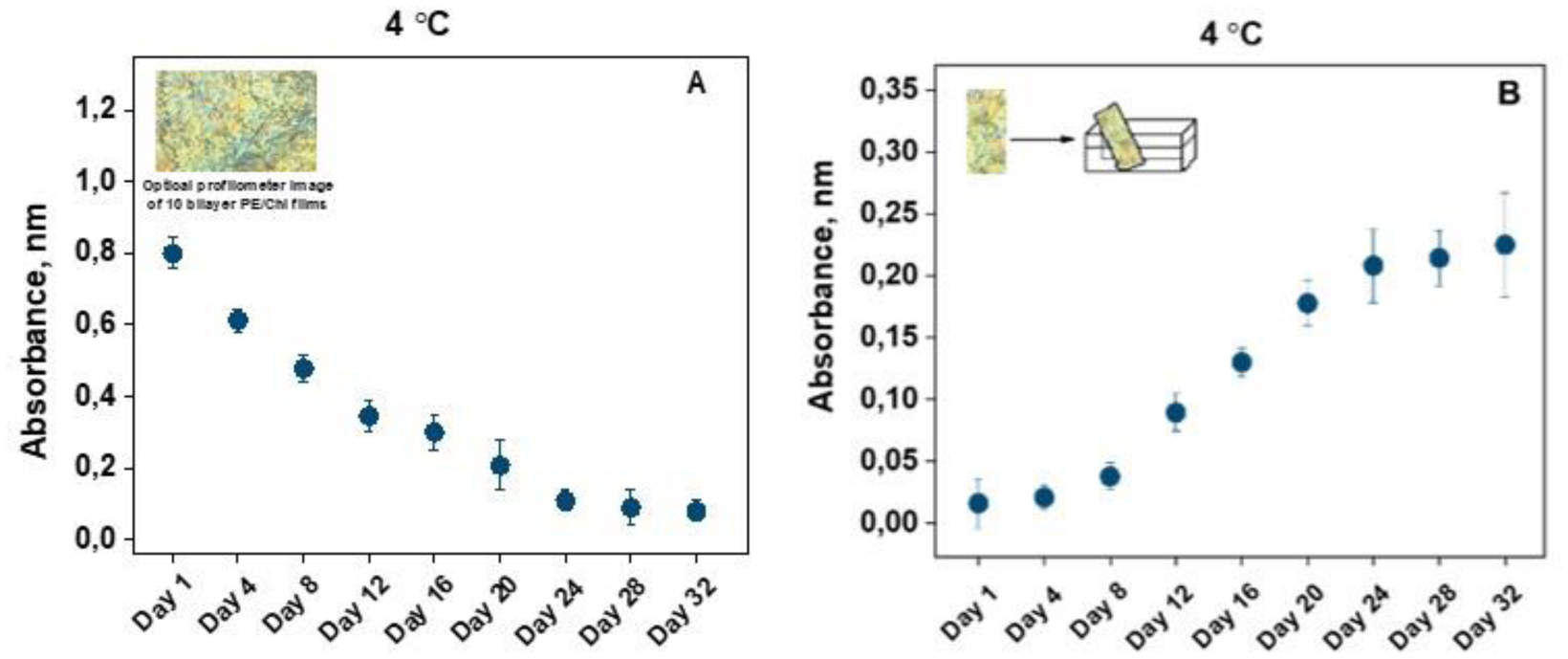
Performing their functions as transporters of oxygen and carbon dioxide in the body, human erythrocytes constantly circulate and are exposed to the constant influence of various substances, including nutrients, drugs, medical devices covered with coatings, etc. Therefore, we aim to investigate the biophysical behavior of erythrocytes obtained from healthy volunteers to observe their morphological type changes, alterations in the zeta potential, the electrical conductivity of the erythrocytes in suspensions, and hemolysis in percentages during cells senescence, both in presence and in absence of natural polyelectrolytes pectin (PE) and chitosan (Chi) in form of multilayer films (PEM-films). Being constructed using the layer–by–layer technique, films are an object of interest of many researchers because of their high potential to be incorporated in biomedicine. By applying optical profilometry, electrophoretic light scattering, and spectrophotometry, we tested the polyelectrolytes for any potential harm on the erythrocytes. Based on our results and the one-way analysis of variance (ANOVA) statistical analysis, we reached the conclusion that the above-mentioned polyelectrolytes were harmlessness; therefore, PE and Chi are suitable substances to implement in the clinical practice in the form of drug delivery carriers and medical devices coatings, thereby directly contacting with the human blood.
