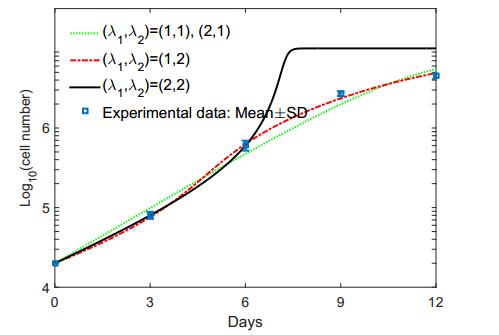
Citation: Hsiu-Chuan Wei. Mathematical modeling of tumor growth: the MCF-7 breast cancer cell line[J]. Mathematical Biosciences and Engineering, 2019, 16(6): 6512-6535. doi: 10.3934/mbe.2019325
[1] | Sandesh Athni Hiremath, Christina Surulescu, Somayeh Jamali, Samantha Ames, Joachim W. Deitmer, Holger M. Becker . Modeling of pH regulation in tumor cells: Direct interaction between proton-coupled lactate transporters and cancer-associated carbonicanhydrase. Mathematical Biosciences and Engineering, 2019, 16(1): 320-337. doi: 10.3934/mbe.2019016 |
[2] | Abeer S. Alnahdi, Muhammad Idrees . Nonlinear dynamics of estrogen receptor-positive breast cancer integrating experimental data: A novel spatial modeling approach. Mathematical Biosciences and Engineering, 2023, 20(12): 21163-21185. doi: 10.3934/mbe.2023936 |
[3] | Li-Bing Wang, Liang Feng, Jing He, Bo Liu, Jian-Guang Sun . MiR-125a-5p inhibits the proliferation and invasion of breast cancer cells and induces apoptosis by targeting GAB2. Mathematical Biosciences and Engineering, 2019, 16(6): 6923-6933. doi: 10.3934/mbe.2019347 |
[4] | Donggu Lee, Sunju Oh, Sean Lawler, Yangjin Kim . Bistable dynamics of TAN-NK cells in tumor growth and control of radiotherapy-induced neutropenia in lung cancer treatment. Mathematical Biosciences and Engineering, 2025, 22(4): 744-809. doi: 10.3934/mbe.2025028 |
[5] | Samantha L Elliott, Emek Kose, Allison L Lewis, Anna E Steinfeld, Elizabeth A Zollinger . Modeling the stem cell hypothesis: Investigating the effects of cancer stem cells and TGF−β on tumor growth. Mathematical Biosciences and Engineering, 2019, 16(6): 7177-7194. doi: 10.3934/mbe.2019360 |
[6] | Ghassen Haddad, Amira Kebir, Nadia Raissi, Amira Bouhali, Slimane Ben Miled . Optimal control model of tumor treatment in the context of cancer stem cell. Mathematical Biosciences and Engineering, 2022, 19(5): 4627-4642. doi: 10.3934/mbe.2022214 |
[7] | Cyrille Agossou, Mintodê Nicodème Atchadé, Aliou Moussa Djibril, Svetlana Vladimirovna Kurisheva . Mathematical modeling and machine learning for public health decision-making: the case of breast cancer in Benin. Mathematical Biosciences and Engineering, 2022, 19(2): 1697-1720. doi: 10.3934/mbe.2022080 |
[8] | Qingqun Huang, Adnan Khalil, Didar Abdulkhaleq Ali, Ali Ahmad, Ricai Luo, Muhammad Azeem . Breast cancer chemical structures and their partition resolvability. Mathematical Biosciences and Engineering, 2023, 20(2): 3838-3853. doi: 10.3934/mbe.2023180 |
[9] | Qiaojun Situ, Jinzhi Lei . A mathematical model of stem cell regeneration with epigenetic state transitions. Mathematical Biosciences and Engineering, 2017, 14(5&6): 1379-1397. doi: 10.3934/mbe.2017071 |
[10] | J. Ignacio Tello . On a mathematical model of tumor growth based on cancer stem cells. Mathematical Biosciences and Engineering, 2013, 10(1): 263-278. doi: 10.3934/mbe.2013.10.263 |
Breast cancer is the second leading cause of cancer death for women worldwide [1,2]. Approximately 75% of breast cancer cases are estrogen receptor positive [3]. The MCF-7 cell line is a common in vitro model used for studies on human breast cancer. The popularity of MCF-7 can be attributed to its estrogen responsiveness through expression of estrogen receptor, making it an idea model to study the effect of hormones on cell proliferation and protein synthesis [4,5,6].
White blood cells are the cells of the immune system that helps the body fight infectious disease and foreign invaders. All WBCs are produced in the bone marrow and found in the blood and lymphatic system [7]. The number of WBCs in the blood is often a marker of disease. A low WBC count may occur as a result of viral infections, cancer treatment, bone marrow condition, or certain diseases [8]. An individual with a low WBC count may increase frequency of infections and fevers and cause low immunity [9]. Shankar et al. [10] have found an association between WBC count and cancer mortality. Other clinical studies have reported that there is no significant association between WBC count and cancer risk or cancer mortality [11,12,13,14].
Natural killer cells are specialized WBCs and form a very important component of the innate system. They are able to detect early-stage developing tumors and secrete immuno-regulatory cytokines and numerous chemokines that directly and effectively eliminate tumor cells. This process is sometimes called immune surveillance, and it is an important process to prevent neoplastic cells from developing into tumors. Cytotoxic T lymphocytes, which are produced in the bone marrow and migrate to the thymus for maturation, are key players of adaptive immune system. Upon activation of CTLs, the T-cell antigen receptors expressed on CTLs allow them to monitor all cells of the body, ready to destroy infected cells. Cytotoxicity is mediated through Fas or perforin pathways inducing apoptosis in target cells [15].
Despite the antitumor effect of NK cells and CTLs, there are still neoplastic cells that escape immune surveillance. However, even a fast growing tumor cannot grow beyond 1-2 mm in diameter without angiogenesis. At this stage, a balance exists between cell proliferation and apoptosis, and tumors may maintain dormant for years. Furthermore, clinical evidence has suggested that many of us have microscopic tumors that remain asymptomatic and never develop and progress to become large and lethal [16,17,18]. In fact, it has been estimated that more than one third of middle aged women who have never been diagnosed with breast cancer throughout their life were found at autopsy with in situ tumors in their breast [16]. When tumors develop their own vascularization, they can grow much larger and become lethal.
Mathematical modeling of tumor growth is a powerful tool to understand, predict, and improve the outcome of a treatment regimen. A mathematical model of tumor growth often includes tumor-immune interaction which is an important component for the model to produce clinical phenomena. Most mathematical models for cancer are based on differential equations [19]. While partial differential equations have been employed to model the spatio-temporal growth and spread of cancer cells, ordinary differential equations (ODEs) have been used to investigate the interactions among cancer cells, immune cells, and cytokines [20]. ODE systems with two or three cell populations, which usually contain tumor cells and effector cells, have been constructed to study the population dynamics [21,22,23,24,25,26,27,28,29,30]. These models are able to capture the essential patterns of tumor growth and decay: exponential/oscillatory growth or decay of tumor cells. Forys et al. [30] and de Vladar and Gonzˊalez [29] have reported the existence of stable small tumor equilibria in their models. These dynamics can describe dormant or persistent microscopic tumors. However these results are merely obtained by mathematical models [29,30] while the interaction terms and parameter values in the mathematical models have not been verified by experimental or clinical data.
More complex models involving more equations are devised to study particular aspects of tumor biology and have been able to provide significant insights into the mechanisms of tumor-immune interactions. These models inevitably involve more parameters and thus less well parameterized [20]. de Boer et al. [31] have considered an ODE system of eleven equations and reported that the cytotoxic T lymphocyte (CTL) count depends on the time of T helper cell activation. They have also pointed out that a tumor with strong antigenicity is destroyed by CD8+ T cells while a tumor with weak antigenicity is destroyed by macrophages. Numerical simulation using an ODE system of six equations by Cappuccio et al. [32] has suggested that IL-21 can be used to treat non-immunogenic tumors but not highly immunogenic tumors. Kronik st al. [33] have proposed a model, which includes gliomas cancer cells, CTLs, MHC class Ⅰ and Ⅱ molecules, and two cytokines, to study the effects of immunotherapy.
Jarrett et al. [34] have proposed a mathematical model of HER2+ breast cancer with immune response and studied the outcomes of trastuzumab treatment. Annan et al. [35] have constructed an ODE system describing the interactions between breast cancer cells and immune cells including CTLs, macrophages, NK cells, and helper T cells. Roe et al. [36] have presented a mathematical model to study the effect of chemotherapy on breast cancer. Most of the parameter values and the functional forms in these studies have not been justified by experimental and clinical data. The purpose of this paper is to build a mathematical model using ODEs which describe MCF-7 breast tumor growth, tumor-immune interaction, and tumor-estrogen interaction. Experimental data from several research studies are used to determine the functional responses and parameter values in the mathematical model. The dynamics produced by the mathematical model are to be compared with experimental and clinical phenomena. To provide details, the mathematical model is presented in Section 2. Numerical examples and discussion are given in Section 3. Finally, a brief conclusion is made in Section 4.
