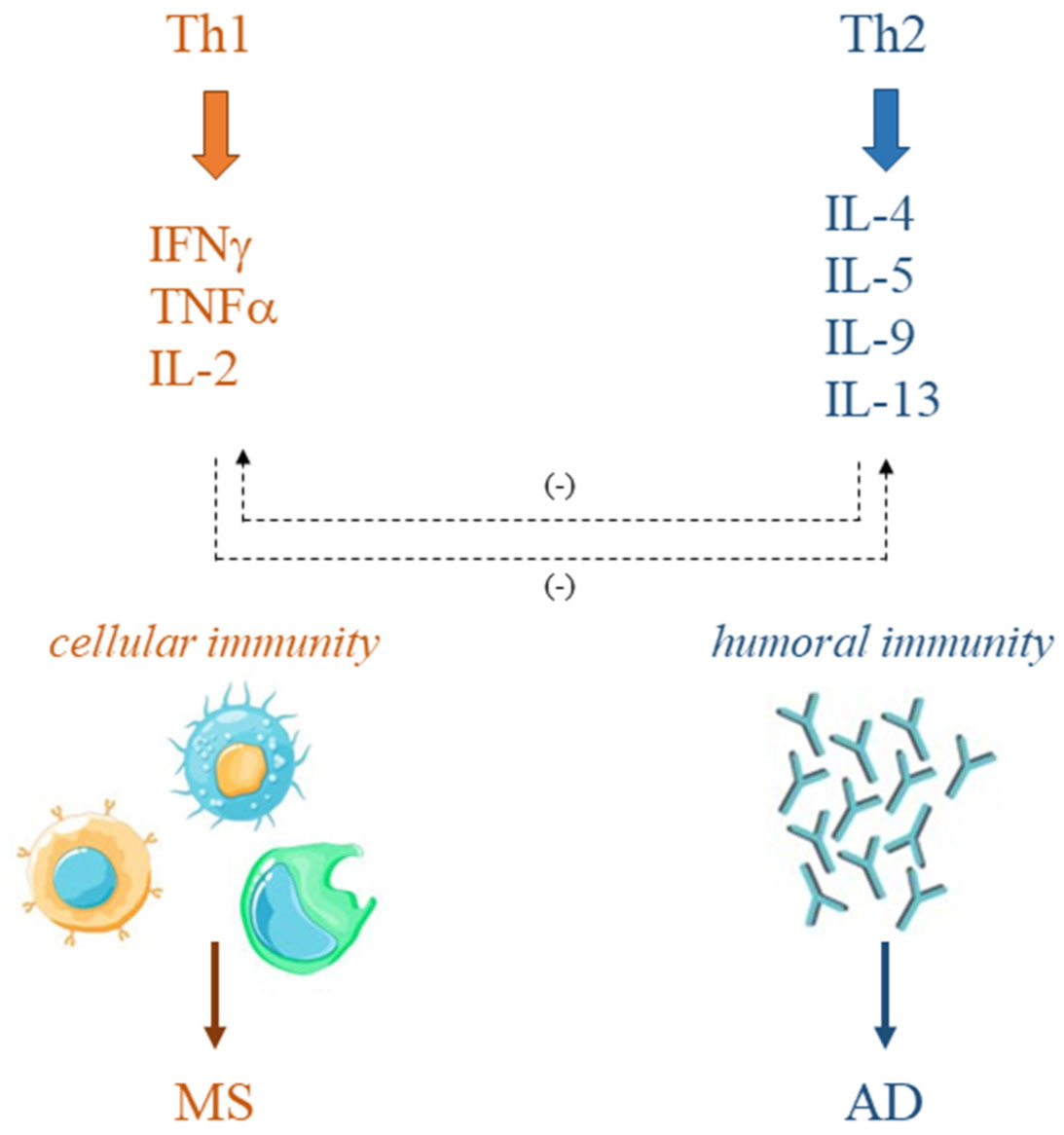
Immune system disorders characterize various diseases such as multiple sclerosis (MS) and allergic diseases (AD). In MS, T-helper (Th)1 cell phenotype is responsible for the disease onset and long-term evolution. On the other hand, excessive Th2 cell activity has been demonstrated in AD. The simultaneous increase of MS and AD in the same geographical areas, observed in recent years, has questioned the mutually exclusive correlation between MS and AD immunopathogenesis supported by the Th1/Th2 paradigm and has moved the interest in understanding possible overlaps. This manuscript aims to discuss the literature, collected over the past two decades, about the association between MS and AD, and both experimental and epidemiological studies have been reviewed. The results do not provide a solid correlation between AD and MS, although experimental studies support the involvement of the same cells and molecules in the immunopathogenesis of both diseases. Further studies, increasing knowledge on the cellular and molecular mechanisms involved in these two disorders, could help to clarify if a positive or negative association links them and provide the possibility for the development of new therapies.
Citation: Lisa Aielli, Federica Serra, Erica Costantini. Multiple sclerosis and allergic diseases: is there a relationship?[J]. AIMS Allergy and Immunology, 2022, 6(3): 126-152. doi: 10.3934/Allergy.2022011
[1] | Haiyan Guo, Mingsheng Lei, Jinan Ma, Hongchun Du, Youming Zhang . Allergy and ferroptosis. AIMS Allergy and Immunology, 2025, 9(1): 8-26. doi: 10.3934/Allergy.2025002 |
[2] | Amolak S Bansal, Alex Nicholas, Nazira Sumar, Veronica Varney . Mast cells, mediators, and symptomatic activation. AIMS Allergy and Immunology, 2024, 8(1): 34-55. doi: 10.3934/Allergy.2024004 |
[3] | Xiujuan Li, Jianmin Lin, Yan Li, Min Zhu, Minchuan Lin, Chenxi Li . Inhalation allergen sensitization patterns in children with allergic rhinitis and asthma. AIMS Allergy and Immunology, 2024, 8(4): 254-264. doi: 10.3934/Allergy.2024015 |
[4] | Katarzyna Nazimek . The complex functions of microRNA-150 in allergy, autoimmunity and immune tolerance. AIMS Allergy and Immunology, 2021, 5(4): 195-221. doi: 10.3934/Allergy.2021016 |
[5] | Ken S. Rosenthal, Daniel H. Zimmerman . J-LEAPS vaccines elicit antigen specific Th1 responses by promoting maturation of type 1 dendritic cells (DC1). AIMS Allergy and Immunology, 2017, 1(2): 89-100. doi: 10.3934/Allergy.2017.2.89 |
[6] | Chunyan Li, Wojciech Dawicki, Xiaobei Zhang, Chris Rudulier, John R. Gordon . IL-10- and retinoic acid-induced regulatory dendritic cells are therapeutically equivalent in mouse models of asthma and food allergy. AIMS Allergy and Immunology, 2021, 5(2): 73-91. doi: 10.3934/Allergy.2021007 |
[7] | Moufag Mohammed Saeed Tayeb . Role of IgG food test in patients with allergic diseases. AIMS Allergy and Immunology, 2023, 7(2): 154-163. doi: 10.3934/Allergy.2023010 |
[8] | Anastasiia D. Kurenkova, Peter S. Timashev . Mast cells: A dark horse in osteoarthritis treatment. AIMS Allergy and Immunology, 2022, 6(4): 228-247. doi: 10.3934/Allergy.2022017 |
[9] | Moufag Mohammed Saeed Tayeb . The relationship between mold sensitization and allergic diseases: a retrospective study (Jeddah, Saudi). AIMS Allergy and Immunology, 2020, 4(1): 14-19. doi: 10.3934/Allergy.2020002 |
[10] | McKenna S. Vininski, Sunanda Rajput, Nicholas J. Hobbs, Joseph J. Dolence . Understanding sex differences in the allergic immune response to food. AIMS Allergy and Immunology, 2022, 6(3): 90-105. doi: 10.3934/Allergy.2022009 |
Immune system disorders characterize various diseases such as multiple sclerosis (MS) and allergic diseases (AD). In MS, T-helper (Th)1 cell phenotype is responsible for the disease onset and long-term evolution. On the other hand, excessive Th2 cell activity has been demonstrated in AD. The simultaneous increase of MS and AD in the same geographical areas, observed in recent years, has questioned the mutually exclusive correlation between MS and AD immunopathogenesis supported by the Th1/Th2 paradigm and has moved the interest in understanding possible overlaps. This manuscript aims to discuss the literature, collected over the past two decades, about the association between MS and AD, and both experimental and epidemiological studies have been reviewed. The results do not provide a solid correlation between AD and MS, although experimental studies support the involvement of the same cells and molecules in the immunopathogenesis of both diseases. Further studies, increasing knowledge on the cellular and molecular mechanisms involved in these two disorders, could help to clarify if a positive or negative association links them and provide the possibility for the development of new therapies.
Multiple sclerosis (MS) and allergic diseases (AD) are both chronic diseases based on dysregulated immune responses against harmless antigens [1],[2] and have been conventionally considered to have mutually exclusive pathogenesis congruent with the T-helper (Th)1/Th2 paradigm [3].
Th1 and Th2 cells represent polarized forms of CD4+ Th cells that drive distinct branches of the immune system, which are “cellular immunity” and “humoral immunity” respectively [4],[5]. The Th1/Th2 ratio has a protective function for the host from pathogenic invasion and its balance has a regulatory effect on immune behavior. However, the uncontrolled activity of Th cells and the imbalance of Th1/Th2 ratio may cause various immune disorders, including autoimmune diseases, such as MS, and AD [6],[7]. In fact, focusing on the involvement of Th1/Th2 cells, evidence is accumulating that Th1 cells play an important role in the development of MS, promoting the upregulation of cell-mediated immune functions with the production of cytokines such as interferon (IFN)γ and tumor necrosis factor (TNF)α which increase inflammation, leading to disease progression and worsening of MS symptoms, whereas Th2 cells appear to modulate the tissue damage and contribute to disease remission through the secretion of anti-inflammatory cytokines including interleukin (IL)-4, IL-10, and IL-13 [7]–[10]. Instead, the uncontrolled humoral immunity promoted by Th2 lymphocytes is the protagonist of AD; indeed, with the production of characteristic cytokines such as IL-4, IL-5, IL-9, and IL-13, allergen-specific Th2 cells mediate inflammatory pathogenesis of allergic disorders supporting both immunoglobulin E (IgE) and eosinophil activity [11],[12]. On the other hand, in the field of AD, it is widely suggested that the shift towards Th1 responses has a protective role [4],[13],[14] (Figure 1).