Citation: Nikolay Kalaydzhiev, Elena Zlatareva, Dessislava Bogdanova, Svetozar Stoichev, Avgustina Danailova. Changes in biophysical properties and behavior of aging human erythrocytes treated with natural polyelectrolytes[J]. AIMS Biophysics, 2025, 12(1): 14-28. doi: 10.3934/biophy.2025002
[1] | Mohamed A. Elblbesy . Impact of erythrocytes properties on blood surface tension. AIMS Biophysics, 2019, 6(2): 39-46. doi: 10.3934/biophy.2019.2.39 |
[2] | Riyaz A. Mir, Jeff Lovelace, Nicholas P. Schafer, Peter D. Simone, Admir Kellezi, Carol Kolar, Gaelle Spagnol, Paul L. Sorgen, Hamid Band, Vimla Band, Gloria E. O. Borgstahl . Biophysical characterization and modeling of human Ecdysoneless (ECD) protein supports a scaffolding function. AIMS Biophysics, 2016, 3(1): 195-210. doi: 10.3934/biophy.2016.1.195 |
[3] | Ulrich Lüttge . Physics and the molecular revolution in plant biology: union needed for managing the future. AIMS Biophysics, 2016, 3(4): 501-521. doi: 10.3934/biophy.2016.4.501 |
[4] | Ahmad Sohrabi Kashani, Muthukumaran Packirisamy . Cellular deformation characterization of human breast cancer cells under hydrodynamic forces. AIMS Biophysics, 2017, 4(3): 400-414. doi: 10.3934/biophy.2017.3.400 |
[5] | Oleksii V. Khorolskyi, Nikolay P. Malomuzh . Macromolecular sizes of serum albumins in its aqueous solutions. AIMS Biophysics, 2020, 7(4): 219-235. doi: 10.3934/biophy.2020017 |
[6] | Takayuki Yoshida, Hiroyuki Kojima . Subcutaneous sustained-release drug delivery system for antibodies and proteins. AIMS Biophysics, 2025, 12(1): 69-100. doi: 10.3934/biophy.2025006 |
[7] | Dewey Brooke, Navid Movahed, Brian Bothner . Universal buffers for use in biochemistry and biophysical experiments. AIMS Biophysics, 2015, 2(3): 336-342. doi: 10.3934/biophy.2015.3.336 |
[8] | James C.L. Chow . Biophysical insights into nanomaterial-induced DNA damage: mechanisms, challenges, and future directions. AIMS Biophysics, 2024, 11(3): 340-369. doi: 10.3934/biophy.2024019 |
[9] | Yu-Ting Huang, Hui-Fen Liao, Shun-Li Wang, Shan-Yang Lin . Glycation and secondary conformational changes of human serum albumin: study of the FTIR spectroscopic curve-fitting technique. AIMS Biophysics, 2016, 3(2): 247-260. doi: 10.3934/biophy.2016.2.247 |
[10] | Moataz M. Fahmy, Sohier M. El-Kholey, Seham Elabd, Mamdouh M. Shawki . Effect of changing the alternating electric current frequency on the viability of human liver cancer cell line (HEPG2). AIMS Biophysics, 2025, 12(1): 1-13. doi: 10.3934/biophy.2025001 |
Performing their functions as transporters of oxygen and carbon dioxide in the body, human erythrocytes constantly circulate and are exposed to the constant influence of various substances, including nutrients, drugs, medical devices covered with coatings, etc. Therefore, we aim to investigate the biophysical behavior of erythrocytes obtained from healthy volunteers to observe their morphological type changes, alterations in the zeta potential, the electrical conductivity of the erythrocytes in suspensions, and hemolysis in percentages during cells senescence, both in presence and in absence of natural polyelectrolytes pectin (PE) and chitosan (Chi) in form of multilayer films (PEM-films). Being constructed using the layer–by–layer technique, films are an object of interest of many researchers because of their high potential to be incorporated in biomedicine. By applying optical profilometry, electrophoretic light scattering, and spectrophotometry, we tested the polyelectrolytes for any potential harm on the erythrocytes. Based on our results and the one-way analysis of variance (ANOVA) statistical analysis, we reached the conclusion that the above-mentioned polyelectrolytes were harmlessness; therefore, PE and Chi are suitable substances to implement in the clinical practice in the form of drug delivery carriers and medical devices coatings, thereby directly contacting with the human blood.
Human erythrocytes are anucleate blood cells, devoid of ribosomes, mitochondria, endoplasmic reticulum, and the Golgi complex. The energy production is performed in the process of glycolysis, in which molecules of adenosine triphosphate (ATP) are formed. Moreover, in the same process, nicotinamide adenine dinucleotide (NAD) and 2, 3-diphosphoglycerate (2,3-DPG) are synthesized. The main function of these cells, specifically oxygen and carbon dioxide exchange in the organism [1], is implemented by the iron-containing protein hemoglobin (Hb), which isa heterotetramer with a concentration in the cell of about 5 x 103 M [2]. Its oxygen affinity and sensitivity to modulate cofactors are strictly regulated by molecular mechanisms, which include homotropic (heme-heme) and heterotropic interections (hydrogen and chloride ions, carbon dioxide, and the intraerythrocytic organophosphates interacting with protein fragments) [3]. Under normal physiological conditions, the erythrocytes have an average lifespan of approximately 120 days [4], and their shape is a biconcave disc with a diameter between 7 and 8 µm with an average volume of 90 fluid ounces [1]. The concentration of these cells in the human blood is about 5 million per µL [4].
The deformability is an essential property of these blood cells due to the passing of these cells through the smallest capillaries in the human body, thereby elongating in these vessels without the occurrence of ruptures [1],[4]. This process depends on the cytoskeleton–two-dimensional triangular meshwork and integral transmembrane complexes. Here, the integral Band 3 protein, a member of the anion exchanger gene family, has an important role due to its attachment with many other membrane proteins, such as Rh complex, glycophorins, and CD47. The membrane integrity and the morphology of erythrocytes depend on the interaction between the cytoskeleton component–spectrin filaments (α- and β-subunits in the form of antiparallel isomers) conjugated with filamentous actin in heterotetramers, respectively. The interconnection with the phospholipid bilayer is implemented by Band 3, either glycophorin or actin, and Band 4.1. It is known that the cytoskeleton is stabilized by myosin filaments [1],[4].
During erythrocyte senescence, the cells undergo various alterations that affect their morphology and functions [5]. The biconcave shape (or slightly crenated cells with normal functions) of the aging erythrocytes changes to echinocytes, which are red blood cells with many thorny projections, though they retain their functionality. This is followed by the change to spherocytes (or spherical red blood cells). The last morphological type is the final form of the cell's existence wherein the functionality is lost. Non-functioning erythrocytes are removed from the blood stream via phagocytosis by reticuloendothelial system macrophages in the liver and the spleen [4].
As the most common type of blood cells, they are an excellent, well-established model in biophysical studies because of their long lifespan, the easy isolation from the whole blood, and the simple storage capable of a prolonged incubation with a purpose to examine the upcoming aging processes within them. [6]–[8]. During circulation in the human body, they are constantly exposed to physical and chemical factors that can affect their condition [4]. Erythrocytes actively respond to changes in the environment, thereby altering their form for a short time. Moreover, the red blood cell (RBC) activity regulation can be influenced, which is understandable, taking the potential of erythrocytes to accumulate and/or to interact superficially with a variety of substances from the cell environment into account.
All of the features of erythrocytes can be affected in connection to different disorders [9], such as chronic hemolytic anemia, sickle cell anemia, thalassemia, neurological, cardiovascular and renal diseases, and malignant diseases [10]–[12]. The state of the cells can be estimated by examining the degree of hemolysis under the influence of stress factors, such as the induced cell volume alteration, which is provoked by erythrocytic resuspension in buffer solutions with different concentrations of their components and with different osmotic pressure. This is an indicator of the membrane stability and fluidity, which depends on the cholesterol in the outer layer. Therefore, the molecules of cholesterol in the cell membrane are considered as a potential regulator of stress-induced hemolysis, including the osmotic one [13].