The model considered in this paper consists of five state variables: the MCF-7 tumor population T (cells), the circulating level of estradiol E (pmol/L), NK cell population N (cells/L), CTL (or CD8+ T) cell population L (cells/L), and WBC population C (cells/L). The mathematical model is described as the following ODEs:
dTdt=(a+ITE(T,E))T(1−T/K)−ITN(T,N)−ITL(T,L), | (2.1) |
dNdt=eC−fN−p2NT+INT(T,N), | (2.2) |
dLdt=(p4LN+p5Iα4+IL)(1−L/KL)Tα5+T−dL, | (2.3) |
dCdt=α−βC, | (2.4) |
where E(t) is a periodic function and t is in days.
Tumor growth includes an initial exponential growth phase followed by a linear growth phase. As tumors grow, the availability of resources (nutrients, oxygen, and space) decreases resulting in deceleration of tumor growth, and the tumor size eventually reaches its maximum value [5,37]. The tumor population T is assumed to follow a logistic or sigmoidal curve including three phases: an exponential phase, a linear phase, and a plateau phase. The parameter a is the intrinsic growth rate, and K is the carrying capacity of the tumor.
The proliferation of MCF-7 cells is known to be stimulated by E2. The term ITE(T,E) represents the interaction between MCF-7 cells and E2. The terms ITN(T,N) and ITL(T,L) represents tumor lysis by NK cells and CTLs, respectively. The term INT(T,N) represents recruitment of NK cells in the presence of tumor cells. Because NK cells are a type of WBCs, the growth of NK cells, eC, depends on the concentration of WBCs. The parameter f represents the death rate of NK cells, and the parameters α and β represent the birth and death rate of WBCs, respectively. NK cells release perforin, which forms pores in the cell membrane of the target cell. They also release granzymes, which enter through these pores, inducing apoptosis. An activated NK cell can kill 4 target cells in 16 hours, and then it appeared to be exhausted due to significant reduction in perforin and granzyme B content [38,39]. The term −p2NT, which is adopted from a model of tumor-immune interactions by de Pillis et al. [28], describes that NK cells become inactivated after some encounters with tumor cells, and the parameter p2=3.42×10−6 represents the mean inactivation rate.
A normal range for the WBC count is 4−12×109 cells per liter [40]. Experimental data by [41] have shown that healthy young individuals have NK cell counts of (3.87±1.64)×108 cells/L (Mean±SD) and healthy elderly individuals have NK cell counts of (3.77±2.21)×108 cells/L. It is known that approximately 34% of WBCs are lymphocytes in human blood [42]. Pascal et al. have reported 2 to 31% of NK cells within blood lymphocytes in a group of healthy adults [43]. Thus an immune system with an equilibrium NK cell count of 4×108/L and WBC count of 5.7×109/L is considered to be a normal healthy immune system. The equilibrium concentration of WBC is α/β which is determined by Eq (2.4). The parameter value β=6.3×10−3 in a research study by de Pillis [44] is used in this paper. An equilibrium WBC concentration of 5.7×109 cells per liter implies α=5.7×109×6.3×10−3=3.6×107. Experimental data from a research study by Zhang et al. [41] have shown that the half-life of NK cells is about 10 days, and this implies f=0.0693. An equilibrium NK cell count of 4×108/L in the absence of tumor cells implies e=fN/C=0.0693×4×108/5.7×109=0.00486. Consider that the size of an MCF-7 cell is 15-25 μm in diameter [45,46] and a tumor of 2 cm in diameter is within the diagnostic capabilities [47]. Assuming that an MFC-7 cell is 20 μm in diameter, an MCF-7 tumor of 2 cm in diameter contains 109 tumor cells. Several research studies [27,28,48,49,50,51] have also used 109 cells for the carrying capacity K in their mathematical models. In this model, the carrying capacity K=109 is used.
The matured T cells are considered as naive T cells as they have not encounter appropriate antigen. The number of mature T cells increases progressively during young life and then remains relatively constant during adulthood [52]. An activation of naive CD8+ T cells requires two signals. The first signal occurs when a naive CD8+ T cell encounters and interacts with an antigen presenting cell, such as a dendritic cell and a macrophages, through the T cell receptor (TCR) binding to MHC molecules. The second signal, the co-stimulatory signal, is provided by interaction between CD28 and B7 on the membrane of the T cell and APC, respectively [15]. Once activated, CD8+ T cells undergoes clonal expansion with interleukin-2 (IL-2) stimulation [53]. At the end of immune response, CD8+ T cells undergo a contraction phase during which about 90% of CD8+ T cells die. The cells that survive apoptosis become memory cells for a rapid secondary response to antigen [54].
The term T/(α5+T) is used to model the phenomenon that CTLs are activated in the presence of tumor cells and undergo apoptosis at the end of immune response. The constant α5 is assumed to be a relatively small number compared with the carrying capacity, K, of tumor cells. In this paper, α5=1000 is assumed. From the clinical data by Gruber et al. [55] in a study of relationship between tumor cells and T lymphocytes in breast cancer patients, the patients had CTL counts of 365±194 cells/μL (Mean±SD) and naive CTL counts of 237±112 cells/μL. The constant LN in Eq (2.3) represents the number of naive CTLs, and LN=2.3×108 cells/L is assumed in this paper. The constant KL represents the carrying capacity of CTLs and KL=8×108 cells/L, which is about mean+2SD, is assumed. Homann et al. [56] have reported that during primary immune response CTLs have a doubling time of 6-10 hours between days 5 and 7 and 24–36 hours between days 7 and 9. The number of CTL population reaches its peak on day 8. De Boer et al. [57] have reported that the half life of CTLs is approximately 41 hours (about 1.7 days) during the contraction phase. This gives a death rate of d=ln2/1.7=0.41 in Eq (2.3). The term p5Iα4+IL represents proliferation of CTLs, where I represents the concentration of IL-2 (g/L). Assume that the average of doubling time during the expansion phase is 8 hours. This gives a growth rate of p5Iα4+I=3ln2=2.07. To estimate the parameter value for p4, assume that T/(α5+T)≈1 in the presence of tumor during the expansion phase, and the number of CTL population reaches a level at 90% of its carrying capacity KL, which is 7.2×108 on day 8. Equation (2.3) is reduced to
dLdt=(p4LN+p5Iα4+IL)(1−L/KL)−dL, | (2.5) |
with conditions L(0)=0 and L(8)=7.2×108. Solving for p4 in the above equation gives p4=9×10−5.
The interactions between immune cells and tumor cells are usually described by functional responses of the predator-prey type [20]. The typical forms of the predator-prey type are based on Lotka Volterra, Holling type Ⅱ, or Holling type Ⅲ [58]. These forms with predator interference can be sumarised as Ixy(x,y)=ˉpxλ1yλ2/(1+ˉαxλ1+ˉβyλ2). For example, the interaction term Ixy is Lotka Volterra functional response if λ1=λ2=1 and ˉα=ˉβ=0, and Ixy is of Holling type Ⅱ with predator interference if λ1=λ2=1. The functional responses with λ1=1 or 2 and λ2=1 or 2 will be considered in this paper.
Let Td be the doubling time of the MCF-7 cell population. Then a=ln2/Td. It has been found that the growth of MCF-7 cells in vitro varies over a large range in the absence of estrogens. Experimental data have shown that the population doubling time ranges between 30 hours and several days [59,60,61]. A doubling time of 30 hours is corresponding to a=ln2/(30/24)=0.55 whereas a doubling time of 10 days is corresponding to a=ln2/10=0.069. A value falls in the range 0.069-0.55 is considered a reasonable parameter value for a. MCF-7 cells contain estrogen receptors and are estrogen responsive. Consider the following functional response for ITE(T,E):
ITE(T,E)=cEλ1Tλ2−11+α1Eλ1+β1Tλ2. | (2.6) |
The parameters λ1=1 or 2, and λ2=1 or 2. Parameters α1 and β1 are positive constants. The MCF-7 growth in the presence of E2 becomes
dTdt=(a+cEλ1Tλ2−11+α1Eλ1+β1Tλ2)T(1−T/K). | (2.7) |
Equation (2.7) is used to fit the experimental data by Nawata et al. [59], where the E2 concentration is 10−8M. Figure 1 shows the experimental data and the fitting curve. The experimental data show that a population level of 4×106 cells is at the log growth phase. Therefore, K=107 and E2=10000 (pmol/L) are used in this fitting. Let xi, i=0,1,⋯,4 be the number of tumor cells from experimental data shown in Figure 1. The parameters a, c, α1, and β1 are to be found for minimizing √∑4i=0((Ti−xi)/xi)2, where Ti=T(3i) is the solution of Eq (2.7) with initial condition T(0)=2×104. Different sets of (λ1,λ2) values are fitted separatedly. The Nelder Mead simplex method is employed to solve the least square error of the fitting. The ODE solver is the Runge Kuta method. The Matlab build-in functions fminsearch and ode45 can also fulfill the tasks. Figure 1 shows that λ1=1 and λ2=2 can best fit Eq (2.7) to the data with the parameter values a=0.3, c=1.93×10−6, α1=0.507, and β1=7.08×10−8.