The monocytic overproduction of IL-12 in MS patients compared to allergic patients has been proposed as a possible mechanism underlying the Th1/Th2 paradigm and the mutual exclusivity between MS and AD, being IL-12 a key Th1-inducing factor and a Th2-cytokines inhibitor. Therefore, the increased production of IL-12 may protect MS individuals from developing allergies but predispose them to autoimmune inflammation of the CNS and its exacerbation [14].
In the last few decades, the observation of a simultaneous increase of both immunological disorders in the same geographic areas [15] and the co-existence of autoimmune disorder and allergy in the same patient have moved the interest towards the search for possible correlations, that go beyond the biological plausibility based on the presence of common risk factors [16].
Identifying possible relationships between allergies and MS can help to better understand the immunological mechanisms underlying these pathologies and consequently to guide preventive and therapeutic approaches. Therefore, summarizing the previously published research on the topic, the aim of this manuscript is to verify a solid correlation, positive or negative, between the two immune diseases.
The search was conducted on PubMed using “allergy”, “allergic disease”, “multiple sclerosis”, “experimental autoimmune encephalomyelitis” and “allergy and multiple sclerosis” as key terms and filtering studies published from 2000 to date. Experimental research focused on the analysis of the molecular mechanisms shared between the two immune-mediated disorders was identified and reviewed. Clinical and epidemiological studies that evaluated the association between AD and MS were included. Additional research was carried out to frame both allergy and MS, using useful keywords to describe their epidemiology, etiology and immunopathogenesis.
After a general summary of the immune mechanisms characterizing MS and AD, the conflicting findings regarding the association between AD and MS were reported and discussed.
The MS is a chronic inflammatory and demyelinating disease of the central nervous system (CNS), based on the abnormal activation of the immune system against CNS autoantigens [17],[18], that predominantly affects young adults between the ages of 20 and 40, with a distribution ratio of women to men of approximately 3:1 [19],[20]. As result of the globally increased incidence of disease, MS affects the health of over 2.8 million people [21] and weighs heavily on the socio-economic sphere due to the high cost of available care and poor employment prospects for patients [22].
The autoimmune attack against self-antigens in the brain and spinal cord causes the characteristic “lesions” or “plaques” of MS, which are areas of myelin and oligodendrocytes destruction that can progress to neuroaxonal degeneration and tissue atrophy [19],[23]. Depending on brain areas affected and nerve transmission pathways compromised [24], the symptoms can affect the most varied skills, such as motor and coordination abilities, sensory capacity, sight, language and control of the viscera. Pain, fatigue and sexual disturbances are also often reported by MS patients [25], and the physical condition evolves to severe disability in about half of the patients [26]. Furthermore, the pathological changes in cortical brain regions justify the deterioration of cognitive functions such as memory, attention and abstract reasoning [27].
CNS lesions must be disseminated in space (DIS) and time (DIT) to proclaim a diagnosis of MS, through a clinical approach supported by radiological techniques of magnetic resonance imaging (MRI) [28] and laboratory findings such as the detection of cerebrospinal fluid-specific oligoclonal bands, which are all components of the recently revisited 2017 McDonald Criteria [29],[30].
Clinically, MS is distinguished in relapsing-remitting (RR), primary-progressive (PP), secondary-progressive (SP) and progressive-relapsing (PR), despite the high variability that characterizes clinical presentation and course of the disease [31]. Most patients initially present with RRMS, defined by acute episodes of neurological symptoms alternating with periods of remission. A variable percentage of these subjects (25–40%) develop a progressive accumulation of disability over time, with rare or absent relapses (SPMS). In 10–15% of patients, PPMS is diagnosed, in which the progressive accumulation of disability is present from the onset. Finally, in PRMS, progressive worsening of disability from the onset is accompanied by defined acute relapses, with or without complete recovery [32].
Considerable efforts are directed to understand the pathophysiology of MS disease to enrich the range of biomarkers able to describe MS phenotypes ever more clearly and earlier, in order to define a prognosis of the disease course in the individual patient [33] and direct treatment not only of symptoms and exacerbations, but also of disease progression with appropriate disease-modifying therapies (DMT) [34]. A greater clinical definition of MS phenotypes would benefit the correct design of clinical studies, ensuring the necessary recruitment of a homogeneous population in order to provide positive results [33]; similarly, it would benefit population studies that aim to define the profile of MS patients in relation to genetic, epigenetic, environmental and comorbid factors.
The exact etiology of MS remains unknown, but epidemiological studies have brought to light an extensive panel of predisposing factors to the disease, therefore collectively defined as multifactorial [35]. The genetic background represents about 1/3 of the total disease risk as evidenced by the higher frequency of MS within families, and the Genome-Wide Association Studies (GWAS) identified more than 100 genes loci associated with MS, including the HLA DR15/DQ6 variants of the Major Histocompatibility Complex (MHC), alleles of the IL-2 receptor alpha gene (IL2RA) and polymorphisms of IL-7 receptor alpha gene (IL7RA) [26],[32]. Other factors affecting the environment and lifestyle interact with genetic predisposition, increasing the risk of developing MS; compelling evidence has been reported for sun exposure and vitamin D levels, smoking, obesity, and Epstein-Barr virus (EBV) infection [20],[36].
Whatever the triggering cause, the onset of MS immunopathogenesis is the impaired immunological tolerance to autologous proteins [37]. Most of the current knowledge on the pathological mechanisms of MS comes from studies carried out on the animal model of experimental autoimmune encephalomyelitis (EAE), starting with the identification of the specific antigenic targets of the autoimmune response. In EAE, the disease is triggered by the animal's immunization with the two main myelin proteins such as myelin basic protein (MBP) and myelin proteolipid protein (PLP) in total or as peptide fragments [38],[39]; therefore, even in MS it is assumed that the autoimmune response is sustained against myelin antigens [40].
The first cells to acquire self-reactivity at the peripheral level are CD4+ T lymphocytes, whose polarization is directed towards the proinflammatory phenotypes Th1 and Th17 which have a predominant role in MS pathogenesis through the secretion of the respective cytokines such as IFNγ, TNFα and IL-2 for Th1, and IL-17 for Th17, whereas the regulatory function of the Th2 phenotype is disadvantaged [32]. The peripheral autoreactive lymphocytes express their pathogenic potential within the CNS transmigrating the blood-brain barrier (BBB) [41],[42], whose important physiological barrier function is altered in the pathological condition by the upregulated expression of adhesion molecules, chemokines, chemokine receptors and matrix metalloproteinases [43]–[45]. In the CNS parenchyma, the activation of lymphocytes is exacerbated by the encountering of myelin autoantigens, triggering a cascade of inflammatory mediators that chemoattract additional immune cells from the periphery, including T cells, B cells and monocytes readily activated to macrophages [32]. The immunological activity of all these cells leads to myelin damage and formation of the characteristic lesions of MS, scarred by the astrocytes [46]. In addition, the plaques of demyelination contribute to breaking the integrity of the BBB [47], facilitating the further infiltration of peripheral cells into the CNS in subsequent episodes of acute inflammation, defining the clinical condition of relapse [33]. The cytotoxic CD8+ T lymphocytes and phagocytic cells contribute to MS pathogenesis by a direct attack of neurons and oligodendrocytes [35]. Moreover, the role of B lymphocytes in MS has been revalidated in recent years; indeed, in addition to being cells producing autoantibodies against myelin structures, B cells also work as antigen-presenting cells (APC), as cytokine-secreting cells, and as cells aggregating in follicular-like structures between the meningeal spaces, defining a trapped inflammation together with chronically activated microglial and astrocytic cells [41],[48],[49]. The presence of persistent inflammation in the CNS, even in the absence of acute inflammation due to the migration of immune cells from the periphery [47], supports the chronicization of the damage, accompanied by the collapse of the mitochondrial energy system, the massive influx of calcium due to the deregulation of ion channels, the increase in oxidative stress, the massive excitotoxic effect mediated by glutamate produced by lymphocytes and microglia, the accumulation of iron, the loss of trophic support by oligodendrocytes, and the complement activation [47],[50],[51]. Activated microglia plays a central role in mediating many of these tissue damage mechanisms with highly proinflammatory behavior. Furthermore, the presence of microglia clusters is not limited to the areas of demyelination but has also been observed in the proximity of the plaques, constituting pre-active lesions that can transform into active demyelinating areas and contribute to the expansion of the damage [52]. The chronicization of brain inflammation causes the exacerbation of the damage up to neuro-axonal loss and the accumulation of irreversible clinical disability [53].
The AD are the most frequent forms of immunological diseases, affecting the health and quality of life of approximately 35% of the world population [54],[55], with high costs for pharmaceutical therapies and inpatient hospitalizations, and socio-economic consequences such as absence from work or reduced work efficiency of allergic people [56].
The term “atopy” is improperly used as a synonym for allergy; instead, atopic individuals have a strong genetic predisposition to the development of allergies, but not necessarily present the symptoms [57]. Allergy is defined as an immediate hypersensitivity reaction, due to the rapid manifestation at the vascular and muscle level of the excessive immunological activation against harmless exogenous antigens; however, the immediate reaction is followed by a late inflammatory response which promotes tissue damage, and most AD are linked to atopy [58],[59].
