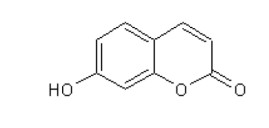
The aim of the study was to evaluate the effect of coumarin and Bacillus cereus derivatives on the productivity and ecology of the caecum of broilers. Assessment of phytochemicals and their synergistic effect with probiotics on the ecological state of individual organs and systems of the body of animals is important for increasing their productivity. The need to address these problems provides sufficient motivation to expand the search for new antimicrobial products. According to the research results, the activity of cellular biomasses to suppress quorum sensing (QS) (Chromobacterium violaceum 026) in Bacillus cereus and a coumarin derivative was revealed. In experiments on animals, i.e. broiler chickens (4 groups, n = 30). Control group: had basic diet (BD); I experimental: was fed with BD + Bacillus cereus probiotic (BC); II experimental: BD + coumarin (CO); III experimental: BD + Bacillus cereus + coumarin (BC + CO). Metagenomic analysis of the bacterial profile of the cecum contents showed the prevalence of representatives of Bacteroidetes phylum in the experimental groups (4.5–15.2%) at 14 days of age, and a decrease in the percentage of Firmicutes phylum (5.9–14.8%), the dominance of Bacteroidaceae families was noted. On the 21st day, a similar increase in representatives of Bacteroidetes phylum (1.7–20.8%), a decrease in Firmicutes and Proteobacteria were noted. At the family level, there is a quantitative change in the microbiome, both in comparison with the control, and with similar data obtained on the 7th day of the experiment. The vast majority belonged to representatives of Bacteroidetes phylum, an increase in bacteria belonging to Firmicutes and Proteobacteria was observed, Rikenellaceae and Ruminococcaceae were minor families. Thus, the additional introduction of Bacillus cereus and a coumarin derivative into the diet of broilers improved the growth parameters and the structure of the intestines of broilers; a positive synergistic effect of Bacillus cereus and coumarin in maintaining the microecological balance of the intestines in poultry was noted.
Citation: Galimzhan Duskaev, Olga Kvan, Dianna Kosyan, Shamil Rakhmatullin, Georgii Levakhin. Coumarin derivative and Bacillus cereus change live weight and cecal ecology in broilers[J]. AIMS Agriculture and Food, 2021, 6(1): 360-380. doi: 10.3934/agrfood.2021022
[1] | Osama A.F. Abdel Wahab, Hassan M. Sobhy, Azza M. M. Badr, Abdallah A. Ghazalah . Effect of Moringa oleifera seeds powder on performance and immunity of broiler chicks. AIMS Agriculture and Food, 2020, 5(4): 896-910. doi: 10.3934/agrfood.2020.4.896 |
[2] | Giovanni Gamba, Dario Donno, Zoarilala Rinah Razafindrakoto, Paolo Sabbatini, Gabriele Loris Beccaro . Development of a preliminary extraction protocol for phenol compounds during table grape grafting formation. AIMS Agriculture and Food, 2024, 9(2): 628-644. doi: 10.3934/agrfood.2024034 |
[3] | Brechtje R. de Haas, Nyncke J. Hoekstra, Jan R. van der Schoot , Eric J.W. Visser, Hans de Kroon, Nick van Eekeren . Combining agro-ecological functions in grass-clover mixtures. AIMS Agriculture and Food, 2019, 4(3): 547-567. doi: 10.3934/agrfood.2019.3.547 |
[4] | Nicholas Mawira Gitonga, Gilbert Koskey, Ezekiel Mugendi Njeru, John M. Maingi, Richard Cheruiyot . Dual inoculation of soybean with Rhizophagus irregularis and commercial Bradyrhizobium japonicum increases nitrogen fixation and growth in organic and conventional soils. AIMS Agriculture and Food, 2021, 6(2): 478-495. doi: 10.3934/agrfood.2021028 |
[5] | Yenni, Mohd Hafiz Ibrahim, Rosimah Nulit, Siti Zaharah Sakimin . Influence of drought stress on growth, biochemical changes and leaf gas exchange of strawberry (Fragaria × ananassa Duch.) in Indonesia. AIMS Agriculture and Food, 2022, 7(1): 37-60. doi: 10.3934/agrfood.2022003 |
[6] | John Samelis, Athanasia Kakouri . Major technological differences between an industrial-type and five artisan-type Greek PDO Galotyri market cheeses as revealed by great variations in their lactic acid microbiota. AIMS Agriculture and Food, 2019, 4(3): 685-710. doi: 10.3934/agrfood.2019.3.685 |
[7] | Sonia Maria Lima Santos do Vale, Amauri Siviero, Lauro Saraiva Lessa, Eduardo Pacca Luna Mattar, Paulo Arthur Almeida do Vale . Biotechnological potential of endophytic bacteria of bamboo Guadua sp. for promotion of growth of micropropagated yam plants (Dioscorea rotundata Poir). AIMS Agriculture and Food, 2020, 5(4): 850-867. doi: 10.3934/agrfood.2020.4.850 |
[8] | Itxaso Filgueira-Garro, Carolina González-Ferrero, Diego Mendiola, María R. Marín-Arroyo . Effect of cultivar and drying methods on phenolic compounds and antioxidant capacity in olive (Olea europaea L.) leaves. AIMS Agriculture and Food, 2022, 7(2): 250-264. doi: 10.3934/agrfood.2022016 |
[9] | Frikkie A. Maré, Yonas T. Bahta . The impact of live sheep export trade on the South Africa economy. AIMS Agriculture and Food, 2023, 8(1): 25-47. doi: 10.3934/agrfood.2023002 |
[10] | Gregorio Gullo, Antonio Dattola, Vincenzo Vonella, Rocco Zappia . Performance of the Brasiliano 92 orange cultivar with six trifoliate rootstocks. AIMS Agriculture and Food, 2021, 6(1): 203-215. doi: 10.3934/agrfood.2021013 |
The aim of the study was to evaluate the effect of coumarin and Bacillus cereus derivatives on the productivity and ecology of the caecum of broilers. Assessment of phytochemicals and their synergistic effect with probiotics on the ecological state of individual organs and systems of the body of animals is important for increasing their productivity. The need to address these problems provides sufficient motivation to expand the search for new antimicrobial products. According to the research results, the activity of cellular biomasses to suppress quorum sensing (QS) (Chromobacterium violaceum 026) in Bacillus cereus and a coumarin derivative was revealed. In experiments on animals, i.e. broiler chickens (4 groups, n = 30). Control group: had basic diet (BD); I experimental: was fed with BD + Bacillus cereus probiotic (BC); II experimental: BD + coumarin (CO); III experimental: BD + Bacillus cereus + coumarin (BC + CO). Metagenomic analysis of the bacterial profile of the cecum contents showed the prevalence of representatives of Bacteroidetes phylum in the experimental groups (4.5–15.2%) at 14 days of age, and a decrease in the percentage of Firmicutes phylum (5.9–14.8%), the dominance of Bacteroidaceae families was noted. On the 21st day, a similar increase in representatives of Bacteroidetes phylum (1.7–20.8%), a decrease in Firmicutes and Proteobacteria were noted. At the family level, there is a quantitative change in the microbiome, both in comparison with the control, and with similar data obtained on the 7th day of the experiment. The vast majority belonged to representatives of Bacteroidetes phylum, an increase in bacteria belonging to Firmicutes and Proteobacteria was observed, Rikenellaceae and Ruminococcaceae were minor families. Thus, the additional introduction of Bacillus cereus and a coumarin derivative into the diet of broilers improved the growth parameters and the structure of the intestines of broilers; a positive synergistic effect of Bacillus cereus and coumarin in maintaining the microecological balance of the intestines in poultry was noted.
Antimicrobial resistance poses a serious threat to human and animal health and well-being; it has a huge impact on food security [1]. The need to address these issues provides sufficient motivation to expand the search for potential drugs from different sources [2]. Plants provide a rich reservoir of compounds with multiple biological properties, including antimicrobial properties [3,4,5], making them a good resource for finding useful and new antimicrobial products.
At the same time, it should be borne in mind that ecological and phylogenetic factors are important in the development of the chemical diversity of plants [6]. The recently discovered phenomenon of "Horizontal natural product transfer" also applies to phenolic plant metabolites, their chemical analogs, and is important for the ecology of plants, the ecology of feed prepared from them, and the ecology of animals consuming these feeds. Therefore, it is known that coumarin, which is also contained in agricultural forage plants (in barley seedlings), was modified by methoxylation, followed by the production of scopoletin [7]. Numerous alkaloids of various structural types are able to diffuse through biomembranes and are absorbed by acceptor plants; this phenomenon is essential for all objects of plant origin, especially for the production of environmentally friendly phytopharmaceuticals [8]. In this regard, the assessment of impact of certain phytochemicals on the animal body is important for the overall ecological safety of food.
Coumarin derivatives may be promising substances in plant extracts [9,10,11]. Thus, recent studies have revealed that coumarins are able to suppress pathogenic plant bacteria [12], reduce the virulence and biofilm formation in Pseudomonas aeruginosa [13], including acting on the quorum of bacteria [14,15,16], low cytotoxic activity [17], are effective against aflatoxicosis in animals [18,19], reduces oxidative stress [20]. At the same time, there is insufficient information on the effect of coumarin derivatives on immunity, productivity and ecology of microorganisms of the gastrointestinal tract of farm animals.
