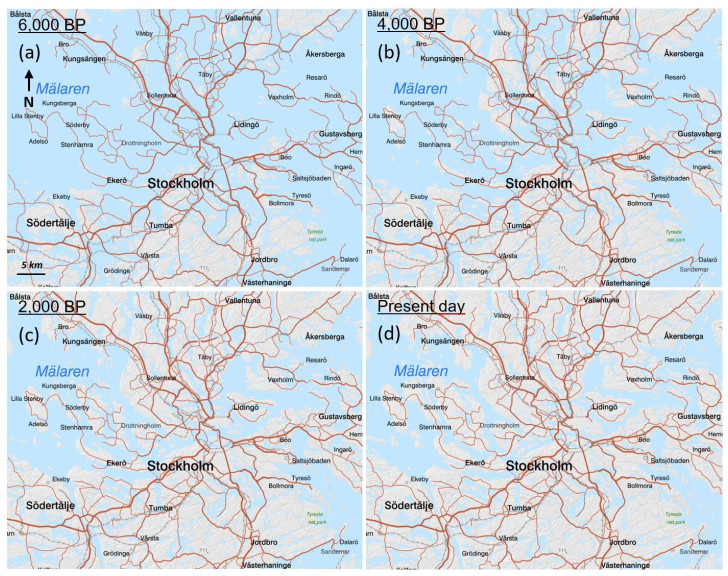
This paper describes the geotechnical characteristics of index and deformation properties of Stockholm clays. These clays exhibit large variations both geographically and with depth. The clays range from highly organic clays with high plasticity to silty clays with low plasticity. First, the geological conditions of the clays are outlined to qualitatively explain the typical soil stratigraphy encountered in the Stockholm area. Second, two large generic databases are presented, containing 3,500 and 1,600 data points, respectively. The data originates from routine testing and constant rate of strain oedometer tests conducted in commercial projects. The data is analyzed and compared with results from high quality block sampling. It is seen that a common feature of the clays are low undrained shear strengths, and consequently low yield stresses and oedometer moduli. Further, several deformation properties such as preconsolidation pressure and several oedometer moduli is shown to depend on the soils natural water content and its plasticity. Differences in sample quality is shown to highly affect some properties, highlighting the importance of quality sampling and handling of samples. Criteria for sample quality for this type of clay is proposed based on the oedometer moduli before and after the preconsolidation pressure. The paper can hopefully work as a useful reference to engineers working on similar soils worldwide.
Citation: Solve Hov, David Gaharia. Geotechnical characterization of index and deformation properties of Stockholm clays[J]. AIMS Geosciences, 2023, 9(2): 258-284. doi: 10.3934/geosci.2023015
[1] | Bruno Di Buò, Marco D’Ignazio, Juha Selänpää, Markus Haikola, Tim Länsivaara, Marta Di Sante . Investigation and geotechnical characterization of Perniö clay, Finland. AIMS Geosciences, 2019, 5(3): 591-616. doi: 10.3934/geosci.2019.3.591 |
[2] | Don J. DeGroot, Tom Lunne, Ravindra Ghanekar, Siren Knudsen, Cody D. Jones, Tor Inge Yetginer-Tjelta . Engineering properties of low to medium overconsolidation ratio offshore clays. AIMS Geosciences, 2019, 5(3): 535-567. doi: 10.3934/geosci.2019.3.535 |
[3] | Priscilla Paniagua, Marco D'Ignazio, Jean-Sébastien L'Heureux, Tom Lunne, Kjell Karlsrud . CPTU correlations for Norwegian clays: an update. AIMS Geosciences, 2019, 5(2): 82-103. doi: 10.3934/geosci.2019.2.82 |
[4] | Marco D'Ignazio, Tom Lunne, Knut. H. Andersen, Shaoli Yang, Bruno Di Buò, Tim Länsivaara . Estimation of preconsolidation stress of clays from piezocone by means of high-quality calibration data. AIMS Geosciences, 2019, 5(2): 104-116. doi: 10.3934/geosci.2019.2.104 |
[5] | Ariane Locat, Pascal Locat, Hubert Michaud, Kevin Hébert, Serge Leroueil, Denis Demers . Geotechnical characterization of the Saint-Jude clay, Quebec, Canada. AIMS Geosciences, 2019, 5(2): 273-302. doi: 10.3934/geosci.2019.2.273 |
[6] | Don J. DeGroot, Melissa E. Landon, Steven E. Poirier . Geology and engineering properties of sensitive Boston Blue Clay at Newbury, Massachusetts. AIMS Geosciences, 2019, 5(3): 412-447. doi: 10.3934/geosci.2019.3.412 |
[7] | Jean-Sébastien L'Heureux, Anders Lindgård, Arnfinn Emdal . The Tiller-Flotten research site: Geotechnical characterization of a very sensitive clay deposit. AIMS Geosciences, 2019, 5(4): 831-867. doi: 10.3934/geosci.2019.4.831 |
[8] | Robert Radaszewski, Jędrzej Wierzbicki . Characterization and engineering properties of AMU Morasko soft clay. AIMS Geosciences, 2019, 5(2): 235-264. doi: 10.3934/geosci.2019.2.235 |
[9] | Tonje Eide Helle, Per Aagaard, Steinar Nordal, Michael Long, Sara Bazin . A geochemical, mineralogical and geotechnical characterization of the low plastic, highly sensitive glaciomarine clay at Dragvoll, Norway. AIMS Geosciences, 2019, 5(4): 704-722. doi: 10.3934/geosci.2019.4.704 |
[10] | Mike Long, Jean Sebastien L’Heureux, Bjørn Kristian Fiskvik Bache, Alf Kristian Lund, Svein Hove, Karl Gunnar Sødal, Helene Alexandra Amundsen, Steinar Nordal, Alberto Montafia . Site characterisation and some examples from large scale testing at the Klett quick clay research site. AIMS Geosciences, 2019, 5(3): 344-389. doi: 10.3934/geosci.2019.3.344 |
This paper describes the geotechnical characteristics of index and deformation properties of Stockholm clays. These clays exhibit large variations both geographically and with depth. The clays range from highly organic clays with high plasticity to silty clays with low plasticity. First, the geological conditions of the clays are outlined to qualitatively explain the typical soil stratigraphy encountered in the Stockholm area. Second, two large generic databases are presented, containing 3,500 and 1,600 data points, respectively. The data originates from routine testing and constant rate of strain oedometer tests conducted in commercial projects. The data is analyzed and compared with results from high quality block sampling. It is seen that a common feature of the clays are low undrained shear strengths, and consequently low yield stresses and oedometer moduli. Further, several deformation properties such as preconsolidation pressure and several oedometer moduli is shown to depend on the soils natural water content and its plasticity. Differences in sample quality is shown to highly affect some properties, highlighting the importance of quality sampling and handling of samples. Criteria for sample quality for this type of clay is proposed based on the oedometer moduli before and after the preconsolidation pressure. The paper can hopefully work as a useful reference to engineers working on similar soils worldwide.