The term ITN(T,N) representing cytolysis of NK cells is modeled as follows:
ITN(T,N)=p1Tλ1Nλ21+α2Tλ1+β2Nλ2. | (2.8) |
Parameter p1, α1, and β1 are positive constants to be determined by experimental data. The interaction between NK cells and tumor cells includes ITN(T,N) and −p2NT, where p2=3.42×10−6. Consider the following equations:
dTdt=−p1Tλ1Nλ21+α2Tλ1+β2Nλ2, | (2.9) |
dNdt=−p2NT. | (2.10) |
The experimental data used in this fitting were provided by Caragine et al. [62], and 4-hour chromium-51 release assays were used in the cytolysis assays. Note that the experiment has been conducted to study MFC-7 tumor cell lysis by NK cells. Figure 2 shows the experimental data and the fitting curve. Let xi, i=0,1,2,3 be the experimental data points in Figure 2 and yi=(T(0)−T(4/24))/T(0), where T(4/24) is the solution to Eqs (2.9) and (2.10) with T(0)=106 given in [62] and N(0)=Ni, where N0=0, N1=25T(0), N2=50T(0), and N3=100T(0). The fitting process is formed using different sets of (λ1,λ2). The parameter values for p1, α2, and β2 are to be determined for minimizing √∑3i=0(yi−xi)2. It has been found that λ1=1 and λ2=2 can best fit Eqs. (2.9) and (2.10) to the data, with the parameter values p1=8.7×10−4, α2=7×106, β2=5.4×10−5.
The term ITL(T,L) representing cytolysis by CTLs is modeled as follows:
ITL(T,L)=p6Tλ1Lλ21+α6Tλ1+β6Lλ2. | (2.11) |
The fitting process is similar to that in Section 2.2. Using the experimental data in [63], (λ1,λ2)=(1,1) or (2, 1) can better fit Eq (2.11) to the data than (λ1,λ2)=(1,2). Note that the experiment has been conducted to study MFC-7 tumor cell lysis by CLTs. The parameter values p6=2.36×10−4, α6=0, β6=6.04×10−5 are determined for (λ1,λ2)=(1,1) and p6=2.38×10−8, α6=0, β6=4.3×10−5 are determined for (λ1,λ2)=(2,1). Figure 3 shows the experimental data and the fitting curve.
The term INT(T,N) represents the recruitment of NK cells to the tumor site. To model the recruitment of NK cells, consider the following equations:
dTdt=aT(1−T/K)−p1TN21+α2T+β2N2−p6T2L1+α6T2+β6L, | (2.12) |
dNdt=eC−fN−p2NT+p3Tλ1Nλ21+α3Tλ1+β3Nλ2, | (2.13) |
dLdt=(p4LN+p5Iα4+IL)(1−L/KL)Tα5+T−dL. | (2.14) |
The form and parameter values of the functional response for recruitment of NK cells are to be determined using the experimental data from a research study by Chen et al. [64]. The experiment has been conducted to study and compare different treatment starting after 7 weeks of the presence of MCF-7 breast cancer in ovariectomized nude mice. Therefore, the term ITE is dropped out of Eq (2.12). The experimental data set used in this fitting is the one without treatment. It is assumed that the CTL and NK counts have reached high levels after 7 weeks of the presence of tumor cells, and thus the initial conditions of Eqs (2.12)–(2.14) are given by T(0)=5.7×106, N(0)=2×108, and L(0)=3×108. The proliferation of CTLs slows down to a doubling time of 24–36 hours after reaching the peak level [56]. Using an average doubling time of 30 hours, 1.25 days, gives p5I/(α4+I)=ln2/1.25=0.55 in this fitting. Other experimental data have shown WBC counts of (4.3±0.8)×103 cells/μL in mice [65]. The concentration of WBC is assumed to remain an equilibrium concentration C=4.3×109 cells/L in this fitting. The other parameter values have been determined in the previous subsections. The fitting process is similar to that in Section 2.1, where p3, α3, and β3 are unknown parameters. Figure 4 shows the experimental data and the fitting curve for all sets of (λ1,λ2) values.
Figure 4 also shows that the model, Eqs (2.12)–(2.14), with the parameter values determined in the previous subsections gives strong cytotoxic function that cannot well fit the in vivo data. The fitting process is repeated using (λ1,λ2)=(1,1) and unknown parameters p3, α3, β3, p6, α6, and β6. It has been found that the result of the fitting is satisfactory, Figure 5 shows the experimental data and the fitting curve, and the parameter values p3=1.87×10−8, α3=1.6×10−5, β3=3.27, p6=2.04×10−3, α6=0.268×10−6, and β6=4343 are determined.
Finally, the blood content of estradiol provided by Wu et al. [66] is used for the periodical function E(t). Let τ=29 be the period of E(t), and ˜E(t), t∈[0,τ) be the function whose graph is shown in Figure 6. Then E(t)=˜E(t−nτ) for t∈[nτ,(n+1)τ).
Using the specific forms for Eqs (2.1)–(2.4) leads to the following equations:
dTdt=T(a+cET1+α1E+β1T2)(1−T/K)−p1TN21+α2T+β2N2 | (2.15) |
−p6T2L1+α6T2+β6L, | (2.16) |
dNdt=eC−fN−p2NT+p3NT1+α3T+β3N, | (2.17) |
dLdt=(p4LN+p5Iα4+IL)(1−L/KL)Tα5+T−dL, | (2.18) |
dCdt=α−βC, | (2.19) |
E(t)=˜E(t−nτ),t∈[nτ,(n+1)τ). | (2.20) |
A simulation study by Nikolopoulou et al. [67] have reported that the IL-2 density is not very dynamic and the equilibrium density of IL-2 is about 2.37×10−8g/L which is also the value for half saturation constant α4. This assumption gives p5=4.14 since p5Iα4+I=2.07. The parameter values are summarized and shown in Table 1.
Parameter | Value | Units | Reference |
a | 0.3 | Day−1 | fitted with data [59] |
c | 1.93×10−6 | L Cell−1 Day−1 pmol−1 | fitted with data [59] |
α1 | 0.507 | L pmol−1 | fitted with data [59] |
β1 | 7.08×10−8 | Cell−1 | fitted with data [59] |
K | 109 | Cell | [27,28,48,49,50,51] |
p1 | 8.7×10−4 | L2 Cell−2 Day−1 | fitted with data [62] |
α2 | 7×106 | Cell−1 | fitted with data [62] |
β2 | 5.4×10−5 | L2 Cell−2 | fitted with data [62] |
β | 6.3×10−3 | Day−1 | [44] |
α | 3.6×107 | Cell L−1 Day−1 | Estimated within bounds [40] |
e | 0.00486 | Day−1 | Estimated within bounds [41] |
f | 0.0693 | Day−1 | Estimated from data [41] |
p2 | 3.42×10−6 | Cell Day−1 | [28,100] |
p3 | 1.87×10−8 | Cell −1 Day−1 | fitted with data [62] |
α3 | 1.6×10−5 | Cell−1 | fitted with data [62] |
β3 | 3.27 | L Cell−1 | fitted with data [62] |
p6 | 2.04×10−3 | L Cell−2 Day−1 | fitted with data [62] |
α6 | 0.268 | Cell−2 | fitted with data [62] |
β6 | 4343 | L Cell−1 | fitted with data [62] |
LN | 2.3×108 | Cell L−1 | Estimated from data [55] |
KL | 8×108 | Cell L−1 | Estimated from data [55] |
p4 | 9×10−5 | Day−1 | Estimated from data [56,55] |
I | 2.3×10−11 | g L−1 | [67,107] |
α4 | 2.3×10−11 | g L−1 | [67,107] |
p5 | 4.14×10−3 | L Cell−2 Day−1 | Estimated from data [56] |
d | 0.41 | Day−1 | Estimated from data [57] |
α5 | 1000 | Cell | Assumed |
In this section, the dynamics of the model, Eqs (2.16)–(2.20), with parameter values shown in Table 1 are to be studied. For the tumor free case, Eqs (2.16)–(2.20) with parameter values shown in Table 1 give that an immune system has equilibrium levels of NK cell population and WBC population are 4×108 cells/L and 5.7×109 cells/L, respectively.
Vacca et al. have reported that the immune system is able to eliminate tumors at initial stages [68], and it has been demonstrated that most tumor cells entering the circulation are destroyed during the first 24 hours [69,70,71]. In the first numerical experiment, an initial tumor burden of 106 cells, T(0)=106, is considered. In this case, it is assumed that the tumor has escaped immune surveillance and grown to reach a population level of 106 cells. Note that a tumor of 106 cells has a size about 2 mm in diameter and is clinically undetectable. Furthermore, assume that this is the first immune response against the cancer so no effector CTL is present initially. That is L(0)=0. While the activation of CTLs requires some time, Figure 7(a) shows that NK cells provide the first line of defense against cancer and are able to quickly eliminate the tumor.
Suppose that the immune system fails to become activated against a tumor so the tumor grows to a population level of 2×106 cells. A tumor of 2×106 cells has a size of less than 3 mm in diameter. Figure 7(b) shows that a healthy immune system is able to control but not able to completely eliminate this tumor. The size of the tumor decreases to a population level of about 6×105 cells and has a size of less than 2 mm in diameter. Then the tumor remains small and stable. Evidence suggests that many microscopic tumors never progress to become invasive, a phenomenon termed "cancer without disease" [16,17,18,72]. At this stage, a balance between immune response and tumor growth could result in long-term persistence of tumor dormancy. Figure 7(c) shows that a healthy immune system is not able to control a tumor of 4×106 cells, which has a size of 3.2 mm in diameter. The tumor grows to its carrying capacity eventually. The NK cell population remains at a low population level after the tumor reaches its carrying capacity.
