. Mol. Cell Biol., 23 (2003), 3575-3582. |
[7] | M. Katoh, Function and cancer genomics of FAT family genes, Int. J. Oncol., 41 (2012), 1913-1918. |
[8] | N. Kwaepila, G. Burns, A. S. Leong, Immunohistological localisation of human FAT1 (hFAT) protein in 326 breast cancers. Does this adhesion molecule have a role in pathogenesis? Pathology, 38 (2006), 125-131. |
[9] | C. E. de Bock, A. Ardjmand, T. J. Molloy, S. M. Bone, D. Johnstone, D. M Campbell, The Fat1 cadherin is overexpressed and an independent prognostic factor for survival in paired diagnosis-relapse samples of precursor B-cell acute lymphoblastic leukemia, Leukemia, 26 (2012), 918-926. |
[10] | B. Dikshit, K. Irshad, E. Madan, N. Aggarwal, C. Sarkar, P. S. Chandra, FAT1 acts as an upstream regulator of oncogenic and inflammatory pathways, via PDCD4, in glioma cells, Oncogene, 32 (2013), 3798-3808. |
[11] | D. Valletta, B. Czech, T. Spruss, K. Ikenberg, P. Wild, Arndt Hartmann, Regulation and function of the atypical cadherin FAT1 in liver cancer, Carcinogenesis., 35 (2014), 1407-1415. |
[12] | C. H. Hung, Y. C. Chiu, C. H. Chen, T. H. Hu, MicroRNAs in hepatocellular carcinoma: Carcinogenesis, progression, and therapeutic target, Biomed. Res. Int., 2014 (2014). |
[13] | C. H. Yu, C. F. Xu, Y. M. Li, Association of MicroRNA-223 expression with hepatic ischemia/reperfusion injury in mice, Dig. Dis. Sci., 54 (2009), 2362. |
[14] | A. Aucher, D. Rudnicka, D. M. Davis, MicroRNAs transfer from human macrophages to hepato-carcinoma cells and inhibit proliferation, J. Immunol., 191 (2013), 6250-6260. |
[15] | J. Xu, C. Wu, X. Che, L. Wang, D. Yu, T. Zhang, Circulating microRNAs, miR-21, miR-122, and miR-223, in patients with liver cancer or chronic hepatitis, Mol. Carcinog., 50 (2), 136-142. |
[16] | X. V. Qadir, W. Chen, C. Han, K. Song, J. Zhang, T. Wu, MiR-223 Deficiency Protects against Fas-Induced Hepatocyte Apoptosis and Liver Injury through Targeting Insulin-Like Growth Factor 1 Receptor, Am. J. Pathol., 185 (2015), 3141-3151. |
[17] | S. Lee, S. Stewart, I. Nagtegaal, J. Luo, Y. Wu, G. Colditz, Differentially expressed genes regulating the progression of ductal carcinoma in situ to invasive breast cancer, Cancer Res., 72 (2012), 4574-4586. |
[18] | R. Hou, L. Liu, S. Anees, S. Hiroyasu, N. E. S. Sibinga, The Fat1 cadherin integrates vascular smooth muscle cell growth and migration signals, J. Cell. Biol., 173 (2006), 417-429. |
[19] | M. J. Moeller, A. Soofi, G. S. Braun, X. Li, C. Watzl, W. Kriz, Protocadherin FAT1 binds Ena/VASP proteins and is necessary for actin dynamics and cell polarization, EMBO. J., 23 (2004), 3769-3779. |
[20] | C. Srivastava, K. Irshad, B. Dikshit, P. Chattopadhyay, C. Sarkarm, D. K. Gupta, FAT1 modulates EMT and stemness genes expression in hypoxic glioblastoma, Int. J. Cancer, 142 (2018), 805-812. |
[21] | X. Hua, Y. Zhai, P. Kong, H. Cui, T. Yan, J. Yang, FAT1 prevents epithelial mesenchymal transition (EMT) via MAPK/ERK signaling pathway in esophageal squamous cell cancer, Cancer Lett., 397 (2017), 83-93. |
[22] | B. G. Giray, G. Emekdas, S. Tezcan, M. Ulger, M. S. Serin, O. Sezgin, Profiles of serum microRNAs; miR-125b-5p and miR223-3p serve as novel biomarkers for HBV-positive liver cancer, Mol. Biol. Rep., 41 (2014), 4513-4519. |