Probiotic substances, including spore-forming ones, for example, Bacillus cereus, are also considered as alternative substances from the point of view of biological safety for animal nutrition. It was earlier believed [21] that all B. cereus strains are toxin producers, while the assessment of their danger depends on their further study and their effect on biological systems. There are probiotic Bacillus strains used as feed additives for animals [22,23], although their mechanism of action is not fully understood. Probiotics enjoy GRAS status and are freely used throughout the world. Their efficacy and safety have been demonstrated in several clinical trials in vitro, in vivo [24,25,26,27], nevertheless several recent studies have raised concerns about their biosecurity and dose in immunocompromised patients [28]. Although Bacillus probiotics have an overall excellent reputation for promoting health, especially in the prevention and treatment of diarrhea, and in maintaining gut homeostasis [29], their use in poultry farming is under-represented and requires careful study.
The aim of the study was to evaluate the effect of coumarin and Bacillus cereus derivatives on the productivity and ecology of the caecum of broilers.
The protocol of the present investigation was approved by the Local Ethics Committee of the Orenburg State University, Orenburg, Russia. All animal studies have been performed in accordance with the ethical standards laid down in the 1964 Declaration of Helsinki and its later amendments.
The active substance of coumarin is shown in Figure 1.
Bacillus cereus strain IP 5832 (ATCC 14893) is a part of probiotic preparations. The primary isolation of microorganisms was carried out on LB agar (Sigma-Aldrich, United States); further cultivation was carried out in LB broth at 37 ± 1℃ with dynamic growth monitoring at 545 nm (OD545). After 72 hours, the bacterial biomass was separated by centrifugation for 10 minutes at 12000 g (MiniSpin, Eppendorf, Germany), and the resulting supernatants were sterilized through a sterile syringe filter attachment, CA (cellulose acetate) (Membrane Solutions, USA) with a pore diameter of 0.22 μm.
The characteristics of the microorganism used to assess the QS LuxI/LuxR-type system C. violaceum ATCC 31532 are presented in Table 1.
Name of biosensor | Genetic peculiarity | Sensor protein | Auto-inducer | Indicator system | Effective feature |
C. violaceum ATCC 31532 | Violacein production is controlled by Quorum sensing | CviR | C6-GSL | vioABEDC | Violacein pigment biosynthesis |
Quantitative accounting of QS inhibitory activity was carried out by measuring the optical density of biomass at a light wavelength of 520 nm and pigmentation at a wavelength of 600 nm using a Stat Fax 303 6VIS microstrip photometer "Awareness Technology Inc" (USA).
Experimental studies were carried out on 120 heads of 7-day broiler chickens (4 groups, n = 30). Control group – BD (Control); Experimental I: BD + probiotic strain of Bacillus cereus (4 ml/kg) (BC); Experimental II: BD + coumarin (9 μg/kg) (CO); Experimental III: BD + Bacillus cereus strain + coumarin (BC + CO) (Table 2). Poultry feeding and drinking was carried out by a group method.
Ingredient composition (%) | Starter (1–20 days) | Grower (21–34 days) | Finisher (35–42 days) |
Control, BC, CO, BC + CO | |||
Wheat | 47.0 | 44.5 | 42.0 |
Barley | 2.6 | 1.45 | 0.3 |
Corn | 7.5 | 14.75 | 22.0 |
Soybean meal (46 % protein) | 25.0 | 20.0 | 15.0 |
Sunflower meal (38 % protein) | 7.0 | 8.5 | 10.0 |
Sunflower oil | 5.0 | 5.0 | 5.0 |
Dicalcium phosphate | 1.6 | 1.5 | 1.4 |
Mel stern | 0.9 | 1.2 | 1.5 |
Limestone | 0.5 | 0.4 | 0.3 |
Salt | 0.36 | 0.28 | 0.2 |
DL-methionine | 0.18 | 0.17 | 0.16 |
L-lysine | 0.35 | 0.26 | 0.17 |
Vitamin-mineral premixa* | 2.0 | 2.0 | 2.0 |
Calculated nutrients metabolizable energy (kcal g-1) | 296.0 | 299.0 | 302.0 |
Crude protein | 22.0 | 20.3 | 18.7 |
Methionine + cysteine | 0.87 | 0.83 | 0.79 |
Lysine | 1.35 | 1.15 | 0.96 |
Calcium | 0.95 | 0.98 | 1.01 |
Available phosphorus | 0.54 | 0.51 | 0.48 |
Note: *Supplied following per kilogram of diet (natural substance): Vitamin A = 7000 IU, Vitamin D3 = 800.0 IU, Vitamin E = 9 IU, Vitamin K3 = 1.1 mg, Thiamine = 0.7 mg, Riboflavin = 3.0 mg, Vitamin B6 = 1 mg, Vitamin B12 = 0.01 mg, Vitamin C = 50 mg, Mn = 23 mg, Fe = 17 mg, Zn = 11 mg, Cu = 2.5 mg, I = 0.4 mg, Se = 0.2 mg. |
The live weight of broilers was determined by the method of weekly weighing of individuals of all groups, in the morning, before feeding and drinking. The feed intake for the experiment was determined by weighing the feed in poultry feeding and feed residues at the end of the day.
Feed conversion rate was calculated as the ratio of feed weight consumed per the experiment for absolute growth to the live weight of broiler at the end of the experiment. Sampling of the contents of the cecal part of the intestine of broilers was carried out on the 42nd day after the slaughter of the bird (ether). Animal care and experimental studies were performed in accordance with the instructions and recommendations of Russian Regulations, 1987 (Order No.755 on 08/12/1977 the USSR Ministry of Healt hand "The Guide for Care and Use of Laboratory Animals (National Academy Press Washington, DC 1996). Efforts have been made in the studies to minimize animal suffering and reduce the number of samples used.
Metagenomic sequencing of genomic DNA (chemical extraction method) was carried out on days 7, 21 and 42 using MiSeq (Illumina) and a MiSeq v3 reagent kit with 2 × 300.
To assess the microbial communities of rumen of cattle, ruminal fluid was sampled. For this, samples of from fistuled animals in an amount of 300 ml were taken before feeding and three hours after the start of feeding. Samples were placed in sterile Eppendorf tubes (Nuova Aptaca S.R.L., Italy), frozen at −70℃ (ULUF65 ARCTICO freezer, Denmark) and stored, preventing re-freezing.
DNA was isolated from the obtained samples by chemical extraction. For this, each sample was incubated in 300 μl of sterile lysis buffer, which was prepared by mixing EDTA 20 mmol/L, NaCl 1400 mmol/L, Tris-HCl 100 mmol/L, pH = 7.5, with the addition of 50 μl of lysozyme in concentration 100 mg/ml at 37℃ for 30 minutes. Subsequently, 10 μl of proteinase K was added at a concentration of 10 mg/ml and sodium dodecyl sulfate to its final concentration of 1.0% and incubated for 30 min at 60℃.
DNA was purified with a mixture of phenol and chloroform, precipitated by adding sodium acetate and three volumes of absolute ethanol at −20℃ for 4 hours. After extraction with phenol-chloroform-isoamyl alcohol (25:24:1) and chloroform-isoamyl alcohol (24:1), DNA was precipitated in the aqueous phase with ammonium acetate (1 mol/L to 10% vol.) And a three-fold volume of anhydrous ethanol during nights at −20℃. After centrifugation and double washing with 80% ethanol, DNA was dried and dissolved in TE buffer.
To assess the purity of the extraction from foreign DNA, a negative parallel control was established by treating 100 μl of autoclaved deionized water using the same method as described above. DNA purity was checked by electrophoresis using a 1.5% agarose gel. DNA concentration was quantified using a Qubit 2.0 fluorometer with dsDNA high sensitivity analysis (Life Technologies).
Preparation of DNA libraries, as well as sequencing, was carried out at the Common Use Centre "Persistence of Microorganisms" of the Institute of Cellular and Intracellular Symbiosis of the Ural Branch of Russian Academy of Sciences (Orenburg, Russia). 16S DNA libraries were prepared according to Illumina workflow with primers targeting the V3-V4 regions of the SSU rRNA gene, namely SD-Bact-0341-bS-17 and reverse SD-Bact-0785-aA-21. Libraries were sequenced in MiSeq (Illumina) using MiSeq V3 reagent kit with 2 × 300 base pairs.
Data analysis was carried out using USEARCH v8.0.1623_win32 (Edgar R.S., 2010) and included the merging of pair readings, quality filtering and choice of amplicon size (minimum size of 415 base pairs). During filtration, reading with N or a total average Q score of < 15 were discarded. Filtering quality assessment was performed using Fast QC v 0.11.3. As a result of decomposition and clustering with USEARCH, operational taxonomic units (OTUs) were determined using similarity levels between sequences of at least 97% to classify the microorganism at the species level. Chimera detection was performed through UCHIME (24) using USEARCH and chimeric sequences were removed. Contaminating OTUs were found and removed using the USEARCH command-articular sequence corresponding to sequences from negative control samples. A taxonomic classification of sequences was performed using VAMPS and the SILVA reference database [30].
