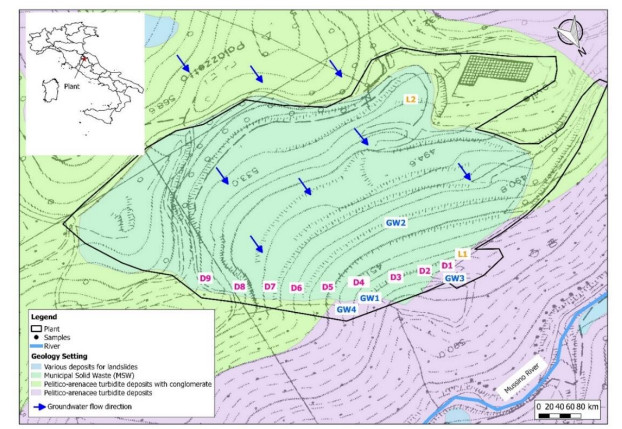
Municipal solid waste landfills leachate can cause serious environmental issues for groundwater quality. Therefore, the application of environmental tracing methods to identify groundwater contamination by municipal solid waste landfills leachate is significant. Hydrogeochemical evaluations to trace municipal solid waste landfills leachate are usually carried out. The study was carried out at a landfill in central Italy (Umbria). Samples of leachate and groundwater have been analyzed to evaluate the impact of leachates on groundwater through the comparison of their hydrogeochemical nature. Parameters like pH, Temperature (T), Electrical Conductivity (EC), redox potential (Eh) and Chemical Oxygen Demand (COD) were also measured in situ using digital instruments. Hydrogeochemical data (Na+, K+, Mg2+, Ca2+, SO42−, HCO3−, Cl−, NO3−), ionic ratios and geochemical correlations were used to confirm the processes that govern the chemistry of the spring water and to identify leachate contamination phenomena. In fact, the main geochemical diagrams (Langelier-Ludwig, Piper, Schoeller) confirm the leachate contamination in a groundwater sample. In particular, the Piper diagram shows that a sample is in Na+ – Cl- – HCO3- mixing zone, indicating a possible influence of the leachate on groundwater chemistry. As a matter of fact, some correlations between major elements, such as Cl- versus Na+ and Cl- versus HCO3-, confirm that the leachate in this study area is highly enriched in Cl- and HCO3- due to wastes dissolution and degradation processes. Further, the assessment of K+/Mg2+ ratio also confirms the presence of a sample heavily impacted from leachate contamination. These results indicate that also one basic hydrogeochemical study can be useful for fingerprinting the leachate pollution for groundwater samples.
Citation: Maurizio Barbieri, Tiziano Boschetti, Giuseppe Sappa, Francesca Andrei. Hydrogeochemistry and groundwater quality assessment in a municipal solid waste landfill (central Italy)[J]. AIMS Geosciences, 2022, 8(3): 467-487. doi: 10.3934/geosci.2022026
[1] | Watcharin Phoemphon, Bantita Terakulsatit . Assessment of groundwater potential zones and mapping using GIS/RS techniques and analytic hierarchy process: A case study on saline soil area, Nakhon Ratchasima, Thailand. AIMS Geosciences, 2023, 9(1): 49-67. doi: 10.3934/geosci.2023004 |
[2] | Nicholas P. Sisto, Laura Maribel Colima Valadez, Ismael Aguilar Barajas, Juan Jacob Ayala Gaytán . Infectious Intestinal Diseases and Residential Water Services in Mexico: a Spatial Analysis. AIMS Geosciences, 2017, 3(3): 450-466. doi: 10.3934/geosci.2017.3.450 |
[3] | Nudthawud Homtong, Wisaroot Pringproh, Kankanon Sakmongkoljit, Sattha Srikarom, Rungtiwa Yapun, Ben Wongsaijai . Remote sensing-based groundwater potential evaluation in a fractured-bedrock mountainous area. AIMS Geosciences, 2024, 10(2): 242-262. doi: 10.3934/geosci.2024014 |
[4] | Diaka Sidibé, Mamadou Diallo, Ahmed Amara Konaté, Muhammad Zaheer . Geotechnical investigations of lead-silver ore processing residues at the Auzelles site, Auvergne (France). AIMS Geosciences, 2024, 10(4): 735-758. doi: 10.3934/geosci.2024036 |
[5] | Stefano Albanese, Annalise Guarino . Assessing contamination sources and environmental hazards for potentially toxic elements and organic compounds in the soils of a heavily anthropized area: the case study of the Acerra plain (Southern Italy). AIMS Geosciences, 2022, 8(4): 552-578. doi: 10.3934/geosci.2022030 |
[6] | António Viana da Fonseca, Cristiana Ferreira, Catarina Ramos, Fausto Molina-Gómez . The geotechnical test site in the greater Lisbon area for liquefaction characterisation and sample quality control of cohesionless soils. AIMS Geosciences, 2019, 5(2): 325-343. doi: 10.3934/geosci.2019.2.325 |
[7] | Palavai Venkateswara Rao, Mangalampalli Subrahmanyam, Bakuru Anandagajapathi Raju . Groundwater exploration in hard rock terrains of East Godavari district, Andhra Pradesh, India using AHP and WIO analyses together with geoelectrical surveys. AIMS Geosciences, 2021, 7(2): 244-267. doi: 10.3934/geosci.2021015 |
[8] | Stefano De Falco, Giulia Fiorentino . Geographical scattering in Italian inner areas, politics and COVID-19. AIMS Geosciences, 2022, 8(1): 137-158. doi: 10.3934/geosci.2022009 |
[9] | José L. Álvarez Cruz, Karla E. Campos Díaz, Erick R. Bandala, Felipe López Sánchez . Landfill Leachate Treatment Using Coupled, Sequential Coagulation-flocculation and Advanced Oxidation Processes. AIMS Geosciences, 2017, 3(4): 526-537. doi: 10.3934/geosci.2017.4.526 |
[10] | Gianni Petino, Donatella Privitera . Uncovering the local foodscapes. Exploring the Etna volcano case study, Italy. AIMS Geosciences, 2023, 9(2): 392-408. doi: 10.3934/geosci.2023021 |
Municipal solid waste landfills leachate can cause serious environmental issues for groundwater quality. Therefore, the application of environmental tracing methods to identify groundwater contamination by municipal solid waste landfills leachate is significant. Hydrogeochemical evaluations to trace municipal solid waste landfills leachate are usually carried out. The study was carried out at a landfill in central Italy (Umbria). Samples of leachate and groundwater have been analyzed to evaluate the impact of leachates on groundwater through the comparison of their hydrogeochemical nature. Parameters like pH, Temperature (T), Electrical Conductivity (EC), redox potential (Eh) and Chemical Oxygen Demand (COD) were also measured in situ using digital instruments. Hydrogeochemical data (Na+, K+, Mg2+, Ca2+, SO42−, HCO3−, Cl−, NO3−), ionic ratios and geochemical correlations were used to confirm the processes that govern the chemistry of the spring water and to identify leachate contamination phenomena. In fact, the main geochemical diagrams (Langelier-Ludwig, Piper, Schoeller) confirm the leachate contamination in a groundwater sample. In particular, the Piper diagram shows that a sample is in Na+ – Cl- – HCO3- mixing zone, indicating a possible influence of the leachate on groundwater chemistry. As a matter of fact, some correlations between major elements, such as Cl- versus Na+ and Cl- versus HCO3-, confirm that the leachate in this study area is highly enriched in Cl- and HCO3- due to wastes dissolution and degradation processes. Further, the assessment of K+/Mg2+ ratio also confirms the presence of a sample heavily impacted from leachate contamination. These results indicate that also one basic hydrogeochemical study can be useful for fingerprinting the leachate pollution for groundwater samples.
