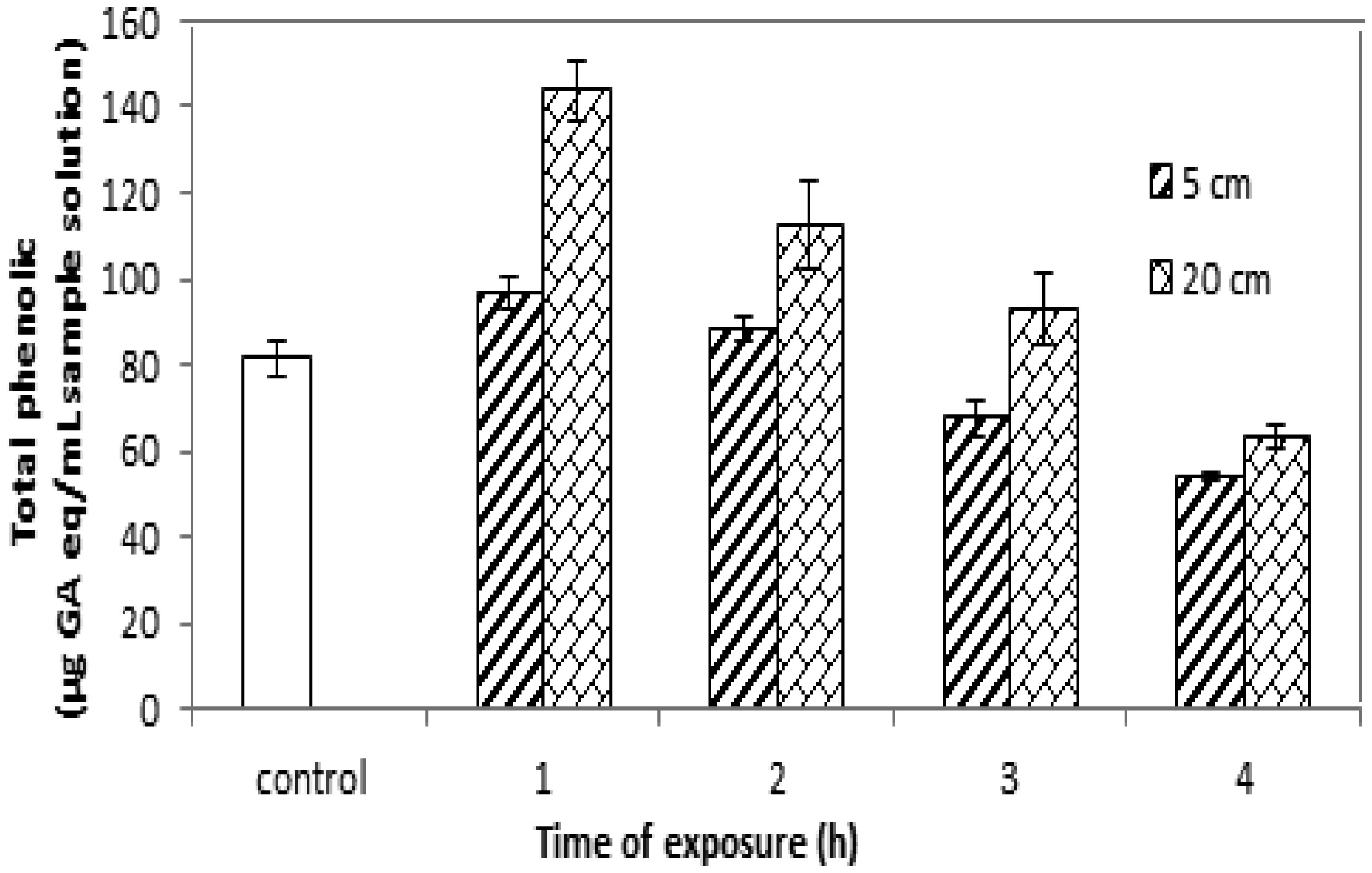
Some seeds are a major source of vitamins and minerals and contain primary and natural antioxidants, making them useful as medical resources to treat infectious diseases in poor and developing countries. This research aimed to study and analyze the effects of ultraviolet A radiation on the molecular structure, chemical composition and photochemical compounds of Salvia hispanica L. seeds (chia seeds). The results show that ultraviolet A radiation significantly affected the arrangement, size, interconnection and orientation of the Salvia hispanica seed molecules. Also, significant changes in the carbohydrate, protein and fat contents were observed, with little variation in the total fibers comprising the Salvia hispanica L. (chia seeds), after exposure to ultraviolet A radiation for different times at dissimilar distances. The phenolic content and flavonoid content in the Salvia hispanica L. seeds varied after exposure to ultraviolet A radiation for 1, 2, 3 and 4 hours at 5-cm and 20-cm distances from the ultraviolet source.