Statistical processing was carried out using the Statistica 10.0 application (Stat Soft Inc., USA). Analysis of poultry live weight included determination of the arithmetic mean (M), standard error of the mean (m). Differences were considered significant at p ≤ 0.05. For bioinformatic processing of sequencing results, the PEAR program (Pair-End AssembeR, PEAR v0.9.8) was used.
Determination of the GSL concentration at EC50 when working on Chromobacterium violaceum 026 revealed the activity of cell biomass to suppress QS in Bacillus cereus and a coumarin derivative (Table 3).
Bacterial strain | Concentration of С6-GSL at EC50 (mM) |
Control | 0.31 |
B. cereus | 2.50 |
Coumarin | 1.13 |
Thus, these substances have a pronounced anti-quorum activity and can be used in experiments on animals.
Studies on poultry (broilers) showed that the feed conversion rate was less than in the control group by 4.2% and 3.6%, respectively for the entire period of the experiment in the experimental groups BC and CO (Table 4). In experimental group BC + CO, the lowest feed consumption was noted, which is by 9.7% less than in the control. The feed conversion rate of chickens from the experimental groups was higher than that of their peers from the control group throughout the entire experiment, and the maximum was in the BC + CO experimental group, which in absolute value was 3.99 kg/bird, which is by 10.0% higher than the control group.
Experiment period, weeks | Group | Two-way ANOWA (P-values) | |||||
Control | BC | CO | BC+CO | BC | CO | BC+CO | |
1 | 362.2 ± 19.2 | 354.4 ± 9.62 | 361.56 ± 9.7 | 370.4 ± 13.3 | 0.722 | 0.975 | 0.556 |
2 | 707.3 ± 57.8 | 741.0 ± 27.2 | 786.0 ± 17.8 | 783.7 ± 19.9 | 0.609 | 0.222 | 0.906 |
3 | 1215.0 ± 91.1 | 1297.0 ± 47.0 | 1371.7 ± 31.0 | 1400.0 ± 57.2 | 0.442 | 0.112 | 0.536 |
4 | 1768.0 ± 45.7 | 1896.7 ± 137.7 | 2044.7 ± 44.5 | 2139.3 ± 120.9 | 0.425 | 0.012 | 0.357 |
5 | 2388.7 ± 70.5 | 2605.3 ± 173.8 | 2694.7 ± 42.6 | 2886.7 ± 180.0 | 0.312 | 0.020 | 0.297 |
Feed consumption per experiment, kg/head | 3.59 ± 0.12 | 3.77 ± 0.274 | 3.94 ± 0.07 | 3.99 ± 0.27 | 0.578 | 0.059 | 0.831 |
Feed conversion rate* | 1, 65 ± 0, 05 | 1, 58 ± 0, 12 | 1, 59 ± 0, 03 | 1, 49 ± 0, 10 | 0.679 | 0.350 | 0.349 |
Note: Data were presented as mean ± standard error. Feed conversion rate: Feed consumption per experiment / absolute growth live weight of broiler. |
The birds of the experimental groups (CO, BC + CO) prevailed over birds from the control and BC experimental groups according to live weight growth intensity. It should be noted that superiority began to manifest already from the age of three weeks and persisted until the end of the experiment. So, on the third week, a significant difference by 13.2% (p ≤ 0.05) was revealed in group BC + CO, compared with the control. On the fourth week of the experiment, the CO experimental group exceeded the control by 13.5% (p ≤ 0.05), and the BC + CO group by 17.4% (p ≤ 0.05). At the end of the experiment, the studied bird of the CO experimental group reached an average live weight of 2694.7 g, it is 11.4% (p ≤ 0.05) higher than the control, in BC + CO experimental group it is 2886.7 g, that is by 17.3% (p ≤ 0.05) exceeded the control group.
Thus, the calculations showed that live weight was the highest in the group of broilers that additionally received Bacillus cereus IP 5832 and coumarin. On average, over 5 weeks of the experiment, the increase in live weight in this group is 19.5% higher than that of the control group. The use of methods of statistical data processing made it possible to establish that the adaptation of the bird's body to drugs occurs within 14 days. The drugs have the maximum effect in the period 28–35 days.
Analyzing the bacterial profile of samples of the cecum contents of broiler chickens from the control group, on the 7th day of the experiment, 117 OTUs were identified, which can be attributed to 2 phyla of eubacteria, whose representatives accounted for 99% of microflora. In particular, representatives of Bacteroidetes accounted for 69.1%, and the share of bacteria in Firmicutes accounted for 30.8%. Other groups of bacteria that were not identified during the study made up no more than 0.1% of the total.
In the analysis of the bacterial profile of the contents of the cecum of broiler chickens from the BC experimental group (addition of a probiotic preparation), 97 OTUs were identified, which can be attributed to 3 phyla. Bacteroidetes were identified as the dominant phylum, as in the control, but the content of representatives of this phylum is by 4.5% higher than in the control group and is 74.5%. As a consequence, a decrease in the percentage of microorganisms of the phylum Firmicutes is observed, and the difference was 5.9% relative to the control. Bacteria belonging to the phylum Actinobacteria in percentage terms make up 0.5% of the total number of microorganisms. The share of other phyla was no more than 0.1% of the total. Metagenomic sequencing of the contents of the cecum of the CO experimental group (addition of coumarin) revealed 88 OTUs, which correspond to 3 phyla. In the control and BC experimental group, the representatives of the phylum Bacteroidetes (75.3%) are dominants. Their number is 6.2% higher than in the control, the difference between groups BC and CO was minimal and amounted to 0.8%. The phylum Firmicutes accounts for 24.4% of the total microorganism content; this is 6.3% lower than in the control group. The difference between groups BC and CO was minimal and amounted to 0.4%. The phylum Actinobacteria accounted for only 0.2% of the total number of bacteria. The share of other phyla did not exceed 0.1% of the total mass of the microbiota. The microbial profile of broiler chickens of the BC + CO experimental group (a combination of coumarin and a probiotic preparation) was represented by microorganisms belonging to 4 phyla. The phylum Bacteroidetes (83.4%) was the dominant species, as in the groups described above, but the difference was maximum. Comparison with the control showed that with a combination of coumarin and a probiotic strain, an increase in bacteria of this phylum was observed by 15.2%, with an intergroup comparison, this indicator was higher by 8.9% relative to group BC and by 8.1% relative to group CO. Against the background of the above, there is a decrease in the number of representatives of Firmicutes by 14.8% relative to the control, by 8.9% and 8.5% compared to groups BC and CO, respectively. Phylums Actinobacteria and Proteobacteria accounted for 0.5% and 0.1% of the total number of microorganisms (Figure 2A).
Assessing the diversity of families and genera identified in the control group, the phylum Bacteroidetes is represented by the families Bacteroidaceae (31.3%) and Rikenellaceae (37%). They are minor, since they account for more than 50% of the total number of microorganisms. The phylum Firmicutes is represented in most of the families of the Clostridia class, in particular, Ruminococcaceae (24.3%), Lachnospiraceae (2.1%), and unclassified Clostridiales (3.2%). The share of other families was not more than 1%; it should be noted that almost half (0.6%) were non-cultivated forms. In terms of the qualitative composition at the family level, the differences in the experimental groups compared with the control were minimal, however, differences in the quantitative ratio were observed. So, the dominant family is Bacteroidaceae, the percentage of its representatives is almost 2 times higher and is 62.6%. At the same time, there is a decrease in the percentage of bacteria of the family Rikenellaceae and Ruminococcaceae by 25.4% and 4.7%, respectively. The share of unclassified representatives is 7.6%. The share of other bacteria in their taxonomic group did not exceed 1%. In the CO experimental group, according to the quantitative ratio of families, it can be noted that the dominant identified families were Bacteroidaceae (61.6%), Rikenellaceae (13.4%) and Ruminococcaceae (16.7%) just like in the groups described above. However, a comparative analysis showed that bacteria of the Bacteroidaceae family are 30.2% higher than in the control group and 1.4% lower than in group BC. The percentage of representatives of the family Rikenellaceae was by 23.7% lower than in the control and by 1.7% higher than in group BC. With the addition of coumarin, a decrease in the percentage of bacteria of the Ruminococcaceae family is observed by 7.6% in comparison with the control and by 2.9% in comparison with the first experimental group. Other families in their taxonomic category did not exceed 1%. Unclassified groups of microorganisms accounted for 0.1%. Carrying out a comparative assessment at a lower taxonomic level, it can be noted that only in group BC + CO the dominant family is Rikenellaceae, since its representatives make up more than 50.0% of the microflora. The difference with the control is 15.0%, with group BC 40.3% and 38.7% with group CO. Against this background, there is a change in the percentage of bacteria of the Bacteroidaceae family (31.3%) in comparison with the groups, since the values were the same with the control. Their content decreased by 31.3% relative to group BC and by 30.3% relative to group CO. The number of microorganisms of the Ruminococcaceae family was lower by 15.9% compared to the control, by 11.2% and 8.3% compared to groups BC and CO, respectively. Representatives of the Lachnospiraceae family were within 10.0%, including non-classified forms 2.7%. Other families account for no more than 1.0% (Figure 2B).