This paper describes the geotechnical characteristics of index and deformation properties of clays in the greater Stockholm area, south-eastern Sweden. The properties of these clays vary greatly geographically and with depth, and ranges from highly organic clays with high plasticity to silty clays with low plasticity.
Clays in the Stockholm area have historically been thoroughly investigated, and the properties of these clays have had a major influence on the development on both field and laboratory tests and ground improvement techniques. Starting in the 1910s, Atterberg [1,2] established the consistency limits, to a large extent based on tests on Stockholm clays, which later was introduced in the international geotechnical community by Casagrande in 1932 [3]. In the 1910s and 1920s, the Swedish Railway Geotechnical Commission performed extensive research, including the development of the Swedish fall cone test [4], later re-developed by Hansbo in the 1950s [5]. After the establishment of the Swedish Geotechnical Institute (SGI) in 1944, extensive research was performed on both sample quality [6,7,8] and development of the in situ vane test [9] among other methods [10,11]. The trial embankments for settlement purposes at Lilla Mellösa and Skå-Edeby, established in 1945 and 1957 [12], respectively, are also located in the Stockholm area. For ground improvement purposes, prefabricated vertical drains in the 1940s, vacuum preloading in the 1950s and dry deep mixing in the 1970s were developed [13].
Despite all these research projects and extensive testing of Stockholm clays, there is no available description of its general engineering properties and the geological preconditions. The previously mentioned research describes the ground conditions at the respective sites in detail; however, since the Stockholm clays exhibit a high spatial variability the conditions do not necessarily apply elsewhere. How, for example, deformation properties depend on the index properties is also by and large unknown. The intent of this paper is thus to present a general characterization of the Stockholm clays based on the results of routine and advanced laboratory tests from a large number of sites. The characterisation covers index properties such as bulk density, natural water content, plasticity and index undrained shear strength and deformation properties from CRS (constant rate of strain) oedometer tests such as preconsolidation pressure, deformation moduli and permeability.
The vast majority of all data presented herein originates from laboratory tests performed in commercial projects. The data is collected in two generic databases containing around 3,500 and 1,600 data points, respectively, originating from around 520 different sites from a geographical area spanning roughly 4,500 km2. These databases are thus big indirect data [14] that is not relevant to one particular site but are nonetheless valuable as it represents the current state of practice. As such, it is however important to consider inherent uncertainties, e.g. spatial variability, measurement errors or correlation uncertainties [15]. For the databases presented herein, which originates from real-life commercial projects, it is important to consider sample quality, i.e. a type of measurement error. A key focus of the paper is therefore to discuss and show effects of sample quality on engineering properties and correlations. Taking this into consideration, the data is valuable to improve the geotechnical engineers understanding of soil behavior and the presented results will form a useful reference to engineers working on similar soils worldwide.
Although statistical analyses are not within the scope of this study, it is shown that several deformation properties such as preconsolidation pressure, oedometer moduli before and after the preconsolidation pressure depends on the soils natural water content and plasticity. Differences in sample quality is shown to highly affect some properties, and criterions for sample quality for this type of clay is proposed. The paper will hopefully contribute to the efforts being made within data-driven site characterisation [16,17].
Stockholm is situated between the Mälaren lake and the Baltic sea. The Scandinavian ice sheet withdrew from the area around 11,000–11,500 years before present day (BP), and the area was then completely submerged by the so-called Baltic ice lake or Yoldia sea [18]. The ice sheet eroded the bedrock and deposited a till layer. Over time, as the ice sheet withdrew, sand and silt were deposited from melting water, whilst the tectonic uplift created more land and several islands which increased in size. An overview of the tectonic uplift and the resulting shorelines over time are shown in Figure 1. As the distance from the ice front increased, the soil deposits became sedimented in an increasingly lower energy environment since coarse particles will deposit close to the river mouth and finer particles will deposit at further distance where the energy is lower. This creates a typical soil stratigraphy from coarse-grained at the bottom to more fine-grained at the top. High areas of bedrock were washed of soil and is now visible rock outcrops up to 40–60 m above sea level in general. As a result, only low-lying areas are covered in clay deposits. A geological map is shown in Figure 2, illustrating the varying soil conditions.
The increasingly lower energy environment over time has created common characteristics of Stockholm clays as illustrated in Figure 3 which shows two typical soil profiles; one without organic deposits and one with organic deposits of peat and gyttja. Figure 4 shows photographs from the varying soil deposits to illustrate the heterogeneity. In general, the grain fraction increases with depth, both with regards to the silt content in the clay fabric and with regards to the frequency and thickness of silt layers, which also includes sand layers varying from a few millimeters to several centimeters in thickness. Underneath the clay there is typically silt and sand deposit on top of the till.
Because of this geological condition, there is typically a general pattern that the water content, plasticity and organic content decreases with depth, whilst its unit weight increases with depth. Due to the topographical characteristics, there have been no considerable alteration of the landscape due to erosion or landslides, and the clay is thus normal to lightly over consolidated due to aging [20]. In urban areas there is also considerable ongoing consolidation settlements, i.e. the clay is normally consolidated.
Most of the clay is characterized as glacial clay which is seen by the varves which generally increases in thickness with depth. Typically, the more homogeneous grey post-glacial clay is limited to around 0, 5 m in thickness on land but can be several meters near-shore [21,22].
In areas without organic soil (Figure 3), the dry crust layer is usually 1–2 m thick, sometimes up to 3–4 m, created by drying and freezing cycles. The ground water table (GWT) is typically located at the bottom of the dry crust with hydrostatical conditions with depth.