It is well known that human erythrocytes are negatively charged and move to the anode in applied external electric fields [14]. The electrochemical properties of the erythrocytes are due to the electrical charges of the molecules, which are situated on the external cell membrane surface, as proteins are a major contributor to this [15]. One important property of erythrocytes is the zeta potential, which can be determined by an electrophoretic mobility assay. It is established that the zeta potential of human erythrocytes is approximately −15.7 mV, and this parameter is significantly lower in pathology (−14.6 mV) because of the protein [16] and glycoprotein expression, the intracellular ion concentrations, and the transmembrane ion flows. It is known that the zeta potential value is bigger in older erythrocytes than in younger erythrocytes and their sizes can be affected from the aging processes, which is provableby the light scattering [17],[18]. The zeta potential is the main prevention of cellular aggregation in the bloodstream [19].
According to existing reports, the electrical conductivity of erythrocytic suspensions can be affected as a result of external factors, and this parameter can be used to characterize the functional state of the cell membrane and the cell in general [17],[20]. Therefore, we suggested that the interaction between the released polyelectrolytes from polyelectrolyte multilayer (PEM)-films and the erythrocytes in suspensions could potentially induce the efflux of electrolytes across the erythrocyte membrane, in turn, this would affect the electrical conductivity values of the medium in which the erythrocytes are suspended (a buffer solution).
The membrane's zeta potential and the morphological and mechanical properties of human erythrocytes are the basis of the proper functioning of these cells, which are inextricably associated with their structure. These parameters may affect the affinity, aggregation, metabolism, and immunity of the RBCs [18].
Many authors are keenly interested in the possibilities of the targeted delivery of therapeutic drugs by using microcapsules as carriers of the medical substances to the relevant organs [21]–[24] and by placing polyelectrolyte multilayered stents in large blood vessels to avoid complications such as restenosis and/or thrombosis [25]–[27]. Keeping in mind that, in the last case, the polyelectrolytes directly contact with the blood, we chose human erythrocytes as our object of study because they are appropriate to investigate the interactions between them and to consider various substances, which can be various types of therapeutic drugs (e.g., pectin has antimicrobial properties, and in combination with chitosan, it is possible to produce microspheres and microcapsules containing medical drugs) [21]–[24]. For this reason, our research seeks to determine the biophysical behavior of human erythrocytes by observing alterations in their morphology, changes in the zeta potentials, changes in the conductivity of the RBC suspensions, and changes in hemolysis during the cellular aging process in the presence and in the absence of the natural polyelectrolytes pectin (PE) and chitosan (Chi), in form of multilayer films.
Chitosan (Chi) (MW 50–190 kDa, 75–85% deacetylated) and pectin (PE) from citrus peel ((Galacturonic acid ≥ 74.0% (dried basis)) were purchased from Sigma Aldrich, Germany. The 0.2% solutions were prepared in milli-Q water. The pH of the Chi and PE solutions was adjusted to 3 and 7, respectively.
PEM coatings (or films) from 10 bilayers were constructed on a hard substrate using the layer–by–layer technique, based on the electrostatic interactions [28]. The construction of the films was performed according to the protocol described in Arnon-Rips and Poverenov, 2018 [29]. In brief, the monolayers were deposited on glass slides with dimensions of 5 by 10 cm, which remained immersed in the corresponding polyelectrolyte solution for 15 minutes with intermediate triple washing in milli-Q water. It is important to note that the first monolayer deposited on the glass slides was pectin after the slides were preliminary activated for the deposition with 2 mg/ml polyethylenimine.
Blood from 15 healthy volunteers was collected in test tubes with K2EDTA as an anticoagulant. The whole blood was centrifuged at 4 °C, 900 g for 15 minutes using the Sigma 2–16 KL centrifuge. The supernatant (blood plasma) was replaced in Eppendorf tubes and the pellets (erythrocytes) were washed twice with a PBS–EDTA buffer solution, pH 7.4, at a ratio of 1: 3; the samples were centrifuged at 4 °C, 1200 g for 15 minutes, after which the erythrocyte suspensions were diluted in a pure PBS buffer solution to a hematocrit (Ht) = 35%. Each of the samples were divided into two parts: one was added to the PEM film, and the other part was used as a control. The samples were incubated in the absence and in the presence of PEM films at 4 °C for a 60-day period of time (monitoring period); moreover, they were characterized every 15 days (monitoring points, mp) with the methods described below.
In order to determine the levels of hemolysis in percentages at each mp, which was measured for all of the samples, we washed the erythrocytes in a PBS buffer solution, pH 7.4. After 15 minutes of centrifugation at 1200 g, the concentrations of the spontaneous released Hb in the supernatants were measured by spectrophotometry and calculated using the following formula:
where C is the concentration of the Hb, AEL is the absorption of the supernatants, and TL is the total lysis level of the cells, which is provoked by resuspension in a hypotonic medium.
A UV/VIS spectrophotometer (Specord 50+Analytic Jena, Germany) was used to determine the concentration of Hb (respectively Ht) in the samples and to determine the hemolysis levels. The measurements were carried out at a wavelength of 540 nm for the Hb concentration determination and at 405 nm for the hemolysis levels establishment.
The zeta potential and the electrical conductivity of the erythrocytes in the suspensions were performed using a Zetasizer Nano ZS analyzer in monomodal mode, at 25 °C, and Ht = 1%. The measurements were performed every 15 days over a period of 60 days.
The optical prophilometer 3D Zeta-20 (Zeta Instruments) was applied to define the morphological types of the untreated and treated erythrocytes with PEM films at each mp, which were preliminary prepared in the form of smears on microscope slides covered with poly–L–lysine.
All data are expressed as mean ± SD. Statistical significance was assessed by applying a one-way analysis of variance (ANOVA) test using the Origin 2018 software package. P < 0.05 is considered statistically significant.
The blood sampling was performed in the Мultiprofile Hospital for Active Treatment in Neurology and Psychiatry “St. Naum” (MHATNP), Sofia, Bulgaria, in accordance with the ethical standards of the Declaration of Helsinki and after obtaining informed consent from the volunteers.