The AD are classically known as IgE-mediated immune responses to harmless foreign allergens [58], and at the heart of understanding how susceptible individuals develop IgE against certain environmental factors is knowledge of how the immune system recognizes and responds to offending agents. Two distinct phases drive the allergic response [60]. In the first phase (or sensitization phase), the dendritic cells (DC) capture the allergen at the site of entry and migrate to the lymph nodes, where present it to the naïve T-cell population and drive the differentiation of lymphocytes preferentially towards the Th2 phenotype. The Th2 secretion of specific cytokines such as IL-4, IL-5, IL-9 and IL-13, is responsible for switching B lymphocytes to produce IgE and for involvement of mast cells, basophils, and eosinophils [61],[62]. IgE play a central role in allergic inflammation, completing the sensitization phase and initiating the elicitation phase. In fact, the IgE antibodies produced bind high-affinity IgE receptor FcϵRI present on the surface of mast cells and basophils, thus made more sensitive and susceptible to a possible subsequent exposure to the specific antigen. The sensitization phase ends without manifestation of symptoms, with sensitized mast cells located in the connective tissue next to the antigen entry route [63],[64]. With subsequent exposure, the binding of IgE antibodies anchored to mast cells by the specific antigen is transduced by the FcϵRI receptor as a signal to activate these cells, pouring out the content of the granules into the surrounding environment [65],[66]; thus begins the elicitation phase. The preformed inflammatory mediators released with degranulation are mainly responsible for the immediate allergic manifestation, which will be almost local due to the fixed tissue localization of mast cells. The product most conspicuously secreted by mast cells degranulation is histamine, a vasoactive amine with a short half-life involved in vasodilation and increasing vascular permeability. Several preformed protease enzymes such as tryptase are also released, causing tissue damage by digesting the matrix. Mast cells do not participate in the allergic response only through the action of pre-stored substances, but also through the instant synthesis and production of other classes of molecules, such as lipid mediators (prostaglandins, leukotrienes, platelet-activating factors) and cytokines, which perpetuate the Th2 response. Lipid mediators are proinflammatory molecules derived from arachidonic acid that rapidly cause smooth muscle contraction and support the action of histamine on blood vessels. Therefore, in addition to causing the immediate manifestation of the allergic response, all these products of mast cells activation, including cytokine and chemokine environment, contribute to the onset of the late inflammatory phase by facilitating the recruitment of leukocyte cells, in particular basophils, eosinophils, neutrophils and Th2 cells [65],[67]. Basophils represent the circulating counterpart of mast cells and, when recalled to the inflammatory site, they too degranulate due to re-exposure to the allergen, releasing mediators like those of mast cells (histamine, eicosanoids, IL-4 and IL-13) [68]. Eosinophils are also an important cellular component of allergic reactions, whose activity is supported by IL-5 produced by Th2 lymphocytes and mast cells [69]. Eosinophil and neutrophil infiltrates strongly support tissue damage with a noticeable release of proteases, accompanied by a broad spectrum of reactive oxygen species, such as superoxide anion, hydrogen peroxide and nitric oxide, produced by neutrophils [70]. Thus, the clinical features of allergies mirror the activities of both resident cells and circulating leukocytes recruited to the site of exposure.
Allergic rhinitis, food allergies, bronchial asthma and anaphylaxis are the most common forms of allergy, each with typical pathological and clinical manifestations based on the route of entry of allergens and on the mediators mainly involved in the immune response. In susceptible individuals, allergic rhinitis is triggered by the inhalation of volatile allergens [71], to which the immune system responds excessively, causing nasal symptoms such as congestion, dripping and itching, often accompanied by allergic conjunctivitis, dry cough, alteration of the auditory tubes and chronic sinusitis [72]. Food allergies result from an abnormal immunological response to otherwise harmless food protein antigens and symptoms can be accompanied by allergic rhinitis, asthma and atopic dermatitis [73],[74]. In childhood, allergies to cow's milk, eggs, peanuts, tree nuts, soy and wheat are common; the first two usually resolve spontaneously at a later age, while the others are typically persistent in adults, in which allergies to fish and shellfish also often arise [74],[75]. However, oral allergic syndrome is the most frequent form of food allergy in adults, caused by allergens present in fruit and vegetables that trigger an allergic reaction of itching and swelling confined to the lips, mouth and throat [74]. Bronchial asthma is due to chronic inflammation of the airways induced by inhaled allergens. The bronchoconstriction and obstruction of the upper airways, that characterize this respiratory allergy, manifest themselves as dyspnea, wheezing, cough and chest tightness, accompanied by tachycardia [76]–[78]. The pathological condition can be exacerbated by factors other than inhalant allergens, such as cold, exercise, cigarette smoke and viral infections [79]. Finally, anaphylaxis is the most serious immediate hypersensitivity reaction and can be triggered by a multitude of factors including drugs, insect bites and foods. The manifestations of the anaphylactic reaction are urticaria, laryngeal edema and breathing difficulties, up to a systemic imbalance with a drop in blood pressure and risk of lethality [80],[81].
The exact etiology of immune system hypersensitivity to external antigens normally recognized as harmless is not known. It is classically accredited that the genetic background plays a fundamental role in allergy susceptibility, thanks to the identification through GWAS of hundreds of genes involved in the alteration of innate and adaptive immune functions [57]. Genes associated with the structure and functionality of epithelial and mucosal barriers have a critical role in supporting the inflammatory phase of the allergic response; for example, in various allergic forms a defective epithelial tissue, with incomplete tight junctions unable to counteract the penetration of allergens and their contact with resident immune cells, was found [82]. In respiratory allergies, the pathological condition is favored by the alteration of the goblet cells, whose overproduction of mucus causes obstruction of the airways [83] and persistent contact with inhaled allergens trapped in the mucus secretion. Furthermore, about the alteration of adaptive immunity, it is not surprising that certain polymorphisms of genes involved in the differentiation and effector functions of Th2 cells, as mediators of Th2 polarization pathway IL-4, IL4RA, signal transducer and activator of transcription 6 (STAT6) and GATA binding protein 3 (GATA3), are associated with a greater risk of allergy [84], being a Th2-mediated pathology.
In recent years, epidemiological studies have found an increase in the prevalence of allergies in developed and developing countries [85], leading researchers to reconsider the influence of environmental factors on the risk of allergic disorders onset; in fact, to date, the weight of the environmental impact in making an individual susceptible to allergic hypersensitivity reactions is considered the same as that of the genetic background [55]. Climate change and the high rate of atmospheric pollution are certainly among the most accredited risk factors for AD [86],[87]. Furthermore, when environmental factors lead to epigenetic modifications of the genes involved in the allergies' development, it is possible that they impact on the susceptibility not only of the individual but also of subsequent generations, since epigenetic regulations can be heritable. In this regard, the polarization of T lymphocyte subpopulations and the consequent differential secretion of inflammatory mediators are known among the immune functions regulated by epigenetics [88],[89].
The increase in allergies in Western countries is justified by the hygiene hypothesis according to which a lifestyle conducted mainly indoors and in excessively clean environments does not allow infants to properly develop the immune defenses, which are more easily directed against environmental antigens or self-antigens, resulting in allergic or autoimmune pathologies respectively [15],[90]. In fact, exposure to frequent infections in poor hygiene environments triggers a strong Th1/Th2 immune response, which is however effectively controlled and regulated by the cytokine IL-10 secreted by regulatory T lymphocytes, as well as by monocytes, macrophages and dendritic cells; on the other hand, in subjects poorly exposed to bacteria and parasites, this immunoregulatory function mainly mediated by Tregs is almost absent [91],[92]. Diet, obesity, the increase in cesarean sections and the use of antibiotics in the first years of life are also risk factors for the development of allergies in the West [90],[93],[94]. The study of the allergies incidence in immigrants from developing countries has highlighted the importance of environmental factors and lifestyle in predisposing to these immunological disorders. Immigrants show a lower prevalence of allergy than the native population, but interestingly the risk of AD increases with length of residence, younger age at migration and birth in the Western environment after parental migration. Therefore, it is likely that the allergies increase in developing regions is due to economic growth and the adoption of a Westernized lifestyle, and this trend will continue to grow [95],[96].
The observation of simultaneous increase of autoimmune diseases and allergies in the same geographical areas [15] has sparked interest in identifying a possible correlation between these immunological disorders that exceeds the oversimplification of Th1/Th2 paradigm.
The critical regulation of the immune response in both autoimmune and allergic inflammatory diseases can be genetically determined by polymorphisms or mutations in multiple genetic sites, influencing the development of Th1 or Th2 cytokines. Given the complexity of the immune response in the two pathological conditions, it might seem that the presence of MS (Th1-mediated) and AD (Th2-mediated) cannot co-exist in the same individual. From the literature, however, the co-existence of the two conditions has emerged and polymorphisms responsible for the Th1/Th2 pathways control are among the most plausible causes [97], although clear data are not yet reported.
Since MS is a highly widespread and disabling autoimmune-based CNS disease [98], many efforts have been devoted to find a correlation with allergic disorders, in order to highlight disease mechanisms and optimize MS clinical and therapeutic approaches. In addition, understanding earlier and better the MS patients' risk of disease progression and disability worsening reflects on the possibility of improving patients' quality of life. Indeed, previous studies have found that the quality of life in subjects with progressive MS forms is significantly lower than in patients with other forms of MS [99],[100]. The study of the correlation between MS and AD could bring out the latter as new predisposing or protective factors for MS, and thus open new clinical approaches to avoid the manifestation of the MS disease or to slow down its course improving the quality of life of people with MS. Nowadays, no clear relation have been identified, also for the high variability in the evaluation of the diseases co-presence.
Experimental studies have shown that immune mechanisms involved in allergic responses play an important role also in the immunopathogenesis of autoimmune demyelination (Table 1).
The study of Lock et al. showed that several genes encoding molecules traditionally associated with allergic responses are increased in MS lesions [101]. Pedotti et al. reported that elevated transcription levels of prostaglandin D synthase and tryptase were in the brain tissue of EAE mice. Furthermore, the expression of the histamine receptor 1 and 2 (H1R and H2R) was detected by immunohistochemical staining of inflammatory infiltrates in demyelinating lesions of EAE brain, with H1R mostly present on myelin-reactive Th1 lymphocytes and H2R expressed mainly on Th2 cells. In addition, the authors stated that the EAE course was counteracted by the pharmacological blockade of the H1R and the platelet-activating factor receptor (PAFR), mainly responsible for murine anaphylaxis, with their respective antagonists Pyrilamine and CV6209 [102]. However, engineered mice for total absence of endogenous histamine showed aggravated autoimmune disease in response to immunization with myelin protein; in fact, the production of proinflammatory cytokines IFNγ and TNFα was increased and CNS inflammatory infiltrates were more numerous compared to wild type (WT) histamine-producing mice [103]. In vitro studies of the action of histamine agonists for both H1R and H2R receptors on PLP-activated mouse T cells indicated that histamine inhibits the proliferation and production of IFNγ and reduces spontaneous and chemokine-induced adhesion of autoreactive T lymphocytes on intercellular adhesion molecule (ICAM)1, likely blocking their ability to transmigrate the inflamed BBB in vivo. Therefore, histamine appears as a complicated modulator of EAE pathogenesis and strategies able to interfere with the histamine axis could be applied in the therapy of CNS autoimmune diseases [104].