In areas with organic soil the GWT is typically at the ground surface. The typical bottom soil profile in these areas are similar to that of non-organic soils, but instead of a dry-crust there is a gradual transition from gyttja (the soil is classified as gyttja when the organic content is over 6%). The organic content originates from plant and animal remains high in fat and protein, and the resulting soil is greenish and highly elastic. The gyttja was normally sedimented in waters with little movement, e.g. in bays. Over time, the uplift withdrew the water table and the soil surface was overgrown by vegetation and peat was formed, e.g. organic soil rich in carbohydrates from desiccated plant remains. Often, a thin layer of sand is seen between the gyttja and peat as a result of a small historical shoreline (beach). The typical depths of the organic soils are limited up to 3–5 m. Near-shore however, the thickness of the gyttja or organic clay can be 10–15 m.
Another feature of Stockholm's quaternary geology is the geographical differences. Typically, a higher silt content is seen towards the south, often classified as clayey silt or silt. Higher sensitivity clays are typically seen towards the northwest, where quick clay is also found, whilst towards the northeast the sulphide content increases. In Sweden, quick clay is defined as sensitivity > 50 and remoulded shear strength < 0.4 kPa.
Most of the data presented in this paper originates from tests on samples taken with the standard Swedish piston sampler [23]. This sampler has been in use since the mid-1960s as a result of an extensive research project performed by the Swedish Geotechnical Institute in the 1950s and 1960s, e.g. [6,7,8,24]. The piston sampler including some geometrical properties is shown schematically in Figure 5. The inner diameter is 50 mm (or 60 mm for a re-designed sampler in the 2020s) with an inside clearance of 0.2 mm allowing the sample to swell somewhat when entering the sampler. The angle of the cutting shoe is around 5°. There are three inner cylinders with 170 mm height which are separated in field and handled and stored separately, which enables an easy handling of the samples, although this involves trimming in the field which can be cumbersome at times. The sampler and the sampling technique are normally assumed to give samples with sufficient quality; however, this depends on the type of clay [8,25].
A few tests presented herein originates from known high-quality sampling using the mini-block sampler developed at the Norwegian University of Technology and Science (NTNU) [26], see photographs in Figure 6. The sampler uses cutting knives and water flushing at the bottom and carves out a 160 mm diameter cylindrical sample up to 350 mm height. The sampler has been tested extensively in Norway and Sweden and is shown to give high-quality samples [26,27,28,29].
All laboratory tests were performed according to their respective ISO EN standard where applicable. Only a few exceptions were made in which the most important is execution and interpretation of the Swedish fall cone test for determination of the index undrained shear strengths. Here, the older Swedish practice was followed where the execution is performed with a higher accuracy than specified in ISO EN 17892–6 [30], and a cone factor is used which is calibrated so that the strength corresponds to the in-situ vane test [31,32]. It is further corrected for effect of plasticity [31].
It is also noted that the plasticity of the clay is described by the liquid limit alone following Swedish practice, and hence the plastic limit nor the plasticity index are available. This is because it has been shown that this type of clay form a narrow band just above the A-line in the Casagrande plot [30,33,34], and there is thus a very good correlation between the liquid limit and the plasticity index. The material behavior is thus described well by the liquid limit alone. This is also seen for Norwegian clays [35]. As the testing procedure for the plastic limit in addition is somewhat cumbersome and time-consuming, especially for clays with low remoulded shear strengths which needs considerable drying, it is seldom determined in Swedish engineering practice.
The CRS oedometer test is performed according to the Swedish standard, also described by Sällfors [36,37]. Samples of 50 × 20 mm are compressed with a constant rate of strain of 0.75% per hour for at least 24 hours where 18% strain is obtained. The top of the sample is drained, and the bottom is undrained to measure the pore pressure response. Teflon oedometer rings coated with high resistance silicon fat are used to lessen the friction between the sample and the ring. All oedometer tests are performed in a climate room where the temperature is held at around 7 degrees to simulate field conditions. This has shown to be of great importance and will affect properties such as preconsolidation pressure and moduli [38,39].
The interpretation of the CRS oedometer test is illustrated in Figure 7. The method is based on Sällfors' method [36,37,40] and has been used exclusively in Sweden since the 1980s. The preconsolidation pressure (σ'c) is interpreted by fitting two straight lines of the oedometer curve before and after the apparent σ'c, i.e. Mi and ML, respectively. Between these an isosceles triangle is drawn with its based tangential to the oedometer curve as shown in the figure, and the σ'c is defined as the intersection of the baseline and the Mi-line. Sällfors [36] showed that this approach gave similar values as incremental oedometer tests and field measurements, and the method thus yields a relatively "conservative" value of σ'c to take the high rate of strain in CRS tests into consideration. It is believed that this method will give somewhat lower values than other methods as summarized in for example Paniagua et al. [41]. It is noted that this interpretation method is seldom used internationally and a direct comparison between other tests results should therefore be done with this in mind.
The moduli Mi and ML are today also in use in Norway to interpret CRS oedometer tests [42]. It should however be noted that the modulus Mi is not used for settlement calculation in Swedish practice as it is highly affected by sample disturbance and loss of radial stress. It is normally assumed that the modulus representative for field conditions, referred to Mo, is around 3–5 times the measured Mi.
The limit pressure (σ'L) is defined as the onset of modulus increase, i.e. the end of the ML-line, however, it is adjusted by a strain rate factor "c", see Figure 7. The parameter M' is equivalent to "m" in the Janbu terminology [43].
The initial permeability is defined as the intersect between the fitted straight line and the x-axis, i.e. at 0% strain, see Figure 7. The change of permeability with strain is referred to as βk.
Worth noting is the correction for false deformation which is done when referring to strain values of the oedometer curve, e.g. for assessing sample quality by strain to in-situ effective stresses [44]. This false deformation typically originates from poor alignment between the sample and the filter or a thin water film at the undrained bottom of the sample, which lately also is noted on Norwegian clays [45]. Normally, this can be avoided by gently pressing the top filter stone onto the sample before mounting the piston and completing the test set up, however, this is not always successful. It is however believed by the authors that this false deformation does not affect the interpreted values.
The data presented herein are divided into two generic databases. The first is labelled CLAY/7/3578 according to [46] and includes results from routine analyses, i.e., depth, bulk density, water content, liquid limit, intact undrained shear strength from fall cone tests and remoulded shear strength from fall cone tests and sensitivity. Basic values are summarized in Table 1. The second database is labelled CLAY/16/1675, which, in addition to results from routine analyses, also includes results from the CRS oedometer tests, i.e., preconsolidation pressure, several moduli and permeability properties. Basic values are summarized in Table 2.