Assessing the generic diversity in the control and experimental groups, a number of differences can be noted. Thus, the control revealed a variety of bacterial genera, among them the dominant position (more than 5.0%) is occupied by the genus Bacteroides (31.4%) and Alistipes (37.1%). The content of representatives of the genus Faecalibacterium did not exceed 8.0%, it should be noted that unidentified forms of bacteria account for 12.1% of the total microbial profile (Figure 3A). In experimental group BC, the dominant species was Bacteroides, its content was 62.6%, which is 31.2% higher than in the control. In this case, the percentage of bacteria of the genera Alistipes and Faecalibacterium decreases by 25.4% and 6.1%, respectively. Considering other identified genera, the content of which did not exceed 5.0%, an increase in the percentage of microorganisms of the Pseudoflavonifractor genus by 6.3% can be noted (Figure 3B). In experimental group CO, the main majority in the microflora is made up of Bacteroides (61.6%), Alistipes (13.4%), Faecalibacterium (13.3%) and Ruminococcus (5.6%). Comparing their content with the control group, it can be noted that bacteria of the genus Bacteroides were more by 30.2%, Alistipes were less by 23.7%, and Faecalibacterium and Ruminococcus were more by 5.5% and 4.1%, respectively. The remaining groups of microorganisms did not exceed 1.0% in terms of their number. It should be noted that the unclassified forms of microorganisms accounted for 2.5% in total (Figure 3C). The main majority in the microflora of group BC + CO are the genus Alistipes (52.1%), Bacteroides (31.3%). These categories of microorganisms also dominated in the above groups. However, the percentage of content is different. Therefore, representatives of the genus Alistipes were 15.0% more than in the control; by 40.4% than in group BC and by 38.7% than in group CO. The content of bacteria of the genus Bacteroides did not differ from the control group, but was lower by 30.0% than in groups BC and CO. The remaining groups of microorganisms did not exceed 1.0% in terms of their number. It should be noted that unclassified forms of microorganisms accounted for 5.2% in total (Figure 3D).
Analysis of metagenomic sequencing of cecum contents of the control and experimental groups of broiler chickens on the 21st day showed a quantitative and qualitative change in the composition of the microbiome (Figure 4). Thus, 168 OTUs belonging to 3 phyla of eubacteria were identified in the content of the control, while representatives of the phylum Firmicutes already occupied a dominant position, their number increased by 22.3% in comparison with the control group on the 7th day. There is also a decrease in the percentage of representatives of the phylum Bacteroidetes by 27.8%. The share of the phylum Actinobacteria was 2.8% of the total number of microorganisms.
In experimental group BC, in comparison with the control (21 days), there was an increase in the representatives of the phylum Bacteroidetes by 20.8% and a decrease in the representatives of the phyla Firmicutes and Proteobacteria by 16.3% and 1.9%, respectively. When comparing with similar indicators of the microbial status of group BC on the 7th day, an insignificant increase in the representatives of individual found phyla can be noted. Thus, the percentage of bacteria of the phyla Bacteroidetes and Actinobacteria decreased by 12.4% and 0.4%, respectively, against the background of an increase in the representatives of the phyla Firmicutes by 12.0% and Proteobacteria by 0.9%.
In experimental group CO, Bacteroidetes and Firmicutes were the dominant phyla on the 21st day of the experiment, as on the 7th day, however, their content changed in comparison with the control. Thus, there is an increase in the percentage of microorganisms belonging to Bacteroidetes by 13.9 % against the background of a decrease in the bacteria of Firmicutes by 10.5%, Actinobacteria by 0.8%, and Proteobacteria by 2.7%. Analysis of the results of metagenomic sequencing of samples of the CO experimental group on the 7th and 21st days among themselves showed that over time there was a decrease in the number of representatives of the phyla Bacteroidetes and Proteobacteria by 20.1% and 0.1%, respectively, while the number of representatives phyla Firmicutes and Actinobacteria increased by 18.2% and 2.0%.
Comparing the data on metagenomic sequencing of samples of the contents of the cecum of the BC + CO experimental group on the 21st day with a similar control group, a number of changes can be noted. Thus, against the background of a decrease in the number of representatives of the phyla Firmicutes and Actinobacteria by 19.9% and 2.6%, there is an increase in bacteria belonging to the phyla Bacteroidetes by 10.7% and Proteobacteria by 11.6%. A big difference is noted in the data obtained in this group on the 7th and 21st days. So, on the 21st day, there is a decrease in the content of bacteria belonging to the phylum Bacteroidetes by 31.4%, while there is an increase in the number of representatives of Firmicutes, Actinobacteria and Proteobacteria by 17.3%, 0.1% and 13.9%, respectively.
Assessing the lower taxonomic category in the control group on the 21st day of the experiment, it can be noted that the dominant family was also Rikenellaceae (31%), however, comparing the sequencing data on the 21st and 7th days, a sharp decrease in the percentage of microorganisms of the family Bacteroidaceae by 21.7% is also remarkable. At the same time, the content of bacteria of the Ruminococcaceae family changed insignificantly and amounted to 25.5%. The number of other families did not exceed 10.0%.
In experimental group BC, at the family level, there is a quantitative change in the microbiome both in comparison with the control and with similar data obtained on the 7th day of the experiment. Therefore, the dominant family is Rikenellaceae, the percentage of which increased by 20.4% relative to the control and by 39.8% compared to the same data on the 7th day. The number of representatives of the Bacteroidaceae family was slightly higher than in the control (by 0.5%), however, in comparison with similar data on the 7th day, their number decreased by 52.4%. At the same time, there is a decrease in the percentage of bacteria of the Ruminococcaceae family by 20.9% relative to the control and by 7.3% lower than the data on the 7th day, respectively. The share of unclassified representatives is 0.5%.
In experimental group CO, the dominant families were Rikenellaceae and Ruminococcaceae on day 21, but their content varied relative to the control. There were 14.4% more bacteria of the Rikenellaceae family and 1.9% less Ruminococcaceae than in the control group. Compared with similar data on the 7th day, on the 21st day, there is an increase in the number of bacteria of the Rikenellaceae families by 32.1%, Ruminococcaceae by 14.6%, against the background of a decrease in microorganisms of the Bacteroidaceae family by 52.1%.
In experimental group BC + CO, the minor family on the 21st day was Rikenellaceae, the percentage of its representatives was 11.4% higher than in the control. There is also an increase in the number of bacteria of the Enterobacteriaceae family by 11.7% relative to the control. In comparison with the data obtained on the 7th day of the experiment, the Rikenellaceae family also remains the dominant family, but its content decreased by 9.6%. There is also a 22.5% decrease in the percentage of bacteria belonging to the Bacteroidacea family. Against this background, there is an increase in the number of representatives of the families Clostridiales, Ruminococcaceae and Enterobacteriaceae by 10.2%, 6.8% and 14.0%, respectively. The share of unclassified bacteria of the phylum Firmicutes is 2.5%; the rest of the bacteria make up 3.7% in total.
On the 42nd day of the experiment, significant changes were observed in the microbial profile of broiler chickens in the control group. In comparison with the results obtained on the 21st day, there is an increase in the representatives of the phyla Bacteroidetes and Actinobacteria by 9% and 30.3%, respectively. At the same time, the percentage of microorganisms of the phyla Firmicutes and Proteobacteria decreases by 36.9% and 2.6%, respectively. Evaluating the overall results at the beginning and end of the experiment, one can observe both smooth and sharp dynamics in the change in the microflora of the cecum over time.
In experimental group BC, on the 42nd day of the experiment, the main majority belonged to representatives of the phylum Bacteroidetes; relative to the control, they were more by 18.9%. There was also an increase in bacteria belonging to the phyla Firmicutes and Proteobacteria by 12.3% and 0.2%, respectively, while the percentage of representatives of the phylum Actinobacteria was 31.3% less than in the control group. When compared with the data obtained on day 21, an increase in the number of representatives of the phyla Bacteroidetes and Actinobacteria by 7.0% and 1.9%, respectively, can be noted, against the background of a decrease in the number of bacteria belonging to other isolated phyla. In particular, the phylum Firmicutes - by 8.3%, the phylum Proteobacteria - 0.6%.
In experimental group CO, on the 42nd day, the content of bacteria of the phylum Bacteroidetes was practically equal to the control, while the number of representatives of phyla Firmicutes increased by 32.3%, Proteobacteria - 0.6%. At the same time, the percentage of representatives of the phylum Actinobacteria was 32.7% less than in the control group. Comparison of similar indicators on the 21st and 42nd days showed that during this period there was a decrease in the number of representatives of the phyla Bacteroidetes and Actinobacteria by 5.4% and 1.3%, respectively, against the background of an increase in the number of representatives of the phyla Firmicutes, Proteobacteria by 5.9% and 0.6%, respectively.