To evaluate the influence of the natural polyelectrolytes PE and Chi on the biophysical properties of human erythrocytes, we conducted a series of experiments to monitor changes in the morphology, zeta potential, and conductivity of the RBCs in suspensions, alongside the levels of hemolysis during cellular senescence in the presence and in absence of the PE/Chi PEMs. We previously tested the degradation period of these films and established that its duration lasts 30 days (Figure 1, Panels A and B). Therefore, during the 60-day follow-up of changes that occured in the erythrocytes, the polyelectrolyte films were replaced on day 30 of the incubation period. With this step, constancy in the release of PE and Chi was ensured in the treated samples.
The erythrocytic morphology data were obtained every 15 days (5 mp) throughout the 60-day aging period, and the data for the untreated erythrocytes are presented in Table 1. Аt the beginning of the aging period (day 1), normocytes constituted the most abundant morphological type, with 96.55% of the total number of RBCs, followed by 3.40% echinocytes and 0.05% spherocytes. On day 15, the value of the normocytes was significantly reduced to 14.64% as compared to day 1. The decrease in the count of the normocytes was evident to the end of the aging period, where the biconcave disc-shaped erythrocytes constituted only 7.1%, 4.98%, and 1.55% of the total number of erythrocytes at days 30, 45, and 60, respectively. At the same time, we observed an increasing tendency in the proportion of both echinocytes and spherocytes, with spherocytes being the dominant morphological type from day 15 (55.85%) to the end of the aging period. The percentage distribution for the spherocytes is as follows: 73.80% (day 30), 85.11% (day 45), and 85.65% (day 60). Comparatively, the percentage distribution for the echinocytes is as follows: 9.91% (day 15), 12.8% (day 30), 19.1% (day 45), and 29.51% (day 60).
Polyelectrolyte-treated erythrocytes demonstrated the same tendency to reduce the percentage of normocytes in the samples and, conversely, increase the number of the echinocytes and spherocytes (Table 1). The decreasing number of the normocytes at the successive mp was as follows: 89% (day 1), 9.65% (day 15), 8.24% (day 30), 2.5% (day 45), and 1.23% (day 60). The total number of echinocytes increased at each subsequent measurement during aging (6.10%, 10.30%, 15.13%, 15.22%, and 30.35%, respectively), as shown in Table 1. The echinocytes increased in the same sequence of the mp, as their percentage content in the samples changed (6.10%, 10.30%, 15.13%, 15.22%, and 30.35%). The spherocytes increased in a similar fashion to the echinocytes; following the order of the mp, we can observe the alterations in their percentages: 4.9% (day 1), 60% (day 15), 76.63% (day 30), 83.55% (day 45), and 87.2% (day 60).
Morphological type | Normal | Echinocytes | Spherocytes | ||||||
Day | Untreated | Treated | p-value | Untreated | Treated | p-value | Untreated | Treated | p-value |
Day 1 | 96.55 ± 3.42 | 89.00 ± 8.90 | 0 | 3.40 ± 2.44 | 6.10 ± 7.90 | 0 | 0.05 ± 0.03 | 4.90 ± 0.00 | 0 |
Day 15 | 14.64 ± 5.46 | 9.65 ± 10.30 | 0 | 9.91 ± 6.47 | 10.30 ± 4.80 | 0 | 55.85 ± 4.33 | 60.00 ± 13.80 | 0 |
Day 30 | 7.10 ± 2.77 | 8.24 ± 4.40 | 0 | 12.80 ± 7.32 | 15.13 ± 10.40 | 0 | 73.80 ± 12.73 | 76.63 ± 7.60 | 0 |
Day 45 | 4.98 ± 2.16 | 2.50 ± 2.30 | 0 | 19.10 ± 8.31 | 15.22 ± 6.60 | 0 | 85.11 ± 11.52 | 83.55 ± 7.60 | 0 |
Day 60 | 1.55 ± 1.39 | 1.23 ± 1.90 | 0 | 29.51 ± 4.89 | 30.35 ± 15.90 | 0 | 85.65 ± 11.97 | 87.20 ± 5.30 | 0 |
A one-way ANOVA test was performed, and the results demonstrate that there is not a significant difference between the polyelectrolyte-treated erythrocytes and the untreated erythrocytes at the individual mp, keeping in mind that a p-value of < 0.05 was considered as statistically significant (Figure 2 and Table 1). The same tendency is observed by both the polyelectrolyte–treated erythrocytes and the untreated erythrocytes, as well as the same life expectancy of the two groups of RBCs and the lack of significant differences, which are proof of the harmlessness of the PE and Chi used.
The large standard deviation at some mp is impressive. Our assumption about it is based on the age and/or habits (e.g., eating habits, smoking, alcohol intake, etc.) differences of the volunteers participating in the study, their specific reactions to the substances, as well as the presence of erythrocytes at different degrees of senescence present in the suspensions.
The electrophoretic behavior of the RBCs in suspension was investigated in the absence and in the presence of the natural polyelectrolytes PE and Chi, in the form of multilayer films. We examined the interaction between these polyelectrolytes and the human erythrocytes membranes with a purpose to establish whether they are either harmful or harmless for these blood cells. The data are summarized in Table 2. We established a trend towards an increased zeta potential during the monitoring period, which is typical for aging cells.
Parameter | ||||||
Zeta potential, mV | Conductivity, mS/cm | |||||
Group | Group | |||||
Day | Untreated erythrocytes | Treated erythrocytes | p-value | Untreated erythrocytes | Treated erythrocytes | p-value |
Day 1 | −16.93 ± 0.57 | −17.17 ± 0.46 | 0 | 16.18 ± 0.51 | 16.51 ± 0.53 | 0 |
Day 15 | −16.64 ± 0.22 | −16.15 ± 0.60 | 0 | 16.80 ± 0.37 | 16.94 ± 0.58 | 0 |
Day 30 | −15.67 ± 0.54 | −15.76 ± 0.26 | 0 | 16.70 ± 0.40 | 16.82 ± 0.24 | 0 |
Day 45 | −15.49 ± 0.65 | −15.44 ± 0.26 | 0 | 16.72 ± 0.41 | 16.79 ± 0.24 | 0 |
Day 60 | −14.73 ± 1.15 | −14.83 ± 0.26 | 0 | 16.56 ± 0.43 | 16.86 ± 0.23 | 0 |
*Statistically significant at p < 0.05
It is important that the established numbers for the zeta potential detected for the untreated cells are very close to these for the treated cells with PEM erythrocytes, and no static differences were established by the one-way Anova test (Figure 3, Panel A, and Table 2) between both groups. This provides supporting evidence about the harmless nature of PE and Chi, in the presence of which the samples were incubated. The values of the untreated erythrocytes increased in the interval from −16.93 mV to −14.73 mV, and the values for the treated cells increased between −17.17 mV and −14.83 mV.