Reference | Type of study | Specimens | Main findings |
Pedotti R et al. (2003) [102] | Experimental study | EAE mice (brain tissue) | Increase in prostaglandin D synthase and tryptase; H1R-H2R expression; Reduction of EAE severity through: H1R and PAFR antagonists, FcγRIII and FcϵRI gene inhibition |
Musio S et al. (2006) [103] | Experimental study | EAE HDC−/− (histamine-deficient) mice vs EAE WT mice | Increase development of autoimmune disease in HDC−/− |
Lapilla M et al. (2011) [104] | Experimental study | PLP-activated mouse T cells | Inhibition of T cells proliferation, IFNγ production and adhesion on ICAM1, by histamine agonist |
Gregory GD et al. (2005) [105] | Experimental study | EAE W/Wv (mast cell-deficient) mice vs EAE WT mice | Worsening of disease by mast cells |
EAE: experimental autoimmune encephalomyelitis; FcϵRI: high-affinity IgE receptor; FcγRIII: Fc receptor for immune-complexed IgG; H1R: histamine receptor 1; H2R: histamine receptor 2; ICAM1: intercellular adhesion molecule 1; IFNγ: interferon γ; PAFR: platelet-activating factor receptor; PLP: myelin proteolipid protein; WT: wild type.
Furthermore, Pedotti's research highlighted that disruption of the genes encoding Fc receptor for immune-complexed IgG (FcγRIII) or both FcγRIII and FcϵRI decreases EAE [102]. All these results allow to affirm that molecules involved in the allergic response can potently modulate EAE and MS disease.
Gregory et al. focused their research on the involvement of mast cells, the key cellular component of the allergic response in EAE, in autoimmune disease mechanisms. The study showed that mast cells can contribute to the severity of EAE disease, inducing in the CNS the expression of cytokines, chemokines and proteases that promote the influx of autoreactive immune cells and myelin damage. Later it was shown that mast cells may also act in periphery, influencing T cells autoreactive response and disease exacerbation. Indeed, in genetically mast cells-deficient mice, the restoration of these cells without reaching an appreciable level in the CNS may led to a worsening of the EAE condition. Compared to WT EAE mice, reduced presence in peripheral T lymphocytes of the hallmark activation markers (such as Th1-specific IFNγ production and greater expression of cluster of differentiation CD44 and CD11a) and reduced lymph node hypertrophy were found in genetically mast cells-deficient EAE mice, as well as fewer autoreactive cells infiltrate in the CNS. The peripheral effects of immune stimulation by mast cells have been traced to direct but also indirect mechanisms on T lymphocytes, by controlling the cytokine production of DC. In the WT EAE mice, mast cells could support the key step of lymphocyte transmigration through the BBB by a histamine-induced increase of vascular permeability. The transfer of encephalitogenic T cells into WT and mutant mice also yielded interesting results, with a comparable onset time of EAE disease but lower severity in mutants, supporting the role of mast cells in altered inflammation both in the inductive peripheral phase of loss of tolerance than in the effector phase of immune attack in the CNS [105].
Thus, some experimental studies, conducted on the animal model of EAE, showed a powerful role of cells and molecules typical of the allergic immune response in the immunopathogenesis of the MS disease, overcoming the Th1/Th2 paradigm and opening new frontiers on potential treatment targets.
Many epidemiological studies have been done, with controversial results. The heterogeneity of the populations enrolled in the research conducted in the last two decades makes it difficult to define a clear and general correlation between AD and MS. In many of the population studies, information on allergy history was self-reported and investigated using digital questionnaires, face-to-face interviews or telephone interviews. Few of the reported research carry out an experimental evaluation of the allergic status of the subjects by laboratory assays for the determination of allergen-specific IgE. To minimize errors in the classification of the allergic status of the subjects, some studies included in the sample only subjects who reported physician-diagnosed allergies or not reported allergies, however risking an underestimation of the allergies prevalence in the population, excluding subjects who reported undiagnosed allergies because self-medicated. Contrary, more detailed clinical profiles were available for MS patients, carefully reported in most of the reviewed articles. The proportion in which the different forms of MS were represented in the enrolled samples is not always specified. Although a sample with a percentage representation of all subtypes of MS may be more representative of the general population and can be used to assess a possible correlation between allergies and MS onset risk, it is important to consider the different clinical courses of MS in assessing the existence of a positive or negative relationship between AD and the grade of MS activity, expressed as severity of symptoms and degree of disability of the patient. These reasons did not make it possible to divide the epidemiological studies reported into specific subcategories, making it necessary to summarize the salient points in a table that would provide a clear and general picture (Table 2).
In 2008 Alonso et al. enrolled 298 women diagnosed with MS and 1248 healthy controls. All subjects were interviewed through a mailed questionnaire on the personal history of any form of allergy, with associated clinical manifestations, and on family history of MS or other autoimmune diseases. The subjects were enrolled from the cohorts of Nurses' Health Study (NHS) I and II, to which many female nurses from different American states are registered and respond every two years to mailed questionnaires about lifestyle, habits and health conditions. Although a limitation of the study is to rely on self-reported information, the high degree of health literacy of the enrolled population consolidates its validity. Statistical analysis was performed considering potential confounding factors such as age or smoking habit. Results showed that personal history of allergy was not associated with MS risk, while autoimmune diseases pointed out familial aggregation and were associated with a higher MS risk, suggesting a mutual genetic background between them [106]. Later, by a case-control study nested in the UK-based General Practice Research Database cohort, 1523 controls and 163 MS incident cases were enrolled to investigate the history of allergy in the 3 years preceding the first symptoms of MS disease (index date) as predisposing factor to the autoimmune disease. Up to 10 controls matched to cases for age, gender, general practice and time in the cohort were selected, having at least 3 years of continuous recording in the database before the index date of their corresponding case. Keeping in mind that the H1R blockers improved the disease course in animal model of MS, also their use in the same reference time frame were assessed through computerized medical records. The results suggested a lack of association between history of allergic condition in the 3 years before the index date and MS risk, while the use of H1R blockers was associated with decreased MS risk. Health information regarding patients registered in this database (more than 3 million Britons) is considered highly reliable for epidemiological research, as it is recorded in a coded manner by selected general practitioners [107]. A case-control study was carried out in Iran to clarify the relationship of MS with allergy and atopy. In keeping with the sample size calculated to obtain statistically significant results, 40 patients with a diagnosis of MS established at most 2 years prior to the study and 40 controls, chosen from voluntary blood donors and matched to patient for age, sex and region of life, were enrolled. Patients with prior corticosteroid or immunomodulatory therapy were excluded from the study as confounding factors for the prevalence of allergy. Patients were diagnosed according to McDonald criteria and defined about clinical course of disease, initial symptoms and EDSS (Expanded Disability Status Scale). All patients were classified as RRMS and 89% of them had EDSS less than 3. Through a face-to-face interview, information was gathered about the personal and family history of allergy and related clinical manifestations in both groups. A serum sample was taken from each enrolled subject to measure total IgE and specific IgE against Weeds mix, Grass mix, Trees mix1, Trees mix2, Dermatophagoides farinae, Dermatophagoides Pteronyssinus and mix of epidermal and animal proteins. Subjects with a history of allergy and/or family history of allergy and/or increased serum total IgE levels were classified as atopic. No significant differences were found between the two groups regarding the history of allergy, the allergic status present at the time of the study, clinical manifestations, atopy, total serum IgE and frequency of specific IgE. Thus, in agreement with other studies, the authors supported no relation between MS and AD. A strength of the study was to collect the family history of MS and the history of vaccination, childhood infections and hygienic behavior in childhood as possible confounding factors known to impact on risk and/or course of MS [108]. Interestingly, in a large retrospective cohort study (1990–2018), a vast number of allergic subjects were enrolled to determine the incidence rate of autoimmune diseases: 782320 patients with allergic rhinitis/conjunctivitis, 1393570 with atopic eczema and 1049868 with asthma. Subjects' information came from the UK electronic database compiled by general practitioners for clinical and management purposes and, for each case, up to two non-allergic controls comparable for age and sex were matched. The results showed that several autoimmune disorders were associated with AD according to age- and sex-related clustering patterns and that many autoimmune diseases were uniformly more frequent in allergic subjects than in controls. A significantly higher long-term risk of autoimmune diseases, such as systemic lupus erythematosus and Sjögren's syndrome, was observed in patients with AD, while no significant effect of the three allergic disorders on the risk of MS was observed [109].
Contrary to the results obtained from previous studies, other case-control investigations have highlighted a positive correlation between allergic condition and MS, with AD classified as a risk factor for MS. The increase in the prevalence of MS in Iran, that shifted from a traditionally low-risk region to an area with the highest incidence of MS in the East, led Mansouri et al. to investigate which risk factors could justify the observed variation in disease distribution, in order to plan a prevention program as well as to clarify the underlying etiology of MS. Therefore, a multicenter case-control study on the Iranian population was performed with a large sample dimensionality, including 1403 patients diagnosed with MS and 883 controls, enrolled over a period of approximately five years. Among patients, all forms of MS progression at different stages of disease were included, the distribution ratio of 3:1 between women and men was respected, as well as the mean age of onset. Through a questionnaire submitted by a face-to-face interview, the two groups were screened for different individual, family and environmental factors. Information on reactions to different types of allergens and associated clinical manifestations was recorded. From data collected and adjusted for age and sex, the personal history of allergy was classified as a risk factor for susceptibility to MS (OR: 1.92, 95% CI: 1.55–2.47, p < 0.001) [110]. In addition, an Australian study identified the allergic condition as one of the most common and work-limiting comorbidities in patients with MS. The research was conducted on a sample of MS patients in working age, afferent to the Australian MS Longitudinal Study, about the presence of 30 comorbidities, to determine their weight on work performance and therefore on the costs of the population. The Australian MS Longitudinal Study includes approximately 3000 participants with MS of whom 96% were diagnosed with definite MS according to the McDonald criteria. The results showed that the allergic condition affected 36.2% of Australian patients and that subjects with this specific comorbidity had a greater average of days of absenteeism from work due to MS than that of individuals without comorbidities, resulting in a population annual cost for the loss of labor productivity of 20 million euros. Finally, the data collection on the severity degree of 13 MS symptoms made it possible to correlate the effects of comorbidities on work performance with the worsening of disease symptoms. The major strength of the study is its large nationally representative sample, proved by the similarity in MS phenotypes (70.5% RRMS; 12.9% SPMS; 5.9% PPMS; 1.4% PRMS; 9.2% unsure) and sex (80.6% females), compared with other MS natural history cohort studies. In conclusion, this study revealed the importance of monitoring comorbidities, including allergies, to improve course of MS disease and therefore productivity at work [111]. On patients belonging to the same Australian MS Longitudinal Study, a preliminary investigation was conducted by Lo and colleagues to identify the most prevalent comorbidities in MS patients. Allergies showed a prevalence of 37.4% as self-reported disorder and a prevalence of 28.6% as comorbidity diagnosed by doctors. Among the five comorbidity patterns identified according to the types and number of comorbidities that coexisted in patients, allergies belonged to the “non-metabolic” and “mental health-allergy” classes, which together accounted for 29.3% of the cohort of patients examined. While the characteristics of sex, MS duration, body mass index and disability influenced belonging to certain classes of comorbidity compared to others, conversely, DMT status, MS onset type (relapse or progressive), education attainment, and relative socioeconomic status were not associated with belonging to any comorbidity class. Although a causal relationship between the pathologies examined was not assessed, this study also helped provide a broader picture of the pathologies to be observed and treated in MS patients [112].