Min. | Average | Max. | Unit | |
Density | 1.15 | 1.69 | 2.13 | t/m3 |
Water content | 19 | 56 | 357 | % |
Liquid limit, wL | 18 | 52 | 309 | % |
Remoulded shear strength, cur,FC | 0.07 | 1.4 | 28 | kPa |
Index shear strength, cu,FC | 1.0 | 14.8 | 114 | kPa |
Sensitivity, St | 1.8 | 17 | 186 | - |
Min. | Average | Max. | Unit | |
Preconsolidation pressure, σ'c | 8 | 61 | 305 | kPa |
Modulus, Mi | 133 | 3,216 | 47,000 | kPa |
Modulus, ML | 65 | 652 | 5,000 | kPa |
Modulus number M' | 5.5 | 15.7 | 34 | - |
Limit pressure, σ'L | 19 | 64 | 550 | kPa |
Permeability, k | 8.5E-11 | 7.1E-10 | 2.3E-08 | m/s |
Change of k with strain, βk | 1.5 | 4.0 | 8.4 | - |
Coefficient of consolidation, cv,NC | 0.05 | 0.73 | 20.14 | m2/s |
Histograms of selected index and deformation properties are shown in Figure 8 and Figure 9, respectively. A total of 92% of all samples are taken between 1 and 10 m depth from the ground surface which also reflects the typical maximum clay depths in the Stockholm area, although samples are not always taken all the way to the bottom. Notably, there are a few areas with up to around 30 m of clay. Most of the samples have natural water contents (wN) between 40 and 80% with an average of 56%. The liquid limit is commonly in the same range as the natural water content, which is typical for Stockholm clays except when the sensitivity is high. The index undrained strength (cu,FC), determined by the Swedish fall cone test [31], shows most values from 5 to 20 kPa with an average value of about 15 kPa. This strength is equivalent to the direct undrained strength according to the ADP-concept of active-direct-passive shear strengths [44,47]. The interpreted preconsolidation pressures (σ'c) ranges between 8 and 305 kPa with an average of around 61 kPa and oedometer modulus in the normally consolidated range (ML) between 65 and 5,000 kPa with an average of 650 kPa.
It is noted that the range of e.g. index properties, strength and preconsolidation pressure are similar to that of for example Finnish clays [48], but are low in comparison to many other natural clays [49].
Index properties from the CLAY/7/3578 database are plotted against depth in Figure 10. Average and median values are plotted together with the 10th and 90th percentile to give indications of the variability. Most properties have a close to normal distribution at each depth interval. Only values between 2 m and 10 m are plotted to avoid non-representative data from desiccated samples at depths above 2 m and due to too few data points below 10 m.
It is seen that the bulk density increases somewhat with depth and that the water content along with the liquid limit decreases somewhat. This is expected from the previous geological description with decreasing grain fraction with depth. The index undrained shear strength determined by the fall cone test increases slightly, and it is seen that the variability decreases with depth which most probably is a result of the effect of sites with organic soils. The remoulded shear strength decreases with depth between 2 and around 4 m, probably due to desiccation effects at the top. As a result, the sensitivity increases with depth and ranges from around 10 at 2 m depth to around 20 at 10 m depth.
Similar plots for deformation properties from the CLAY/16/1675 database are shown in Figure 11. The preconsolidation pressure increases from around 40 kPa at 2 m depth to around 80 at 10 m depth. The rate of increase is around 5.4 kPa/m, slightly less than the average increase of in-situ effective stress assuming an average density of 1.69 t/m3 (Table 1) and hydrostatic pore water pressure, giving a 6.9 kPa/m increase. There is no apparent effect of desiccation at the top, most probably since oedometer tests normally are avoided if there are visual signs of desiccation.
The modulus in the normally consolidated range (ML) and the modulus number M' also exhibits a slight increase with depth. The variability of ML is however large. The lowest values are around and slightly below 100 kPa, illustrating the high compressibility of clays in some areas. From the authors' experience, the lowest values of ML are seen on samples of organic clays and inorganic clays with water contents around 80–100%.
In Figure 11 it is also seen that the permeability decreases somewhat with depth, despite the expected increasing grain fraction, i.e. decreasing clay fraction, with depth. It has however been shown that the permeability is not affected by clay fraction alone, but also highly depends on plasticity, clay fabric etc. [50]. The change of permeability with strain (βk) is however fairly constant with depth with an average around 4. The coefficient of consolidation at normally consolidated state (cv,NC) is also fairly constant with an average around 0.5–1.0 m2/year, although with considerable variability. The values of is relatively low compared to many other clays.
The moduli Mi, ML and M' and permeability properties are plotted against natural water contents (wN) in Figure 12. The figure distinguishes between samples taken with the standard piston sampler and the mini block sampler to illustrate effects of sample quality. Despite considerable scatter, it is seen that the moduli Mi and ML decreases with increasing water contents. For water contents above 100–120%, i.e. typically organic clays and gyttja for Swedish clays [21,32,33], ML never seem to exceed 250 kPa. Similarly, the Mi is lower than 500. The samples of known high quality, e.g. the mini-block samples, show higher Mi and lower ML. This is discussed later in the paper.
The modulus number M' decreases with increasing water content, and even though the scatter is large the data follows the empirical correlation given by Larsson and Sällfors [37] as well that seen on Norwegian clays [42]. All these moduli seem to have similar correlations with the liquid limit.
The data presented in these figures naturally exhibit a large scatter since it originates from the tests on the highly heterogeneous Stockholm clay from a large number of sites. Based on the authors experience it is however often possible to find relatively similar results from limited geographical areas. An example is shown in Figure 13. Despite the large variation in natural water content there is a clear correlation between both ML and water content and Mi and the preconsolidation pressure.
For the permeability properties such as initial permeability and change of permeability with strain there seem to be a slight change with water content; however, there is considerable scatter and no conclusion can be drawn based on these results. This was also noted by Tavenas et al. [50] who describes that the permeability is also highly affected by clay fabric and plasticity. The coefficient of consolidation decreases with increasing water content, which is because the modulus ML decreases with increasing water content. For change of permeability with strain (βk) there is no established Swedish correlation, but the values are similar to those for Norwegian clays [42].