The standard deviation (SD) varied between 0.22 and 1.15 for the untreated erythrocytes. A relatively low SD was established for the period of time between day 1 and day 45, which is associated with the availability of erythrocytes at different ages in the samples. The biggest number for the SD was found for day 60, which is probably due to the presence of both functional and non-functional cells (Table 2).
Polyelectrolytes probably stabilize the erythrocytes membrane through electrostatic interactions; therefore, the SD of the zeta potential was lower after day 30 as compared to day 1 (0.46) and day 15 (0.26) (Table 2).
The measured electrical conductivity data of untreated and treated samples with PE/Chi–PEMs erythrocytes in suspension during the aging period are shown in Figure 3, Panel B, and in Table 2. Here, we consider the conductivity of the medium (the solution) in which the erythrocytes were suspended, because it can be influenced by the release of ions from the cells under the influence of different factors. Therefore, we tested the electrical condition of this medium in the presence and in the absence of polyelectrolytes in the samples in order to understand whether the PE/Chi–PEMs were detrimental or not. To avoid any unwanted side effects, we used the same buffer solution, namely PBS, pH 7.4.
The values established for the conductivity of the aforementioned medium demonstrate that there were no statistical differences between the separate mp (Figure 3, Panel B and Table 2) for both untreated and treated samples with PE/Chi–PEMs. The range of the numbers (16.18 to 16.94) is typical for PBS-buffer solutions at room temperature (because of the ions content), and the statistical similarity between thеse values is evidence for the biocompatibility of the films with human RBCs. The large SD is likely due to the age difference of the cells in the samples.
For the purposes of the study, the levels of hemolysis during the 60-day aging period of the erythrocytes were also determined (Figure 4 and Table 3). No statistically significant differences were observed (through the use of the one-way ANOVA statistical test) between non-contacted and PEM-contacted RBCs. In both groups of erythrocytes, the day 1 and day 15 hemolysis values were the lowest and were statistically indistinguishable (with very low SD values).
Data from day 30 to the end of the 60-day aging period showed a significant and statistically distinguishable increase in the levels of hemolysis, possibly because of the natural aging processes in cells. Furthermore, the SD was larger compared to the day 1 and day 15 data. Given the different ages of the cells in the samples from the time of blood-collection, this is likely due to the fact that cells age at different rates in the samples. Additionally, the comparable values of hemolysis for the two groups of cells at each mp are proof of the biocompatibility of PEM with this chemical composition, and there are no statistically significant differences between the levels of the hemolysis in both groups at the relevant mp (Figure 4 and Table 3).
Hemolysis (%) |
|||
Group |
|||
Day | Untreated erythrocytes | Treated erythrocytes | p-value |
Day 1 (mp 1) | 3.85 ± 0.58 | 3.02 ± 0.58 | 0 |
Day 15 (mp 2) | 6.11 ± 3.82 | 6.44 ± 1.52 | 0 |
Day 30 (mp 3) | 29.48 ± 8.22 | 27.32 ± 8.88 | 0 |
Day 45 (mp 4) | 48.78 ± 8.01 | 42.26 ± 7.27 | 0 |
Day 60 (mp 5) | 52.79 ± 9.75 | 54.03 ± 7.25 | 0 |
When treated with PE and Chi (in form of multilayer films) and incubated for a period of 60 days in the presence of these films, erythrocytes are constantly exposed to the gradually released substances. The obtained results showed that there were no statistically significant differences between the morphological type, zeta potential, electrical conductivity of the erythrocytes suspension, and hemolysis, which were measured for the treated and untreated samples; moreover, this is evidence about the harmless nature of PE and Chi. Since we studied erythrocytic aging (untreated and treated), it would be beneficial to investigate more indicators for cellular senescence (except those described in this article), for example, one could investigate the levels of oxidative stress at each mp [30] and the reaction to radiation, keeping in mind that this model enables insights into the mechanisms of the antioxidant action of small molecules and nanoparticles. [31]. However, the limiting factor here is the amount of blood samples obtained from the volunteers. Тhis is the reason why we will pursue further investigations, which will complement this study and will clarify the molecular mechanisms of untreated and treated aging erythrocytes.
In their reports, some authors describe that young erythrocytes are more negatively charged than the older ones [32],[33]. Considering the zeta potential, we can see that the numbers, which were measured at the respective mp for the untreated and treated samples with [PE/Chi]10 film erythrocytes, were almost equal, and they subsequently increased during the monitoring period is for both groups of cells. Therefore, we assumed that the PE and Chi did not provoke any negative reaction in the RBCs. Additionally, we suppose that it is possible that the polyelectrolytes can stabilize the erythrocyte's membrane through electrostatic interactions; therefore, the SD of the zeta potential was lower after day 30 compared to day 1 (0.46) and day 15 (0.26). Interesting, the values for the electrical conductivity of the medium in which the RBCs were suspended was just a little lower at day 1; after day 15, they were slightly elevated and these numbers were not significantly changed to day 60 in the both groups of cells. Therefore, our opinion is that PE and Chi do not contribute to the electrical conductivity in the human erythrocytes suspensions, and these substances are not capable of altering the cellular functionality.
Мany authors present evidence that described the harmful effects of Chi on the blood, based on the fact that this polyelectrolyte is a polycation, and have expressed statements that this provokes a lysis of the erythrocytes [34]–[37]; however, some of them have tried to develop strategies to improve the biocompatibility of this polycation, thereby associating it with compounds that exhibit complementary properties, structural modifications etc. [38]–[40]. Considering our results, we can see that there were no significant differences for spontaneous Hb release in the untreated and treated samples with the PEM erythrocytes, probably due to the low concentration of the relatively slow PEM degradation, thereby avoiding the negative effects of the polycation.