Landfill leachate is a serious source of groundwater pollution [1,2]. It can form by interaction between rainfall percolating and municipal solid wastes, thorough a range of biodegradation and leaching reactions [2,3]. The leachate contains nutrients, chlorinated organics, dissolved organic matter, inorganic compounds (e.g., ammonium, calcium, magnesium, sodium, potassium, iron, sulfates, chlorides) and heavy metals (e.g., cadmium, chromium, copper, lead, zinc, nickel) [4,5,6,7,8]. In fact, leachate coming from biological and physico-chemical decomposition of waste can cause damage to the environment when it percolates to the surrounding groundwater and stream water systems [4,8,9]. Therefore, it is fundamental the assessment of environmental risks due to landfill leachate going to water resources, specially to groundwater. So, it is necessary to perform environmental monitoring programs, in order to detect the potential leachate influence on groundwater near to the landfill area. Pollution levels from landfill leachate plumes in groundwater can be assessed using hydrogeochemical and isotopic signatures of the landfill leachate. This kind of information can be used to provide proper measures for the remediation of landfill-impacted groundwater systems [1,9,10,11]. In fact, several studies [1,8,12,13] have proposed multidisciplinary approaches to supply information about landfill environmental impacts. Landfill leachates can undergo several bio-geochemical reactions, if they are set into surrounding groundwater. Therefore, these bio-geochemical reactions must be traced using parameters as signatures of the leachates and surrounding groundwater [1,14]. In literature, several hydrogeochemical and isotopic approaches have been proposed to study the biogeochemical processes occurring during organic waste degradation to control the leachate impacted aquifers [1,8,13,14,15,16,17,18,19,20]. Parameters such as total dissolved solids (TDS), total suspended solids (TSS), hardness, alkalinity, chloride, chemical oxygen demand (COD), biochemical oxygen demand (BOD), total organic carbon (TOC), and common inorganic ions can provide more information about landfill environmental impacts. A study of hydrogeochemical processes in groundwater in this area of a landfill location, in Umbria (central Italy), has been carried out to understand the impact of the landfill leachates on groundwater quality, in order to distinguish between the rock-water interactions and anthropogenic influences. According to interaction with aquifer minerals or internal mixing of different groundwater along subsurface flow-paths, groundwater can chemically evolve [21]. A basic hydrogeochemical study has been proposed, applied to an aquifer near a landfill in Umbria, such as to consider the evaluation of the chemical-physical parameters (pH, Temperature—T, Electrical Conductivity—EC, redox potential—Eh and Chemical Oxygen Demand—COD) and of groundwater major elements (Na+, K+, Mg2+, Ca2+, SO42−, HCO3−, Cl−, NO3−), in order to verify the suitability as fingerprinting tracers of the leachate pollution. The ratios of major ions provide critical clues to explain different hydrogeochemical processes of water resource [22]. The aim is to assess the impact of the landfill on groundwater quality, to identify the hydrogeochemical processes related to groundwater quality, to carry on a hydrogeochemical evaluation of the aquifer system and to delineate the various factors controlling the water chemistry.
The study area (Figure 1) is characterized by a hilly morphology, with altitude ranging between 500 and 600 m a.s.l. According to Geological Map of Italy (scale 1:100.000), it is possible to identify the presence of two outcropping deposits: upper sandy conglomerate deposits and marly arenaceous formation (Figure 1). The latter outcrops all over the area and it is made of marly and arenaceous layers, alternated with clay and limestone lenses. Moreover, the study area is characterized by marly and arenaceous layers, with low permeability, alternated with limestone lenses which, if fractured, can host suspended aquifers. The landfill plant, designed in the 80s, covers an area of approximately 0.12 km2. Actually, it is used for the storage of municipal solid waste (MSW). In the study area, there is a debris layer, as shown in Figure 1, which has been therefore caused the design of an impermeable layer at the landfill bottom, capable of ensuring a perfect water seal of the landfill bottom. Moreover, an embankment has been located at downstream of the landfill. It is consisting of variable dimensions soil, with lithoid elements of sandstone and marl. On the embankment downstream of the landfill, a series of sub-horizontal drains have been drilled to drain most part of the landfill percolation water. These drains have been made with a slope, such as to drain the percolation water to the downstream of the embankment, towards the drains channels of the shallow waters. During the executive phase, in order to reduce the connection between rainwater and embanked waste and to avoid the production of leachate, drainage channels have been created. They are due to discharge the surface water into the canal at downstream of the embankment. In the end, a final covering with waterproofing layers has been located.
Figure 1 shows groundwater sampling points (identified by "GW" annotation), leachate tanks sampling points (identified by "L" annotation), and drainage water sampling points (identified by "D" annotation). Moreover, for the study a rainfall sample (identified by "RW" annotation) has been considered.
The water sampling survey was carried out during the groundwater monitoring sampling in the rainy season (January 2020) to include any possible dilution phenomena. Two monitoring sampling surveys have been carried out: the first one on January 9th, 2020 (in this study identified as case A), for a total of 12 samples, and the second one on January 27th, 2020 (in this study identified as case B), for a total of 8 samples. They are referred to the same rainy season and this fact makes more sound the output of the elaborations and interpretations of data, here presented. Groundwater sampling has been carried out according to the low flow sampling procedure, established by Environmental Protection Agency [23] for groundwater samples collection from monitoring wells. The sampling procedure provides [23] that, prior to sampling, each well was purged of approximately one to two well volumes until stabilization of temperature (T), electrical conductivity (EC), and pH values in order to ensure the removal of stagnant water and the sampling of water from the aquifer. In fact, for the study case, the monitoring wells purging was accomplished by using a submersible pump, with a pumping rate ranging from 1 to 5 l/min. Moreover, according to the sampling procedure provides [23], the samples were collected in high-density polyethylene (HDPE) bottles and include, for each well, (i) one filtered and unacidified aliquot for alkalinity determination and anion analysis and (ii) one filtered aliquot acidified with ultrapure nitric acid (1% v/v) for analysis of major cations. Filtration was performed in the field with 0.45-μm membrane filters using disposable plastic syringes. The chemical-physical parameters were measured in the field using a multiparameter probe (WTW, 3320). Once collected in HDPE bottles, the samples were stored in ice until delivery to the laboratory and stored at 4 ℃ until analysis. The chemical composition has been determined using standard analytical methods [24]. Bicarbonate was determined on site by titration with 0.1 N HCl using color turning method with methyl orange. According to guidelines [25,26] element concentrations (Na+, K+, Mg2+, Ca2+, SO42−, HCO3−, Cl−, NO3−) were determined at the Geochemistry Laboratory of Sapienza University in Rome with a Dionex ICS5000 ion chromatograph (gradient proportioning accuracy and precision ± 0.5% at 2 mL/min, analytical only) using a Dionex AS9-SC column for anions (eluent—1.8 mMsodiumcarbonate, 1.7 mM sodium bicarbonate; flow rate—2.0 mL/min; injection volume—50 μL; detection suppressed conductivity, ASRS® ULTRA 4-mm, AutoSuppression®, recycle mode) and a Dionex ICS 1100 ion chromatograph (precision ± 5% at 0.25 mL/min, analytical only) using a Dionex CS-12 column for cations. Sigma-Aldrich PRIMUS-Primary Multi-Ion Standards for Ion Chromatography (10 mg/kg of each anion and cation solutions) were used for calibration.