Figure 14 plots the moduli Mi and ML with the preconsolidation pressure and the difference between the limit stress and preconsolidation pressure, i.e. the length of the ML-line, respectively. There is a clear correlation between Mi and the preconsolidation pressure where Mi ranges between around 15 and 70 times the preconsolidation pressure, on average Mi≈30×σ'c. If one assumes that the Mi underestimates the in-situ modulus M0 by a factor 3 to 5, as explained in chapter 3, the correlation 90×σ'c≲M0≲150×σ'c can be used for settlement calculations. This is much higher than empirical correlations given by Larsson et al. [51]. Correlating with the index undrained shear strength one obtains on average Mi≈145×cu, i.e. 400×cu≲M0≲700×cu for settlement calculations.
There is also an increase of ML with increasing preconsolidation pressure. The scatter is however much larger, and no conclusive correlations can be given. This is unfortunate since ML, i.e., settlement modulus in the normally consolidated range, governs much of the total settlements for the typical normally consolidated Stockholm clay. It is however generally seen that it decreases with increasing sample quality.
Figure 14d shows a correlation between the length of the ML-line and the absolute value of ML -the longer the ML-line, the higher ML. The authors are not aware of any previous research on this, and with the current available data is has not been possible to analyse this behavior further. The reason for this is therefore unknown, but it might indicate that the linear part of the oedometer curve after the preconsolidation pressure is limited by a strain interval instead of a stress interval.
Tavenas et al. [50] and Leroueil et al. [52] compiled results from oedometer tests on Canadian and some Swedish clays with regards to e.g. the permeability change index Ck, defined as the slope of a curve in a void ratio vs. permeability plot (Ck=(1+e0/βk). It was concluded that Ck can be simplified to 0.5 times the initial void ratio, e0 with a possible lower bound of around 0.4 times e0, also noted by [53].
Figure 15 shows a plot of the Ck vs. initial void ratios for all tests. Here, the initial void ratio is calculated by the natural water content assuming full saturation and a grain density of 2.65 [54]. It is seen that the correlation Ck≈0.4×e0 is a better fit for Stockholm clays.
The anisotropy has investigated on samples from a few sites where samples were extracted using the redesigned standard piston sampler with 60 mm inner diameter. This allows for trimming of 20 mm high specimens for CRS oedometer tests in both vertical and horizontal direction. Preconsolidation pressure evaluated from tests on the same sample depth indicates the degree of anisotropy, i.e. values of K0, assuming that the OCR is equal in both vertical and horizontal direction. Results from tests on samples south of Stockholm are shown in Figure 16 along with an empirical correlation by Larsson et al. [55] given as K0,NC=0.31+0.71×(wL−0.2) which is valid for normally consolidated clays. Despite few data points and a considerable scatter, the data indicates a slight dependency of the liquid limit on K0 and a linear regression is similar to the empirical correlation by [55].
This dependence of plasticity on K0 have also been shown by other researchers on Swedish clays [56], whilst tests on Norwegian clays have shown negligible effects of plasticity [57]. The Norwegian clays, however, had liquid limits ranging between 30 and 74 [57], a far smaller range than the Swedish clays ranging from around 40 to 150 [55,56], and between around 40 and 90 in this study (Figure 16). The difference is thus probably due to the relatively narrow range of liquid limits for Norwegian clays where any plasticity effect is hidden by the typical scatter. For both clays, K0 is highly dependent on OCR, however, this effect is not investigated herein.
Sample quality have been shown to be of vital importance for establishing relevant engineering properties [27,58,59,60,61]. Sample disturbance, i.e. altered properties of the soil sample from its in-situ state to its state at the time of laboratory testing, can be categorized as follows: changes in effective stresses, changes in water content, structural changes and chemical changes [62].
A few studies on sample quality and comparative testing between the standard piston sampler and block samplers on Swedish clays have been made [25,27,29,63,64]. These have shown varying results, ranging from large differences in quality to in practice equal quality between different samplers. In general, it is seen that low plastic clays are more prone to disturbance than high-plastic clays. Results from [27] is presented in Figures 17 and 18, see location in Figure 2. Examples of comparative CRS oedometer tests results are shown in Figure 17. These clearly indicates large differences in both the oedometer curves and moduli curves, affecting several properties such as preconsolidation pressure and moduli. Figure 19 show resulting preconsolidation pressures and ML against depth in one location. There is clearly a large difference in both these properties. It can be generalized that with increasing sample quality:
• The initial modulus Mi increases;
• The modulus ML decreases;
• The difference between the limit stress (σ'L) and the preconsolidation pressure (σ'c), i.e. the length of the linear part of the oedometer curve (the ML-line), decreases;
• The modulus M' is not affected;
• Often higher preconsolidation pressure, especially for large sampling depths;
• Less scatter of several properties, e.g. preconsolidation pressure.
Most of these findings are also supported by comparative sampling with the standard piston sampler and the mini block sampler performed by SGI at the Skå-Edeby test field [65].
There are several methods proposed to quantify sample quality, e.g., change in void ratio in relation to initial void ratio during reconsolidation to in situ effective stress [61], volumetric strain to in situ effective stress [66], moduli quotient between Mi and ML [42] and shear wave velocity and suction [67]. Recommendations from SGI, most often are used in Sweden to assess sample quality, involve a redevelopment of the change in void ratio in relation to initial void ratio proposed by Lunne et al. [61]. Here, the change in void ratio have been re-calculated to total strain taking the "false" deformation into account (Figure 7). By plotting this against the natural water content instead of initial void ratio one can use the Lunne et al. [61] criteria for assessing sample quality, as described by Larsson et al. [51]. In general, the lower strain, the higher quality since the clay structure is less altered.
There is no available data on in situ effective stresses in the database CLAY/16/1675, however, this procedure has been simplified by the strain to 85% of the preconsolidation pressure (ϵ0.85σ'c), i.e. strain to in situ effective stress if OCR is 1/0.85 ≈ 1.15–1.2, which are typical for Stockholm clays. These values have been plotted against natural water content and the difference between the limit stress and the preconsolidation pressure in Figure 19a and b. There seems to be no correlation, which supports the authors' experience that the strain to in situ effective stresses are not applicable to CRS oedometer tests. For example, a stiff response (high Mi) with a low strain value can be obtained on a visually clearly disturbed clay sample with desiccation effects, and vice versa for extremely soft samples which inherently have considerably lower values of Mi and hence large strains to in situ stresses.