The SD determined for the measured parameters at each of the mp was due to the differences in the cells of different ages in the incubated samples because of the constant production of erythrocytes in the bone morrow, which was found later in the volunteers' samples.
Our results demonstrated that these natural polyelectrolytes were harmless and could be used in the clinical practice, for example, about covers for different medical devices, directly contacting with the blood, or as carriers for target delivery of medical substances. It is an important fundamental scientific discovery because many patients with cardiovascular disorders require the placement of coronary stents, some of which are uncovered. This kind of stents may lead to restenosis and/or thrombosis, which worsen the health condition of patients [41]. The covered stents significantly reduce the risk of cardiovascular incidents due to the placement of uncovered stents. [42]. The need to administer many medications in patients with different disorders can provoke the engineering of polyelectrolyte-based systems (e.g., microcapsules) for targeting and/or the controlled delivery of medical substances in different organs in order to avoid their incomplete assimilation. Taking our results into account, we assert that Pe and Chi are suitable substances for drug delivery systems engineering.
The alterations of the erythrocyte morphological type, changes in the zeta potential, electrical conductivity, and hemolysis were similar in both groups of untreated and treated samples with PEM films during cellular aging. These data demonstrated the harmless nature of PE/Chi multilayer films for human erythrocytes, hemocompatibility, and implementation benefits in medical practices.
The authors declare they have not used artificial intelligence (AI) tools in the creation of this article.
[1] |
Kuck L, Peart JN, Simmonds MJ (2020) Active modulation of human erythrocyte mechanics. Am J Physiol Cell Physiol 319: C250-C257. https://doi.org/10.1152/ajpcell.00210.2020 ![]() |
[2] |
Sakamoto W, Azegami N, Konuma T, et al. (2021) Single-cell native mass spectrometry of human erythrocytes. J Anal Chem 93: 6583-6588. https://doi.org/10.1021/acs.analchem.1c00588 ![]() |
[3] |
Giardina B, Messana I, Scatena R, et al. (1995) The multiple functions of hemoglobin. J Crit Rev Biochem Mol Biol 30: 165-196. https://doi.org/10.3109/10409239509085142 ![]() |
[4] |
Thiagarajan P, Parker CJ, Prchal JT (2021) How do red blood cells die?. J Front Physiol 12: 655393. https://doi.org/10.3389/fphys.2021.655393 ![]() |
[5] |
Dinarelli S, Longo G, Dietler G, et al. (2018) Erythrocyte's aging in microgravity highlights how environmental stimuli shape metabolism and morphology. Sci Rep-UK 8: 5277. https://doi.org/10.1038/s41598-018-22870-0 ![]() |
[6] |
Goodman SR, Hughes KMH, Kakhniashvili DG, et al. (2007) The isolation of reticulocyte-free human red blood cells. J Exp Biol Med (Maywood) 232: 1470. https://doi.org/10.3181/0706-RM-163 ![]() |
[7] |
Pretini V, Koenen MH, Kaestner L, et al. (2019) Red blood cells: chasing interactions. Front Physiol 10: 945. https://doi.org/10.3389/fphys.2019.00945 ![]() |
[8] |
Grebowski J, Kazmierska-Grebowska P, Cichon N, et al. (2022) The effect of fullerenol C60(OH)36 on the antioxidant defense system in erythrocytes. Int J Mol Sci 23: 119. https://doi.org/10.3390/ijms23010119 ![]() |
[9] |
Lawrence C, Meier E (2021) Chapter 17-erythrocyte disorders. Biochemical and Molecular Basis of Pediatric Disease : 529-560. https://doi.org/10.1016/B978-0-12-817962-8.00023-8 ![]() |
[10] |
Maheshwari N, Khan FH, Mahmood R (2019) Pentachlorophenol-induced cytotoxicity in human erythrocytes: enhanced generation of ROS and RNS, lowered antioxidant power, inhibition of glucose metabolism, and morphological changes. Environ Sci Pollut Res 13: 12985-13001. https://doi.org/10.1007/s11356-019-04736-8 ![]() |
[11] |
Piscopo M, Notariale R, Tortora F, et al. (2020) Novel insights into mercury efects on hemoglobin and membrane proteins in human erythrocytes. Molecules 25: 3278. https://doi.org/10.3390/molecules25143278 ![]() |
[12] | Berzuini A, Bianco C, Migliorini AC, et al. (2021) Red blood cell morphology in patients with COVID-19-related anaemia. J Blood Transfus 19: 34-36. https://doi.org/10.2450/2020.0242-20 |
[13] | Yamaguchi T, Ishimatu T (2020) Effects of cholesterol on membrane stability of human erythrocytes. J Biol Pharm Bull 43: 604-1608. https://doi.org/10.1248/bpb.b20-00435 |
[14] |
Jan KM, Chien SH (1973) Role of surface electric charge in red blood cell interactions. J Gen Physiol 61: 638-654. https://doi.org/10.1085/jgp.61.5.638 ![]() |
[15] |
Vincy A, Mazumder S, Amrita Banerjee I, et al. (2022) Recent progress in red blood cells-derived particles as novel bioinspired drug delivery systems: challenges and strategies for clinical translation. Front Chem 10: 905256. https://doi.org/10.3389/fchem.2022.905256 ![]() |