A number of changes also took place in the BC + CO experimental group. On the 42nd experimental day, there was an increase in the number of representatives of the phyla Bacteroidetes, Firmicutes and Proteobacteria by 9.7%, 22.7% and 0.4%, respectively, in comparison with the control group. The content of bacteria of the phylum Actinobacteria was 32.8% lower than the control. Analysis of the data on the 21st and 42nd day of the experiment showed that during this period of time there was an increase in the number of representatives of the phyla Bacteroidetes, Firmicutes and Actinobacteria by 7.9%, 5.9% and 0.3%, respectively, against this background, the number of representatives of the phyla Proteobacteria by 13.8% (Table 5).
Phylum | 7 days | 21 days | 42 days |
Control | |||
Bacteroidetes | 69, 1 | 41, 2 | 50, 2 |
Firmicutes | 30, 8 | 53, 2 | 16, 3 |
Actinobacteria | 0, 01 | 2, 8 | 33, 3 |
Proteobacteria | 0 | 2, 7 | 0, 1 |
BC | |||
Bacteroidetes | 74, 6 | 62, 1 | 69, 1 |
Firmicutes | 24, 9 | 36, 9 | 28, 6 |
Actinobacteria | 0, 5 | 0, 1 | 2 |
Proteobacteria | 0 | 0, 9 | 0, 3 |
CO | |||
Bacteroidetes | 75, 3 | 55, 4 | 50 |
Firmicutes | 24, 5 | 42, 7 | 48, 6 |
Actinobacteria | 0 | 2 | 0, 7 |
Proteobacteria | 0, 2 | 0, 1 | 0, 7 |
BC + CO | |||
Bacteroidetes | 83, 4 | 52 | 59, 9 |
Firmicutes | 16 | 33, 3 | 39 |
Actinobacteria | 0, 1 | 0, 2 | 0, 5 |
Proteobacteria | 0, 5 | 14, 4 | 0, 6 |
Based on the above, changes have occurred at a lower taxonomic level (Figure 5). At the end of the experiment, 2 dominant families were noted in the control group: Coriobacteriaceae and Rikenellaceae. Their content did not exceed 34%. It should be noted that there are significantly more representatives of the Coriobacteriaceae family than at the beginning of the experiment. The content of the Rikenellaceae family was relatively constant over the entire reference period. The difference between the data on the 7th, 21st and 42nd days was in the range from 5 to 8%. However, a sharp decrease from 24.3% (on the 7th day) to 7.5 on the 42nd day should be noted in other dominant family of Ruminococcaceae.
In group BC, on the 42nd day of the experiment, the Rikenellaceae family was characteristic. This family was considered minor, since its content exceeded 50%. In comparison with the control, the content of this family was almost 2 times higher. In general, throughout the entire accounting period, there is an upward trend (from 11.7% to 66.3%). Against this background, the percentage of representatives of the Bacteroidaceae family decreased (from 62.6% to 2.6%). The relative consistency in number was recorded among the Ruminococcaceae family. The difference between the accounting periods was about 6%, and then only at the beginning of the experiment.
In experimental group CO, minor families were Rikenellaceae and Ruminococcaceae. At the same time, the content of the Rikenellaceae family slightly differed from the control values, and over the entire period there was a tendency to their growth (from 13.4% to 35.9% with a maximum of 45.5% on day 21). A similar picture was observed in relation to the Ruminococcaceae family. It should be noted that the Bacteroidaceae family, which at the initial stage constituted the main majority (61.6%), at the end of the reference period was 13.7%.
Analysis of the sequencing data of samples of the cecum of broiler chickens of the BC + CO experimental group on the 42nd day of the experiment showed that the main majority are representatives of the family Rikenellaceae. Their content was 24.9% higher than the control. Throughout the entire accounting period, their content varied slightly in the range of 5%. The Ruminococcaceae family also makes up the majority in comparison with other families. The number of bacteria of this family was higher than the control values by 16.4%. During the entire accounting period, their content increased.
The Shannon and Simpson diversity indices were used for quantitative description of the generic diversity of microbial communities (Table 6).
Groups | Period of experiment, days | ||
7 | 21 | 42 | |
Shannon index | |||
Control | 1, 80 | 1, 56 | 2, 18 |
BC | 1, 47 | 1, 80 | 1, 57 |
CO | 1, 32 | 0, 99 | 1, 29 |
BC + CO | 1, 38 | 1, 89 | 1, 79 |
Simpson index | |||
Control | 0,018 | 0,016 | 0,022 |
BC | 0,015 | 0,018 | 0,016 |
CO | 0,013 | 0,011 | 0,013 |
BC + CO | 0,014 | 0,019 | 0,018 |
Based on the data obtained, it can be noted that the maximum diversity on the 7th day is typical for the control group (1.80), and the minimum is for the BC + CO experimental group. A similar situation is typical for IP results. On the 21st day, it can be noted that the maximum diversity is typical for the BC + CO experimental group (1.89), and the minimum - for the CO. A similar trend is characteristic for Simpson index results. At the end of the experiment, the maximum diversity was observed in the control group, and the minimum in group CO. Thus, it can be noted that the trend remains within the groups. However, the data obtained contradict the main provisions of theoretical ecology, which indicates the unreliability of the obtained effects of the investigated substances.
Our results confirm the previously obtained information that various isolates belonging to the Bacillus cereus group have QS inhibitory activity, including the ability to inhibit the formation of biofilms of Pseudomonas aeruginosa, and have probiotic potential [31]. In addition, the results for coumarin are consistent with recent studies highlighting the ability of coumarins to block QS signaling systems and inhibit biofilm formation in clinically significant pathogens [32].
Determination of GSL concentration at EC50 working on Chromobacterium violaceum 026 revealed the activity of cell biomass to suppress QS in Bacillus cereus and a coumarin derivative in the luxR-luxI type system. In contrast to our studies, the quorum-sensory system PlcR-PapR quenching in Bacillus cereus was previously studied [33]. There is little information on the effect of coumarin and Bacillus cereus on productivity and general health of poultry in the available sources. There are conflicting data on Bacillus on growth performance of chickens [34,35] it is assumed that this may be associated with various bacterial strains [36] and the manifested effects. At the same time, there is evidence that Osthole (a coumarin derivative) in broiler diets increases growth rates [37]. This effect was observed in our CO experimental group. It is known that the introduction of Bacillus, including Bacillus cereus (108 cfu/kg) into the diet of broilers, significantly improved the final body weight, daily gain [38], it was also confirmed in BC group.
In turn, the positive synergistic effect of the combined action of the probiotic drug and coumarin on the live weight of animals is indirectly confirmed by the studies of [39,40].
The extent after the addition of B. cereus and coumarin, including synergistic effects, can affect the composition and functionality of the intestinal microbiota, is hardly studied. In the last decade, research into the poultry microbiome has gained increasing popularity [41]. This is because the microflora of various parts of the gastrointestinal tract is involved in digestion and assimilation of nutrients, supporting the immune system's metabolic processes [42]. At the same time, there are many factors that affect the structure of the microbiome, for example, age, gender, type of diet, etc. [43,44]. In terms of diversity, the part of the cecum surpasses the others both in the complexity of the structure and in the absolute number of microorganisms [45]. A number of studies show that the dominant place in the structure of the microflora of this section is occupied by two phyla: Firmicutes and Bacteroidetes [46]. The percentage of representatives of these phyla reaches 80% of the total number of microorganisms in this section of the gastrointestinal tract [47]. This consequence correlates with the results obtained in the course of our experiment. The exception is the results obtained on day 42 in the control group, where at the end of the study there is a sharp jump in representatives of the phylum Actinobacteria. This fact can be explained by the influence of age, as one of the most important factors that determine the structure of the bird microbiome [48].
Evaluating the effect of the drugs used in the experiment, it can be noted that the effect is observed not at the phylum level, but at lower taxonomic levels. In particular, during the entire experimental period, changes were observed in the number of such families as Rikenellaceae, Ruminococcaceae and Bacteroidaceae. These are important groups of microorganisms that are involved in metabolism [49]. At the same time, the maximum fluctuations in the number of these microorganisms can be observed under the action of the probiotic preparation and coumarin separately. Studies have shown that the effect of a probiotic affects the composition of the microbiome of poultry, in particular, the number of Bacteroidaceae, which are involved in the metabolism of glucose and amino acids [31]. However, against the background of an increase in some "beneficial" bacteria, there is a decrease in others, which are also important for the body. A change in their numbers indicates a consequence of inflammation [41].
The mechanisms affecting the growth of broilers in the groups may be associated with modulation of broiler intestinal microbiota. So it is assumed that probiotics, plant phenols, including coumarins, improve the oxidative and anti-inflammatory status of the intestine, preserve the function of the epithelial barrier [23,24,25,26]. Probiotics increase the concentration of some beneficial bacteria [13], and B. cereus increase the height, width, depth of intestinal villi, and surface area [9].
In addition, Bacteroides, prevailing in the experimental groups, plays an important role in the breakdown of complex molecules into simpler compounds, stimulation of the production of anti-inflammatory cytokines, while Ruminococcus is associated with the degradation and utilization of polysaccharides in the intestines of broilers [21,22,23,24,25,26,27,28,29]. Bacteroidetes are considered the main polysaccharide degraders and are found in all studied ecosystems.