Other authors have contributed to the literature on comorbidities represented in MS patients and which affect the individual's daily functions, in order to understand, prevent and manage them as an integral part of patient care. To characterize the frequency of asthma in MS patients, a cross-sectional study was conducted using electronic health record information of 56.6 million Americans from 50 U.S. states. The subjects enrolled belonged to two cohorts: 141880 patients with MS and 56416790 individuals without a diagnosis of MS. This retrospective study revealed a significantly higher prevalence of asthma in MS patients than in individuals without an MS diagnosis, matched by age, gender, and racial subpopulations. Furthermore, in MS patients, asthma was more common among the young and the elderly, while a uniform age distribution was observed in the general population [113]. In a cross-sectional study conducted on 2012 RRMS patients, the presence of hypertension, hyperlipidemia and asthma was analyzed, and patients were characterized for age, sex, race, MS duration, body mass index, smoking habit, treatment with DMT, and other comorbidities. Results showed that RRMS patients burdened by asthma were women, obese, hypertensive, with a medium/low income and less likely treated with interferons or glatiramer acetate as DMT. The authors suggest that there may be additional unique differences in the specific subgroups of MS patients that need further study for a more exhaustive characterization [114].
Therefore, these results contrast with the hypothesis of an inverse relationship between the two diseases based on the Th1/Th2 paradigm, instead supported by other studies. Tremlett et al. investigated if MS patients have a reduced risk of allergy. The evidence of a lower frequency of asthma in MS patients compared to controls emerged from their retrospective case-control study conducted on 320 MS patients and 320 controls, matched for age, gender, residence and smoking habits, and selected from the Welsh General Practice Morbidity Database from 1995–1999 [115]. A similar study was also conducted, in northern Italy, by Bergamaschi's team looking for correlations between allergic respiratory diseases (ARD) and the development of MS. Results underlined that respiratory allergic conditions were less prevalent in MS patients. No relevant difference was found for the age at MS onset, the disease duration, the disease clinical course or the use of immune therapies, comparing “MS plus ARD” patients with “MS alone” patients; moreover, based on the mean scores of EDSS and MSSS (Multiple Sclerosis Severity Score), in coexistence with ARD, the MS disease appeared less severe than in “MS alone” patients, although the differences found did not reach statistical significance. Thus, the authors proposed that other MS cohorts must be evaluated to confirm these results and to investigate if MS patients affected by AD show specific clinical profiles [116]. Sahraian et al. referred that, in an equal number of patients and controls (195 subjects per group) questioned face-to-face about the presence of different types of allergies such as respiratory tract allergy (RTA), coetaneous allergy (CA) and food/drug allergy (FDA), a lower risk of MS diagnosis was found in subjects affected by RTA or FDA, whose protective role against the onset of autoimmune demyelinating disease is enhanced if co-present in the same subject. Furthermore, by comparing progressive MS forms with the less severe form of RRMS, in relation to the presence of above-mentioned allergies, a negative correlation also between allergic disorders and MS clinical course was found. In fact, more RRMS patients had past medical history of allergy than the patients in progressive phases (57.4% vs 36.7%; p = 0.04). This capability of atopic allergy in prediction of less disabling forms of MS is another interesting finding of the study, that deserves further investigation [117]. In the research conducted by Pedotti, the interview on personal allergic history submitted by telephone allowed the enrollment of a greater number of subjects (423 cases and 643 population controls). The investigation covered the history of allergic asthma, rhinitis and conjunctivitis due to inhalant allergens, atopic dermatitis and atopic eczema, contact dermatitis, food allergy, drug allergy and allergy to insect sting or bite; as a whole, atopic allergies were associated with a reduced risk of MS, with greater significance for the asthmatic subtype (p < 0.01) [118]. The protective role of allergies for MS onset was also suggested in the article published by Ren et al. based on results obtained from 829 MS patients and 2441 controls of American population (clinical information collected in the National Ambulatory Medical Care Survey database from 2006 to 2013) [119]. However, a correlation study between autoimmune diseases and AD by the detection of specific IgE against inhalant allergens showed that the Hazard ratio estimated by Cox regression does not prove a statistically significant association between atopy and MS (HR = 1.97, 95% CI: 0.95, 4.11) as well as with other autoimmune diseases such as Crohn's disease or psoriasis vulgar [120].
These results are in contrast with findings of Manouchehrinia et al. research, performed on England population to verify if asthma comorbidity is related to an increase in MS-related injury and disability. No difference in the prevalence of asthma between patients with MS and subjects without MS disease was detected. Furthermore, the study of asthma associated with a possible accumulation of increased disability evaluated as EDSS score also did not lead to significant results. Indeed, after controlling for sex, age and smoking status at the MS onset, initial clinical course of the disease and treatment with DMT, MS patients with asthma were not at higher risk of achieving a more severe EDSS score than those without asthma. However, there was a worsening of psychological conditions in asthmatic MS patients [121]. In the past, the association between asthma and MS had already been studied by Australian research, in relation to family structure. The rationale for this research was based on the accepted hygiene hypothesis that cohabitation with siblings in early childhood exposes to an infectious burden that trains the immune system in Th2 responses, reducing the risk of developing Th2-related disorders, such as asthma. Conversely, the beneficial effect of such exposure on Th1 disorders, such as MS, is less evident. The case-control study, conducted on 136 confirmed MS cases and 272 controls, showed that MS patients were more likely to develop asthma than controls and that the AD appeared before the onset of MS symptoms. As expected, this association between asthma and MS was strengthened in subjects with reduced sibling exposure, while it was reduced in individuals who had cohabited with younger siblings during childhood [122]. In the following decade, a multicenter case-control study conducted on adults with a first clinical diagnosis of central nervous system demyelination (FCD) gave conflicting results. Compared to controls, personal or family allergy was not over-represented in FCD patients. Evaluation of the course of FCD autoimmune disease and of asthma as an allergic disease showed that sibship characteristics influence differently both FCD and asthma risk. In fact, in contrast to the conclusions of Ponsonby et al., exposure to younger siblings reduced the risk of FCD but not asthma; conversely, cohabitation with older siblings decreased the probability of asthma but not of FCD diagnosis. Therefore, this research has highlighted the impact of the siblinghood characteristics on both autoimmune and allergic disorders, although with different mechanisms not yet fully clarified [123].
With their increased incidence over the past three decades [124], food allergies also reached a great interest in the relationship with MS development. The most impacting food allergy, in childhood, is the cow's milk allergy (CMA), one of the possible causes of vitamin D deficiency [125], which is an accredited risk factor for MS [126]. Ramagopalan et al. found no differences in the frequency of CMA in 6638 MS cases (71.9% RRMS) and 2509 consort controls extrapolated from the Canadian Collaborative Project on Genetic Susceptibility to MS, which stores personal and family information of patients belonging to all Canadian clinics by submitting standardized questionnaires [127]. Vitamin D has shown the ability to inhibit both Th1 and Th2 immune responses, by suppressing the production of IL-12-generated IFN-γ as well as IL-4 and IL-4-induced expression of IL-13 [128]. Furthermore, vitamin D exhibited the ability to enhance the development of IL-10-producing Treg cells [126], that play an important role in maintaining immune homeostasis [129]. In the field of AD, effects of vitamin D deficiency in their development, severity and course are known. The IL-10 inhibits inappropriate allergen-specific Th2-driven immune responses and regulates allergic sensitization [130], while Treg cell deficiencies are considered among the possible mechanisms underlying impaired self-tolerance in MS pathogenesis [131]. How changes in vitamin D levels can affect the balance of the immune response and can help to deepen the relationship between AD and MS needs to be better investigated.