This paragraph shows the results of the chemical-physical parameters (Table 1) and the concentrations of the major elements (Tables 2 and 3), anions and cations, determined with the ion chromatograph. Table 1 shows the results of the chemical-physical parameters (pH, Temperature—T, Electrical Conductivity—EC, redox potential—Eh and Chemical Oxygen Demand—COD).
Case A | |||||
Sample | pH | EC | T | COD | Eh |
μS/cm | ℃ | mg/L O2 | mV | ||
D1 | 7.73 | 1630 | 10.8 | 19 | 162 |
D2 | 7.44 | 5690 | 10.7 | 449 | 167 |
D3 | 8.23 | 1310 | 10.5 | 35 | 171 |
D4 | 7.86 | 1100 | 7.86 | 21 | 175 |
D5 | 8.04 | 1210 | 10.9 | 17 | 171 |
L1 | 8.35 | 11100 | − | 2060 | − |
L2 | 8.45 | 15200 | − | 4590 | − |
GW1 | 8.12 | 1070 | 9.8 | 28 | 133 |
GW2 | 8.32 | 850 | 8.32 | 11 | 172 |
GW3 | 8.25 | 840 | 9.7 | 21 | 151 |
GW4 | 7.75 | 1380 | 7.75 | 34 | 177 |
RW | 6.55 | 11 | 12.3 | 11 | 190 |
Case B | |||||
Sample | pH | EC | T | COD | Eh |
μS/cm | ℃ | mg/L O2 | mV | ||
D2 | 7.37 | 5790 | 10.3 | 479 | 199 |
D6 | 7.94 | 1710 | 10.5 | 22 | 187 |
D3 | 8.01 | 1430 | 10.1 | 29 | 189 |
D4 | 7.96 | 1240 | 10.4 | 11 | 192 |
D7 | 8.03 | 1390 | 10.7 | 10 | 195 |
D5 | 7.91 | 1250 | 10.5 | 9 | 197 |
D8 | 8.05 | 1080 | 10.8 | 9 | 194 |
D9 | 7.65 | 1200 | 10.2 | 11 | 203 |
Case A | |||||||||
Sample | Cations | Anions | |||||||
Ca2+ | Mg2+ | Na+ | K+ | Cl- | SO42- | HCO3- | NO3- | ||
mg/l | mg/l | mg/l | mg/l | mg/l | mg/l | mg/l | mg/l | ||
Detenction Limit | 0.5 | 0.5 | 0.5 | 0.1 | 0.1 | 0.1 | 6 | 0.1 | |
D1 | 281 | 47.7 | 43.8 | 4.0 | 234 | 50.5 | 683 | 5.3 | |
D2 | 306 | 72.4 | 419 | 174 | 980 | 16 | 720 | 0.1 | |
D3 | 235 | 29.9 | 68.2 | 10.4 | 210 | 60.8 | 510 | 12.8 | |
D4 | 199 | 23.2 | 65.4 | 1.6 | 107 | 67.2 | 543 | 3.3 | |
D5 | 259 | 27.9 | 48.9 | 1.1 | 120 | 57.4 | 670 | 0.8 | |
L1 | 133 | 38.2 | 869 | 660 | 1230 | 80.3 | 6470 | 0.1 | |
L2 | 57.7 | 29.7 | 1350 | 1210 | 2200 | 299 | 8400 | 0.1 | |
GW1 | 175 | 26.7 | 37.8 | 1.53 | 91.1 | 127 | 458 | 5.6 | |
GW2 | 129 | 39 | 12.1 | 2.61 | 12.9 | 111 | 485 | 0.84 | |
GW3 | 132 | 25.9 | 16.9 | 2.86 | 28.8 | 101 | 421 | 2.19 | |
GW4 | 223 | 25.1 | 41.3 | 15.8 | 45.4 | 146 | 695 | 28.8 | |
RW | 1.1 | 0.5 | 0.7 | 0.4 | 1.53 | 0.9 | 18 | 0.1 | |
Case B | |||||||||
Sample | Cations | Anions | |||||||
Ca2+ | Mg2+ | Na+ | K+ | Cl- | SO42- | HCO3- | NO3- | ||
mg/l | mg/l | mg/l | mg/l | mg/l | mg/l | mg/l | mg/l | ||
D2 | 299.0 | 70.4 | 476.0 | 196.0 | 950 | 8.4 | 1500 | 0.1 | |
D6 | 365.0 | 20.2 | 48.7 | 4.2 | 241 | 27.6 | 800 | 0.1 | |
D3 | 215.0 | 29.4 | 81.1 | 10.8 | 230 | 64 | 430 | 19.8 | |
D4 | 204.0 | 27.0 | 69.2 | 1.8 | 127 | 67.5 | 540 | 2.64 | |
D7 | 272.0 | 26.0 | 54.3 | 1.2 | 143 | 58.5 | 670 | 0.1 | |
D5 | 236.0 | 30.5 | 46.2 | 1.2 | 140 | 54.3 | 630 | 0.66 | |
D8 | 206.0 | 25.0 | 30.1 | 0.7 | 76.7 | 28.9 | 610 | 0.1 | |
D9 | 228.0 | 24.6 | 47.9 | 1.2 | 84.2 | 68.5 | 653 | 5.45 |
Case A | Δ (%) | ||||||||
Sample | Cations | Anions | |||||||
Ca2+ | Mg2+ | Na+ | K+ | Cl- | SO42- | HCO3- | NO3- | ||
meq/l | meq/l | meq/l | meq/l | meq/l | meq/l | meq/l | meq/l | ||
D1 | 14.02 | 3.92 | 1.90 | 0.10 | 6.60 | 1.05 | 11.19 | 0.09 | 3% |
D2 | 15.27 | 5.95 | 18.22 | 4.45 | 27.64 | 0.33 | 11.80 | 0.00 | 5% |
D3 | 11.73 | 2.46 | 2.97 | 0.27 | 5.92 | 1.27 | 8.359 | 0.21 | 5% |
D4 | 9.93 | 1.91 | 2.84 | 0.04 | 3.02 | 1.40 | 8.90 | 0.05 | 5% |
D5 | 12.92 | 2.29 | 2.13 | 0.03 | 3.38 | 1.20 | 10.98 | 0.01 | 5% |
L1 | 6.64 | 3.14 | 37.78 | 16.88 | 34.69 | 1.67 | 106.04 | 0.00 | −2% |
L2 | 2.88 | 2.44 | 58.70 | 30.95 | 62.04 | 6.23 | 137.68 | 0.00 | −1% |
GW1 | 8.73 | 2.196 | 1.64 | 0.04 | 2.57 | 2.64 | 7.51 | 0.09 | −1% |
GW2 | 6.44 | 3.21 | 0.53 | 0.07 | 0.36 | 2.31 | 7.95 | 0.01 | −2% |
GW3 | 6.59 | 2.13 | 0.73 | 0.07 | 0.81 | 2.10 | 6.90 | 0.04 | −2% |
GW4 | 11.13 | 2.06 | 1.80 | 0.40 | 1.28 | 3.04 | 11.39 | 0.46 | −3% |
RW | 0.0549 | 0.0411 | 0.0304 | 0.0102 | 0.0431 | 0.0187 | 0.2950 | 0.0016 | − |
Case B | Δ (%) | ||||||||
Sample | Cations | Anions | |||||||
Ca2+ | Mg2+ | Na+ | K+ | Cl- | SO42- | HCO3- | NO3- | ||
meq/l | meq/l | meq/l | meq/l | meq/l | meq/l | meq/l | meq/l | ||
D2 | 14.92 | 5.79 | 20.70 | 5.01 | 26.790 | 0.175 | 24.58 | 0.002 | −5% |
D6 | 18.21 | 1.66 | 2.12 | 0.11 | 6.796 | 0.575 | 13.11 | 0.002 | 4% |
D3 | 10.73 | 2.42 | 3.53 | 0.28 | 6.486 | 1.332 | 7.04 | 0.319 | 5% |
D4 | 10.18 | 2.22 | 3.01 | 0.05 | 3.581 | 1.405 | 8.85 | 0.043 | 5% |
D7 | 13.57 | 2.14 | 2.36 | 0.03 | 4.033 | 1.218 | 10.98 | 0.002 | 5% |
D5 | 11.78 | 2.51 | 2.01 | 0.03 | 3.948 | 1.131 | 10.32 | 0.011 | 3% |
D8 | 10.28 | 2.06 | 1.31 | 0.02 | 2.163 | 0.602 | 9.99 | 0.002 | 3% |
D9 | 11.38 | 2.02 | 2.08 | 0.03 | 2.374 | 1.426 | 10.70 | 0.088 | 3% |
Table 1 shows that the D2 sample has high EC, i.e., 5690 μS/cm for case A and 5790 μS/cm for case B. In fact, the L1 sample by leachate tanks showed also comparatively high EC values, i.e., 11,100 μS/cm for case A (Table 1). D2 is a sub-horizontal drain positioned at the embankment downstream of the landfill and it is near L1, a tank used for the leachate storage. Table 2 shows the major elements results. The chemical composition has been determined using standard analytical methods [24]. Bicarbonate was determined on site by titration with 0.1 N HCl using color turning method with methyl orange.