The methodology proposed by Karlsrud and Hernandez-Martinez [42], i.e., criteria for the moduli quotient Mi/ML, herein referred to the sample quality index (SQI), have been assessed for the Stockholm clays. Figure 19 (c) and (d) plots this SQI against the natural water content and the difference between the limit stress and the preconsolidation pressure, respectively. It is seen that the SQI in general increases with increasing water content, and a decrease with increasing difference between limit stress and the preconsolidation pressure. Karlsrud and Hernandez-Martinez [42] proposed the following criteria for sample quality: < 1 very poor; 1–1.5 poor; 1.5–2 good to fair and; > 2 very good to excellent sample quality. From Figure 19 it is obvious that these criteria are not suitable for Stockholm clays since most samples would be classified as "very good to excellent", which is not the case. Notably, from the authors' experience, these criteria are not suitable for any type of Swedish clays along the East or West coast.
Unfortunately, there is no "perfect" value of the SQI (Mi/ML), compared to an ideal 0% strain to in situ effective stress for a "perfect" sample with no alteration of the clay structure. The higher Mi/ML, the higher quality; however, the highest possible value will differ between the exact type of clay. Any type of criteria on sample quality based on this methodology will thus have to be based on a relative distribution for different types of clays. Figure 20 shows histograms of Mi/ML, and strain to 85% of the preconsolidation pressure. Based on this and Figure 19 sample quality criteria in Table 3 is proposed for Stockholm clays. The table also notes its respective frequency is the database CLAY/16/1675. Around 20% of all samples are here classified having a poor quality, whilst around 45% are classified having good to very good quality. Most of the mini block samples are thus good to very good quality. It is believed that this simple methodology with these criteria is suitable in engineering practice for this type of clay.
Mi/ML | Frequency | |
Poor quality | < 2 | ~20% |
Fair quality | 2 – 4 | ~35% |
Good quality | 4 – 8 | ~32% |
Very good quality | > 8 | ~13% |
This paper presents data from two generic databases to characterise the index and deformation properties of Stockholm clays. It is found that the clays are highly heterogeneous with highly variable natural water contents, plasticity and other index properties, as well as yield stresses and moduli. Due to its geological history, the Stockholm clays are normally consolidated with low index shear strengths and high compressibility.
It is found that the natural water content, or the liquid limit, affects the moduli, such as Mi and ML, and the modulus number M' with decreasing moduli with increasing water content. The initial permeability is however not particularly affected by the water content, except the coefficient of consolidation which decreases with increasing water content (as an effect of ML). The permeability change index is found to be around 0.4 times the initial void ratio.
The modulus Mi is further highly correlated to the preconsolidation pressure, however these values are not representative for field conditions. The modulus ML is also increasing with increasing preconsolidation pressure, but there is a considerable scatter. The modulus ML also increases with increasing difference between the limit stress and preconsolidation pressure.
Effects of samples quality is analyzed. It is found that the quality affects several deformation properties, especially preconsolidation pressure and the moduli Mi and ML, however, M' is not affected. A methodology of assessing sample quality using the sample quality index Mi/ML is found to be suitable for CRS oedometer tests; however, it is noted that previously recommended criteria is not applicable for Stockholm clays, and new criteria are thus proposed.
The authors are thankful to the meticulous work by the laboratory technicians at LabMind geotechnical laboratory in Stockholm, Sweden.
The authors are also grateful to Jean-Sébastien L'Heureux at the Norwegian Geotechnical Institute and the anonymous reviewers for reviewing the manuscript and giving valuable comments.
The authors declare no conflict of interest.
[1] | Atterberg A (1911) Clays and its water contents and plasticity limits[In Swedish]. Kungliga Lantbruksakademiens Handlingar och Tidskrift 50: 132–158. |
[2] | Atterberg A (1912) Consistency of soils[In Swedish]. Kungliga Lantbruksakademiens Handlingar och Tidskrift 51: 93–123. |
[3] | Casagrande A (1932) Research on the Atterberg limits of soils. Public Roads 13: 121–136. |
[4] | Statens Järnvägar (1922) Swedish State Railways Geotechnical Commission 1914–1922.[In Swedish]. |
[5] | Hansbo S (1957) A new approach to the determination of the shear strength of clay by the fall-cone test, Swedish Geotechnical Institute, Stockholm. |
[6] | Jakobson B (1954) Influence of sampler type and testing method on shear strength of clay samples, Statens Geotekniska Institut, Stockholm. |
[7] | Kallstenius T (1958) Mechanical disturbances in clay samples taken with standard piston sampler, Swedish Geotechnical Institute, Stockholm. |
[8] | Kallstenius T (1963) Studies on clay samples taken with standard piston sampler, Swedish Geotechnical Institute, Stockholm. |
[9] | Cadling L, Odenstad D (1950) The vane borer. An apparatus for determining the shear strength of clay soils directly in the ground, Swedish Geotechnical Institute, Stockholm. |
[10] |
Bjerrum L, Flodin N (1960) The development of soil mechanics in Sweden, 1900–1925. Géotechnique 10: 1–18. https://doi.org/10.1680/geot.1960.10.1.1 doi: 10.1680/geot.1960.10.1.1