Clinical and experimental observations have demonstrated the existence of immunosurveillance process that can destroy cancer cells or prevent primary tumor growth. The cancer immunoediting hypothesis has been developed based on these findings. Cancer immunoediting, which describes the relation between the tumor cells and the immune system, is composed of elimination, equilibrium, and escape phases [73,74]. Elimination is refered to as classical concept of immunosurveillance. Equilibrium occurs when the immune system fails to completely eliminate the tumor but is able to control the tumor leading to long term dormancy. Escape refers to the tumor outgrowth. Figs. 7(a)-(c) show that the system has three locally stable equilibria: the tumor free equilibrium, the equilibrium representing a microscopic tumor, and the equilibrium representing a large tumor.
During the contraction phase, 5-10% of CTLs differentiate into memory T cells [56]. Memory T cells can be immediately activated and trigger the second immune response when the individual is exposed to the same pathogen the next time. Figs. 7(b) and (c) shows that the CTL population reaches a high level of 6.4×108 cells/L after the expansion phase. Assume T(0)=4×107 which is about 6% of the CTL population at the peak level. Figure 7(d) shows that the second immune response is able to eliminate a tumor of 2×106 cells which is larger than the tumor that an immune system can eliminate in the first response. A tumor of 4×106 cells grows to its carrying capacity during the second response (the graph is not shown). Note that although the solutions shown in Figs. 7(a)-(c) appear to approach equilibria, these solutions oscillate with very small amplitudes since E(t) is periodic. These periodic solutions are referred to as equilibria throughout the paper for convenience because their oscillation amplitudes are too small to be seen.
Recall that the parameter values fitted to the in vitro data, Figure 3, gives relatively strong cytotoxicity of CTLs against tumor cells compared to the in vivo data. Tumors may secrete molecules that weaken and inhibit CTL functions [75] or express molecules that directly inhibit the killing ability of CTLs such as immune checkpoint molecules CD80 or PD-L1 [76]. Adoptive cell transfer (ACT) therapy can produce highly active CTLs which give strong immune response [77]. Immune checkpoint therapy using checkpoint inhibitor antibodies, such as anti PD-1, anti PD-L1, or anti CTLA-4, can overcome tumor-induced immune dysfunction and enhance immune response against cancer [78].
The parameter p6, which is related to the killing activity of CTLs, can be used as an indicator of the strength of cytotoxicity of CTLs. Let p6=4×10−3 which is twice the cytotoxic strength used in Section 3.1. Figure 8(a) shows that the immune system is able to eliminate a tumor of 4×106 cells. When the immune system detects the presence of the tumor, the NK cells fight against the tumor cells during the activation phase, where CTLs are at a low population level. The tumor continues to grow during the activation and expansion phases. After the expansion phase, CTLs are able to control the growth of the tumor and then eliminate the tumor. It is followed by a contraction phase at the end of the immune response. Figure 8(b) shows this immune system is able to eliminate a tumor of 3×107 cells, which has a size of 6 mm in diameter, when p6=2.04×10−2. The population level of NK cells decreases initially when the immune system is fighting against the tumor. After the tumor has been killed, the immune system starts to recover and strengthen.
The dynamical system, Eqs (2.16)–(2.20), with parameter values listed in Table 1 exhibits multistability. The asymptotic behavior of a solution depends on its initial conditions, which are the initial level of tumor cell population T(0), the initial NK cell count N(0), the initial NK cell count N(0), and the initial WBC count, C(0). Lymphopenia is the condition of having an abnormally low lymphocyte count in the bloodstream. Ebbo et al. [79] have studied the impact of low circulating NK cell counts and found a correlation between NK cell lymphopenia and invasive bacterial infections. Patients with severe NK cell lymphopenia have higher incidence of invasive bacterial infections. Although the experimental data in their study have shown negative association between cancer risk and the NK cell count, the correlation is not significant.
An individual with severe or mild NK cell lymphopenia has an NK cell count of <5×107cells/L or 5−9×107cells/L, respectively [79]. In a cohort of 457 common variable immunodeficiency patients, 21.7% of the patients have severe NK cell lymphopenia, 25.8% of the patients have mild NK cell lymphopenia, and 52.5% of the patients have normal NK cell counts. In a cohort of 145 healthy adults, 5% of theses healthy controls have NK cell counts below 63×106 NK cells/L (Figure S1 of [79]) indicating that a healthy individual may have severe NK cell lymphopenia. Suppose that a low NK cell count is temporary. The case of persistent lymphopenia will be discussed in the next subsection. Figure 9(a) shows that an individual with mild NK cell lymphopenia, where N(0)=8×107, can rapidly eliminate a tumor of 2×105 cells, which has a size of 1 mm in diameter. Figure 9(b) shows that the immune system is able to control a tumor of 106 cells but is not able to completely eliminate the tumor which a heathy immune system can effectively eliminate.
When an individual suffers from severe NK cell lymphopenia, where N(0)=2×107, Figure 9(c) show that the immune system is able to eliminate a tumor of 1 mm in diameter after the CTL population is expanded. Figure 9(a)–(c) show that an individual with severe or mild NK cell lymphopenia can eliminate a tumor of 2×105 cells but is not able to completely eliminate a tumor of 106 cells which a heathy immune system can effectively eliminate.
Low WBC counts weaken the immune system and increase the risk of bacteria and virus infection. Some studies have suggested correlation between WBC count and coronary heart disease, stroke, and cancer mortality [80,81,82]. Other studies have reported that impact of the WBC count on cardiovascular disease is inconsistent [83,84]. Clinical studies have found that a weakened immune system may increase risk of new cases of cancer in immunocompromised populations [11,85,86,87]. Jee et al. [80] have shown that patients with WBC counts of <5×109 have higher cancer mortality than patients with WBC counts of 5−8×109 do. Nevertheless Kim et al. [11] have reported that the association between cancer risk and WBC count is inconsistent.
Conditions, such as infectious diseases, chemotherapy, drugs, anemia, splenic dysplasia, birth disorders, or damaging the bone marrow can lead to a low WBC count [88]. In this subsection, a system with an eqilibrium WBC count and an eqilibrium NK cell count lower than the normal ranges is considered. The parameter α is related to the production rate of while blood cells. A lower production rate of WBCs corresponds to a smaller α value. An individual with α=1.575×107 has an equilibrium WBC count of α/β=2.5×109, where clinical studies consider 2.5×109 cells/L as a low WBC count [89,90,91,92]. Let e=8.32×10−4, and the equilibrium NK cell count in the absence of a tumor becomes 5×107 cells/L which is lower than the normal values. Furthermore, let p4=3×10−5 indicating a lower activation in CTL immune response. All other parameter values are listed in Table 1. Figure 10 shows the ability of an immune system, with a low WBC count and a low NK cell count, to fight a cancer. The immune system is able to effectively eliminate a tumor of 2×105 cells as shown in Figure 10(a). Figure 10(b) shows that the immune system is able to control a tumor with an initial population level of 106 cells, and Figure 10(c) shows that a tumor with an initial population level of 2×106 cells will eventually grow to its carrying capacity.
Experimental and clinical data support that circulating estradiol is positively associated with breast cancer risk in postmenopausal women [93,94,95,96]. However, in premenopausal women, most prospective studies found no significant association between breast cancer risk and estrogens [96,97,98,99]. The numerical simulation using parameter values in Table 1 with a 10-fold increase in the circulating estradiol level shows that the immune system is able to effectively eliminate a tumor of 106 cells and control a tumor of 2×106 cells. A tumor of 4×106 cells grows to its carrying capacity. In the case of a 100-fold increase in the circulating estradiol level, the numerical simulation shows that the immune response against cancer is similar to the case of a 10-fold increase. Figures of numerical simulation in this subsection are not shown because the figures resemble those that appear in the previous subsections. The numerical results suggest that an increase in the circulating estradiol level does not show a significant increase in cancer risk.
A review of previously published mathematical models of tumor-immune interactions [20] has pointed out that most of these studies present the results derived from mathematical models and very few experimental or clinical results have been compared with these results obtained with mathematical models. Moreover, many of these models have not considered a specific cancer type or justified the functional forms using clinical or experimental data. In this paper, a mathematical model for the growth of breast tumors of MCF-7 cell line is constructed. All the parameter values (except for p2, β, and α5) as well as the forms of functional responses for the effect of estradiol, tumor lysis by NK cells, and recruitment for NK cells have been estimated using experimental data [40,41,45,46,55,56,57,59,60,61,62,63,64,66]. The parameter values for the mean inactivation rate for NK cells p2 and the death rate of WBCs β can be found in the study by de Pillis et al. [28,44,100]. The results of the fitting procedures are shown in Figures 1–6.
Numerical simulation shows that a healthy normal immune system exhibits multistability where the tumor free, microscopic tumor, and large tumor equilibria are stable. The phenomena resemble the 3 E's of cancer immunoediting [73,74]. The immune system is able to rapidly eliminate a tumor with a restricted initial size and the critical size of a tumor that the immune system can eliminate is about 2 mm in diameter (Figure 7). Several experimental studies have demonstrated that NK cells can rapidly eliminate a tumor at initial stages [68,101,102,103]. A healthy normal immune system may also produce long-term dormancy (Figure 7(b)). The tumor does not grow to become large or lethal and remains at an equilibrium size of less than 2 mm in diameter. This phenomenon has been widely observed in clinical studies [16,17,18,72]. Clinical and experimental studies have suggested that an immune system may increase its antitumor effect by enhancing the cytotoxicity of CTLs using ACT therapy, tumor vaccines, or checkpoint antibodies [104,105,106]. Numerical simulation shows that an immune system with a stronger killing activity, a larger p6 value, can eliminate a larger tumor (Figure 8).