The major elements are expressed in milligrams per liter (mg/L) in Table 2. However, thanks to the conversion factors, the major elements concentrations have been converted in milliequivalents per liter (meq/L) in Table 3.
The analytical precision of the data has been measured using the normalized inorganic charge balance, which is defined as and represents the fractional difference between the total cations () and total anions (), considering both case A and case B. According to the standard method 1030E "checking analyses correctness" [24], the charge balance percentage between anions and cations concentrations sum in meq/L was assessed and analyses were accepted for deviations of < ± 5%.
Analysis results of the groundwater major elements have been plotted with the main geochemistry diagrams: Schoeller, Langelier-Ludwig and Piper. These graphical methods are designed to simultaneously represent the Total Dissolved Solid concentration (TDS) and the relative proportions of certain major ionic species [21,27,28,29,30]. Figure 2 shows the Schoeller's (Figure 2a) and Piper's (Figure 2b) diagram for the case A and Figure 3 shows the Schoeller's (Figure 2 a) and Piper's (Figure 2b) diagram for the case B.
For case B, the diagrams of the groundwater major elements show also the rainfall sample RW and the two leachate samples L1 and L2 of case A in order to perform some comparisons. The geochemical results show that D2 sample has strange geochemical behaviour with respect to the other groundwater samples. In fact, the Schoeller diagram, for case A (Figure 2a) and case B (Figure 3a), shows that for sample D2 (in red in the Figure 2a and Figure 3a) the main cation is Na+, with abundance order of Na+ > Ca2+ > Mg+ > K+, while the anions are dominated by Cl-, with abundance order of Cl- > HCO3- > SO4-. The geochemical behaviour, in fact, is more similar to leachate samples L1 (in orange in Figure 2a) and L2 (in purple in Figure 3a), thus showing a possible phenomenon of groundwater contamination for D2 sample. At the same time, the Piper diagram (Figure 2b and Figure 3b) shows that these groundwater samples (GW1 – GW4) are characterized by a prevalence of magnesium bicarbonate facies. On the contrary, sample D2 is located in the Na+–Cl-–HCO3- mixing zone (Figure 2b and Figure 3b). Leachate samples L1 and L2, as well as sample D2, in the study area are highly enriched in Na+, Cl-, and HCO3- due to waste dissolution and degradation processes [1]. The groundwater chemistry has evolved from Ca2+–HCO3- association to Na+–Cl-–HCO3- association, due to the presence of leachate on groundwater chemistry (Figure 2b and Figure 3b). Moreover, Figure 4 shows the Langelier-Ludwig diagram.
Figure 4 shows only the samples carried out during both monitoring sampling surveys. The drainage water samples D3, D4, D5 and the rainfall sample RW show a prevalence of the bicarbonate (HCO3-) ions, thus proving a magnesium bicarbonate facies. The sample D2 shows a prevalence of the Na+ + K+ cationic association and bicarbonate, as well as samples L1 and L2.
Bivariate major element versus TDS diagrams (Figure 5 and Figure 6) are, usually, applied to assess the geochemical processes, and in particular to identify possible contamination between leachate and groundwater [15,16,17,30]. In the following elaborations, for case B, the chemical results of the groundwater samples and leachate samples L1 and L2 of the case A have been considered, due to lack of data.
Figure 5 (a–c) shows a statistically significant linear trend (R2 ≥ 0.9, N = 12) between TDS and Na+ (Figure 5a), Cl- (Figure 5b) and HCO3- (Figure 5c) for the case A. However, sample D2 has an anomalous geochemical behaviour, closer to L1 and L2 leachate samples, in comparison with the other samples, which in fact are closer to the origin where the rainfall sample RW is present. On the contrary, sample D2 has very high concentrations of Na+ (419 mg/L) and Cl- (980 mg/L) and HCO3- (720 mg/L), as well as a high TDS content (2690.52 mg/L), closer to the behaviour to L1 and L2 leachate samples. This is also highlighted by Case B, in Figure 6 (a–c), verifying a statistically significant linear trend (R2 = 0.9, N = 15). In fact, for case B, the sample D2 shows a geochemical behaviour as well as the leachate samples L1 and L2, with very high concentrations of Na+ (476 mg/L), Cl- (950 mg/L), HCO3- (1500 mg/L). Moreover, Figure 7 (a and b) shows a bivariate SO4- versus TDS diagram for the two cases.
Figure 7 (a–b) shows geochemical trends for groundwater samples (GW) similar to each other, but nevertheless different from drainage water samples (D). However, sample D2 both in case A (Figure 7a) and in case B (Figure 7b) appears to have geochemical behaviours same as leachate samples L1 and L2, with high TDS values. However, the extent of landfill impact on groundwater has been assessed by comparing the chemistry of groundwater, drainage water and leachates, combining with the hydrogeological information about probable flow paths [30]. In fact, the geochemical variations in the ionic concentrations in groundwater and drainage water can easily be understood, when they are plotted along an X–Y coordinate [17,21,27]. Results from the chemical analyses were used to identify the geochemical processes and mechanisms in the groundwater aquifer system and to assess possible contamination phenomena (Figure 8 a–d).