Citation: Reham Ebrahim, Aya Abdelrazek, Hamed El-Shora, Abu Bakr El-Bediwi. Effect of ultraviolet radiation on molecular structure and photochemical compounds of Salvia hispanica medical seeds[J]. AIMS Biophysics, 2022, 9(2): 172-181. doi: 10.3934/biophy.2022015
[1] | Soad Hasanin, A. G. ELshahawy, Hamed M El-Shora, Abu Bakr El-Bediwi . Gamma radiation effects on vitamins, antioxidant, internal and molecular structure of Purslane seeds. AIMS Biophysics, 2022, 9(3): 246-256. doi: 10.3934/biophy.2022021 |
[2] | Auwal Muhammad, Kanikar Muangchoo, Ibrahim A. Muhammad, Ya'u S. Ajingi, Aliyu M. Bello, Ibrahim Y. Muhammad, Tasi'u A. Mika'il, Rakiya Aliyu . A molecular modeling study of novel aldose reductase (AR) inhibitors. AIMS Biophysics, 2020, 7(4): 380-392. doi: 10.3934/biophy.2020026 |
[3] | Arjun Acharya, Madan Khanal, Rajesh Maharjan, Kalpana Gyawali, Bhoj Raj Luitel, Rameshwar Adhikari, Deependra Das Mulmi, Tika Ram Lamichhane, Hari Prasad Lamichhane . Quantum chemical calculations on calcium oxalate and dolichin A and their binding efficacy to lactoferrin: An in silico study using DFT, molecular docking, and molecular dynamics simulations. AIMS Biophysics, 2024, 11(2): 142-165. doi: 10.3934/biophy.2024010 |
[4] | Abdulrahaman Mahmoud Dogara, Ateeq Ahmed Al-Zahrani, Sarwan W. Bradosty, Saber W. Hamad, Shorsh Hussein Bapir, Talar K. Anwar . Antioxidant, α-glucosidase, antimicrobial activities chemical composition and in silico analysis of eucalyptus camaldulensis dehnh. AIMS Biophysics, 2024, 11(3): 255-280. doi: 10.3934/biophy.2024015 |
[5] | Morteza Vaezi, G. Rezaei Behbehani, Nematollah Gheibi, Alireza Farasat . Thermodynamic, kinetic and docking studies of some unsaturated fatty acids-quercetin derivatives as inhibitors of mushroom tyrosinase. AIMS Biophysics, 2020, 7(4): 393-410. doi: 10.3934/biophy.2020027 |
[6] | Murtala Muhammad, I. Y. Habib, Abdulmumin Yunusa, Tasiu A. Mikail, A. J. ALhassan, Ahed J. Alkhatib, Hamza Sule, Sagir Y. Ismail, Dong Liu . Identification of potential SARS-CoV-2 papain-like protease inhibitors with the ability to interact with the catalytic triad. AIMS Biophysics, 2023, 10(1): 50-66. doi: 10.3934/biophy.2023005 |
[7] | Warin Rangubpit, Pornthep Sompornpisut, R.B. Pandey . Thermal-induced unfolding-refolding of a nucleocapsid COVN protein. AIMS Biophysics, 2021, 8(1): 103-110. doi: 10.3934/biophy.2021007 |
[8] | Yun Chen, Bin Liu, Lei Guo, Zhong Xiong, Gang Wei . Enzyme-instructed self-assembly of peptides: Process, dynamics, nanostructures, and biomedical applications. AIMS Biophysics, 2020, 7(4): 411-428. doi: 10.3934/biophy.2020028 |
[9] | Jaouhra Cherif, Anis Raddaoui, Ghofrane Ben Fraj, Asma Laabidi, Nada Souissi . Escherichia coli's response to low-dose ionizing radiation stress. AIMS Biophysics, 2024, 11(2): 130-141. doi: 10.3934/biophy.2024009 |
[10] | Mikayel Ginovyan, Svetlana Hovhannisyan, Hayarpi Javrushyan, Gohar Sevoyan, Zaruhi Karabekian, Narine Zakaryan, Naira Sahakyan, Nikolay Avtandilyan . Screening revealed the strong cytotoxic activity of Alchemilla smirnovii and Hypericum alpestre ethanol extracts on different cancer cell lines. AIMS Biophysics, 2023, 10(1): 12-22. doi: 10.3934/biophy.2023002 |