In our experiment, the prevalence of representatives of the Rikenellaceae family and specifically of the genus Alistipes was noted; it was previously noted that a high number of Alistipes positively correlated with body weight [38,39,40,41]. In the genomes of Bacteroidetes, enzymes that break down carbohydrates are located in gene clusters called polysaccharide utilization loci [44,45]. These data make it possible to partially explain the increase in live weight in the experimental groups in comparison with the control.
In conclusion, the addition of Bacillus cereus and a coumarin derivative to broiler diets improved the growth performance and gut structure of broilers, the likely mechanism of which was their role in the formation of gut microbial composition. Specifically, individual and joint supplementation of Bacillus cereus and coumarin has altered broilers' caecal microbiota towards a healthy balance by increasing the number of beneficial bacteria as well as improving the interaction patterns in the community. This study may expand our fundamental knowledge of the synergistic role of Bacillus cereus and coumarin in maintaining intestinal microecological balance in poultry.
This research was performed with financial support from the RSF 16-16-10048 and project 0761-2019-0005.
All authors declare no conflict of interest in this paper.
[1] | Hoelzer K, Wong N, Thomas J, et al. (2017) Antimicrobial drug use in food-producing animals and associated human health risks: what, and how strong, is the evidence? BMC Vet Res 13: 211. |
[2] | Betts JW, Hornsey M, La Ragione RM (2018) Novel Antibacterials: Alternatives to Traditional Antibiotics. Adv Microb Physiol 123–169. |
[3] |
Romulo A, Ea Z, Rondevaldova J, et al. (2020) Screening of in vitro antimicrobial activity of plants used in traditional Indonesian medicine. Pharm Biol 56: 287–293. doi: 10.1080/13880209.2018.1462834
![]() |
[4] | Icdo R, Ega M, Rt C, et al. (2018) Plants Of The Cerrado With Antimicrobial Effects Against Staphylococcus Spp. And Escherichia coli From Cattle. BMC Vet Res 14: 32. |
[5] |
Oo O, Pa S, Ps F (2018) Antimicrobial and antiprotozoal activities of twenty-four Nigerian medicinal plant extracts. S Afr J Bot 117: 240–246. doi: 10.1016/j.sajb.2018.05.028
![]() |
[6] |
Allevato DM, Groppo M, Kiyota E, et al. (2019) Evolution of phytochemical diversity in Pilocarpus (Rutaceae). Phytochemistry 163: 132–146. doi: 10.1016/j.phytochem.2019.03.027
![]() |
[7] |
Hijazin T, Radwan A, Abouzeid S, et al. (2019) Uptake and modification of umbelliferone by various seedlings. Phytochemistry 157: 194–199. doi: 10.1016/j.phytochem.2018.10.032
![]() |
[8] |
Yahyazadeh M, Nowak M, Kima H, et al. (2017) Horizontal natural product transfer: A potential source of alkaloidal contaminants in phytopharmaceuticals. Phytomedicine 34: 21–25. doi: 10.1016/j.phymed.2017.07.007
![]() |
[9] |
He R, Zhang Y, Wu L, et al. (2017) Benzofuran glycosides and coumarins from the bark of Streblus indicus (Bur.) Corner Phytochemistry 138: 170–177. doi: 10.1016/j.phytochem.2017.01.011
![]() |
[10] |
Tuan Anh HL, Kim DC, Ko W, et al. (2017) Anti-inflammatory coumarins from Paramignya trimera. Pharm Biol 55: 1195–1201. doi: 10.1080/13880209.2017.1296001
![]() |
[11] | Deryabin D, Galadzhieva A, Kosyan D, et al. (2019) Plant-Derived Inhibitors of AHL-Mediated Quorum Sensing in Bacteria: Modes of Action. Int J Mol Sci 8: 20–22. |
[12] |
Yang L, Wu L, Yao X, et al. (2018) Hydroxycoumarins: New, effective plant-derived compounds reduce Ralstonia pseudosolanacearum populations and control tobacco bacterial wilt. Microbiol Res 215: 15–21. doi: 10.1016/j.micres.2018.05.011
![]() |
[13] |
Zhang Y, Sass A, Van Acker H, et al. (2018) Coumarin Reduces Virulence and Biofilm Formation in Pseudomonas aeruginosa by Affecting Quorum Sensing, Type III Secretion and C-di-GMP Levels. Front Microbiol 21: 1952. doi: 10.3389/fmicb.2018.01952
![]() |
[14] |
D'Almeida RE, Molina RDI, Viola CM, et al. (2017) Comparison of seven structurally related coumarins on the inhibition of Quorum sensing of Pseudomonas aeruginosa and Chromobacterium violaceum. Bioorg Chem 73: 37–42. doi: 10.1016/j.bioorg.2017.05.011
![]() |
[15] |
Zhang S, Liu N, Liang W, et al. (2017) Quorum sensing-disrupting coumarin suppressing virulence phenotypes in Vibrio splendidus. Appl Microbiol Biotechnol 101: 3371–3378. doi: 10.1007/s00253-016-8009-3
![]() |
[16] |
Reen FJ, Gutiérrez-Barranquero JA, Parages ML, et al. (2018) Coumarin: a novel player in microbial quorum sensing and biofilm formation inhibition. Appl Microbiol Biotechnol 102: 2063–2073. doi: 10.1007/s00253-018-8787-x
![]() |
[17] |
Yasser FM, Moath AN, Zena ST (2018) Coumarins from Creston Apple Seeds: Isolation, Chemical Modification and Cytotoxicity Study. J App Pharm Sci 8: 49–56. doi: 10.7324/JAPS.2018.8908
![]() |
[18] |
Abdel-Latif MS, Elmeleigy KM, Aly TAA, et al. (2017) Pathological and biochemical evaluation of coumarin and chlorophyllin against aflatoxicosis in rat. Exp Toxicol Pathol 69: 285–291. doi: 10.1016/j.etp.2017.01.014
![]() |
[19] |
Hassan AA, Abu HSH, Elghandour MMY, et al. (2019) Dietary Supplementation with sodium bentonite and coumarin alleviates the toxicity of aflatoxin B1 in rabbits. Toxicon 171: 35–42. doi: 10.1016/j.toxicon.2019.09.014
![]() |
[20] |
Mahmoud AM, Hozayen WG, Hasan IH, et al. (2019) Umbelliferone Ameliorates CCl4-Induced Liver Fibrosis in Rats by Upregulating PPARγ and Attenuating Oxidative Stress, Inflammation, and TGF-β1/Smad3 Signaling. Inflammation 42: 1103–1116. doi: 10.1007/s10753-019-00973-8
![]() |
[21] | European Commission Health & Consumer Protection Directorate-General (2000) Directorate B - scientific health opinions unit. B3 - management of scientific committees II. Opinion of the scientific committee on animal nutrition on the safety of use of bacillus species in animal nutrition, p 18. |
[22] |
Mietke H, Beer W, Schleif J, et al. (2010) Differentiation between probiotic and wild-type Bacillus cereus isolates by antibiotic susceptibility test and Fourier transform infrared spectroscopy (FT-IR). Int J Food Microbiol 140: 57–60. doi: 10.1016/j.ijfoodmicro.2010.02.009
![]() |
[23] |
Mingmongkolchai S, Panbangred W (2018) Bacillus probiotics: an alternative to antibiotics for livestock production. J Appl Microbiol 124: 1334–1346. doi: 10.1111/jam.13690
![]() |
[24] |
Jeong JS, Kim IH (2014) Effect of Bacillus subtilis C‐3102 spores as a probiotic feed supplement on growth performance, noxious gas emission, and intestinal microflora in broilers. Poult Sci 93: 3097–3103. doi: 10.3382/ps.2014-04086
![]() |
[25] |