An individual suffering from temporary NK cell lymphopenia has a low NK cell count. Numerical simulation demonstrates that there is a positive association between the size of a tumor which an immune system is able to effectively eliminate and the initial NK cell count. Although an immune system with NK cell lymphopenia is not able to effectively eliminate a tumor that a healthy normal immune system is able to (Figure 7(b)), it can rapidly eliminate a smaller tumor (Figure 9(a)). This also suggests that a lower NK cell count may increase cancer risk but the relationship is not significant. Clinical data [79] have shown a similar result that the group with lower NK cell counts has higher tumor incidence rate but the association is not significant.
Immune system strength is important in determining whether or not an immune system is able to eliminate a small tumor. Leukopenia, a low WBC count, is associated with several clinical disorders [88]. Numerical simulation shows that a weakened immune system with persistently low NK cell and WBC counts and a slower activation of CTL immune response may fail to control the growth of a tumor which a healthy immune system is able to control (Figures 7(b) and 10(c)). Finally, numerical simulation also shows that the effect of the estradiol concentration E2 on formation of a tumor is not significant which agrees with observations by previous research studies [96,97,98,99].
This research work was supported by the Ministry of Science and Technology under the grant MOST107-2115-M-035-006.
The author declare there is no conflict of interest.
[1] | D. R. Jutagir, B. B. Blomberg, C. S. Carver, et al., Social well-being is associated with less pro-inflammatory and pro-metastatic leukocyte gene expression in women after surgery for breast cancer, Breast Cancer Res. Treat., 165 (2017), 169–180. |
[2] | S. Katkuri and M. Gorantla, Awareness about breast cancer among women aged 15 years and above in urban slums: a cross sectional study, Int. J. Community Med. Public Health, 5 (2018), 929–932. |
[3] | A. Pawlik, M. Slomi´ nska-Wojewódzka and A. Herman-Antosiewicz, Sensitization of estrogen receptor-positive breast cancer cell lines to 4-hydroxytamoxifen by isothiocyanates present in cruciferous plants, Eur. J. Nutr., 55 (2016), 1165–1180. |
[4] | D. L. Holliday and V. Speirs, Choosing the right cell line for breast cancer research, Breast Cancer Res., 13 (2011), 215–215. |
[5] | R. L. Sutherland, R. E. Hall and I. W. Taylor, Cell proliferation kinetics of MCF-7 human mammary carcinoma cells in culture and effects of tamoxifen on exponentially growing and plateau-phase cells, Cancer Res., 43 (1983), 3998–4006. |
[6] | B. S. Katzenellenbogen, K. L. Kendra, M. J. Norman, et al., Proliferation, hormonal responsiveness, and estrogen receptor content of MCF-7 human breast cancer cells grown in the short-term and long-term absence of estrogens, Cancer Res., 47(1987), 4355–4360. |
[7] | A. Maton, Human biology and health, 1st edition, Prentice Hall, New Jersey, 1997. |
[8] | L. V. Rao, B. A. Ekberg, D. Connor, et al., Evaluation of a new point of care automated complete blood count (CBC) analyzer in various clinical settings, Clin. Chim. Acta., 389 (2008), 120–125. |
[9] | S. Bernard, E. Abdelsamad, P. Johnson, et al., Pediatric leukemia: diagnosis to treatment a review, J. Cancer Clin. Trials, 2(2017), 131. |
[10] | A. Shankar, J. J. Wang, E. Rochtchina, et al., Association between circulating white blood cell count and cancer mortality: a population-based cohort study, Arch. Intern. Med., 166 (2006), 188–194. |
[11] | K. Kim, J. Lee, N. J. Heo, et al., Differential white blood cell count and all-cause mortality in the Korean elderly, Exp. Gerontol., 48 (2013), 103–108. |
[12] | C. Ruggiero, E. J. Metter, A. Cherubini, et al., White blood cell count and mortality in the Baltimore Longitudinal Study of Aging, J. Am. Coll. Cardiol., 49 (2007), 1841–1850. |
[13] | D. S. Bell and J. H. O'Keefe, White cell count, mortality, and metabolic syndrome in the Baltimore longitudinal study of aging, J. Am. Coll. Cardiol., 50(2007), 1810. |
[14] | G. D. Friedman and B. H. Fireman, The leukocyte count and cancer mortality, Am. J. Epidemiol., 133 (1991), 376–380. |
[15] | M. H. Andersen, D. Schrama, P. thor Straten, et al., Cytotoxic T cells, J. Invest. Dermatol., 126 (2006), 32–41. |
[16] | J. Folkman and R. Kalluri, Cancer without disease, Nature, 427 (2004), 787. |
[17] | T. Fehm, V. Mueller, R. Marches, et al., Tumor cell dormancy: implications for the biology and treatment of breast cancer, Apmis, 116 (2008), 742–753. |
[18] | N. Almog, Molecular mechanisms underlying tumor dormancy, Cancer Lett., 294 (2010), 139–146. |
[19] | A. Friedman, Cancer as multifaceted disease, Math. Model. Nat. Pheno., 7(2012), 3–28. |
[20] | R. Eftimie, J. L. Bramson and D. J. Earn, Interactions between the immune system and cancer: a brief review of non-spatial mathematical models, Bull. Math. Biol., 73 (2011), 2–32. |
[21] | S. Banerjee and R. R. Sarkar, Delay-induced model for tumor–immune interaction and control of malignant tumor growth, Biosystems, 91 (2008), 268–288. |
[22] | H. Moore and N. K. Li, A mathematical model for chronic myelogenous leukemia (CML) and T cell interaction, J. Theor. Biol., 227 (2004), 513–523. |
[23] | L. Anderson, S. Jang and J. Yu, Qualitative behavior of systems of tumor-CD4+-cytokine interactions with treatments, Math. Method. Appl. Sci., 38 (2015), 4330–4344. |
[24] | A. d'Onofrio, Metamodeling tumor–immune system interaction, tumor evasion and immunotherapy, Math. Comput. Model., 47 (2008), 614–637. |
[25] | A. Khar, Mechanisms involved in natural killer cell mediated target cell death leading to spontaneous tumour regression, J. Biosci., 22 (1997), 23–31. |
[26] | T. Boon and P. van der Bruggen, Human tumor antigens recognized by T lymphocytes, J. Exp. Med., 183 (1996), 725–729. |
[27] | D. Kirschner and J. C. Panetta, Modeling immunotherapy of the tumor–immune interaction, J. Math. Biol., 37 (1998), 235–252. |
[28] | L. G. de Pillis, W. Gu and A. E. Radunskaya, Mixed immunotherapy and chemotherapy of tumors: modeling, applications and biological interpretations, J. Theor. Biol., 238 (2006), 841–862. |
[29] | H. P. de Vladar and J. A. González, Dynamic response of cancer under the influence of immunological activity and therapy, J. Theor. Biol., 227 (2004), 335–348. |
[30] | U. Fory´ s, J. Waniewski and P. Zhivkov, Anti-tumor immunity and tumor anti-immunity in a mathematical model of tumor immunotherapy, J. Biol. Syst., 14 (2006), 13–30. |
[31] | R. W. De Boer, J. M. Karemaker and J. Strackee, Relationships between short-term blood-pressure fluctuations and heart-rate variability in resting subjects I: a spectral analysis approach, Med. Biol. Eng. Comput., 23 (1985), 352–358. |
[32] | A. Cappuccio, M. Elishmereni and Z. Agur, Cancer immunotherapy by interleukin-21: potential treatment strategies evaluated in a mathematical model, Cancer Res., 66 (2006), 7293–7300. |
[33] | N. Kronik, Y. Kogan, V. Vainstein, et al., Improving alloreactive CTL immunotherapy for malignant gliomas using a simulation model of their interactive dynamics, Cancer Immunol. Immunother., 57 (2008), 425–439. |
[34] | A. M. Jarrett, J. M. Bloom, W. Godfrey, et al., Mathematical modelling of trastuzumab-induced immune response in an in vivo murine model of HER2+ breast cancer, Math. Med. Biol., (2018), dqy014. |
[35] | K. Annan, M. Nagel and H. A. Brock, A mathematical model of breast cancer and mediated immune system interactions, J. Math. Syst. Sci., 2(2012), 430–446. |
[36] | R. Roe-Dale, D. Isaacson and M. Kupferschmid, A mathematical model of breast cancer treatment with CMF and doxorubicin, Bull. Math. Biol., 73 (2011), 585–608. |