To evaluate the association of MS with food allergies to cow's milk, eggs and fish, Ashtari et al. recruited 96 individuals, equally distributed between MS patients (n.48) and healthy controls (n.48), with comparable mean age and with a ratio between men and women reflecting the higher prevalence of MS in women. The specific serum IgE were measured for the allergens investigated and were not found in any subjects enrolled. Therefore, with zero frequency of these food allergies in both groups, the authors concluded an unlikely influence on MS susceptibility, although to have analyzed allergies to cow's milk and eggs which are known to resolve spontaneously in most adults is a limitation of the study [132],[133]. Consistently, a different prevalence of food allergies in MS and in healthy controls was not found in a sample of the U.S. pediatric population enrolled by Bourne and colleagues [134]. However, an effect of allergy was observed in relation to MS disease activity, and they reported fewer relapses in MS patients with food allergic disorders than in non-allergic MS patients, suggesting that allergic Th2-imbalance may be a negative predictor of relapse and therefore a protective factor. The personal allergy history referred to the first five years of life and the relapse rate for patients with follow-up controls was normalized based on parameters of age of MS onset, sex, race, ethnicity and treatment with DMT [134]. Recently, Fakih et al. turned the picture on the relationship between food allergies and MS. These researchers looked for a correlation between self-reported history of allergic conditions and MS course, described by clinical parameters like attacks numbers, EDSS and MSSS, and radiological MRI-assessed disease activity and lesion load. Based on the type of allergy reported at the time of the questionnaire, 1349 patients enrolled by the Comprehensive Longitudinal Investigation of Multiple Sclerosis at the Brigham and Women's Hospital (CLIMB) study were divided into four groups: (1) environmental allergic, (2) drugs allergic, (3) food allergic, and (4) non-allergic subjects. Evidence of a higher number of attacks and increased radiological disease activity in MS patients with food allergy has led to support a worsening effect of this disorder on the MS course. Multivariable analyses were adjusted for sex, age at symptom onset, disease category (progressive vs relapsing), percentage of time on DMT, and disease duration [135]. Albatineh and colleagues did not find the same negative role of food allergies, while identified education level and physical activity as factors to look out for to improve the quality of life of MS patients, as associated with a reduced MSSS and therefore with a less severe clinical course of MS. Of the 128 patients with MS included in the study, nearly all (96.9%) had RRMS [136]. Therefore, to date, the link between food allergies and MS onset or activity is unclear. Research by Fakih et al., which reported more relapses in MS patients who have food (and not other types) allergies [135], could better clarify the correlation between AD and MS, if supported by further research. Indeed, food allergies drive the modification of the intestinal microbiome that, by modulating the immune system, could alter BBB integrity and function, interact with different cell types present in CNS and trigger autoimmune demyelination [137]. Thus, gut inflammation and intestinal bacterial flora alteration, caused by the extensive variability between allergens contained in food, could be factors responsible for a greater susceptibility to the development of MS, in accordance with the presence of the gut-brain axis [138].
Reference | Type of study | Study group | Allergies/Symptoms | Main findings |
Alonso A et al. (2008) [106] | Case-control study (questionnaire on allergy history) | US population (298 MS women and 1248 controls) | Pollens, house dust, animal dander, foods, drugs, other | No correlation allergy-MS |
Alonso A et al. (2006) [107] | Case-control study (medical records on allergy history and H1R antagonist use) | UK population (163 MS and 1523 controls) | Rhinitis/hay fever, asthma, urticaria/angioedema, eczema/atopic dermatitis, other | No correlation allergy-MS; negative correlation H1R antagonist-MS |
Karimi P et al. (2013) [108] | Case-control study (questionnaire on allergy history and IgE measure on serum sample) | Iranian population (40 MS and 40 controls) | Conjunctivitis, rhinitis, urticaria and eczema, asthma; plant and animal allergies | No differences in allergy history and IgE levels between MS and controls |
Krishna MT et al. (2019) [109] | Retrospective cohort study (database on autoimmune disorders) | UK population (782320 allergic rhinitis/conjunctivitis, 1393570 atopic eczema, 1049868 asthma patients) | Rhinitis/conjunctivitis, eczema, asthma | No correlation allergy-MS |
Mansouri B et al. (2014) [110] | Multicenter case-control study (questionnaire on environmental and familial factors) | Iranian population (1403 MS and 883 controls) | Foods, drugs, pollens, house dust, animal dander, other | Positive correlation allergy-MS |
Chen J et al. (2020) [111] | Cohort study (questionnaire on comorbidities and employment outcomes) | Australian population (929 MS working-aged) | Not reported | Positive correlation allergy-MS; worsening of MS symptoms; reduction of work performance; increase of annual costs |
Lo LMP et al. (2021) [112] | Cohort study (medical records on comorbidities) | Australian population (1518 MS) | Not reported | No correlation allergy-MS; allergy comorbidity (37.4% self-reported, 28.6% diagnosed) |
Hill E at al. (2019) [113] | Retrospective study (records on asthma) | US population (141880 MS and 56416790 controls) | Asthma | Asthma prevalence among young and elderly MS |
Sorensen A et al. (2021) [114] | Cross-sectional study (medical records on asthma and other comorbidities) | US population (2012 RRMS) | Asthma | Characterization of asthmatic RRMS patients: women, obese, hypertensive; with a medium/low income; no treated with DMT |
Tremlett HL et al. (2002) [115] | Retrospective case-control study (medical records on asthma, eczema and dermatitis) | Wales population (320 MS and 320 controls) | Asthma | Asthma lower prevalence among MS |
Bergamaschi R et al. (2009) [116] | Case-control study (questionnaire on ARD) | Italian population (200 MS and 200 controls) | ARD | Negative correlation allergy-MS; less MS severity in coexistence with ARD |
Sahraian MA et al. (2013) [117] | Case-control study (questionnaire on RTA, CA and FDA) | Iranian population (195 MS and 195 controls) | RTA, CA and FDA | RTA and FDA protective role against MS onset (negative correlation) |
Pedotti R et al. (2009) [118] | Case-control study (questionnaire on allergy history) | Italian population (423 MS and 643 controls) | Allergic asthma, rhinitis, conjunctivitis, atopic dermatitis and eczema, contact dermatitis, food, drug and insect allergies | Negative correlation atopic allergies-MS |
Ren J et al. (2017) [119] | Case-control study (database on AD) | American population (829 MS and 2441 controls) | Respiratory tract allergies and other | Protective role of allergies for MS onset (negative correlation) |
Skaaby T et al. (2015) [120] | Cohort study (detection of allergy specific IgE) | Danish population (14849 persons including 834 autoimmune diseases with 35 MS) | Respiratory allergens | No correlation allergy-MS |
Manouchehrinia A et al. (2015) [121] | Cohort study (questionnaire on asthma and MS grade) | England population (680 MS) | Asthma | No correlation allergy-MS; worsening of psychological conditions in asthmatic MS |
Ponsonby AL et al. (2006) [122] | Case-control study (questionnaire on asthma and sibling exposure) | Australian population (136 MS and 272 controls) | Asthma | Positive correlation MS-allergy (strengthened in MS with reduced sibling exposure) |
Hughes AM et al. (2013) [123] | Multicenter case-control study (questionnaire on comorbidities and sibship structure) | Australian population (282 FCD adults and 588 control) | Asthma, hayfever, eczema | No correlation allergy-FCD; exposure to younger siblings reduced FCD but not asthma risk |
Ramagopalan SV et al. (2010) [127] | Cohort study (questionnaire on CMA) | Canadian population (6638 MS and 2509 controls) | CMA | No correlation allergy-MS |
Ashtari F et al. (2013) [132],[133] | Case-control study (detection of allergy specific IgE) | Iranian population (48 MS and 48 controls) | Cow's milk, eggs and fish allergies | No correlation allergy-MS |
Bourne T et al. (2017) [134] | Case-control study (questionnaire on allergy history) | US pediatric population (271 MS and 418 controls) | Environmental and food allergies | No correlation environmental allergy-MS; negative correlation food allergy-MS activity |
Fakih R et al. (2019) [135] | Cohort study (questionnaire on allergy history) | US population (1349 MS) | Environmental, food and drug allergies | Positive correlation food allergy-MS activity (worsening effect) |
Albatineh AN et al. (2020) [136] | Cross-sectional study (questionnaire on lifestyle habits) | Kuwaiti population (128 MS) | Food allergy | No correlation allergy-MSSS |
AD: allergic diseases; ARD: allergic respiratory diseases; CA: coetaneous allergy; CMA: cow's milk allergy; DMT: disease-modifying therapies; FCD: first clinical diagnosis of central nervous system demyelination; FDA: food/drug allergy; H1R: histamine receptor 1; IgE: immunoglobulin E; MS: multiple sclerosis; MSSS: MS severity score; RRMS: relapsing-remitting MS; RTA: respiratory tract allergy.
We have summarized the strengths and limitations of experimental and epidemiological studies that have tried to understand the association between AD and MS. Based on Th1/Th2 paradigm, a protective effect of Th2-mediated disorders on MS was proposed. On the other hand, population studies that evaluated link between AD (including environmental, food and drug allergies) and MS did not provide convincing evidence. Given the variability of epidemiological data accumulated over the past two decades due to several factors (heterogeneous populations, sample size, self-reported information, others), it is not surprising that a conclusive cause and effect relationship between AD and MS has not been established yet. However, results of the experimental studies suggest that the main actors of allergic response are strongly involved in MS immunopathogenesis, although without the identification of a solid positive or negative correlation between the two immune disorders.
In the near future, new generations of transcriptomic and proteomic techniques will be used in the study of a cell landscape in allergic and autoimmune conditions to improve the understanding of cell molecular phenotype and regulatory molecules activity. The comparison of these data in AD and MS could pave the way for the identification of common pathways and genetic/epigenetic regulatory mechanisms, allowing to clearly establish the mutual risk and to develop new therapies and diagnostic biomarkers. Early diagnosis of MS determines a higher likelihood of recovery than those diagnosed at a later stage, with the control of disease symptoms and possible comorbidities such as AD, slowing the disability and preventing the exacerbations associated with the disease progression.