Some seeds are a major source of vitamins and minerals and contain primary and natural antioxidants, making them useful as medical resources to treat infectious diseases in poor and developing countries. This research aimed to study and analyze the effects of ultraviolet A radiation on the molecular structure, chemical composition and photochemical compounds of Salvia hispanica L. seeds (chia seeds). The results show that ultraviolet A radiation significantly affected the arrangement, size, interconnection and orientation of the Salvia hispanica seed molecules. Also, significant changes in the carbohydrate, protein and fat contents were observed, with little variation in the total fibers comprising the Salvia hispanica L. (chia seeds), after exposure to ultraviolet A radiation for different times at dissimilar distances. The phenolic content and flavonoid content in the Salvia hispanica L. seeds varied after exposure to ultraviolet A radiation for 1, 2, 3 and 4 hours at 5-cm and 20-cm distances from the ultraviolet source.
Ultraviolet (UV) radiation is a part of the non-ionizing region of the electromagnetic spectrum, within which the UVA component has the longest wavelength, followed by the UVB and UVC components, which have the shortest wavelengths. UVA (3200–4000 Å) represents the least hazardous component of UV radiation. Both the inhibitory and stimulatory effects on biomass accumulation and morphology are common responses of plants to UVA radiation. Internal and external factors, such as enzymes and UV radiation, can have a significant impact on the synthesis and accumulation of secondary metabolites, which are both very complicated processes. UVB radiation improved the generation of hydroxyl, hydrogen peroxide, superoxide radicals and singlet oxygen [1],[2]. The superoxide dismutase enzyme and peroxidase, which scavenge ROS (reactive oxygen species) and protect lipids, proteins and nucleic acids, are activated when plants respond to oxidative injury [3],[4]. For protection from damage, phenolic and flavonoid compounds are also produced by plants as UVB-absorbing compounds [5]. Secondary metabolites have been synthesized as a result of abiotic stresses like drought, a deficiency of the nutrients in the soil and heavy metal pollution [6],[7]. Plants' enzymatic and non-enzymatic antioxidants provide adequate defense against ROS and free radicals produced as a result of the photooxidative damage caused by UVB radiation [8],[9]. Seeds of Nigella sativa, garden cress, Ammi majus and Foeniculum vulgare were exposed to UVC radiation for varying lengths of time and from various distances, and their growth, internal structure, antioxidants and vitamins changed as a result [10]–[13]. A significant increase in the bioactive components of Prunella vulgaris was observed after exposure to drought [14], heavy metals [15] and different soil nutrient concentrations [16]. Salvia hispanica L. (commonly known as chia seeds) has natural antioxidants such as chlorogenic acid, caffeine, myricetin, quercetin and kaempferol, and is also a good source of vitamins and minerals. UV exposure results in more total phenolic compounds, flavonoids and antioxidants under opposing conditions. For these reasons, a primary goal of this research was to study and analyze the effects of VUA on the molecular structure, chemical composition and phenolic compounds of medicinal Salvia hispanica seeds.