[37] | B. Ribba, N. H. Holford, P. Magni, et al., A review of mixed-effects models of tumor growth and effects of anticancer drug treatment used in population analysis, CPT Pharmacometrics Syst. Pharmacol., 3(2014), 1–10. |
[38] | R. Bhat and C. Watzl, Serial killing of tumor cells by human natural killer cells–enhancement by therapeutic antibodies, PloS One, 2 (2007), e326. |
[39] | T. Sutlu and E. Alici, Natural killer cell-based immunotherapy in cancer: current insights and future prospects, J. Intern. Med., 266 (2009), 154–181. |
[40] | T. R. Stravitz, T. Lisman, V. A. Luketic, et al., Minimal effects of acute liver injury/acute liver failure on hemostasis as assessed by thromboelastography, J. Hepatol., 56 (2012), 129–136. |
[41] | Y. Zhang, D. L. Wallace, C. M. De Lara, et al., In vivo kinetics of human natural killer cells: the effects of ageing and acute and chronic viral infection, Immunotherapy, 121(2007), 258–265. |
[42] | P. Wilding, L. J. Kricka, J. Cheng, et al., Integrated cell isolation and polymerase chain reaction analysis using silicon microfilter chambers, Anal. Biochem., 257(1998), 95–100. |
[43] | V. Pascal, N. Schleinitz, C. Brunet, et al., Comparative analysis of NK cell subset distribution in normal and lymphoproliferative disease of granular lymphocyte conditions, Eur. J. Immunol., 34(2004), 2930–2940. |
[44] | L. de Pillis, T. Caldwell, E. Sarapata, et al., Mathematical modeling of the regulatory T cell effects on renal cell carcinoma treatment, Discrete Continuous Dyn. Syst. Ser. B, 18(2013), 915–943. |
[45] | T. D. To, A. T. T. Truong, A. T. Nguyen, et al., Filtration of circulating tumour cells MCF-7 in whole blood using non-modified and modified silicon nitride microsieves, Int. J. Nanotechnol., 15(2018), 39–52. |
[46] | C. Chen, Y. Chen, D. Yao, et al., Centrifugalfilter device for detection of rare cells with immuno-binding, IEEE T. Nanobiosci., 14(2015), 864–869. |
[47] | P. Dua, V. Dua and E. N. Pistikopoulos, Optimal delivery of chemotherapeutic agents in cancer, Comput. Chem. Eng., 32(2008), 99–107. |
[48] | A. G. López, J. M. Seoane and M. A. Sanjuán, A validated mathematical model of tumor growth including tumor–host interaction, cell-mediated immune response and chemotherap, Bull. Math. Biol., 76(2014), 2884–2906. |
[49] | K. Liao, X. Bai and A. Friedman, The role of CD200–CD200R in tumor immune evasion, J. Theor. Biol., 328(2013), 65–76. |
[50] | M. C. Martins, A. M. A. Rocha, M. F. P. Costa, et al., Comparing immune-tumor growth models with drug therapy using optimal control, AIP Conf. Proc., 1738(2016), 300005. |
[51] | M. Fernandez, M. Zhou and L. Soto-Ortiz, A computational assessment of the robustness of cancer treatments with respect to immune response strength, tumor size and resistance, Int. J. Tumor Ther., 7(2018), 1–26. |
[52] | D. F. Tough and J. Sprent, Life span of naive and memory T cells, Stem Cells, 13(1995), 242–249. |
[53] | C. M. Rollings, L. V. Sinclair, H. J. M. Brady, et al., Interleukin-2 shapes the cytotoxic T cell proteome and immune environment–sensing programs, Sci. Signal., 11(2018), eaap8112. |
[54] | E. M. Janssen, E. E. Lemmens, T. Wolfe, et al., CD4+ T cells are required for secondary expansion and memory in CD8+ T lymphocytes, Nature, 421(2003), 852. |
[55] | I. Gruber, N. Landenberger, A. Staebler, et al., Relationship between circulating tumor cells and peripheral T-cells in patients with primary breast cancer, Anticancer Res., 33(2013), 2233–2238. |
[56] | D. Homann, L. Teyton and M. B. Oldstone, Differential regulation of antiviral T-cell immunity results in stable CD8+ but declining CD4+ T-cell memory, Nat. Med., 7(2001), 913–919. |
[57] | R. J. De Boer, D. Homann and A. S. Perelson, Different dynamics of CD4+ and CD8+ T cell responses during and after acute lymphocytic choriomeningitis virus infection, J. Immunol., 171(2003), 3928–3935. |
[58] | G. T. Skalski and J. F. Gilliam, Functional responses with predator interference: viable alternatives to the Holling type II model, Ecology, 82(2001), 3083–3092. |
[59] | H. Nawata, M. T. Chong, D. Bronzert, et al., Estradiol-independent growth of a subline of MCF-7 human breast cancer cells in culture, J. Biol. Chem., 256(1981), 6895–6902. |
[60] | R. Clarke, N. Brünner, B. S. Katzenellenbogen, et al., Progression of human breast cancer cells from hormone-dependent to hormone-independent growth both in vitro and in vivo, Proc. Natl. Acad. Sci. USA, 86(1989), 3649–3653. |
[61] | N. T. Telang, G. Li, M. Katdare, et al., The nutritional herb Epimedium grandiflorum inhibits the growth in a model for the Luminal A molecular subtype of breast cancer, Oncol. Lett., 13 (2017), 2477–2482. |
[62] | T. A. Caragine, M. Imai, A. B. Frey, et al., Expression of rat complement control protein Crry on tumor cells inhibits rat natural killer cell–mediated cytotoxicity, Blood, 100 (2002), 3304–3310. |
[63] | M. R. Müller, F. Grünebach, A. Nencioni, et al., Transfection of dendritic cells with RNA induces CD4-and CD8-mediated T cell immunity against breast carcinomas and reveals the immunodominance of presented T cell epitopes, J. Immunol., 170 (2003), 5892–5896. |
[64] | J. Chen, E. Hui, T. Ip, et al., Dietary flaxseed enhances the inhibitory effect of tamoxifen on the growth of estrogen-dependent human breast cancer (mcf-7) in nude mice, Clin. Cancer Res., 10(2004), 7703–7711. |
[65] | P. V. Sivakumar, R. Garcia, K. S. Waggie, et al., Comparison of vascular leak syndrome in mice treated with IL21 or IL2, Comparative Med., 63 (2013), 13–21. |
[66] | C.Wu, T.Motosha, H.A.Abdel-Rahman, etal., Freeandprotein-boundplasmaestradiol-17during the menstrual cycle, J. Clin. Endocrinol. Metab., 43(1976), 436–445. |
[67] | E. Nikolopoulou, L. R. Johnson, D. Harris, et al., Tumour-immune dynamics with an immune checkpoint inhibitor, Lett. Biomath., 5(2018), S137–S159. |
[68] | P. Vacca, E. Munari, N. Tumino, et al., Human natural killer cells and other innate lymphoid cells in cancer: friends or foes? Immunol. Lett., 201(2018), 14–19. |
[69] | A. Cerwenka, J. Kopitz, P. Schirmacher, et al., HMGB1: the metabolic weapon in the arsenal of NK cells, Mol. Cell. Oncol., 3(2016), e1175538. |
[70] | I. J. Fidler, Metastasis: quantitative analysis of distribution and fate of tumor emboli labeled with 125i-5-iodo-2'-deoxyuridine, J. Natl. Cancer Inst., 4(1970), 773–782. |
[71] | G. G. Page and S. Ben-Eliyahu, A role for NK cells in greater susceptibility of young rats to metastatic formation, Dev. Comp. Immunol., 23(1999), 87–96. |
[72] | O. E. Franco, A. K. Shaw, D. W. Strand, et al., Cancer associated fibroblasts in cancer pathogenesis, Semin. Cell Dev. Bio., 21(2010), 33–39. |
[73] | G. P. Dunn, A. T. Bruce, H. Ikeda, et al., Cancer immunoediting: from immunosurveillance to tumor escape, Nat. Immunol., 3(2002), 991. |
[74] | D. Mittal, M. M. Gubin, R. D. Schreiber, et al., New insights into cancer immunoediting and its three component phases-elimination, equilibrium and escape, Curr. Opin. Immunol., 27(2014), 16–25. |
[75] | J. Nowak, P. Juszczynski and K. Warzocha, The role of major histocompatibility complex polymorphisms in the incidence and outcome of non-Hodgkin lymphoma, Curr. Immunol. Rev., 5(2009), 300–310. |
[76] | J. G. B. Alvarez, M. González-Cao, N. Karachaliou, et al., Advances in immunotherapy for treatment of lung cancer, Cancer Biol. Med., 12(2015), 209–222. |
[77] | M. E. Dudley and S. A. Rosenberg, Adoptive-cell-transfer therapy for the treatment of patients with cancer, Nat. Rev. Cancer, 3(2003), 666–675. |
[78] | M. Su, C. Huang and A. Dai, Immune checkpoint inhibitors: therapeutic tools for breast cancer, Asian Pac. J. Cancer Prev., 17 (2016), 905–910. |