[1] |
Akdis M, Akdis CA (2014) Mechanisms of allergen-specific immunotherapy: multiple suppressor factors at work in immune tolerance to allergens. J Allergy Clin Immunol 133: 621-631. https://doi.org/10.1016/j.jaci.2013.12.1088 ![]() |
[2] |
Buckley CD, McGettrick HM (2018) Leukocyte trafficking between stromal compartments: lessons from rheumatoid arthritis. Nat Rev Rheumatol 14: 476-487. https://doi.org/10.1038/s41584-018-0042-4 ![]() |
[3] | Kidd P (2003) Th1/Th2 balance: the hypothesis, its limitations, and implications for health and disease. Altern Med Rev 8: 223-246. |
[4] |
Romagnani S (2004) Immunologic influences on allergy and the TH1/TH2 balance. J Allergy Clin Immunol 113: 395-400. https://doi.org/10.1016/j.jaci.2003.11.025 ![]() |
[5] |
Lee GR, Kim ST, Spilianakis CG, et al. (2006) T helper cell differentiation: regulation by cis elements and epigenetics. Immunity 24: 369-379. https://doi.org/10.1016/j.immuni.2006.03.007 ![]() |
[6] |
Matsuzaki J, Tsuji T, Imazeki I, et al. (2005) Immunosteroid as a regulator for Th1/Th2 balance: its possible role in autoimmune diseases. Autoimmunity 38: 369-375. https://doi.org/10.1080/08916930500124122 ![]() |
[7] |
Ngoc PL, Gold DR, Tzianabos AO, et al. (2005) Cytokines, allergy, and asthma. Curr Opin Allergy Clin Immunol 5: 161-166. https://doi.org/10.1097/01.all.0000162309.97480.45 ![]() |
[8] |
Podbielska M, Banik NL, Kurowska E, et al. (2013) Myelin recovery in multiple sclerosis: the challenge of remyelination. Brain Sci 3: 1282-1324. https://doi.org/10.3390/brainsci3031282 ![]() |
[9] |
Arellano G, Acuña E, Reyes LI, et al. (2017) Th1 and Th17 cells and associated cytokines discriminate among clinically isolated syndrome and multiple sclerosis phenotypes. Front Immunol 8: 753. https://doi.org/10.3389/fimmu.2017.00753 ![]() |
[10] |
Wang K, Song F, Fernandez-Escobar A, et al. (2018) The properties of cytokines in multiple sclerosis: pros and cons. Am J Med Sci 356: 552-560. https://doi.org/10.1016/j.amjms.2018.08.018 ![]() |
[11] |
Hirahara K, Nakayama T (2016) CD4+ T-cell subsets in inflammatory diseases: beyond the Th1/Th2 paradigm. Int Immunol 28: 163-171. https://doi.org/10.1093/intimm/dxw006 ![]() |
[12] |
Wambre E, Bajzik V, DeLong JH, et al. (2017) A phenotypically and functionally distinct human TH2 cell subpopulation is associated with allergic disorders. Sci Transl Med 9: eaam9171. https://doi.org/10.1126/scitranslmed.aam9171 ![]() |
[13] |
Tang C, Inman MD, van Rooijen N, et al. (2001) Th type 1-stimulating activity of lung macrophages inhibits Th2-mediated allergic airway inflammation by an IFN-gamma-dependent mechanism. J Immunol 166: 1471-1481. https://doi.org/10.4049/jimmunol.166.3.1471 ![]() |
[14] |
Tang L, Benjaponpitak S, DeKruyff RH, et al. (1998) Reduced prevalence of allergic disease in patients with multiple sclerosis is associated with enhanced IL-12 production. J Allergy Clin Immunol 102: 428-435. https://doi.org/10.1016/S0091-6749(98)70131-9 ![]() |
[15] |
Okada H, Kuhn C, Feillet H, et al. (2010) The “hygiene hypothesis” for autoimmune and allergic diseases: an update. Clin Exp Immunol 160: 1-9. https://doi.org/10.1111/j.1365-2249.2010.04139.x ![]() |
[16] |
Bach JF (2002) The effect of infections on susceptibility to autoimmune and allergic diseases. N Engl J Med 347: 911-920. https://doi.org/10.1056/NEJMra020100 ![]() |
[17] |
Cunniffe N, Coles A (2021) Promoting remyelination in multiple sclerosis. J Neurol 268: 30-44. https://doi.org/10.1007/s00415-019-09421-x ![]() |
[18] | Lazibat I, Rubinić-Majdak M, Županić S (2018) Multiple sclerosis: new aspects of immunopathogenesis. Acta Clin Croat 57: 352-361. https://doi.org/10.20471/acc.2018.57.02.17 |
[19] |
Yamout B, Alroughani R (2018) Multiple sclerosis. Semin Neurol 38: 212-225. https://doi.org/10.1055/s-0038-1649502 ![]() |
[20] |
Dobson R, Giovannoni G (2019) Multiple sclerosis—a review. Eur J Neurol 26: 27-40. https://doi.org/10.1111/ene.13819 ![]() |
[21] |
Walton C, King R, Rechtman L, et al. (2020) Rising prevalence of multiple sclerosis worldwide: Insights from the Atlas of MS, third edition. Mult Scler 26: 1816-1821. https://doi.org/10.1177/1352458520970841 ![]() |
[22] |
Filippi M, Bar-Or A, Piehl F, et al. (2018) Multiple sclerosis. Nat Rev Dis Primers 4: 43. https://doi.org/10.1038/s41572-018-0041-4 ![]() |
[23] |
Lassmann H, Brück W, Lucchinetti CF (2007) The immunopathology of multiple sclerosis: an overview. Brain Pathol 17: 210-218. https://doi.org/10.1111/j.1750-3639.2007.00064.x ![]() |
[24] |
Kearney H, Altmann DR, Samson RS, et al. (2015) Cervical cord lesion load is associated with disability independently from atrophy in MS. Neurology 84: 367-373. https://doi.org/10.1212/WNL.0000000000001186 ![]() |
[25] |
Schiess N, Calabresi PA (2016) Multiple sclerosis. Semin Neurol 36: 350-356. https://doi.org/10.1055/s-0036-1585456 ![]() |
[26] |
Dendrou CA, Fugger L, Friese MA (2015) Immunopathology of multiple sclerosis. Nat Rev Immunol 15: 545-558. https://doi.org/10.1038/nri3871 ![]() |
[27] |
Amato MP, Ponziani G, Siracusa G, et al. (2001) Cognitive dysfunction in early-onset multiple sclerosis: a reappraisal after 10 years. Arch Neurol-Chicago 58: 1602-1606. https://doi.org/10.1001/archneur.58.10.1602 ![]() |
[28] |
Oh J, Vidal-Jordana A, Montalban X (2018) Multiple sclerosis: clinical aspects. Curr Opin Neurol 31: 752-759. https://doi.org/10.1097/WCO.0000000000000622 ![]() |
[29] |
McGinley MP, Goldschmidt CH, Rae-Grant AD (2021) Diagnosis and treatment of multiple sclerosis: a review. JAMA 325: 765-779. https://doi.org/10.1001/jama.2020.26858 ![]() |
[30] |
Przybek J, Gniatkowska I, Mirowska-Guzel D, et al. (2015) Evolution of diagnostic criteria for multiple sclerosis. Neurol Neurochir Pol 49: 313-321. https://doi.org/10.1016/j.pjnns.2015.07.006 ![]() |
[31] |
Milo R, Miller A (2014) Revised diagnostic criteria of multiple sclerosis. Autoimmun Rev 13: 518-524. https://doi.org/10.1016/j.autrev.2014.01.012 ![]() |
[32] |
Garg N, Smith TW (2015) An update on immunopathogenesis, diagnosis, and treatment of multiple sclerosis. Brain Behav 5: e00362. https://doi.org/10.1002/brb3.362 ![]() |
[33] |
Klineova S, Lublin FD (2018) Clinical course of multiple sclerosis. Cold Spring Harb Perspect Med 8: a028928. https://doi.org/10.1101/cshperspect.a028928 ![]() |
[34] | Hart FM, Bainbridge J (2016) Current and emerging treatment of multiple sclerosis. Am J Manag Care 22: s159-s170. |
[35] |
Baecher-Allan C, Kaskow BJ, Weiner HL (2018) Multiple sclerosis: mechanisms and immunotherapy. Neuron 97: 742-768. https://doi.org/10.1016/j.neuron.2018.01.021 ![]() |
[36] |
Alfredsson L, Olsson T (2019) Lifestyle and environmental factors in multiple sclerosis. Cold Spring Harb Perspect Med 9: a028944. https://doi.org/10.1101/cshperspect.a028944 ![]() |
[37] | Selter RC, Hemmer B (2013) Update on immunopathogenesis and immunotherapy in multiple sclerosis. ImmunoTargets Ther 2: 21-30. https://doi.org/10.2147/ITT.S31813 |
[38] |
Baxter AG (2007) The origin and application of experimental autoimmune encephalomyelitis. Nat Rev Immunol 7: 904-912. https://doi.org/10.1038/nri2190 ![]() |
[39] |
Kuerten S, Lichtenegger FS, Faas S, et al. (2006) MBP-PLP fusion protein-induced EAE in C57BL/6 mice. J Neuroimmunol 177: 99-111. https://doi.org/10.1016/j.jneuroim.2006.03.021 ![]() |
[40] |
Derdelinckx J, Cras P, Berneman ZN, et al. (2021) Antigen-specific treatment modalities in MS: The past, the present, and the future. Front Immunol 12: 624685. https://doi.org/10.3389/fimmu.2021.624685 ![]() |
[41] |
D'Angelo C, Reale M, Costantini E, et al. (2018) Profiling of canonical and non-traditional cytokine levels in interferon-β-treated relapsing-remitting-multiple sclerosis patients. Front Immunol 9: 1240. https://doi.org/10.3389/fimmu.2018.01240 ![]() |
[42] |
Marchetti L, Engelhardt B (2020) Immune cell trafficking across the blood-brain barrier in the absence and presence of neuroinflammation. Vasc Biol 2: H1-H18. https://doi.org/10.1530/VB-19-0033 ![]() |
[43] |
Gold R, Wolinsky JS (2011) Pathophysiology of multiple sclerosis and the place of teriflunomide. Acta Neurol Scand 124: 75-84. https://doi.org/10.1111/j.1600-0404.2010.01444.x ![]() |
[44] |
Holman DW, Klein RS, Ransohoff RM (2011) The blood-brain barrier, chemokines and multiple sclerosis. Biochim Biophys Acta 1812: 220-230. https://doi.org/10.1016/j.bbadis.2010.07.019 ![]() |
[45] |
Sonar SA, Lal G (2018) Blood-brain barrier and its function during inflammation and autoimmunity. J Leukocyte Biol 103: 839-853. https://doi.org/10.1002/JLB.1RU1117-428R ![]() |
[46] |