Scatter plots have been performed between major elements: Cl- versus Na+, for case A (Figure 8a) and for case B (Figure 8c), Cl- versus HCO3-, for case A (Figure 8b) and for case B (Figure 8d). Na+ concentrations is positively correlated with Cl- content [1,31], with R2 equal to 0.9782 for case A (Figure 8a) and equal to 0.9853 for case B (Figure 8c). However, the drainage water sample D2 and the leachate samples L1 and L2 show anomalous geochemical behaviour due to high content of chloride (Cl-) concentrations. In fact, according to Olosson et al., 2005 [16], most composite landfills produce a leachate rich in chloride since chloride ions usually follow the water flow. In fact, Cl- is a conservative tracer of the direct influence of landfill leachate [1] as high Cl- concentrations can indicate an external input from the landfill, like leachate, as samples L1 shows, since there is no possible natural source of chloride in the study area. Moreover, the trend of chloride versus bicarbonates has been also represented, for case A in Figure 8b and for case B in Figure 8d. These scatter plots show high HCO3- concentrations for sample D2 and for leachate samples L1 and L2. In fact, the Piper diagram in Figure 2b and Figure 3b have been shown that the sample D2, as well as the leachate samples L1 and L2, are located in the Na+ – Cl- –HCO3- mixing zone due to waste dissolution and degradation processes [1]. This is supported by the positive correlation between Cl- and HCO3- concentrations, with R2 = 0.856 for case A in Figure 8b and equal to 0.896 for case B in Figure 8d, indicating the influence of the leachate plumes [1]. Furthermore, to verify possible impacts of leachate on groundwater and drainage water samples, comparisons between magnesium (Mg2+) and potassium (K+) concentrations have been performed through a scatter plot on log scale (Figure 9 a, b).
The Mg2+ versus K+ graph in Figure 9 on log scale highlights significant differences between the sample's compositions [30]. Figure 9 shows that leachate L1 and L2 has the highest K+/Mg2+ratio (symbol size) [30], due to high values of potassium, as well as the drainage water sample D2, whose geochemical characteristics are influenced by leachate contamination. For case A, the drainage water sample D2 shows a K+/Mg2+ ratio equal to 0.748 (Figure 9a) and for case B the K+/Mg2+ ratio is equal to 0.866 (Figure 9b). The leachate in the study site is highly enriched in Na+, K+ and HCO3- due to waste dissolution and degradation processes [1,30]. Moreover, a plot of the ratio magnesium/chloride (Mg2+/Cl-) versus sodium/chloride (Na+/Cl-) [16] have been shown in Figure 10 (a, b).
Figure 10 (a, b) shows that the groundwater samples are characterized by high Mg2+/Cl- ratios, because they have high Mg2+ concentrations, according to magnesium bicarbonate facies of aquifer, shown in Piper diagram (Figure 2a and Figure 3b). On the contrary, the leachate samples L1 and L2 have high Na+/Cl- due to high Cl- concentrations, produced by landfills [16]. In fact, D2 sample stretch along a line towards leachate, confirming contamination phenomena.
Groundwater is one of the most important naturale resource for different uses water supply, worldwide. This research indicates that the water-rock interaction affects, sensitively, groundwater quality, especially when the main groundwater flow system is local. The hydrogeochemical results confirm the leachate contamination in some drainage samples. This shows that the leachate samples are highly enriched in Na+, K+, HCO3- and Cl- due to waste dissolution and degradation processes. The correlation between Cl- versus Na+ (for case A in Figure 8a and for case B in Figure 8c) e between Cl- versus HCO3- (for case A in Figure 8b and for case B in Figure 8d) show that D2 are enriched in Cl-, as well as in HCO3-. In fact, according to Olosson et al., 2005 [16], most composite landfills produce a leachate rich in chloride since chloride ions usually follow the water flow. The ion Cl- is a conservative tracer that may indicate the direct influence of landfill leachate[1]. Therefore, the high Cl- concentrations in D2 sample can indicate an external input from the landfill, maybe from leachate samples. At the same time, the correlation between Mg2+ and K+, shown on log scale graph in Figure 9 (a, b), confirms a contamination of D2 sample by leachate. The highest K+/Mg2+ratio for D2 sample, as well as for L1 and L2 leachate samples, shows high concentrations of potassium due to waste dissolution and degradation processes [1,30].
Therefore, performing monitoring programs of geochemical parameters, in correspondence with municipal solid waste landfills (MSW), is useful for assess and control possible contamination phenomena by leachate. In fact, a particular attention should be paid to the wells situated down gradient of the landfill and in the direction of groundwater flow.
The authors declare no conflict of interest.
[1] |
Lee KS, Ko KS, Kim EY (2020) Application of stable isotopes and dissolved ions for monitoring landfill leachate contamination. Environ Geochem Health 42: 1387–1399. https://doi.org/10.1007/s10653-019-00427-y doi: 10.1007/s10653-019-00427-y
![]() |
[2] |
Mohammadzadeh H, Clark I (2008) Degradation pathways of dissolved carbon in landfill leachate traced with compound-specific 13C analysis of DOC. Isotopes Environ Health Stud 44: 267–294. https://doi.org/10.1080/10256010802309814 doi: 10.1080/10256010802309814