Salvia hispanica L. seeds received from the Egyptian Ministry of Agriculture were used as research samples. The UV radiation system consisted of one fluorescent lamp (type-A) that was completely covered with aluminum foil to illuminate the sample from all sides. The physical structure of the Salvia hispanica L. seeds were studied by using scanning electron microscopy (SEM, JEOL JSM-6510LV, Japan), and the molecular structure was studied by using a Nicolet™ iS™ 10 FT-IR spectrometer from the USA. The data were analyzed by applying the analysis of variance using SPSS software, and the differences among the means were determined for significance at P < 0.05 using Tukey's test.
The total phenolic content was determined using the Florin–Ciocalteu method described by Attard [17]. The procedure consisted of mixing 10 µL of the sample/standard with 100 µL of Folin-Ciocalteu reagent (diluted 1: 10) in a 96-well microplate. Then, 80 µL of 1 M Na2CO3 was added and incubated at room temperature (25 °C) for 20 min in the dark. At the end of the incubation time, the resulting blue complex color was measured at 630 nm. The data are represented as mean ± SD.
The total flavonoid content was determined using the aluminum chloride method described by Kiranmai et al. [18], with minor modifications that were carried out in the microplates. Briefly, 15 µL of the sample/standard was placed in a 96-well microplate; then, 175 µL of methanol was added, followed by 30 µL of 1.25% AlCl3. Finally, 30 µL of 0.125 M C2H3NaO2 was added and incubated for 5 min. At the end of the incubation time, the resulting yellow color was measured at 420 nm. The data are represented as mean ± SD.
Concentrations ranging from 0.4 g / 100 g to 2 g / 100 g were prepared with methanol from each sample (100 µL) extract and 2,2-diphenyl-1-picrylhydrazyl (DPPH) radicals (100 µL, 2 mm) were dissolved in methanol. The mixture was stirred and left to stand for 15 min in the dark. Then, the absorbance was measured at 517 nm against a blank. The percentage scavenging effect was calculated as [(Ao – A1)/A0] × 100, where Ao is the absorbance of the control (without the seed sample) and A1 is the absorbance in the presence of the seed sample.
The carbohydrate content was calculated using the following equation: Carbohydrate content % = 100 – [% protein + % ash + % lipids + % fiber]. The moisture content was determined according to the method described by AOAC (Association of Official Agricultural Chemists) [19]. A known weight of air-dried seeds (2 g) was dried at 105 °C in an air-drying oven to obtain a constant weight. Then, the percentage of the moisture content was calculated. The ash content was determined according to AOAC, as follows. Exactly 2 g of the air-dried seeds were place in a silica crucible and ignited at 600 °C in a muffle furnace to obtain a constant weight; the percentage of ash content was then calculated.
Crude fiber is a mixed material that is defined as the sum of the lignin and polysaccharide contents that cannot be digested by a diluted acid or alkali. The crude fiber content was estimated according to the method described by AOAC. A known weight of the air-dried seeds (2 g) was mixed with 0.5 g of asbestos; then, 200 mL of sulphuric acid (1.25% v/v) was added. The mixture was boiled under reflux for 30 minutes, followed by being subjected to filtration through a Gooch crucible. The residue was boiled again with an aqueous sodium hydroxide solution (200 mL, 1.25% w/v) for 30 minutes, and then filtration was repeated in the same manner. Finally, the residue was washed with hot water, followed by diethyl ether, and dried at 110 °C to a constant weight. The contents of the Gooch crucible were then ignited in the muffle furnace at 600 °C to a constant weight. The fiber content was calculated by subtracting the ash content from the weight of the digested sample. The percentage of crude fiber content was then calculated.
The crude protein content was determined using the official Kjeldahl method described in AOAC, as follows. A known weight of the air-dried seeds (0.5 g) was digested with 8 mL of concentrated sulphuric acid in a Kjeldahl flask in the presence of 2.14 g of the digestion mixture [1 kg of potassium sulphate and 60 g of mercuric oxide (red)]. After digestion, the solution was treated with 10 mL of 40% sodium hydroxide solution. The liberated NH3 was received in 10 mL of 1% boric acid in the presence of two drops of Tachero indicator (1.25 g of methyl red + 0.32 g of methylene blue in 1 L of 90% ethanol). The received ammonia was titrated with 0.01 M sulphuric acid. The percentage of total nitrogen was estimated and the crude protein content was calculated by using 6.25 as a factor of protein.