[79] | M. Ebbo, L. Gérard, S. Carpentier, et al., Low circulating natural killer cell counts are associated with severe disease in patients with common variable immunodeficiency, EBioMedicine., 6(2016), 222–230. |
[80] | S. H. Jee, J. Y. Park, H. Kim, et al., White blood cell count and risk for all-cause, cardiovascular, and cancer mortality in a cohort of Koreans, Am. J. Epidemiol., 162 (2005), 1062–1069. |
[81] | W. B. Kannel, K. Anderson and T. W. Wilson, White blood cell count and cardiovascular disease: insights from the Framingham Study, Jama, 267 (1992), 1253–1256. |
[82] | K. L. Margolis, J. E. Manson, P. Greenland, et al., Leukocyte count as a predictor of cardiovascular events and mortality in postmenopausal women: the Women's Health Initiative Observational Study, Arch. Intern. Med., 165(2005), 500–508. |
[83] | B. K. Duffy, H. S. Gurm, V. Rajagopal, et al., Usefulness of an elevated neutrophil to lymphocyte ratio in predicting long-term mortality after percutaneous coronary intervention, Am. J. Cardiol., 97 (2006), 993–996. |
[84] | B. D. Horne, J. L. Anderson, J. M. John, et al., Which white blood cell subtypes predict increased cardiovascular risk? J. Am. Coll. Cardiol., 45(2005), 1638–1643. |
[85] | R. N. O. Cobucci, H. Saconato, P. H. Lima, et al., Comparative incidence of cancer in HIV-AIDS patients and transplant recipients, Cancer Epidemiol., 36(2012), e69–e73. |
[86] | C. Bodelon, M. M. Madeleine, L. F. Voigt, et al., Is the incidence of invasive vulvar cancer increasing in the United States? Cancer Causes Control, 20(2009), 1779–1782. |
[87] | M. R. Shurin, Cancer as an immune-mediated disease, Immunotargets Ther., 1 (2012), 1–6. |
[88] | J. S. Lawrence, Leukopenia: its mechanism and therapy, J. Chronic. Dis., 6(1957), 351–364. |
[89] | P. Venigalla, B. Motwani, A. Nallari, et al., A patient on hydroxyurea for sickle cell disease who developed an opportunistic infection, Blood, 100(2002), 363–364. |
[90] | M. Iwamuro, S. Tanaka, A. Bessho, et al., Two cases of primary small cell carcinoma of the stomach, Acta. Med. Okayama, 63(2009), 293–298. |
[91] | A. O. O. Chan, I. O. L. Ng, C. M. Lam, et al., Cholestatic jaundice caused by sequential carbimazole and propylthiouracil treatment for thyrotoxicosis, Hong Kong Med. J., 9(2003), 377–380. |
[92] | J. H. Goodchild and M. Glick, A different approach to medical risk assessment, Endod. Topics, 4(2003), 1–8. |
[93] | S. E. Hankinson, Endogenous hormones and risk of breast cancer in postmenopausal women, Breast Dis., 24(2006), 3–15. |
[94] | R. Kaaks, S. Rinaldi, T. J. Key, et al., Postmenopausal serum androgens, oestrogens and breast cancer risk: the European prospective investigation into cancer and nutrition, Endocr. Relat. Cancer, 12(2005), 1071–1082. |
[95] | S. A. Missmer, A. H. Eliassen, R. L. Barbieri, et al., Endogenous estrogen, androgen, and progesterone concentrations and breast cancer risk among postmenopausal women, J. Natl. Cancer Inst., 96(2004), 1856–1865. |
[96] | A. A. Arslan, R. E. Shore, Y. Afanasyeva, et al., Circulating estrogen metabolites and risk for breast cancer in premenopausal women, Cancer Epidemiol. Biomarkers Prev., 18(2009), 2273–2279. |
[97] | S. B. Brown and S. E. Hankinson, Endogenous estrogens and the risk of breast, endometrial, and ovarian cancers, Steroids, 99(2015), 8–10. |
[98] | L. C. Houghton, D. Ganmaa, P. S. Rosenberg, et al., Associations of breast cancer risk factors with premenopausal sex hormones in women with very low breast cancer risk, Int. J. Environ. Res. Public Health, 13(2016), 1066. |
[99] | R. Kaaks, K. Tikk, D. Sookthai, et al., Premenopausal serum sex hormone levels in relation to breast cancer risk, overall and by hormone receptor status-results from the EPIC cohortk, Int. J. cancer, 134(2014), 1947–1957. |
[100] | A. Diefenbach, E. R. Jensen, A. M. Jamieson, et al., Rae1 and H60 ligands of the NKG2D receptor stimulate tumour immunity, Nature, 413(2001), 165. |
[101] | A. Iannello and D. H. Raulet, Cold Spring Harbor symposia on quantitative biology, 1st edition, Cold Spring Harbor Laboratory Press, New York, 2013. |
[102] | M. B. Pampena and E. M. Levy, Natural killer cells as helper cells in dendritic cell cancer vaccines, Front. Immunol., 6 (2015), 1–8. |
[103] | E. Vivier, S. Ugolini, D. Blaise, et al., Targeting natural killer cells and natural killer T cells in cancer, Nat. Rev. Immunol., 12(2012), 239–252. |
[104] | G. Liu, X. Fan, Y. Cai, et al., Efficacy of dendritic cell-based immunotherapy produced from cord blood in vitro and in a humanized NSG mouse cancer model, Immunotherapy, 11(2019), 599–616. |
[105] | M. Schnekenburger, M. Dicato and M. F. Diederich, Anticancer potential of naturally occurring immunoepigenetic modulators: A promising avenue? Cancer, 125(2019), 1612–1628. |
[106] | X. Feng, L. Lu, K. Wang, et al., Low expression of CD80 predicts for poor prognosis in patients with gastric adenocarcinoma, Future Oncol., 15 (2019), 473–483. |
[107] | X. Lai and A. Friedman, Combination therapy of cancer with cancer vaccine and immune checkpoint inhibitors: a mathematical model, PloS One, 12 (2017), e0178479. |
1. | Clara Burgos-Simón, Juan-Carlos Cortés, David Martínez-Rodríguez, Rafael J. Villanueva, Modeling breast tumor growth by a randomized logistic model: A computational approach to treat uncertainties via probability densities, 2020, 135, 2190-5444, 10.1140/epjp/s13360-020-00853-3 | |
2. | Muhammad Idrees, Ayesha Sohail, Bio-algorithms for the modeling and simulation of cancer cells and the immune response, 2021, 0, 1896-530X, 10.1515/bams-2020-0054 | |
3. | Regina Padmanabhan, Hadeel Shafeeq Kheraldine, Nader Meskin, Semir Vranic, Ala-Eddin Al Moustafa, Crosstalk between HER2 and PD-1/PD-L1 in Breast Cancer: From Clinical Applications to Mathematical Models, 2020, 12, 2072-6694, 636, 10.3390/cancers12030636 | |
4. | Samuel Ruiz-Arrebola, Damián Guirado, Mercedes Villalobos, Antonio M. Lallena, Evaluation of Classical Mathematical Models of Tumor Growth Using an On-Lattice Agent-Based Monte Carlo Model, 2021, 11, 2076-3417, 5241, 10.3390/app11115241 | |
5. | Fuzhang Wang, M Idrees, Ayesha Sohail, “AI-MCMC” for the parametric analysis of the hormonal therapy of cancer, 2022, 154, 09600779, 111618, 10.1016/j.chaos.2021.111618 | |
6. | Xiaoyun Wen, Yu Chen, Xiansong Fang, Overexpression of HOXD8 inhibits the proliferation, migration and invasion of breast cancer cells by downregulating ILP2 expression, 2021, 22, 1792-0981, 10.3892/etm.2021.10439 | |
7. | Léon Masurel, Carlo Bianca, Annie Lemarchand, Space-velocity thermostatted kinetic theory model of tumor growth, 2021, 18, 1551-0018, 5525, 10.3934/mbe.2021279 | |
8. | Kolade M. Owolabi, Albert Shikongo, Abdon Atangana, 2022, Chapter 13, 978-3-030-77168-3, 319, 10.1007/978-3-030-77169-0_13 | |
9. | Asmaa Amer, Ahmed Nagah, Mojeeb AL-Rahman El-Nor Osman, Abdul Majid, Modeling the pathway of breast cancer in the Middle East, 2021, 0, 2444-8656, 10.2478/amns.2021.2.00050 | |
10. | Hsiu-Chuan Wei, Mathematical modeling of tumor growth and treatment: Triple negative breast cancer, 2023, 204, 03784754, 645, 10.1016/j.matcom.2022.09.005 | |
11. | Muhammad Idrees, Ayesha Sohail, João Manuel R.S. Tavares, Forecasting the stochastic vicious cycle of cancer progression and immune response, 2021, 26, 22113797, 104420, 10.1016/j.rinp.2021.104420 | |
12. | Willie Oliveira Pinheiro, Mayara Simonelly Costa do Santos, Gabriel Ribeiro Farias, Maria Luiza Fascineli, Khellida Loiane Vieira Ramos, Eliza Carla Barroso Duarte, Emanuel Adelino Medeiros Damasceno, Jaqueline Rodrigues da Silva, Graziella Anselmo Joanitti, Ricardo Bentes de Azevedo, Marcelo Henrique Sousa, Zulmira Guerrero Marques Lacava, Ewa Mosiniewicz-Szablewska, Piotr Suchocki, Paulo Cesar Morais, Laise Rodrigues de Andrade, Combination of selol nanocapsules and magnetic hyperthermia hinders breast tumor growth in aged mice after a short-time treatment, 2022, 33, 0957-4484, 205101, 10.1088/1361-6528/ac504c | |