![]() |
[3] |
Mukherjee S, Mukhopadhyay S, Hashim MA, et al. (2015) Contemporary environmental issues of landfill leachate: Assessment and remedies. Crit Rev Environ Sci Technol 45: 472–590. https://doi.org/10.1080/10643389.2013.876524 doi: 10.1080/10643389.2013.876524
![]() |
[4] |
Qin M, Molitor H, Brazil B, et al. (2016) Recovery of nitrogen and water from landfill leachate by a microbial electrolysis cell-forward osmosis system. Bioresour Technol 200: 485–492. https://doi.org/10.1016/j.biortech.2015.10.066 doi: 10.1016/j.biortech.2015.10.066
![]() |
[5] |
Adeolu AO, Ada OV, Gbenga AA, et al. (2011) Assessment of groundwater contamination by leachate near a municipal solid waste landfill. Afr J Environ Sci Technol 5: 933–940. https://doi.org/10.5897/AJEST11.272 doi: 10.5897/AJEST11.272
![]() |
[6] |
Liu ZP, Wu WH, Shi P, et al. (2015) Characterization of dissolved organic matter in landfill leachate during the combined treatment process of air stripping, Fenton, SBR and coagulation. Waste Manag 41: 111–118. https://doi.org/10.1016/j.wasman.2015.03.044 doi: 10.1016/j.wasman.2015.03.044
![]() |
[7] |
Foo KY, Hameed BH (2009) An overview of landfill leachate treatment via activated carbon adsorption process. J Hazard Mater 171: 54–60. https://doi.org/10.1016/j.jhazmat.2009.06.038 doi: 10.1016/j.jhazmat.2009.06.038
![]() |
[8] |
De Medeiros Engelmann P, dos Santos VHJM, Barbieri CB, et al. (2018) Environmental monitoring of a landfill area through the application of carbon stable isotopes, chemical parameters and multivariate analysis. Waste Manage 76: 591–605. https://doi.org/10.1016/j.wasman.2018.02.027 doi: 10.1016/j.wasman.2018.02.027
![]() |
[9] |
Castañeda SS, Sucgang RJ, Almoneda RV., et al. (2012) Environmental isotopes and major ions for tracing leachate contamination from a municipal landfill in Metro Manila, Philippines. J Environ Radioact 110: 30–37. https://doi.org/10.1016/j.jenvrad.2012.01.022 doi: 10.1016/j.jenvrad.2012.01.022
![]() |
[10] |
Hackley LC, Liu CI, Coleman DD (1996) Environmental Isotope Characteristics of Landfill Leachates and Gases. Groundwater 34: 827–836. https://doi.org/10.1111/j.1745-6584.1996.tb02077.x doi: 10.1111/j.1745-6584.1996.tb02077.x
![]() |
[11] |
North JC, Frew RD, van Hale R (2006) Can stable isotopes be used to monitor landfill leachate impact on surface waters? J Geochem Explor 88: 49–53. https://doi.org/10.1016/j.gexplo.2005.08.003 doi: 10.1016/j.gexplo.2005.08.003
![]() |
[12] |
Naveen BP, Mahapatra DM, Sitharam TG, et al. (2017) Physico-chemical and biological characterization of urban municipal landfill leachate. Environ Pollut 220: 1–12. https://doi.org/10.1016/j.envpol.2016.09.002 doi: 10.1016/j.envpol.2016.09.002
![]() |
[13] |
Nigro A, Sappa G, Barbieri M (2017) Application of boron and tritium isotopes for tracing landfill contamination in groundwater. J Geochem Explor 172: 101–108. https://doi.org/10.1016/j.gexplo.2016.10.011 doi: 10.1016/j.gexplo.2016.10.011
![]() |
[14] |
Cozzarelli IM, Böhlke JK, Masoner J, et al. (2011) Biogeochemical evolution of a landfill leachate plume, Norman, Oklahoma. Ground Water 49: 663–687. https://doi.org/10.1111/j.1745-6584.2010.00792.x doi: 10.1111/j.1745-6584.2010.00792.x
![]() |
[15] |
Krishan G, Sejwal P, Bhagwat A, et al. (2021) Role of Ion Chemistry and Hydro-Geochemical Processes in Aquifer Salinization—A Case Study from a Semi-Arid Region of Haryana, India. Water 13: 617. https://doi.org/10.3390/w13050617 doi: 10.3390/w13050617
![]() |
[16] |
Olofsson B, Jernberg H, Rosenqvist A (2006) Tracing leachates at waste sites using geophysical and geochemical modelling. Environ Geol 49: 720–732. https://doi.org/10.1007/s00254-005-0117-9 doi: 10.1007/s00254-005-0117-9
![]() |
[17] |
Najet T, Belgacem A, Adel K (2019) Assessment of groundwater quality of El Ouara aquifer (southeastern Tunisia), geochemical and isotopic approaches. Arab J Geosci 12: 116. https://doi.org/10.1007/s12517-018-4203-1 doi: 10.1007/s12517-018-4203-1
![]() |
[18] |
Andrei F, Barbieri M, Sappa G (2021) Application of 2H and 18O isotopes for tracing municipal solid waste landfill contamination of groundwater: Two Italian case histories. Water 13: 1065. https://doi.org/10.3390/w13081065 doi: 10.3390/w13081065
![]() |
[19] |
Nigro A, Sappa G, Barbieri M (2018) Boron isotopes and rare earth elements in the groundwater of a landfill site. J Geochem Explor 190: 200–206. https://doi.org/10.1016/j.gexplo.2018.02.019 doi: 10.1016/j.gexplo.2018.02.019
![]() |
[20] |
Wimmer B, Hrad M, Huber-Humer M, et al. (2013) Stable isotope signatures for characterising the biological stability of landfilled municipal solid waste. Waste Manag 33: 2083–2090. https://doi.org/10.1016/j.wasman.2013.02.017 doi: 10.1016/j.wasman.2013.02.017
![]() |
[21] |
Srivastava SK, Ramanathan AL (2008) Geochemical assessment of groundwater quality in vicinity of Bhalswa landfill, Delhi, India, using graphical and multivariate statistical methods. Environ Geol 53: 1509–1528. https://doi.org/10.1007/s00254-007-0762-2 doi: 10.1007/s00254-007-0762-2
![]() |
[22] |
Zhang Y, Xu M, Li X, et al. (2018) Hydrochemical characteristics and multivariate statistical analysis of natural water system: A case study in Kangding County, Southwestern China. Water 10: 80. https://doi.org/10.3390/w10010080 doi: 10.3390/w10010080