[16] |
Tokumasu F, Ostera GR, Amaratunga Ch, et al. (2012) Modifications in erythrocyte membrane zeta potential by plasmodium falciparum Infection. Exp Parasitol 131: 245-251. https://doi.org/10.1016/j.exppara.2012.03.005 ![]() |
[17] | Selim NS (2010) Comparative study on the effect of radiation on whole blood and isolated red blood cells. Romanian J Biophys 20: 127-136. |
[18] |
Chen XY, Huang YX, Liu W, et al. (2007) Membrane surface charge and morphological and mechanical properties of young and old erythrocytes. J Curr Appl Phys 7: 94-96. https://doi.org/10.1016/j.cap.2006.11.024 ![]() |
[19] |
Kuchinka J, Willems Ch, Telyshev DV, Groth T (2021) Control of blood coagulation by hemocompatible material surfaces—a review. Bioengineering 8: 215. https://doi.org/10.3390/bioengineering8120215 ![]() |
[20] |
Doltchinkova V, Stoylov S, Angelova P (2021) Viper toxins affect membrane characteristics of human erythrocytes. Biophys Chem 270: 106532. https://doi.org/10.1016/j.bpc.2020.106532 ![]() |
[21] |
Qian J, Chen Y, Wang Q, et al. (2021) Preparation and antimicrobial activity of pectin-chitosan embedding nisin microcapsules. Eur Polym J 157: 110676. https://doi.org/10.1016/j.eurpolymj.2021.110676 ![]() |
[22] |
Xie Q, Zheng X, Li L, et al. (2021) Effect of curcumin addition on the properties of biodegradable pectin/chitosan films. Molecules 26: 2152. https://doi.org/10.3390/molecules26082152 ![]() |
[23] |
Jovanović J, Ćirković J, Radojković A, et al. (2021) Chitosan and pectin-based films and coatings with active components for application in antimicrobial food packaging. Prog Org Coat 158: 106349. https://doi.org/10.1016/j.porgcoat.2021.106349 ![]() |
[24] |
Jiang Z, Zhao S, Yang M, et al. (2022) Structurally stable sustained-release microcapsules stabilized by self-assembly of pectin-chitosan-collagen in aqueous two-phase system. J Food Hydrocolloids 125: 107413. https://doi.org/10.1016/j.foodhyd.2021.107413 ![]() |
[25] |
Mallory A, Giannopoulos S, Lee P, et al. (2021) Covered stents for endovascular treatment of aortoiliac occlusive disease: a systematic review and meta-analysis. Vasc Endovasc Surg 55: 560-570. https://doi.org/10.1177/15385744211010381 ![]() |
[26] | Kawagoe Y, Otuka F, Onozuka D, et al. (2022) Early vascular responses to abluminal biodegradable polymer-coated versus circumferential durable polymer-coated newer-generation drug-eluting stents in humans: a pathologic study. EuroIntervention 18: 1284-1294. https://doi.org/10.4244/EIJ-D-22-00650 |
[27] |
McKenna CG, Vaughan TJ (2022) A computational framework examining the mechanical behaviour of bare and polymer-covered self-expanding laser-cut stents. J Cardiovasc Eng Tech 13: 466-480. https://doi.org/10.1007/s13239-021-00597-w ![]() |
[28] |
Hu B, Guo Y, Li H, et al. (2021) Recent advances in chitosan-based layer-by-layer biomaterials and their biomedical applications. Carbohyd Polym 271: 118427. https://doi.org/10.1016/j.carbpol.2021.118427 ![]() |
[29] |
Arnon-Ripsb H, Poverenova E (2018) Improving food products' quality and storability by using layer by layer edible coatings. Trends Food Sci Tech 75: 81-92. https://doi.org/10.1016/j.tifs.2018.3.003 ![]() |
[30] |
Grebowski J, Konarska A, Piotrowski P, et al. (2024) Kinetics of metallofullerenol reactions with the products of water radiolysis: implications for radiotherapeutics. ACS Appl Nano Mater 7: 539-549. https://doi.org/10.1021/acsanm.3c04747 ![]() |
[31] |
Silva DCN, Jovino CN, Silva CAL, et al. (2012) Optical tweezers as a new biomedical tool to measure zeta potential of stored red blood cells. Plos One 7: e31778. https://doi.org/10.1371/journal.pone.0031778 ![]() |
[32] |
Raat NJ, Verhoeven AJ, Mik EG (2005) The effect of storage time of human red cells on intestinal microcirculatory oxygenation in a rat isovolemic exchange model. Crit Care Med 33: 39-45. https://doi.org/10.1097/01.ccm.0000150655.75519.02 ![]() |
[33] |
Chen KY, Lin TH, Yang CY, et al. (2018) Mechanics for the adhesion and aggregation of red blood cells on chitosan. J Mechanics 34: 725-732. https://doi.org/10.1017/jmech.2018.27 ![]() |
[34] |
Kou S, Peters L, Mucalo M (2022) Chitosan: a review of molecular structure, bioactivities and interactions with the human body and microorganisms. J Carbohyd Polym 282: 119132. https://doi.org/10.1016/j.carbpol.2022.119132 ![]() |
[35] |
Nadesh R, Narayanan D, Sreerekha PR, et al. (2013) Hematotoxicological analysis of surface-modified and unmodified chitosan nanoparticles. J Biomed Mater Res Part A 101A: 2957-2966. https://doi.org/10.1002/jbm.a.34591 ![]() |
[36] |
Balan V, Verestiuc L (2014) Strategies to improve chitosan hemocompatibility: a review. Eur Polym J 53: 171-188. https://doi.org/10.1016/j.eurpolymj.2014.01.033 ![]() |