13. | Navid Mohammad Mirzaei, Sumeyye Su, Dilruba Sofia, Maura Hegarty, Mohamed H. Abdel-Rahman, Alireza Asadpoure, Colleen M. Cebulla, Young Hwan Chang, Wenrui Hao, Pamela R. Jackson, Adrian V. Lee, Daniel G. Stover, Zuzana Tatarova, Ioannis K. Zervantonakis, Leili Shahriyari, A Mathematical Model of Breast Tumor Progression Based on Immune Infiltration, 2021, 11, 2075-4426, 1031, 10.3390/jpm11101031 | |
14. | Imane Ait Oumghar, Abdelwahed Barkaoui, Patrick Chabrand, Abdellatif El Ghazi, Charlotte Jeanneau, Daphne Guenoun, Peter Pivonka, Experimental-based mechanobiological modeling of the anabolic and catabolic effects of breast cancer on bone remodeling, 2022, 21, 1617-7959, 1841, 10.1007/s10237-022-01623-z | |
15. | Yan Fu, Tian Lu, Meng Zhou, Dongwei Liu, Qihang Gan, Guowei Wang, Effect of color cross-correlated noise on the growth characteristics of tumor cells under immune surveillance, 2023, 20, 1551-0018, 21626, 10.3934/mbe.2023957 | |
16. | Heng Yang, Haofeng Lin, Xiaoqiang Sun, Multiscale modeling of drug resistance in glioblastoma with gene mutations and angiogenesis, 2023, 21, 20010370, 5285, 10.1016/j.csbj.2023.10.037 | |
17. | Sana Abdulkream Alharbi, Kaushik Dehingia, Awatif Jahman Alqarni, Mona Alsulami, AA Al Qarni, Anusmita Das, Evren Hinçal, A study on ODE-based model of risk breast cancer with body mass, 2023, 31, 2769-0911, 10.1080/27690911.2023.2259059 | |
18. | Mohammad Bakhshivand, Javad Masoumi, Farid Ghorbaninezhad, Leili Aghebati-Maleki, Dariush Shanebandi, Siamak Sandoghchian Shotorbani, Farhad Jadidi-Niaragh, Amir Baghbanzadeh, Nima Hemmat, Elham Baghbani, Amir Ghaffari, Behzad Baradaran, Boosting immunotherapy efficacy: Empowering the Potency of Dendritic cells loaded with breast cancer lysates through CTLA-4 suppression, 2024, 10, 24058440, e37699, 10.1016/j.heliyon.2024.e37699 | |
19. | Francesca Cialdai, Austin M. Brown, Cory W. Baumann, Debora Angeloni, Sarah Baatout, Alexandra Benchoua, Juergen Bereiter-Hahn, Daniele Bottai, Judith-Irina Buchheim, Marco Calvaruso, Eugénie Carnero-Diaz, Sara Castiglioni, Duccio Cavalieri, Gabriele Ceccarelli, Alexander Choukér, Gianni Ciofani, Giuseppe Coppola, Gabriella Cusella, Andrea Degl’Innocenti, Jean-Francois Desaphy, Jean-Pol Frippiat, Michael Gelinsky, Giada Genchi, Maria Grano, Daniela Grimm, Alain Guignandon, Christiane Hahn, Jason Hatton, Raúl Herranz, Christine E. Hellweg, Carlo Saverio Iorio, Thodoris Karapantsios, Jack van Loon, Matteo Lulli, Jeanette Maier, Jos Malda, Emina Mamaca, Lucia Morbidelli, Angelique van Ombergen, Andreas Osterman, Aleksandr Ovsianikov, Francesco Pampaloni, Elizabeth Pavezlorie, Veronica Pereda-Campos, Cyrille Przybyla, Christopher Puhl, Petra Rettberg, Chiara Risaliti, Angela Maria Rizzo, Kate Robson-Brown, Leonardo Rossi, Giorgio Russo, Alessandra Salvetti, Daniela Santucci, Matthias Sperl, Felice Strollo, Kevin Tabury, Sara Tavella, Christiane Thielemann, Ronnie Willaert, Nathaniel J. Szewczyk, Monica Monici, How do gravity alterations affect animal and human systems at a cellular/tissue level?, 2023, 9, 2373-8065, 10.1038/s41526-023-00330-y | |
20. | Carlo Bianca, A decade of thermostatted kinetic theory models for complex active matter living systems, 2024, 50, 15710645, 72, 10.1016/j.plrev.2024.06.015 | |
21. | Juan C. López-Alvarenga, Antonmaria Minzoni-Alessio, Arturo Olvera-Chávez, Gustavo Cruz-Pacheco, Juan C. Chimal-Eguia, Joselín Hernández-Ruíz, Mario A. Álvarez-Blanco, María Y. Bautista-Hernández, Rosa M. Quispe-Siccha, A Mathematical Model to Optimize the Neoadjuvant Chemotherapy Treatment Sequence for Triple-Negative Locally Advanced Breast Cancer, 2023, 11, 2227-7390, 2410, 10.3390/math11112410 | |
22. | Abeer S. Alnahdi, Muhammad Idrees, Nonlinear dynamics of estrogen receptor-positive breast cancer integrating experimental data: A novel spatial modeling approach, 2023, 20, 1551-0018, 21163, 10.3934/mbe.2023936 | |
23. | Isidora Stanisavljevic, Sladjana Pavlovic, Bojana Simovic Markovic, Milena Jurisevic, Tamara Krajnovic, Sanja Mijatovic, Marija Spasojevic, Slobodanka Mitrovic, Irfan Corovic, Ivan Jovanovic, Semaglutide decelerates the growth and progression of breast cancer by enhancing the acquired antitumor immunity, 2024, 181, 07533322, 117668, 10.1016/j.biopha.2024.117668 | |
24. | Oghenejoboh Ufuoma Modupe, Sonibare Oluwadayo Olatunde, Muhammad Waseem, Ekundayo Olusegun, Sirajul Haq, Ahad Amer Alsaiari, Jamoliddin Razzokov, Green Hydrothermal Synthesis of Nickel and Zinc-Doped Nickel Ferrite Nanoparticles Using Dalbergiella welwitschii Extracts and Their Biological Studies, 2024, 24058440, e40759, 10.1016/j.heliyon.2024.e40759 | |
25. | Safura Absalan, Hamidreza Vaziri, Mahvash Hadavi, Study the Expression of Two Circular RNAs, hsa_circ_0003227 and hsa_circ_0001666, in the Primary Breast Cancer Cell Line BT-20 and the Metastatic Breast Cancer Cell Line MCF-7, 2025, 0006-2928, 10.1007/s10528-025-11051-0 |
Parameter | Value | Units | Reference |
a | 0.3 | Day−1 | fitted with data [59] |
c | 1.93×10−6 | L Cell−1 Day−1 pmol−1 | fitted with data [59] |
α1 | 0.507 | L pmol−1 | fitted with data [59] |
β1 | 7.08×10−8 | Cell−1 | fitted with data [59] |
K | 109 | Cell | [27,28,48,49,50,51] |
p1 | 8.7×10−4 | L2 Cell−2 Day−1 | fitted with data [62] |
α2 | 7×106 | Cell−1 | fitted with data [62] |
β2 | 5.4×10−5 | L2 Cell−2 | fitted with data [62] |
β | 6.3×10−3 | Day−1 | [44] |
α | 3.6×107 | Cell L−1 Day−1 | Estimated within bounds [40] |
e | 0.00486 | Day−1 | Estimated within bounds [41] |
f | 0.0693 | Day−1 | Estimated from data [41] |
p2 | 3.42×10−6 | Cell Day−1 | [28,100] |
p3 | 1.87×10−8 | Cell −1 Day−1 | fitted with data [62] |
α3 | 1.6×10−5 | Cell−1 | fitted with data [62] |
β3 | 3.27 | L Cell−1 | fitted with data [62] |
p6 | 2.04×10−3 | L Cell−2 Day−1 | fitted with data [62] |
α6 | 0.268 | Cell−2 | fitted with data [62] |
β6 | 4343 | L Cell−1 | fitted with data [62] |
LN | 2.3×108 | Cell L−1 | Estimated from data [55] |
KL | 8×108 | Cell L−1 | Estimated from data [55] |
p4 | 9×10−5 | Day−1 | Estimated from data [56,55] |
I | 2.3×10−11 | g L−1 | [67,107] |
α4 | 2.3×10−11 | g L−1 | [67,107] |
p5 | 4.14×10−3 | L Cell−2 Day−1 | Estimated from data [56] |
d | 0.41 | Day−1 | Estimated from data [57] |
α5 | 1000 | Cell | Assumed |
Parameter | Value | Units | Reference |
a | 0.3 | Day−1 | fitted with data [59] |
c | 1.93×10−6 | L Cell−1 Day−1 pmol−1 | fitted with data [59] |
α1 | 0.507 | L pmol−1 | fitted with data [59] |
β1 | 7.08×10−8 | Cell−1 | fitted with data [59] |
K | 109 | Cell | [27,28,48,49,50,51] |
p1 | 8.7×10−4 | L2 Cell−2 Day−1 | fitted with data [62] |
α2 | 7×106 | Cell−1 | fitted with data [62] |
β2 | 5.4×10−5 | L2 Cell−2 | fitted with data [62] |
β | 6.3×10−3 | Day−1 | [44] |
α | 3.6×107 | Cell L−1 Day−1 | Estimated within bounds [40] |
e | 0.00486 | Day−1 | Estimated within bounds [41] |
f | 0.0693 | Day−1 | Estimated from data [41] |
p2 | 3.42×10−6 | Cell Day−1 | [28,100] |
p3 | 1.87×10−8 | Cell −1 Day−1 | fitted with data [62] |
α3 | 1.6×10−5 | Cell−1 | fitted with data [62] |
β3 | 3.27 | L Cell−1 | fitted with data [62] |
p6 | 2.04×10−3 | L Cell−2 Day−1 | fitted with data [62] |
α6 | 0.268 | Cell−2 | fitted with data [62] |
β6 | 4343 | L Cell−1 | fitted with data [62] |
LN | 2.3×108 | Cell L−1 | Estimated from data [55] |
KL | 8×108 | Cell L−1 | Estimated from data [55] |
p4 | 9×10−5 | Day−1 | Estimated from data [56,55] |
I | 2.3×10−11 | g L−1 | [67,107] |
α4 | 2.3×10−11 | g L−1 | [67,107] |
p5 | 4.14×10−3 | L Cell−2 Day−1 | Estimated from data [56] |
d | 0.41 | Day−1 | Estimated from data [57] |
α5 | 1000 | Cell | Assumed |