![]() |
[23] | US. ENVIRONMENTAL PROTECTION AGENCY (2017) Low Stress (low flow) Purging and Sampling Procedure for the Collection of Groundwater Samples From Monitoring Wells. Available from: https://www.epa.gov/sites/default/files/2017-10/documents/eqasop-gw4.pdf |
[24] | APHA AWWA WEF (2017) Standard Methods for examination of water and wastewater. 23rd Edition. American Public Health Association (APHA), the American Water Works Association (AWWA), and the Water Environment Federation (WEF). |
[25] | APAT, CNR, IRSA (2003) 3010—Metalli e Specie Metalliche. Available from: https://www.irsa.cnr.it/wp/wp-content/uploads/2022/04/Vol1_Sez_3000_Metalli.pdf |
[26] | APAT, CNR, IRSA (2003) 3020—Determinazione di elementi chimici mediante spettroscopia di emissione con sorgente al plasma (ICP-OES). Available from: https://www.irsa.cnr.it/wp/wp-content/uploads/2022/04/Vol1_Sez_3000_Metalli.pdf |
[27] | APAT, CNR, IRSA (2003) 4020—Anioni (fluoruro, cloruro, nitrito, bromuro, nitrato, fosfato e solfato) in cromatografia ionica. Available from: https://www.irsa.cnr.it/wp/wp-content/uploads/2022/04/Vol2_Sez_4000_InorganiciNonMetallici.pdf |
[28] |
Güler C, Thyne GD, McCray JE, et al. (2002) Evaluation of graphical and multivariate statistical methods for classification of water chemistry data. Hydrogeol J 10: 455–474. https://doi.org/10.1007/s10040-002-0196-6 doi: 10.1007/s10040-002-0196-6
![]() |
[29] |
Boschetti T (2011) Application of brine differentiation and Langelier—Ludwig plots to fresh-to-brine waters from sedimentary basins: Diagnostic potentials and limits. J Geochem Explor 108: 126–130. https://doi.org/10.1016/j.gexplo.2010.12.002 doi: 10.1016/j.gexplo.2010.12.002
![]() |
[30] |
Singh UK, Kumar M, Chauhan R, et al. (2008) Assessment of the impact of landfill on groundwater quality: A case study of the Pirana site in western India. Environ Monit Assess 141: 309–321. https://doi.org/10.1007/s10661-007-9897-6 doi: 10.1007/s10661-007-9897-6
![]() |
[31] |
Wang H, Chen Q, Wei J, et al. (2020) Geochemical Characteristics and Influencing Factors of Groundwater Fe in Seawater Intrusion Area. Water Air Soil Pollut 231: 348. https://doi.org/10.1007/s11270-020-04724-6 doi: 10.1007/s11270-020-04724-6
![]() |
Case A | |||||
Sample | pH | EC | T | COD | Eh |
μS/cm | ℃ | mg/L O2 | mV | ||
D1 | 7.73 | 1630 | 10.8 | 19 | 162 |
D2 | 7.44 | 5690 | 10.7 | 449 | 167 |
D3 | 8.23 | 1310 | 10.5 | 35 | 171 |
D4 | 7.86 | 1100 | 7.86 | 21 | 175 |
D5 | 8.04 | 1210 | 10.9 | 17 | 171 |
L1 | 8.35 | 11100 | − | 2060 | − |
L2 | 8.45 | 15200 | − | 4590 | − |
GW1 | 8.12 | 1070 | 9.8 | 28 | 133 |
GW2 | 8.32 | 850 | 8.32 | 11 | 172 |
GW3 | 8.25 | 840 | 9.7 | 21 | 151 |
GW4 | 7.75 | 1380 | 7.75 | 34 | 177 |
RW | 6.55 | 11 | 12.3 | 11 | 190 |
Case B | |||||
Sample | pH | EC | T | COD | Eh |
μS/cm | ℃ | mg/L O2 | mV | ||
D2 | 7.37 | 5790 | 10.3 | 479 | 199 |
D6 | 7.94 | 1710 | 10.5 | 22 | 187 |
D3 | 8.01 | 1430 | 10.1 | 29 | 189 |
D4 | 7.96 | 1240 | 10.4 | 11 | 192 |
D7 | 8.03 | 1390 | 10.7 | 10 | 195 |
D5 | 7.91 | 1250 | 10.5 | 9 | 197 |
D8 | 8.05 | 1080 | 10.8 | 9 | 194 |
D9 | 7.65 | 1200 | 10.2 | 11 | 203 |
Case A | |||||||||
Sample | Cations | Anions | |||||||
Ca2+ | Mg2+ | Na+ | K+ | Cl- | SO42- | HCO3- | NO3- | ||
mg/l | mg/l | mg/l | mg/l | mg/l | mg/l | mg/l | mg/l | ||
Detenction Limit | 0.5 | 0.5 | 0.5 | 0.1 | 0.1 | 0.1 | 6 | 0.1 | |
D1 | 281 | 47.7 | 43.8 | 4.0 | 234 | 50.5 | 683 | 5.3 | |
D2 | 306 | 72.4 | 419 | 174 | 980 | 16 | 720 | 0.1 | |
D3 | 235 | 29.9 | 68.2 | 10.4 | 210 | 60.8 | 510 | 12.8 | |
D4 | 199 | 23.2 | 65.4 | 1.6 | 107 | 67.2 | 543 | 3.3 | |
D5 | 259 | 27.9 | 48.9 | 1.1 | 120 | 57.4 | 670 | 0.8 | |
L1 | 133 | 38.2 | 869 | 660 | 1230 | 80.3 | 6470 | 0.1 | |
L2 | 57.7 | 29.7 | 1350 | 1210 | 2200 | 299 | 8400 | 0.1 | |
GW1 | 175 | 26.7 | 37.8 | 1.53 | 91.1 | 127 | 458 | 5.6 | |
GW2 | 129 | 39 | 12.1 | 2.61 | 12.9 | 111 | 485 | 0.84 | |
GW3 | 132 | 25.9 | 16.9 | 2.86 | 28.8 | 101 | 421 | 2.19 | |
GW4 | 223 | 25.1 | 41.3 | 15.8 | 45.4 | 146 | 695 | 28.8 | |
RW | 1.1 | 0.5 | 0.7 | 0.4 | 1.53 | 0.9 | 18 | 0.1 | |
Case B | |||||||||
Sample | Cations | Anions | |||||||
Ca2+ | Mg2+ | Na+ | K+ | Cl- | SO42- | HCO3- | NO3- | ||
mg/l | mg/l | mg/l | mg/l | mg/l | mg/l | mg/l | mg/l | ||
D2 | 299.0 | 70.4 | 476.0 | 196.0 | 950 | 8.4 | 1500 | 0.1 | |
D6 | 365.0 | 20.2 | 48.7 | 4.2 | 241 | 27.6 | 800 | 0.1 | |
D3 | 215.0 | 29.4 | 81.1 | 10.8 | 230 | 64 | 430 | 19.8 | |
D4 | 204.0 | 27.0 | 69.2 | 1.8 | 127 | 67.5 | 540 | 2.64 | |
D7 | 272.0 | 26.0 | 54.3 | 1.2 | 143 | 58.5 | 670 | 0.1 | |
D5 | 236.0 | 30.5 | 46.2 | 1.2 | 140 | 54.3 | 630 | 0.66 | |
D8 | 206.0 | 25.0 | 30.1 | 0.7 | 76.7 | 28.9 | 610 | 0.1 | |
D9 | 228.0 | 24.6 | 47.9 | 1.2 | 84.2 | 68.5 | 653 | 5.45 |
Case A | Δ (%) | ||||||||
Sample | Cations | Anions | |||||||
Ca2+ | Mg2+ | Na+ | K+ | Cl- | SO42- | HCO3- | NO3- | ||
meq/l | meq/l | meq/l | meq/l | meq/l | meq/l | meq/l | meq/l | ||
D1 | 14.02 | 3.92 | 1.90 | 0.10 | 6.60 | 1.05 | 11.19 | 0.09 | 3% |
D2 | 15.27 | 5.95 | 18.22 | 4.45 | 27.64 | 0.33 | 11.80 | 0.00 | 5% |
D3 | 11.73 | 2.46 | 2.97 | 0.27 | 5.92 | 1.27 | 8.359 | 0.21 | 5% |
D4 | 9.93 | 1.91 | 2.84 | 0.04 | 3.02 | 1.40 | 8.90 | 0.05 | 5% |
D5 | 12.92 | 2.29 | 2.13 | 0.03 | 3.38 | 1.20 | 10.98 | 0.01 | 5% |
L1 | 6.64 | 3.14 | 37.78 | 16.88 | 34.69 | 1.67 | 106.04 | 0.00 | −2% |
L2 | 2.88 | 2.44 | 58.70 | 30.95 | 62.04 | 6.23 | 137.68 | 0.00 | −1% |