![]() |
[11] | Lundin S (2000) Geotechnics in Sweden 1920–1945, Swedish Geotechnical Society Report 1. |
[12] | Larsson R (1986) Consolidation of soft soils, Swedish Geotechnical Institute. |
[13] | Massarsch R, Aronsson S (2014) The Heritage of Swedish Foundation Engineering. Proceedings, DFI/EFFC International Conference on Piling and Deep Foundations, Stockholm, 1031–1054. |
[14] |
Phoon KK, Ching J, Cao Z (2022) Unpacking data-centric geotechnics. Underground Space 7: 967–989. https://doi.org/10.1016/j.undsp.2022.04.001 doi: 10.1016/j.undsp.2022.04.001
![]() |
[15] |
Phoon KK, Kulhawy FH (1999) Evaluation of geotechnical property variability. Can Geotech J 36: 625–639. https://doi.org/10.1139/t99-039 doi: 10.1139/t99-039
![]() |
[16] |
Phoon KK, Ching J, Shuku T (2022) Challenges in data-driven site characterization. Georisk Assess Manage Risk Eng Syst Geohazards 16: 114–126. https://doi.org/10.1080/17499518.2021.1896005 doi: 10.1080/17499518.2021.1896005
![]() |
[17] | TC304, TC304 databases. Available from: http://140.112.12.21/issmge/tc304.htm? = 6. |
[18] | Swedish Geotechnical Society, Classification and description of soils. Report 1: 2016. |
[19] | Swedish Geological Survey, Interactive online maps. 2022. Available from: https://www.sgu.se/om-sgu/nyheter/2022/juni/sgus-strsndforskjutningsmodell-ger-en-bild-av-den-forntida-fordelningen-mellan-hav-och-land/. |
[20] |
Bjerrum L (1967) Engineering geology of Norwegian normally-consolidated marine clays as related to settlements of buildings. Géotechnique 17: 83–118. https://doi.org/10.1680/geot.1967.17.2.83 doi: 10.1680/geot.1967.17.2.83
![]() |
[21] | Hov S, Borgström K, Paniagua P (2022) Full-flow CPT tests in a nearshore organic clay. Cone Penetration Test, 452–458. |
[22] | Swedish Geological Survey, Description of the geological map Stockholm. 1964. |
[23] | Swedish Geotechnical Society, Geotechnical Field Hand Book[In Swedish]. Report 1: 2013. |
[24] | Swedish Geotechnical Institute, Swedish Committe on piston samling, Standard piston sampler, Stockholm. 1961. |
[25] | Larsson R (1981) Do we obtain undisturbed samples from the standard piston samplers? A comparison with the Laval sampler[In Swedish]. Swedish Geotechnical Insititute. |
[26] |
Emdal A, Gylland A, Amundsen H, et al. (2016) Mini-block sampler. Can Geotech J 53: 1235–1245. https://doi.org/10.1139/cgj-2015-0628 doi: 10.1139/cgj-2015-0628
![]() |
[27] | Hov S, Garcia de Herreros C (2020) Comparison of the mini block sampler and the standard piston sampler in a varved East Swedish clay. Report LabMind. |
[28] | Karlsson M, Bergström A, Djikstra J (2015) Comparison of the performance of mini-block and piston sampling in high plasticity clays, Technical Report. Chalmers University of Technology, Gothenburg, Sweden. |
[29] |
Karlsson M, Emdal A, Dijkstra J (2016) Consequences of sample disturbance for predicting long-term settlements in soft clay. Can Geotech J 53. https://doi.org/10.1139/cgj-2016-0129 doi: 10.1139/cgj-2016-0129
![]() |
[30] | Hov S, Holmén M (2018) The liquid limit[In Swedish]. Swedish Geotechnical Society. |
[31] | Hov S, Holmén M (2018) The fall cone test[In Swedish]. Swedish Geotechnical Society. |
[32] |
Hov S, Prästings A, Persson E, et al. (2021) On Empirical Correlations for Normalised Shear Strengths from Fall Cone and Direct Simple Shear Tests in Soft Swedish Clays. Geotech Geol Eng 39: 4843–4854. https://doi.org/10.1007/s10706-021-01797-w doi: 10.1007/s10706-021-01797-w
![]() |
[33] | Karlsson R (1981) Consistency Limits in cooperation with the Laboratory Committee of the Swedish Geotechnical Society. Swed Council Build Res, Stockholm, Sweden, Document D6. |
[34] | Karlsson R (1961) Suggested improvements in the liquid limit test, with reference to flow properties of remoulded clays. Proc 5th Int Conf Soil Mech Found Eng 1: 171–184. |
[35] | Christensen S (2014) Interpretation of field tests, choice of design cuA based on field and laboratory tests[In Norwegian]. Norway's water resources and energy directorate in cooperation with the Norwegian road and railway administrations report no. |
[36] | Sällfors G (1975) Preconsolidation pressure of soft, high-plastic clays. Chalmers University of Technology. |
[37] | Larsson R, Sällfors G (1986) Automatic Continuous Consolidation Testing in Sweden, Consolidation of Soils: Testing and Evaluation, ASTM STP, 299–328. |
[38] |
Tidfors M, Sällfors G (1989) Temperature effects on preconsolidation pressure. Geotech Test J 12: 93–97. doi: 10.1520/GTJ10679J
![]() |
[39] | Boudali M, Leroueil S, Murthy S (1994) Viscous behaviour of natural clays. XⅢ ICSMFE, 411–416. |
[40] | Larsson R, Sällfors G (1981) Calculation of settlements in clay[In Swedish]. Väg-och Vattenbyggaren 3: 39–42. |
[41] | Paniagua P, L'Heureux JS, Yang SY, et al. (2016) Study on the practices for preconsolidation stress evaluation from oedometer tests. Proc 17th Nord Geotech Meet, 547–555. |
[42] |
Karlsrud K, Hernandez-Martinez F (2013) Strength and deformation properties of Norwegian clays from laboratory tests on high-quality block samples. Can Geotech J 50: 1273–1293. https://doi.org/10.1139/cgj-2013-0298 doi: 10.1139/cgj-2013-0298
![]() |
[43] | Janbu N (1963) Soil compressibility as determined by oedometer and triaxial tests. Proc Eur Conf SMFE 1: 19–25. |
[44] | Larsson R, Sällfors G, Bengtsson PE, et al. (2007) Shear strength in cohesive soils[In Swedish]. Swedish Geotechnical Institute. |
[45] |
Berre T, Lunne T, L'Heureux JS (2022) Quantification of sample disturbance for soft, lightly overconsolidated, sensitive clay samples. Can Geotech J 59: 300–303. https://doi.org/10.1139/cgj-2020-0551 doi: 10.1139/cgj-2020-0551