The total phenolic content in the Salvia hispanica seeds varied after being exposed to UVA radiation, as presented in Figure 1, which shows increases of 18.53% and 76.2% after exposure for 1 hour at 5-cm and 20-cm distances, respectively, but decreases of 33.5% and 22.1% after exposure for 4 hours at the same respective distances from the UVA source. UV promotes phenylalanine ammonia lyase activity which increases the production of phenolic metabolites that were used to protect against UV induced damage or antioxidants [20],[21].
The flavonoid content in the Salvia hispanica seeds varied after exposure to UVA radiation, as shown in Figure 2, where shows increase of 23.5% and 27.3% after being exposed for 1 hour at 5-cm and 20-cm distances, respectively, but decreases of 18.8% and 7.5% after exposure for 4 hours at the same respective distances from the UV source. The change in flavonoid content in the Salvia hispanica seeds was due to the flavonoids acting as a protective wall against UV radiation damage [4],[22], and this change depends on the exposure time and distance from the source.
Figure 3 shows that the DPPH scavenging activity of the Salvia hispanica L. seeds varied after exposure to UVA, increasing after being exposed for 1 hour or 4 hours at 5-cm and 20-cm distances from the source [23],[24].
IR (Infrared) spectral graphs, which were derived based on the relation between the wavenumber (X-axis) and transmittance (Y-axis) for the Salvia hispanica after exposure to UVA radiation for 1, 2, 3, or 4 hours at 5-cm and 20-cm distances from the source, are shown in Figure 4. IR spectrum analysis of the Salvia hispanica shows that the % transmittance intensity, broadness and position at ≅ 1745, ≅ 2925 and ≅ 3420 cm–1 varied after UVA exposure. This means that the molecular bonds {C-O bond ≅ 1745 cm–1, C-H bond ≅ 2925 cm–1 and O-H bond ≅ 3420 cm–1} in the Salvia hispanica were changed after UVA exposure because each interatomic bond vibrated with several different motions and individual bonds were absorbed at more than one IR frequency.
Figure 5 shows the SEM images of the Salvia hispanica seeds following UVA exposure for 1 hour or 4 hours at 5-cm and 20-cm distances. The micrographs show a change in the Salvia hispanica seed structure, such as the shape, size, interconnection and orientation of the molecules. These structural changes and cell disruptions were due to the oxidative stress caused by the UVA radiation interacting with the molecules.
The chemical composition results for the Salvia hispanica seeds following UVA exposure, as listed in Table 1, show significant changes in the carbohydrates, protein and fats, with little variation in the total fibers. The carbohydrate content increased after 1 hour of exposure and decreased after 4 hours of exposure at 5- and 20-cm distances from the UVA source. The protein content increased after being exposed to UVA radiation for 1 hour or 4 hours at a 5-cm distance. The fat content decreased after exposure for 1 hour, then increased after exposure for 4 hours at 5- and 20-cm distances.
Time (h) | Exposure at 5 cm | |||||
Carbohydrates | Protein | Fats | Moisture | Fibers | Ash | |
control | 11.04% | 15.56% | 12.4% | 7.18% | 57.4% | 3.6% |
1 | 22.46% | 16.31% | 10.3% | 8.52% | 46.5% | 4.43% |
4 | 1.54% | 16.44% | 23.2% | 8.01% | 54.9% | 3.92% |
Time (h) | Exposure at 20 cm | |||||
Carbohydrates | Protein | Fats | Moisture | Fibers | Ash | |
control | 11.04% | 15.56% | 12.4% | 7.18% | 57.4% | 3.6% |
1 | 14.95% | 11.69% | 10.7% | 7.05% | 58.5% | 4.16% |
4 | 9.32% | 16.94% | 16.1% | 7.65% | 53.7% | 3.94% |
The effects of UVA radiation are based on the interactions with the atoms or molecules in the cells, particularly water, to produce free radicals, which damage different components or compounds, such as the hydroxyl groups, proteins or fats, and induce changes in the enzymatic and non-enzymatic antioxidants. UV rays have enough energy to break, destroy or disturb the atoms and molecules in the cell matrices of plant seeds. The change in antioxidant enzymes was directly linked to protein synthesis. The irradiation of seeds with UV radiation disturbs the synthesis of proteins, enzyme activity and water exchange [25]. The variations in the phenolic and flavonoid contents of the seeds may be credited to the degradation of larger compounds into smaller ones by the UV radiation [26]. The antioxidant content and DPPH of the seeds varied after exposure to UV radiation because it works as a protective wall for the cells against the harmful effects of UV radiation.