[23] | Z. B. Han, L. Zhong, M. J. Teng, J. W. Fan, H. M. Tang, J. Y. Wu, Identification of recurrence-related microRNAs in hepatocellular carcinoma following liver transplantation, Mol. Oncol., 6 (2012), 445-457. |
1. | Xun Yin, Yingjie Wang, Yingfei Sun, Yun Han, Huanling Sun, Mengyun Zou, Ronglong Luo, Xiuli Peng, Down-regulated gga-miR-223 inhibits proliferation and induces apoptosis of MG-infected DF-1 cells by targeting FOXO3, 2021, 08824010, 104927, 10.1016/j.micpath.2021.104927 | |
2. | Shuyue Jiao, Xin Zhang, Dapeng Wang, Hongyong Fu, Qingxin Xia, Genetic Alteration and Their Significance on Clinical Events in Small Cell Lung Cancer, 2022, Volume 14, 1179-1322, 1493, 10.2147/CMAR.S356037 | |
3. | Chen‐Kun He, Zeng‐Bo Li, Da Yi, Xiang‐Ya Zhu, Rong‐Rong Liu, Dong‐Xin Zhang, Qian Cao, Yi‐Ping Chen, LncRNA FGD5‐AS1 enhances the proliferation and stemness of hepatocellular carcinoma cells through targeting miR‐223 and regulating the expression of ECT2 and FAT1, 2022, 52, 1386-6346, 614, 10.1111/hepr.13767 | |
4. | Yuli Wang, Shuang Shi, Yunshan Wang, Xuhua Zhang, Xiaoyan Liu, Juan Li, Peilong Li, Lutao Du, Chuanxin Wang, miR ‐223‐3p targets FBXW7 to promote epithelial‐mesenchymal transition and metastasis in breast cancer , 2022, 13, 1759-7706, 474, 10.1111/1759-7714.14284 | |
5. | Lili Nie, Hui Zou, Chi Ma, Xiaoke Wang, Liaqat Ali, miR-223-3p Regulates NLRP3 to Inhibit Proliferation and Promote Apoptosis of ONG Cells, 2022, 2022, 1748-6718, 1, 10.1155/2022/2805645 | |
6. | Zhenxing Feng, Yan Yin, Bin Liu, Yafang Zheng, Dongsheng Shi, Hong Zhang, Jianwen Qin, Prognostic and Immunological Role of FAT Family Genes in Non-Small Cell Lung Cancer, 2022, 29, 1073-2748, 107327482210766, 10.1177/10732748221076682 | |
7. | Oliver Buchhave Pedersen, Erik Lerkevang Grove, Peter H. Nissen, Sanne Bøjet Larsen, Leonardo Pasalic, Steen Dalby Kristensen, Anne-Mette Hvas, Expression of microRNA Predicts Cardiovascular Events in Patients with Stable Coronary Artery Disease, 2023, 123, 0340-6245, 307, 10.1055/s-0042-1760258 | |
8. | Bingxue Zhang, Li Deng, Xiaodan Liu, Yao Hu, Wenyi Wang, Minghua Li, Ting Xu, Li Pang, Meifen Lv, Transcranial direct current stimulation combined with swimming exercise improves the learning and memory abilities of vascular dementia rats by regulating microglia through miR-223-3p/PRMT8, 2024, 46, 0161-6412, 525, 10.1080/01616412.2024.2337517 | |
9. | Shuyan Li, Jian Zhang, Zhengming Wang, Wenjun Li, Downregulated lncRNA DRAIC enhances the radiotherapy sensitivity of human HCC cell line HepG2 by targeting miR-223-3p, 2022, 8, 2095-9621, 293, 10.1007/s10330-022-0584-4 | |
10. | Tao Wang, Junting Li, Jun Du, Wei Zhou, Guang Lu, Recent advances in the role of atypical cadherin FAT1 in tumorigenesis (Review), 2024, 29, 1792-1074, 10.3892/ol.2024.14856 | |
11. | MENG HU, ZIYI FANG, YONGFU SHAO, JIANING YAN, GUOLIANG YE, Biological roles and molecular mechanism of circular RNAs in epithelial-mesenchymal transition of gastrointestinal malignancies, 2025, 33, 1555-3906, 549, 10.32604/or.2024.051589 |