GW1 | 8.73 | 2.196 | 1.64 | 0.04 | 2.57 | 2.64 | 7.51 | 0.09 | −1% |
GW2 | 6.44 | 3.21 | 0.53 | 0.07 | 0.36 | 2.31 | 7.95 | 0.01 | −2% |
GW3 | 6.59 | 2.13 | 0.73 | 0.07 | 0.81 | 2.10 | 6.90 | 0.04 | −2% |
GW4 | 11.13 | 2.06 | 1.80 | 0.40 | 1.28 | 3.04 | 11.39 | 0.46 | −3% |
RW | 0.0549 | 0.0411 | 0.0304 | 0.0102 | 0.0431 | 0.0187 | 0.2950 | 0.0016 | − |
Case B | Δ (%) | ||||||||
Sample | Cations | Anions | |||||||
Ca2+ | Mg2+ | Na+ | K+ | Cl- | SO42- | HCO3- | NO3- | ||
meq/l | meq/l | meq/l | meq/l | meq/l | meq/l | meq/l | meq/l | ||
D2 | 14.92 | 5.79 | 20.70 | 5.01 | 26.790 | 0.175 | 24.58 | 0.002 | −5% |
D6 | 18.21 | 1.66 | 2.12 | 0.11 | 6.796 | 0.575 | 13.11 | 0.002 | 4% |
D3 | 10.73 | 2.42 | 3.53 | 0.28 | 6.486 | 1.332 | 7.04 | 0.319 | 5% |
D4 | 10.18 | 2.22 | 3.01 | 0.05 | 3.581 | 1.405 | 8.85 | 0.043 | 5% |
D7 | 13.57 | 2.14 | 2.36 | 0.03 | 4.033 | 1.218 | 10.98 | 0.002 | 5% |
D5 | 11.78 | 2.51 | 2.01 | 0.03 | 3.948 | 1.131 | 10.32 | 0.011 | 3% |
D8 | 10.28 | 2.06 | 1.31 | 0.02 | 2.163 | 0.602 | 9.99 | 0.002 | 3% |
D9 | 11.38 | 2.02 | 2.08 | 0.03 | 2.374 | 1.426 | 10.70 | 0.088 | 3% |
Case A | |||||
Sample | pH | EC | T | COD | Eh |
μS/cm | ℃ | mg/L O2 | mV | ||
D1 | 7.73 | 1630 | 10.8 | 19 | 162 |
D2 | 7.44 | 5690 | 10.7 | 449 | 167 |
D3 | 8.23 | 1310 | 10.5 | 35 | 171 |
D4 | 7.86 | 1100 | 7.86 | 21 | 175 |
D5 | 8.04 | 1210 | 10.9 | 17 | 171 |
L1 | 8.35 | 11100 | − | 2060 | − |
L2 | 8.45 | 15200 | − | 4590 | − |
GW1 | 8.12 | 1070 | 9.8 | 28 | 133 |
GW2 | 8.32 | 850 | 8.32 | 11 | 172 |
GW3 | 8.25 | 840 | 9.7 | 21 | 151 |
GW4 | 7.75 | 1380 | 7.75 | 34 | 177 |
RW | 6.55 | 11 | 12.3 | 11 | 190 |
Case B | |||||
Sample | pH | EC | T | COD | Eh |
μS/cm | ℃ | mg/L O2 | mV | ||
D2 | 7.37 | 5790 | 10.3 | 479 | 199 |
D6 | 7.94 | 1710 | 10.5 | 22 | 187 |
D3 | 8.01 | 1430 | 10.1 | 29 | 189 |
D4 | 7.96 | 1240 | 10.4 | 11 | 192 |
D7 | 8.03 | 1390 | 10.7 | 10 | 195 |
D5 | 7.91 | 1250 | 10.5 | 9 | 197 |
D8 | 8.05 | 1080 | 10.8 | 9 | 194 |
D9 | 7.65 | 1200 | 10.2 | 11 | 203 |
Case A | |||||||||
Sample | Cations | Anions | |||||||
Ca2+ | Mg2+ | Na+ | K+ | Cl- | SO42- | HCO3- | NO3- | ||
mg/l | mg/l | mg/l | mg/l | mg/l | mg/l | mg/l | mg/l | ||
Detenction Limit | 0.5 | 0.5 | 0.5 | 0.1 | 0.1 | 0.1 | 6 | 0.1 | |
D1 | 281 | 47.7 | 43.8 | 4.0 | 234 | 50.5 | 683 | 5.3 | |
D2 | 306 | 72.4 | 419 | 174 | 980 | 16 | 720 | 0.1 | |
D3 | 235 | 29.9 | 68.2 | 10.4 | 210 | 60.8 | 510 | 12.8 | |
D4 | 199 | 23.2 | 65.4 | 1.6 | 107 | 67.2 | 543 | 3.3 | |
D5 | 259 | 27.9 | 48.9 | 1.1 | 120 | 57.4 | 670 | 0.8 | |
L1 | 133 | 38.2 | 869 | 660 | 1230 | 80.3 | 6470 | 0.1 | |
L2 | 57.7 | 29.7 | 1350 | 1210 | 2200 | 299 | 8400 | 0.1 | |
GW1 | 175 | 26.7 | 37.8 | 1.53 | 91.1 | 127 | 458 | 5.6 | |
GW2 | 129 | 39 | 12.1 | 2.61 | 12.9 | 111 | 485 | 0.84 | |
GW3 | 132 | 25.9 | 16.9 | 2.86 | 28.8 | 101 | 421 | 2.19 | |
GW4 | 223 | 25.1 | 41.3 | 15.8 | 45.4 | 146 | 695 | 28.8 | |
RW | 1.1 | 0.5 | 0.7 | 0.4 | 1.53 | 0.9 | 18 | 0.1 | |
Case B | |||||||||
Sample | Cations | Anions | |||||||
Ca2+ | Mg2+ | Na+ | K+ | Cl- | SO42- | HCO3- | NO3- | ||
mg/l | mg/l | mg/l | mg/l | mg/l | mg/l | mg/l | mg/l | ||
D2 | 299.0 | 70.4 | 476.0 | 196.0 | 950 | 8.4 | 1500 | 0.1 | |
D6 | 365.0 | 20.2 | 48.7 | 4.2 | 241 | 27.6 | 800 | 0.1 | |
D3 | 215.0 | 29.4 | 81.1 | 10.8 | 230 | 64 | 430 | 19.8 | |
D4 | 204.0 | 27.0 | 69.2 | 1.8 | 127 | 67.5 | 540 | 2.64 | |
D7 | 272.0 | 26.0 | 54.3 | 1.2 | 143 | 58.5 | 670 | 0.1 | |
D5 | 236.0 | 30.5 | 46.2 | 1.2 | 140 | 54.3 | 630 | 0.66 | |
D8 | 206.0 | 25.0 | 30.1 | 0.7 | 76.7 | 28.9 | 610 | 0.1 | |
D9 | 228.0 | 24.6 | 47.9 | 1.2 | 84.2 | 68.5 | 653 | 5.45 |
Case A | Δ (%) | ||||||||
Sample | Cations | Anions | |||||||
Ca2+ | Mg2+ | Na+ | K+ | Cl- | SO42- | HCO3- | NO3- | ||
meq/l | meq/l | meq/l | meq/l | meq/l | meq/l | meq/l | meq/l | ||
D1 | 14.02 | 3.92 | 1.90 | 0.10 | 6.60 | 1.05 | 11.19 | 0.09 | 3% |
D2 | 15.27 | 5.95 | 18.22 | 4.45 | 27.64 | 0.33 | 11.80 | 0.00 | 5% |
D3 | 11.73 | 2.46 | 2.97 | 0.27 | 5.92 | 1.27 | 8.359 | 0.21 | 5% |
D4 | 9.93 | 1.91 | 2.84 | 0.04 | 3.02 | 1.40 | 8.90 | 0.05 | 5% |
D5 | 12.92 | 2.29 | 2.13 | 0.03 | 3.38 | 1.20 | 10.98 | 0.01 | 5% |
L1 | 6.64 | 3.14 | 37.78 | 16.88 | 34.69 | 1.67 | 106.04 | 0.00 | −2% |
L2 | 2.88 | 2.44 | 58.70 | 30.95 | 62.04 | 6.23 | 137.68 | 0.00 | −1% |
GW1 | 8.73 | 2.196 | 1.64 | 0.04 | 2.57 | 2.64 | 7.51 | 0.09 | −1% |
GW2 | 6.44 | 3.21 | 0.53 | 0.07 | 0.36 | 2.31 | 7.95 | 0.01 | −2% |
GW3 | 6.59 | 2.13 | 0.73 | 0.07 | 0.81 | 2.10 | 6.90 | 0.04 | −2% |
GW4 | 11.13 | 2.06 | 1.80 | 0.40 | 1.28 | 3.04 | 11.39 | 0.46 | −3% |
RW | 0.0549 | 0.0411 | 0.0304 | 0.0102 | 0.0431 | 0.0187 | 0.2950 | 0.0016 | − |
Case B | Δ (%) | ||||||||
Sample | Cations | Anions | |||||||
Ca2+ | Mg2+ | Na+ | K+ | Cl- | SO42- | HCO3- | NO3- | ||
meq/l | meq/l | meq/l | meq/l | meq/l | meq/l | meq/l | meq/l | ||
D2 | 14.92 | 5.79 | 20.70 | 5.01 | 26.790 | 0.175 | 24.58 | 0.002 | −5% |
D6 | 18.21 | 1.66 | 2.12 | 0.11 | 6.796 | 0.575 | 13.11 | 0.002 | 4% |
D3 | 10.73 | 2.42 | 3.53 | 0.28 | 6.486 | 1.332 | 7.04 | 0.319 | 5% |
D4 | 10.18 | 2.22 | 3.01 | 0.05 | 3.581 | 1.405 | 8.85 | 0.043 | 5% |
D7 | 13.57 | 2.14 | 2.36 | 0.03 | 4.033 | 1.218 | 10.98 | 0.002 | 5% |
D5 | 11.78 | 2.51 | 2.01 | 0.03 | 3.948 | 1.131 | 10.32 | 0.011 | 3% |
D8 | 10.28 | 2.06 | 1.31 | 0.02 | 2.163 | 0.602 | 9.99 | 0.002 | 3% |
D9 | 11.38 | 2.02 | 2.08 | 0.03 | 2.374 | 1.426 | 10.70 | 0.088 | 3% |