A significant change (P < 0.001) in the phenolic content of the Salvia hispanica seeds was observed after exposure to UVA radiation for different periods time and at dissimilar distances; this is because the UVA radiation affected the accumulation of the phenolic compounds, which are capable of breaking the hydroxyl bonds of the poly phenols, thereby releasing soluble phenols of low molecular weight. Also, a change was observed in the chemical composition, including the protein, after exposure to UVA radiation; this affected the phenolic content. The changes in the hydroxyl groups (O-H) which play important roles in carrying the proteins and in phenolic compound transfer, caused a variation in phenolic content.
A significant effect (P < 0.001) was observed on the flavonoids which is the chief group of phenolics; the content decreased after UVA exposure. It decreased from by 6.4% to 15.96% and from 6.1% to 58.3% after exposure for 1 hour and 4 hours, respectively, at distances of 5 cm and 20 cm from the UV source. UV radiation has enough energy to break the chemical bonds that cause photochemical reactions, which affected the flavonoid content of the Salvia hispanica seeds. The UV radiation affected the biological compounds, phenolic compounds and hydroxyl bonds of the seeds because of their water content, and that affected the capacity of the ions to penetrate and reach the embryo, which had an effect on their structural phenolic compounds.
A significant change (P < 0.001) was observed in the percentage scavenging effect (i.e., the DPPH scavenging activity) of the Salvia hispanica seeds, which varied after UVA exposure at 5- and 20-cm distances from the UVA source for different periods time; this is because it represented the biotic stress response to UV radiation, or acted as a wall to protect the biological cell from UV radiation-induced stress.
The chemical composition and biological contents of the Salvia hispanica seeds changed after exposure to UVA radiation due to penetration and subsequent reaction or collision with almost all structural and functional organic molecules, as well as the absorption of UVA energy that resulted in the cracking of or damage to the hydroxyl groups, oxygen molecules, carbons, proteins, fats, carbohydrates, etc.
The molecular structure, including the arrangement, size, orientation or interconnection of molecules, as well as the bonding strength of the Salvia hispanica seeds changed after being exposed to UVA radiation for 1, 2, 3 or 4 hours at 5-cm and 20-cm distances from the UV source. The chemical composition, including the proteins, carbohydrates, fats, total fiber and phenolic compounds (phenols and flavonoids) of the Salvia hispanica seeds were greatly affected by the exposure to UVA radiation. The antioxidant content, i.e., DPPH, of the Salvia hispanica seeds varied after exposure to UV radiation.