[37] |
Zhou X, Zhang X, Zhou J, et al. (2017) An investigation of chitosan and its derivatives on red blood cell agglutination. RSC Adv 7: 12247-12254. https://doi.org/10.1039/C6RA27417 ![]() |
[38] |
Wang W, Xue Ch, Mao X (2020) Chitosan: structural modification, biological activity and application. Int J Biol Macromol 164: 4532-4546. https://doi.org/10.1016/j.ijbiomac.2020.09.042 ![]() |
[39] |
Azmana M, Mahmood S, Hilles AR, et al. (2021) A review on chitosan and chitosan-based bionanocomposites: Promising material for combatting global issues and its applications. Int J Biol Macromol 185: 832-848. https://doi.org/10.1016/j.ijbiomac.2021.07.023 ![]() |
[40] |
Negm NA, Hefni HHH, Abd-Elaal AAA, et al. (2020) Advancement on modification of chitosan biopolymer and its potential applications. Int J Biol Macromol 152: 681-702. https://doi.org/10.1016/j.ijbiomac.2020.02.196 ![]() |
[41] |
Erdogan E, Bajaj R, Lansky A, et al. (2022) Intravascular imaging for guiding in-stent restenosis and stent thrombosis therapy. J Am Heart Assoc 11: e026492. https://doi.org/10.1161/JAHA.122.026492 ![]() |
[42] |
Nagaraja V, Schwarz K, Moss S, et al. (2020) Outcomes of patients who undergo percutaneous coronary intervention with covered stents for coronary perforation: a systematic review and pooled analysis of data. Catheter Cardio Inte 96: 1360-1366. https://doi.org/10.1002/ccd.28646 ![]() |
Morphological type | Normal | Echinocytes | Spherocytes | ||||||
Day | Untreated | Treated | p-value | Untreated | Treated | p-value | Untreated | Treated | p-value |
Day 1 | 96.55 ± 3.42 | 89.00 ± 8.90 | 0 | 3.40 ± 2.44 | 6.10 ± 7.90 | 0 | 0.05 ± 0.03 | 4.90 ± 0.00 | 0 |
Day 15 | 14.64 ± 5.46 | 9.65 ± 10.30 | 0 | 9.91 ± 6.47 | 10.30 ± 4.80 | 0 | 55.85 ± 4.33 | 60.00 ± 13.80 | 0 |
Day 30 | 7.10 ± 2.77 | 8.24 ± 4.40 | 0 | 12.80 ± 7.32 | 15.13 ± 10.40 | 0 | 73.80 ± 12.73 | 76.63 ± 7.60 | 0 |
Day 45 | 4.98 ± 2.16 | 2.50 ± 2.30 | 0 | 19.10 ± 8.31 | 15.22 ± 6.60 | 0 | 85.11 ± 11.52 | 83.55 ± 7.60 | 0 |
Day 60 | 1.55 ± 1.39 | 1.23 ± 1.90 | 0 | 29.51 ± 4.89 | 30.35 ± 15.90 | 0 | 85.65 ± 11.97 | 87.20 ± 5.30 | 0 |
Parameter | ||||||
Zeta potential, mV | Conductivity, mS/cm | |||||
Group | Group | |||||
Day | Untreated erythrocytes | Treated erythrocytes | p-value | Untreated erythrocytes | Treated erythrocytes | p-value |
Day 1 | −16.93 ± 0.57 | −17.17 ± 0.46 | 0 | 16.18 ± 0.51 | 16.51 ± 0.53 | 0 |
Day 15 | −16.64 ± 0.22 | −16.15 ± 0.60 | 0 | 16.80 ± 0.37 | 16.94 ± 0.58 | 0 |
Day 30 | −15.67 ± 0.54 | −15.76 ± 0.26 | 0 | 16.70 ± 0.40 | 16.82 ± 0.24 | 0 |
Day 45 | −15.49 ± 0.65 | −15.44 ± 0.26 | 0 | 16.72 ± 0.41 | 16.79 ± 0.24 | 0 |
Day 60 | −14.73 ± 1.15 | −14.83 ± 0.26 | 0 | 16.56 ± 0.43 | 16.86 ± 0.23 | 0 |
*Statistically significant at p < 0.05
Hemolysis (%) |
|||
Group |
|||
Day | Untreated erythrocytes | Treated erythrocytes | p-value |
Day 1 (mp 1) | 3.85 ± 0.58 | 3.02 ± 0.58 | 0 |
Day 15 (mp 2) | 6.11 ± 3.82 | 6.44 ± 1.52 | 0 |
Day 30 (mp 3) | 29.48 ± 8.22 | 27.32 ± 8.88 | 0 |
Day 45 (mp 4) | 48.78 ± 8.01 | 42.26 ± 7.27 | 0 |
Day 60 (mp 5) | 52.79 ± 9.75 | 54.03 ± 7.25 | 0 |
Morphological type | Normal | Echinocytes | Spherocytes | ||||||
Day | Untreated | Treated | p-value | Untreated | Treated | p-value | Untreated | Treated | p-value |
Day 1 | 96.55 ± 3.42 | 89.00 ± 8.90 | 0 | 3.40 ± 2.44 | 6.10 ± 7.90 | 0 | 0.05 ± 0.03 | 4.90 ± 0.00 | 0 |
Day 15 | 14.64 ± 5.46 | 9.65 ± 10.30 | 0 | 9.91 ± 6.47 | 10.30 ± 4.80 | 0 | 55.85 ± 4.33 | 60.00 ± 13.80 | 0 |
Day 30 | 7.10 ± 2.77 | 8.24 ± 4.40 | 0 | 12.80 ± 7.32 | 15.13 ± 10.40 | 0 | 73.80 ± 12.73 | 76.63 ± 7.60 | 0 |
Day 45 | 4.98 ± 2.16 | 2.50 ± 2.30 | 0 | 19.10 ± 8.31 | 15.22 ± 6.60 | 0 | 85.11 ± 11.52 | 83.55 ± 7.60 | 0 |
Day 60 | 1.55 ± 1.39 | 1.23 ± 1.90 | 0 | 29.51 ± 4.89 | 30.35 ± 15.90 | 0 | 85.65 ± 11.97 | 87.20 ± 5.30 | 0 |
Parameter | ||||||
Zeta potential, mV | Conductivity, mS/cm | |||||
Group | Group | |||||
Day | Untreated erythrocytes | Treated erythrocytes | p-value | Untreated erythrocytes | Treated erythrocytes | p-value |
Day 1 | −16.93 ± 0.57 | −17.17 ± 0.46 | 0 | 16.18 ± 0.51 | 16.51 ± 0.53 | 0 |
Day 15 | −16.64 ± 0.22 | −16.15 ± 0.60 | 0 | 16.80 ± 0.37 | 16.94 ± 0.58 | 0 |
Day 30 | −15.67 ± 0.54 | −15.76 ± 0.26 | 0 | 16.70 ± 0.40 | 16.82 ± 0.24 | 0 |
Day 45 | −15.49 ± 0.65 | −15.44 ± 0.26 | 0 | 16.72 ± 0.41 | 16.79 ± 0.24 | 0 |
Day 60 | −14.73 ± 1.15 | −14.83 ± 0.26 | 0 | 16.56 ± 0.43 | 16.86 ± 0.23 | 0 |
Hemolysis (%) |
|||
Group |
|||
Day | Untreated erythrocytes | Treated erythrocytes | p-value |
Day 1 (mp 1) | 3.85 ± 0.58 | 3.02 ± 0.58 | 0 |
Day 15 (mp 2) | 6.11 ± 3.82 | 6.44 ± 1.52 | 0 |
Day 30 (mp 3) | 29.48 ± 8.22 | 27.32 ± 8.88 | 0 |
Day 45 (mp 4) | 48.78 ± 8.01 | 42.26 ± 7.27 | 0 |
Day 60 (mp 5) | 52.79 ± 9.75 | 54.03 ± 7.25 | 0 |