Guyard‐Nicodème M, Keita A, Quesne S, et al. (2016) Efficacy of feed additives against Campylobacter in live broilers during the entire rearing period. Poult Sci 95: 298–305. doi: 10.3382/ps/pev303
![]() |
[26] |
Knap I, Kehlet A, Bennedsen M, et al. (2011) Bacillus subtilis (DSM17299) significantly reduces Salmonella in broilers. Poult Sci 90:1690–1694. doi: 10.3382/ps.2010-01056
![]() |
[27] |
Latorre JD, Hernandez‐Velasco X, Bielke L, et al. (2015) Evaluation of a Bacillus direct‐fed microbial candidate on digesta viscosity, bacterial translocation, microbiota composition and bone mineralisation in broiler chickens fed on a rye‐based diet. Br Poult Sci 56: 723–732. doi: 10.1080/00071668.2015.1101053
![]() |
[28] |
Redman MG, Ward EJ, Phillips RS (2014) The efficacy and safety of probiotics in people with cancer: a systematic review. Ann Oncol 25: 1919–1929. doi: 10.1093/annonc/mdu106
![]() |
[29] |
Alkaya B, Laleman I, Keceli S, et al. (2017) Clinical effects of probiotics containing Bacillus species on gingivitis: a pilot randomized controlled trial. J Periodontal Res 52: 497–504. doi: 10.1111/jre.12415
![]() |
[30] | Lopetuso LR, Scaldaferri F, Franceschi F, et al. (2016) Bacillus clausii and gut homeostasis: state of the art and future perspectives. Expert Rev Gastroenterol Hepatol 10: 943–948. |
[31] |
Candela M, Vitali B, Matteuzzi D, et al. (2004) Evaluation of the run operon copy number in Bifidobacterium using real-time PCR. Lett Appl Microbiol 38: 229–232. doi: 10.1111/j.1472-765X.2003.01475.x
![]() |
[32] |
Duskaev GK, Rakhmatullin SG, Kazachkova NM, et al. (2018) Effect of the combined action of Quercus cortex extract and probiotic substances on the immunity and productivity of broiler chickens. Vet World 11: 1416–1422. doi: 10.14202/vetworld.2018.1416-1422
![]() |
[33] |
Crhanova M, Karasova D, Juricova H, et al. (2019) Systematic Culturomics Shows that Half of Chicken Caecal Microbiota Members can be Grown in Vitro Except for Two Lineages of Clostridiales and a Single Lineage of Bacteroidetes. Microorganisms 7: 496. doi: 10.3390/microorganisms7110496
![]() |
[34] |
Petrovic V, Marcincak S, Popelka P, et al. (2012) The effect of supplementation of clove and agrimony or clove and lemon balm on growth performance, antioxidant status and selected indices of lipid profile of broiler chickens. J Anim Physiol Anim Nutr (Berl) 96: 970–977. doi: 10.1111/j.1439-0396.2011.01207.x
![]() |
[35] |
Lu J, Idris U, Harmon B, et al. (2003) Diversity and succession of the intestinal bacterial community of the maturing broiler chicken. Appl Environ Micr 69: 6816–6824. doi: 10.1128/AEM.69.11.6816-6824.2003
![]() |
[36] |
Eeckhaut V, Wang J, Van Parys A, et al. (2016) The Probiotic Butyricicoccus pullicaecorum Reduces Feed Conversion and Protects from Potentially Harmful Intestinal Microorganisms and Necrotic Enteritis in Broilers. Front Microbiol 7: 1416. doi: 10.3389/fmicb.2016.01416
![]() |
[37] |
Waqas M, Wang Y, Li A, et al. (2019) Osthole: A Coumarin Derivative Assuage Thiram-Induced Tibial Dyschondroplasia by Regulating BMP-2 and RUNX-2 Expressions in Chickens. Antioxidants (Basel) 8: 330. doi: 10.3390/antiox8090330
![]() |
[38] |
Kohl KD (2012) Diversity and function of the avian gut microbiota. J Compar Physiol Biochem Syst Environ Physiol 182: 591–602. doi: 10.1007/s00360-012-0645-z
![]() |
[39] |
Kubasova T, Kollarcikova M, Crhanova M, (2019) Gut anaerobes capable of chicken caecum colonisation. Microorganisms 7: 597. doi: 10.3390/microorganisms7120597
![]() |
[40] |
Magoc T, Salzberg SL (2011) FLASH: fast length adjustment of short reads to improve genome assemblies. Bioinformatics 27: 2957–2963. doi: 10.1093/bioinformatics/btr507
![]() |
[41] |
Wei S, Morrison M, Yu Z (2013) Bacterial census of poultry intestinal microbiome. Poult Sci Sympos 92: 671–683. doi: 10.3382/ps.2012-02822
![]() |
[42] |
Shang Y, Kumar S, Oakley B, et al. (2018) Chicken Gut Microbiota: Importance and Detection Technology. Front Vet Sci 5: 254. doi: 10.3389/fvets.2018.00254
![]() |
[43] |
Pan D, Yu Z (2014) Intestinal microbiome of poultry and its interaction with host and diet. Gut Microbes 5: 108–119. doi: 10.4161/gmic.26945
![]() |
[44] |
Oakley BB, Lillehoj HS, Kogut MH, et al. (2014) The chicken gastrointestinal microbiome. FEMS Microbiol Lett 360: 100–112. doi: 10.1111/1574-6968.12608
![]() |
[45] |
Rychlik I (2020) Composition and Function of Chicken Gut Microbiota. Animals 10: 103. doi: 10.3390/ani10010103
![]() |
[46] |
Nordentoft S, Molbak L, Bjerrum L, et al. (2011) The influence of the cage system and colonisation of Salmonella Enteritidis on the microbial gut flora of laying hens studied by T-RFLP and 454 pyrosequencing. BMC Microbiol 11: 187. doi: 10.1186/1471-2180-11-187
![]() |
[47] |
Medvecky M, Cejkova D, Polansky O, et al. (2018) Whole genome sequencing and function prediction of 133 gut anaerobes isolated from chicken caecum in pure cultures. BMC Genom 19: 561. doi: 10.1186/s12864-018-4959-4
![]() |
[48] |
Shaufi MA, Sieo CC, Chong CW, et al. (2015) Deciphering chicken gut microbial dynamics based on high-throughput 16S rRNA metagenomics analyses. Gut Pathol 7: 4. doi: 10.1186/s13099-015-0051-7
![]() |
[49] |
Videnska P, Sedlar K, Lukac M, et al. (2014) Succession and replacement of bacterial populations in the caecum of egg laying hens over their whole life. PLoS One 9: e115142. doi: 10.1371/journal.pone.0115142
![]() |
1. | Galimzhan K. Duskaev, Kristina S. Lazebnik, Tatyana A. Klimova, Microbial diversity in the cecum of broiler chickens after introduction of coumarin and feed antibiotic into the diet, 2022, 17, 2312-7988, 555, 10.22363/2312-797X-2022-17-4-555-566 | |
2. | Dmitry Deryabin, Christina Lazebnik, Ludmila Vlasenko, Ilshat Karimov, Dianna Kosyan, Alexander Zatevalov, Galimzhan Duskaev, Broiler Chicken Cecal Microbiome and Poultry Farming Productivity: A Meta-Analysis, 2024, 12, 2076-2607, 747, 10.3390/microorganisms12040747 | |
3. | Aqsa Ameer, Youqi Cheng, Farrukh Saleem, Aaron McKenna, Anne Richmond, Ozan Gundogdu, William T. Sloan, Sundus Javed, Umer Zeeshan Ijaz, Temporal stability and community assembly mechanisms in healthy broiler cecum, 2023, 14, 1664-302X, 10.3389/fmicb.2023.1197838 |
Name of biosensor | Genetic peculiarity | Sensor protein | Auto-inducer | Indicator system | Effective feature |
C. violaceum ATCC 31532 | Violacein production is controlled by Quorum sensing | CviR | C6-GSL | vioABEDC | Violacein pigment biosynthesis |
Ingredient composition (%) | Starter (1–20 days) | Grower (21–34 days) | Finisher (35–42 days) |
Control, BC, CO, BC + CO | |||
Wheat | 47.0 | 44.5 | 42.0 |