[1] |
A.-H.-Mackerness S, Surplus SL, Jordan BR, et al. (1998) Effects of supplementary ultraviolet-B radiation on photosynthetic transcripts at different stages of leaf development and light levels in pea (Pisum sativum L.): Role of active oxygen species and antioxidant enzymes. Photochem Photobiol 68: 88-96. https://doi.org/10.1111/j.1751-1097.1998.tb03257.x ![]() |
[2] |
He YY, Häder DP (2002) Involvement of reactive oxygen species in the UV-B damage to the cyanobacterium Anabaena sp. J Photoch Photobio B 66: 73-80. https://doi.org/10.1016/S1011-1344(01)00278-0 ![]() |
[3] | Jain K, Kataria S, Guruprasad KN (2004) Effect of UV-B radiation on antioxidant enzymes and its modulation by benzoquinone and α-tocopherol in cucumber cotyledons. Curr Sci India 87: 87-90. https://www.jstor.org/stable/24107986 |
[4] |
Zu YG, Pang HH, Yu JH, et al. (2010) Responses in the morphology, physiology and biochemistry of Taxus chinensis var. mairei grown under supplementary UV-B radiation. J Photoch Photobio B 98: 152-158. https://doi.org/10.1016/j.jphotobiol.2009.12.001 ![]() |
[5] |
Tegelberg R, Julkunen-Tiitto R, Aphalo PJ (2001) The effects of long-term elevated UV-B on the growth and phenolics of field-grown silver birch (Betula pendula). Global Change Biol 7: 839-848. https://doi.org/10.1046/j.1354-1013.2001.00453.x ![]() |
[6] |
Shohael AM, Ali MB, Yu KW, et al. (2006) Effect of light on oxidative stress, secondary metabolites and induction of antioxidant enzymes in eleutherococcus senticosus somatic embryos in bioreactor. Process Biochem 41: 1179-1185. https://doi.org/10.1016/j.procbio.2005.12.015 ![]() |
[7] |
Pu GB, Ma DM, Chen JL, et al. (2009) Salicylic acid activates artemisinin biosynthesis in Artemisia annua L. Plant Cell Rep 28: 1127-1135. https://doi.org/10.1007/s00299-009-0713-3 ![]() |
[8] |
Rai R, Meena RP, Smita SS, et al. (2011) UV-B and UV-C pre-treatments induce physiological changes and artemisinin biosynthesis in Artemisia annua L.—an antimalarial plant. J Photoch Photobio B 105: 216-225. https://doi.org/10.1016/j.jphotobiol.2011.09.004 ![]() |
[9] |
Takshak S, Agrawal SB (2015) Defense strategies adopted by the medicinal plant Coleus forskohlii against supplemental ultraviolet-B radiation: augmentation of secondary metabolites and antioxidants. Plant Physiol Bioch 97: 124-138. https://doi.org/10.1016/j.plaphy.2015.09.018 ![]() |
[10] | El-Bediwi AB, Hasanin S, Abdelrazek A, et al. (2018) Influence of UVC on growth behavior, internal structure, enzymes and free radical of Nigella Sativa plant. Res Rev Biosic 13: 142. |
[11] | El-Bediwi AB, Hasanin S, Abdelrazek A, et al. (2018) Effect of ultraviolet on morphological and secondary metabolites content of garden cress. Int J Sci Res Sci Eng Techn 4: 187-194. |
[12] | El-Bediwi AB, Hager Y, El-Shora HM (2020) UVC radiation effects on the internal structure and medical contents of ammimajus. Int J Biotech & Bioeng 6: 7-21. |
[13] | El-Bediwi AB, Hager Y, El-Shora HM (2021) Effect of Ultraviolet radiation on growth, structure, and bio-contents of Foeniculum vulgare. Int J Med Res Health Sci 10: 94-102. |
[14] | Chen YH, Guo QS, Liu L, et al. (2011) Influence of fertilization and drought stress on the growth and production of secondary metabolites in Prunella vulgaris L. J Med Plants Res 5: 1749-1755. https://doi.org/10.5897/JMPR.9000792 |
[15] | Wu Z, Guo Q, Wang Q, et al. (2010) Effects of lead, copper and cadmium stresses on growth and inherent quality of Prunalla vulgaris. China J Chin Mater Med 35: 263-267. https://doi.org/10.4268/cjcmm20100301 |