![]() |
[46] | Ching J, Li DQ, Phoon KK (2016) Statistical characterization of multivariate geotechnical data. Reliability of geotechnical structures in ISO2394, Balkema, CRC Press. |
[47] | Bjerrum L (1972) Embankments on soft ground. State of the art report, ASCE Specialty conference on performance of earth and earth-supported structure. |
[48] |
D'Ignazio M, Phoon KK, Tan SA, et al. (2016) Correlations for undrained shear strength of Finnish soft clays. Can Geotech J 53: 1628–1645. https://doi.org/10.1139/cgj-2016-003 doi: 10.1139/cgj-2016-003
![]() |
[49] |
Ching J, Phoon KK (2012) Modeling parameters of structured clays as a multivariate normal distribution. Can Geotech J 49: 522–545. https://doi.org/10.1139/t2012-015 doi: 10.1139/t2012-015
![]() |
[50] |
Tavenas F, Jean P, Leblond P, et al. (1983) The permeability of natural soils. Part Ⅱ: permeability characteristics. Can Geot J 20: 645–660. https://doi.org/10.1139/t83-073 doi: 10.1139/t83-073
![]() |
[51] | Larsson R, Bengtsson PE, Eriksson L (1997) Prediction of settlements of embankments on soft, fine-grained soils. Calculation of settlements and their course with time, Swedish Geotechnical Institute. |
[52] |
Leroueil S, Bouclin G, Tavenas F, et al. (1990) Permeability anisotropy of natural clays as a function of strain. Can Geotech J 27: 568–579. https://doi.org/10.1139/t90-072 doi: 10.1139/t90-072
![]() |
[53] | Mesri G, Feng T, Ali S, et al. (1994) Permeability characteristics of soft clays. XⅡ ICSMFE, 187–192. |
[54] | Larsson R (2008) Properties of soils[In Swedish]. Swedish Geotechnical Institute. |
[55] | Larsson R (1977) Basic behaviour of Scandinavian soft clays, Swedish Geotechnical Institute. |
[56] | Massarsch R (1979) Lateral earth pressure in normally consolidated clay. Design Parameters in Geotechnical Engineering. Proc 7th Eur Conf Soil Mech Found Eng 2: 245–249. |
[57] | L'Heureux JS, Ozkul Z, Lacasse S, et al. (2017) A revised look at the coefficient of earth pressure at rest for Norwegian Clays. NGF Geoteknikkdagen 35. |
[58] | DeGroot DJ, Poirier SE, Landon MM (2005) Sample disturbance—soft clays. Studia Geotechnica et Mechanica, Vol. XXVⅡ, 107–120. |
[59] |
Hight DW, Boese R, Butcher AP, et al. (1992) Disturbance of the Bothkennar clay prior to laboratory testing. Géotechnique 42: 199–217. https://doi.org/10.1680/geot.1992.42.2.199 doi: 10.1680/geot.1992.42.2.199
![]() |
[60] | Lacasse S, Berre T, Lefevbre G (1985) Block sampling of sensitive clays. Proceedings of the 11th conference on soil mechanics and foundation engineering, San Francisco, CA, 887–892. |
[61] | Lunne T, Berre T, Strandvik S (1997) Sample disturbance effects in soft low plastic Norwegian clay. Proceedings of the conference on recent developments in soil and pavement mechanics, Rio de Janeiro, 81–102. |
[62] |
Clayton CRI, Siddique A (1999) Tube sampling disturbances—fotgotten truths and new perspectives. ICE Geotech Eng 137: 127–135. https://doi.org/10.1680/gt.1999.370302 doi: 10.1680/gt.1999.370302
![]() |
[63] | Larsson R (2011) SGIs 200 mm diameter block sampler—Undisturbed sampling in fine-grained soils[In Swedish]. Swedish Geotechnical Institute Report 33. |
[64] | Löfroth H (2012) Sampling in normal and high sensitive clay—a comparison of results from specimens taken with the SGI large-diameter sampler and the standard piston sampler St Ⅱ. Swedish Geotechnical Institute. |
[65] | Andersson M (2022) Personal communication. Swedish Geotechnical Institute. |
[66] | Terzaghi K, Peck R, Mesri G (1997) Soil mechanics in engineering practice, Jon Wiley & Sons. |
[67] |
Donohue S, Long M (2010) Assessment of sample quality in soft clay using shear wave velocity and suction measurements. Géotechnique 60: 883–889. https://doi.org/10.1680/geot.8.T.007.3741 doi: 10.1680/geot.8.T.007.3741
![]() |
Min. | Average | Max. | Unit | |
Density | 1.15 | 1.69 | 2.13 | t/m3 |
Water content | 19 | 56 | 357 | % |
Liquid limit, wL | 18 | 52 | 309 | % |
Remoulded shear strength, cur,FC | 0.07 | 1.4 | 28 | kPa |
Index shear strength, cu,FC | 1.0 | 14.8 | 114 | kPa |
Sensitivity, St | 1.8 | 17 | 186 | - |
Min. | Average | Max. | Unit | |
Preconsolidation pressure, σ'c | 8 | 61 | 305 | kPa |
Modulus, Mi | 133 | 3,216 | 47,000 | kPa |
Modulus, ML | 65 | 652 | 5,000 | kPa |
Modulus number M' | 5.5 | 15.7 | 34 | - |
Limit pressure, σ'L | 19 | 64 | 550 | kPa |
Permeability, k | 8.5E-11 | 7.1E-10 | 2.3E-08 | m/s |
Change of k with strain, βk | 1.5 | 4.0 | 8.4 | - |
Coefficient of consolidation, cv,NC | 0.05 | 0.73 | 20.14 | m2/s |
Mi/ML | Frequency | |
Poor quality | < 2 | ~20% |
Fair quality | 2 – 4 | ~35% |
Good quality | 4 – 8 | ~32% |
Very good quality | > 8 | ~13% |
Min. | Average | Max. | Unit | |
Density | 1.15 | 1.69 | 2.13 | t/m3 |
Water content | 19 | 56 | 357 | % |
Liquid limit, wL | 18 | 52 | 309 | % |
Remoulded shear strength, cur,FC | 0.07 | 1.4 | 28 | kPa |
Index shear strength, cu,FC | 1.0 | 14.8 | 114 | kPa |
Sensitivity, St | 1.8 | 17 | 186 | - |
Min. | Average | Max. | Unit | |
Preconsolidation pressure, σ'c | 8 | 61 | 305 | kPa |
Modulus, Mi | 133 | 3,216 | 47,000 | kPa |
Modulus, ML | 65 | 652 | 5,000 | kPa |
Modulus number M' | 5.5 | 15.7 | 34 | - |
Limit pressure, σ'L | 19 | 64 | 550 | kPa |
Permeability, k | 8.5E-11 | 7.1E-10 | 2.3E-08 | m/s |
Change of k with strain, βk | 1.5 | 4.0 | 8.4 | - |
Coefficient of consolidation, cv,NC | 0.05 | 0.73 | 20.14 | m2/s |
Mi/ML | Frequency | |
Poor quality | < 2 | ~20% |
Fair quality | 2 – 4 | ~35% |
Good quality | 4 – 8 | ~32% |
Very good quality | > 8 | ~13% |