Barley | 2.6 | 1.45 | 0.3 |
Corn | 7.5 | 14.75 | 22.0 |
Soybean meal (46 % protein) | 25.0 | 20.0 | 15.0 |
Sunflower meal (38 % protein) | 7.0 | 8.5 | 10.0 |
Sunflower oil | 5.0 | 5.0 | 5.0 |
Dicalcium phosphate | 1.6 | 1.5 | 1.4 |
Mel stern | 0.9 | 1.2 | 1.5 |
Limestone | 0.5 | 0.4 | 0.3 |
Salt | 0.36 | 0.28 | 0.2 |
DL-methionine | 0.18 | 0.17 | 0.16 |
L-lysine | 0.35 | 0.26 | 0.17 |
Vitamin-mineral premixa* | 2.0 | 2.0 | 2.0 |
Calculated nutrients metabolizable energy (kcal g-1) | 296.0 | 299.0 | 302.0 |
Crude protein | 22.0 | 20.3 | 18.7 |
Methionine + cysteine | 0.87 | 0.83 | 0.79 |
Lysine | 1.35 | 1.15 | 0.96 |
Calcium | 0.95 | 0.98 | 1.01 |
Available phosphorus | 0.54 | 0.51 | 0.48 |
Note: *Supplied following per kilogram of diet (natural substance): Vitamin A = 7000 IU, Vitamin D3 = 800.0 IU, Vitamin E = 9 IU, Vitamin K3 = 1.1 mg, Thiamine = 0.7 mg, Riboflavin = 3.0 mg, Vitamin B6 = 1 mg, Vitamin B12 = 0.01 mg, Vitamin C = 50 mg, Mn = 23 mg, Fe = 17 mg, Zn = 11 mg, Cu = 2.5 mg, I = 0.4 mg, Se = 0.2 mg. |
Bacterial strain | Concentration of С6-GSL at EC50 (mM) |
Control | 0.31 |
B. cereus | 2.50 |
Coumarin | 1.13 |
Experiment period, weeks | Group | Two-way ANOWA (P-values) | |||||
Control | BC | CO | BC+CO | BC | CO | BC+CO | |
1 | 362.2 ± 19.2 | 354.4 ± 9.62 | 361.56 ± 9.7 | 370.4 ± 13.3 | 0.722 | 0.975 | 0.556 |
2 | 707.3 ± 57.8 | 741.0 ± 27.2 | 786.0 ± 17.8 | 783.7 ± 19.9 | 0.609 | 0.222 | 0.906 |
3 | 1215.0 ± 91.1 | 1297.0 ± 47.0 | 1371.7 ± 31.0 | 1400.0 ± 57.2 | 0.442 | 0.112 | 0.536 |
4 | 1768.0 ± 45.7 | 1896.7 ± 137.7 | 2044.7 ± 44.5 | 2139.3 ± 120.9 | 0.425 | 0.012 | 0.357 |
5 | 2388.7 ± 70.5 | 2605.3 ± 173.8 | 2694.7 ± 42.6 | 2886.7 ± 180.0 | 0.312 | 0.020 | 0.297 |
Feed consumption per experiment, kg/head | 3.59 ± 0.12 | 3.77 ± 0.274 | 3.94 ± 0.07 | 3.99 ± 0.27 | 0.578 | 0.059 | 0.831 |
Feed conversion rate* | 1, 65 ± 0, 05 | 1, 58 ± 0, 12 | 1, 59 ± 0, 03 | 1, 49 ± 0, 10 | 0.679 | 0.350 | 0.349 |
Note: Data were presented as mean ± standard error. Feed conversion rate: Feed consumption per experiment / absolute growth live weight of broiler. |
Phylum | 7 days | 21 days | 42 days |
Control | |||
Bacteroidetes | 69, 1 | 41, 2 | 50, 2 |
Firmicutes | 30, 8 | 53, 2 | 16, 3 |
Actinobacteria | 0, 01 | 2, 8 | 33, 3 |
Proteobacteria | 0 | 2, 7 | 0, 1 |
BC | |||
Bacteroidetes | 74, 6 | 62, 1 | 69, 1 |
Firmicutes | 24, 9 | 36, 9 | 28, 6 |
Actinobacteria | 0, 5 | 0, 1 | 2 |
Proteobacteria | 0 | 0, 9 | 0, 3 |
CO | |||
Bacteroidetes | 75, 3 | 55, 4 | 50 |
Firmicutes | 24, 5 | 42, 7 | 48, 6 |
Actinobacteria | 0 | 2 | 0, 7 |
Proteobacteria | 0, 2 | 0, 1 | 0, 7 |
BC + CO | |||
Bacteroidetes | 83, 4 | 52 | 59, 9 |
Firmicutes | 16 | 33, 3 | 39 |
Actinobacteria | 0, 1 | 0, 2 | 0, 5 |
Proteobacteria | 0, 5 | 14, 4 | 0, 6 |
Groups | Period of experiment, days | ||
7 | 21 | 42 | |
Shannon index | |||
Control | 1, 80 | 1, 56 | 2, 18 |
BC | 1, 47 | 1, 80 | 1, 57 |
CO | 1, 32 | 0, 99 | 1, 29 |
BC + CO | 1, 38 | 1, 89 | 1, 79 |
Simpson index | |||
Control | 0,018 | 0,016 | 0,022 |
BC | 0,015 | 0,018 | 0,016 |
CO | 0,013 | 0,011 | 0,013 |
BC + CO | 0,014 | 0,019 | 0,018 |
Name of biosensor | Genetic peculiarity | Sensor protein | Auto-inducer | Indicator system | Effective feature |
C. violaceum ATCC 31532 | Violacein production is controlled by Quorum sensing | CviR | C6-GSL | vioABEDC | Violacein pigment biosynthesis |
Ingredient composition (%) | Starter (1–20 days) | Grower (21–34 days) | Finisher (35–42 days) |
Control, BC, CO, BC + CO | |||
Wheat | 47.0 | 44.5 | 42.0 |
Barley | 2.6 | 1.45 | 0.3 |
Corn | 7.5 | 14.75 | 22.0 |
Soybean meal (46 % protein) | 25.0 | 20.0 | 15.0 |
Sunflower meal (38 % protein) | 7.0 | 8.5 | 10.0 |
Sunflower oil | 5.0 | 5.0 | 5.0 |
Dicalcium phosphate | 1.6 | 1.5 | 1.4 |
Mel stern | 0.9 | 1.2 | 1.5 |
Limestone | 0.5 | 0.4 | 0.3 |
Salt | 0.36 | 0.28 | 0.2 |
DL-methionine | 0.18 | 0.17 | 0.16 |
L-lysine | 0.35 | 0.26 | 0.17 |
Vitamin-mineral premixa* | 2.0 | 2.0 | 2.0 |
Calculated nutrients metabolizable energy (kcal g-1) | 296.0 | 299.0 | 302.0 |
Crude protein | 22.0 | 20.3 | 18.7 |
Methionine + cysteine | 0.87 | 0.83 | 0.79 |
Lysine | 1.35 | 1.15 | 0.96 |
Calcium | 0.95 | 0.98 | 1.01 |
Available phosphorus | 0.54 | 0.51 | 0.48 |
Note: *Supplied following per kilogram of diet (natural substance): Vitamin A = 7000 IU, Vitamin D3 = 800.0 IU, Vitamin E = 9 IU, Vitamin K3 = 1.1 mg, Thiamine = 0.7 mg, Riboflavin = 3.0 mg, Vitamin B6 = 1 mg, Vitamin B12 = 0.01 mg, Vitamin C = 50 mg, Mn = 23 mg, Fe = 17 mg, Zn = 11 mg, Cu = 2.5 mg, I = 0.4 mg, Se = 0.2 mg. |
Bacterial strain | Concentration of С6-GSL at EC50 (mM) |
Control | 0.31 |
B. cereus | 2.50 |
Coumarin | 1.13 |
Experiment period, weeks | Group | Two-way ANOWA (P-values) | |||||
Control | BC | CO | BC+CO | BC | CO | BC+CO | |
1 | 362.2 ± 19.2 | 354.4 ± 9.62 | 361.56 ± 9.7 | 370.4 ± 13.3 | 0.722 | 0.975 | 0.556 |
2 | 707.3 ± 57.8 | 741.0 ± 27.2 | 786.0 ± 17.8 | 783.7 ± 19.9 | 0.609 | 0.222 | 0.906 |
3 | 1215.0 ± 91.1 | 1297.0 ± 47.0 | 1371.7 ± 31.0 | 1400.0 ± 57.2 | 0.442 | 0.112 | 0.536 |
4 | 1768.0 ± 45.7 | 1896.7 ± 137.7 | 2044.7 ± 44.5 | 2139.3 ± 120.9 | 0.425 | 0.012 | 0.357 |
5 | 2388.7 ± 70.5 | 2605.3 ± 173.8 | 2694.7 ± 42.6 | 2886.7 ± 180.0 | 0.312 | 0.020 | 0.297 |
Feed consumption per experiment, kg/head | 3.59 ± 0.12 | 3.77 ± 0.274 | 3.94 ± 0.07 | 3.99 ± 0.27 | 0.578 | 0.059 | 0.831 |
Feed conversion rate* | 1, 65 ± 0, 05 | 1, 58 ± 0, 12 | 1, 59 ± 0, 03 | 1, 49 ± 0, 10 | 0.679 | 0.350 | 0.349 |
Note: Data were presented as mean ± standard error. Feed conversion rate: Feed consumption per experiment / absolute growth live weight of broiler. |
Phylum | 7 days | 21 days | 42 days |
Control | |||
Bacteroidetes | 69, 1 | 41, 2 | 50, 2 |
Firmicutes | 30, 8 | 53, 2 | 16, 3 |
Actinobacteria | 0, 01 | 2, 8 | 33, 3 |
Proteobacteria | 0 | 2, 7 | 0, 1 |
BC | |||
Bacteroidetes | 74, 6 | 62, 1 | 69, 1 |
Firmicutes | 24, 9 | 36, 9 | 28, 6 |
Actinobacteria | 0, 5 | 0, 1 | 2 |
Proteobacteria | 0 | 0, 9 | 0, 3 |
CO | |||
Bacteroidetes | 75, 3 | 55, 4 | 50 |
Firmicutes | 24, 5 | 42, 7 | 48, 6 |
Actinobacteria | 0 | 2 | 0, 7 |
Proteobacteria | 0, 2 | 0, 1 | 0, 7 |
BC + CO | |||
Bacteroidetes | 83, 4 | 52 | 59, 9 |
Firmicutes | 16 | 33, 3 | 39 |
Actinobacteria | 0, 1 | 0, 2 | 0, 5 |
Proteobacteria | 0, 5 | 14, 4 | 0, 6 |
Groups | Period of experiment, days | ||
7 | 21 | 42 | |
Shannon index | |||
Control | 1, 80 | 1, 56 | 2, 18 |
BC | 1, 47 | 1, 80 | 1, 57 |
CO | 1, 32 | 0, 99 | 1, 29 |
BC + CO | 1, 38 | 1, 89 | 1, 79 |
Simpson index | |||
Control | 0,018 | 0,016 | 0,022 |
BC | 0,015 | 0,018 | 0,016 |
CO | 0,013 | 0,011 | 0,013 |
BC + CO | 0,014 | 0,019 | 0,018 |