[16] |
Chen Y, Yu M, Zhu Z, et al. (2013) Optimisation of potassium chloride nutrition for proper growth, physiological development and bioactive component production in Prunella vulgaris L. Plos One 8: e66259. https://doi.org/10.1371/journal.pone.0066259 ![]() |
[17] |
Attard E (2013) A rapid microtitre plate Folin-Ciocalteu method for the assessment of polyphenols. Open Life Sci 8: 48-53. https://doi.org/10.2478/s11535-012-0107-3 ![]() |
[18] | Kiranmai M, Kumar CBM, Mohammed I (2011) Comparison of total flavanoid content of Azadirachta indica root bark extracts prepared by different methods of extraction. Res J Pharm Biol Chem Sci 2: 254-261. http://rjpbcs.com/pdf/2011_2(3)/31.pdf |
[19] | Horwitz W, Fisher HJ, Robertson AH, et al. (1955) Official methods of analysis of the association of official agricultural chemists. Association of Official Analytical Chemists. Washington, D.C.: . https://doi.org/10.1093/clinchem/2.5.375a |
[20] | Hideg É, Jansen MAK, Strid Å (2013) UVB exposure, ROS, and stress: Inseparable companions or loosely linked associates? Trends Plant Sci 18: 107-115. https://doi.org/10.1016/j.tplants.2012.09.003 |
[21] |
Köhler H, Contreras RA, Pizarro M, et al. (2017) Antioxidant responses induced by UV-B radiation in deschampsia antarctica Desv. Front Plant Sci 8: 921. https://doi.org/10.3389/fpls.2017.00921 ![]() |
[22] |
Sun M, Gu X, Fu H, et al. (2010) Change of secondary metabolites in leaves of Ginkgo biloba L. in response to UV-B induction. Innov Food Sci Emerg 11: 672-676. https://doi.org/10.1016/j.ifset.2010.08.006 ![]() |
[23] | Dang YF (2015) Influence of enhanced UV-B radiation on Prunella vulgaris ‘growth and various of chemical index components [dissertation], Xi'an: Northwest University. Xi'an: . |
[24] |
Liu RL, Xiong QJ, Shu Q, et al. (2012) Hyperoside protects cortical neurons from oxygen-glucose deprivation—reperfusion induced injury via nitric oxide signal pathway. Brain Res 1469: 164-173. https://doi.org/10.1016/j.brainres.2012.06.044 ![]() |
[25] | Hameed A, Shah TB, Atta BM, et al. (2008) Gamma irradiation effects on seed germination and growth, protein content, peroxidase and protease activity, lipid peroxidation in desi and kabuli chickpea. Pak J Bot 40: 1033-1041. |
[26] |
Harrison K, Were LM (2007) Effect of gamma irradiation on total phenolic content yield and antioxidant capacity of almond skin extracts. Food Chem 102: 932-937. https://doi.org/10.1016/j.foodchem.2006.06.034 ![]() |
Time (h) | Exposure at 5 cm | |||||
Carbohydrates | Protein | Fats | Moisture | Fibers | Ash | |
control | 11.04% | 15.56% | 12.4% | 7.18% | 57.4% | 3.6% |
1 | 22.46% | 16.31% | 10.3% | 8.52% | 46.5% | 4.43% |
4 | 1.54% | 16.44% | 23.2% | 8.01% | 54.9% | 3.92% |
Time (h) | Exposure at 20 cm | |||||
Carbohydrates | Protein | Fats | Moisture | Fibers | Ash | |
control | 11.04% | 15.56% | 12.4% | 7.18% | 57.4% | 3.6% |
1 | 14.95% | 11.69% | 10.7% | 7.05% | 58.5% | 4.16% |
4 | 9.32% | 16.94% | 16.1% | 7.65% | 53.7% | 3.94% |
Time (h) | Exposure at 5 cm | |||||
Carbohydrates | Protein | Fats | Moisture | Fibers | Ash | |
control | 11.04% | 15.56% | 12.4% | 7.18% | 57.4% | 3.6% |
1 | 22.46% | 16.31% | 10.3% | 8.52% | 46.5% | 4.43% |
4 | 1.54% | 16.44% | 23.2% | 8.01% | 54.9% | 3.92% |
Time (h) | Exposure at 20 cm | |||||
Carbohydrates | Protein | Fats | Moisture | Fibers | Ash | |
control | 11.04% | 15.56% | 12.4% | 7.18% | 57.4% | 3.6% |
1 | 14.95% | 11.69% | 10.7% | 7.05% | 58.5% | 4.16% |
4 | 9.32% | 16.94% | 16.1% | 7.65% | 53.7% | 3.94% |