Thompson AJ, Baranzini SE, Geurts J, et al. (2018) Multiple sclerosis. Lancet 391: 1622-1636. https://doi.org/10.1016/S0140-6736(18)30481-1 ![]() |
[47] |
Piehl F (2021) Current and emerging disease-modulatory therapies and treatment targets for multiple sclerosis. J Intern Med 289: 771-791. https://doi.org/10.1111/joim.13215 ![]() |
[48] |
Claes N, Fraussen J, Stinissen P, et al. (2015) B cells are multifunctional players in multiple sclerosis pathogenesis: Insights from therapeutic interventions. Front Immunol 6: 642. https://doi.org/10.3389/fimmu.2015.00642 ![]() |
[49] |
Milo R (2019) Therapies for multiple sclerosis targeting B cells. Croat Med J 60: 87-98. https://doi.org/10.3325/cmj.2019.60.87 ![]() |
[50] |
McFarland HF, Martin R (2007) Multiple sclerosis: a complicated picture of autoimmunity. Nat Immunol 8: 913-919. https://doi.org/10.1038/ni1507 ![]() |
[51] | Inglese M (2006) Multiple sclerosis: new insights and trends. Am J Neuroradiol 27: 954-957. |
[52] |
Voet S, Prinz M, van Loo G (2019) Microglia in central nervous system inflammation and multiple sclerosis pathology. Trends Mol Med 25: 112-123. https://doi.org/10.1016/j.molmed.2018.11.005 ![]() |
[53] |
Strik M, Cofré Lizama LE, Shanahan CJ, et al. (2021) Axonal loss in major sensorimotor tracts is associated with impaired motor performance in minimally disabled multiple sclerosis patients. Brain Commun 3: fcab032. https://doi.org/10.1093/braincomms/fcab032 ![]() |
[54] |
Akdis CA (2006) Allergy and hypersensitivity: mechanisms of allergic disease. Curr Opin Immunol 18: 718-726. https://doi.org/10.1016/j.coi.2006.09.016 ![]() |
[55] | Murphy K, Travers P, Walport M (2008) Allergy and hypersensitivity. Janeway's Immunobiology. UK: Garland Science. https://doi.org/10.1007/978-3-8274-2219-4 |
[56] |
Simon D (2018) Recent advances in clinical allergy and immunology. Int Arch Allergy Imm 177: 324-333. https://doi.org/10.1159/000494931 ![]() |
[57] |
Kumar Y, Bhatia A (2013) Immunopathogenesis of allergic disorders: current concepts. Expert Rev Clin Immunol 9: 211-226. https://doi.org/10.1586/eci.12.104 ![]() |
[58] | Vaillant AAJ, Vashisht R, Zito PM (2022) Immediate hypersensitivity reactions In: StatPearls, Treasure Island (FL): StatPearls Publishing. |
[59] |
Breiteneder H, Peng YQ, Agache I, et al. (2020) Biomarkers for diagnosis and prediction of therapy responses in allergic diseases and asthma. Allergy 75: 3039-3068. https://doi.org/10.1111/all.14582 ![]() |
[60] |
Galli SJ, Tsai M, Piliponsky AM (2008) The development of allergic inflammation. Nature 454: 445-454. https://doi.org/10.1038/nature07204 ![]() |
[61] |
Yao Y, Chen CL, Yu D, et al. (2021) Roles of follicular helper and regulatory T cells in allergic diseases and allergen immunotherapy. Allergy 76: 456-470. https://doi.org/10.1111/all.14639 ![]() |
[62] | Muñoz-Carrillo JL, Castro-García FP, Chávez-Rubalcaba F, et al. (2018) Immune system disorders: hypersensitivity and autoimmunity. Immunoregulatory Aspects of Immunotherapy. London: InTechOpen 1-30. https://doi.org/10.5772/intechopen.75794 |
[63] |
Remington B, Broekman HCH, Blom WM, et al. (2018) Approaches to assess IgE mediated allergy risks (sensitization and cross-reactivity) from new or modified dietary proteins. Food Chem Toxicol 112: 97-107. https://doi.org/10.1016/j.fct.2017.12.025 ![]() |
[64] |
Kanagaratham C, El Ansari YS, Lewis OL, et al. (2020) IgE and IgG antibodies as regulators of mast cell and basophil functions in food allergy. Front Immunol 11: 603050. https://doi.org/10.3389/fimmu.2020.603050 ![]() |
[65] | Janeway CA, Travers P, Walport M, et al. (2001) Immunobiology: The immune system in health and disease. Effector Mechanisms in Allergic Reactions. New York: Garland Science. |
[66] |
Kitaura J, Song J, Tsai M, et al. (2003) Evidence that IgE molecules mediate a spectrum of effects on mast cell survival and activation via aggregation of the FcepsilonRI. P Natl Acad Sci USA 100: 12911-12916. https://doi.org/10.1073/pnas.1735525100 ![]() |
[67] |
Dispenza MC (2019) Classification of hypersensitivity reactions. Allergy Asthma Proc 40: 470-473. https://doi.org/10.2500/aap.2019.40.4274 ![]() |
[68] |
Miyake K, Karasuyama H (2017) Emerging roles of basophils in allergic inflammation. Allergol Int 66: 382-391. https://doi.org/10.1016/j.alit.2017.04.007 ![]() |
[69] |
Prussin C, Metcalfe DD (2006) 5. IgE, mast cells, basophils, and eosinophils. J Allergy Clin Immunol 117: S450-S456. https://doi.org/10.1016/j.jaci.2005.11.016 ![]() |
[70] |
Sampson AP (2000) The role of eosinophils and neutrophils in inflammation. Clin Exp Allergy 30: 22-27. https://doi.org/10.1046/j.1365-2222.2000.00092.x ![]() |
[71] |
Drazdauskaitė G, Layhadi JA, Shamji MH (2020) Mechanisms of allergen immunotherapy in allergic rhinitis. Curr Allergy Asthma Rep 21: 2. https://doi.org/10.1007/s11882-020-00977-7 ![]() |
[72] | Akhouri S, House SA (2022) >Allergic rhinitis In: StatPearls, Treasure Island (FL): StatPearls Publishing. |
[73] |
Yu W, Freeland DMH, Nadeau KC (2016) Food allergy: immune mechanisms, diagnosis and immunotherapy. Nat Rev Immunol 16: 751-765. https://doi.org/10.1038/nri.2016.111 ![]() |
[74] |
Iweala OI, Choudhary SK, Commins SP (2018) Food allergy. Curr Gastroenterol Rep 20: 17. https://doi.org/10.1007/s11894-018-0624-y ![]() |
[75] |
Peters RL, Krawiec M, Koplin JJ, et al. (2021) Update on food allergy. Pediatr Allergy Immu 32: 647-657. https://doi.org/10.1111/pai.13443 ![]() |
[76] | Rehman A, Amin F, Sadeeqa S (2018) Prevalence of asthma and its management: A review. J Pak Med Assoc 68: 1823-1827. |
[77] | Ogeyingbo OD, Ahmed R, Gyawali M, et al. (2021) The relationship between vitamin D and asthma exacerbation. Cureus 13: e17279. https://doi.org/10.1111/pai.13443 |
[78] |
Bush A (2019) Pathophysiological mechanisms of asthma. Front Pediatr 7: 68. https://doi.org/10.3389/fped.2019.00068 ![]() |
[79] |
Nakamura Y, Tamaoki J, Nagase H, et al. (2020) Japanese guidelines for adult asthma. Allergol Int 69: 519-548. https://doi.org/10.1016/j.alit.2020.08.001 ![]() |
[80] |
Bilò MB, Martini M, Tontini C, et al. (2021) Anaphylaxis. Eur Ann Allergy Clin Immunol 53: 4-17. https://doi.org/10.23822/EurAnnACI.1764-1489.158 ![]() |
[81] | Pflipsen MC, Colon KMV (2020) Anaphylaxis: recognition and management. Am Fam Physician 102: 355-362. |
[82] |
Hackett TL, Singhera GK, Shaheen F, et al. (2011) Intrinsic phenotypic differences of asthmatic epithelium and its inflammatory responses to respiratory syncytial virus and air pollution. Am J Resp Cell Mol 45: 1090-1100. https://doi.org/10.1165/rcmb.2011-0031OC ![]() |
[83] |
Siddiqui S, Johansson K, Joo A, et al. (2021) Epithelial miR-141 regulates IL-13-induced airway mucus production. JCI Insight 6: e139019. https://doi.org/10.1172/jci.insight.139019 ![]() |
[84] |
Vercelli D (2008) Discovering susceptibility genes for asthma and allergy. Nat Rev Immunol 8: 169-182. https://doi.org/10.1038/nri2257 ![]() |
[85] |
Akdis CA (2021) Does the epithelial barrier hypothesis explain the increase in allergy, autoimmunity and other chronic conditions?. Nat Rev Immunol 21: 739-751. https://doi.org/10.1038/s41577-021-00538-7 ![]() |
[86] |
Kansen HM, Lebbink MA, Mul J, et al. (2020) Risk factors for atopic diseases and recurrent respiratory tract infections in children. Pediatr Pulm 55: 3168-3179. https://doi.org/10.1002/ppul.25042 ![]() |
[87] |
D'Amato G, Chong-Neto HJ, Ortega OPM, et al. (2020) The effects of climate change on respiratory allergy and asthma induced by pollen and mold allergens. Allergy 75: 2219-2228. https://doi.org/10.1111/all.14476 ![]() |
[88] |
Prescott S, Saffery R (2011) The role of epigenetic dysregulation in the epidemic of allergic disease. Clin Epigenetics 2: 223-232. https://doi.org/10.1007/s13148-011-0028-4 ![]() |
[89] |
Wilson CB, Rowell E, Sekimata M (2009) Epigenetic control of T-helper-cell differentiation. Nat Rev Immunol 9: 91-105. https://doi.org/10.1038/nri2487 ![]() |
[90] |
Thorburn AN, Macia L, Mackay CR (2014) Diet, metabolites, and “western-lifestyle” inflammatory diseases. Immunity 40: 833-842. https://doi.org/10.1016/j.immuni.2014.05.014 ![]() |
[91] |
Yazdanbakhsh M, Kremsner PG, van Ree R (2002) Allergy, parasites, and the hygiene hypothesis. Science 296: 490-494. https://doi.org/10.1126/science.296.5567.490 ![]() |
[92] |
Frei R, Lauener RP, Crameri R, et al. (2012) Microbiota and dietary interactions: an update to the hygiene hypothesis?. Allergy 67: 451-461. https://doi.org/10.1111/j.1398-9995.2011.02783.x ![]() |
[93] |
Raj D, Kabra SK, Lodha R (2014) Childhood obesity and risk of allergy or asthma. Immunol Allergy Clin 34: 753-765. https://doi.org/10.1016/j.iac.2014.07.001 ![]() |
[94] |
Metsälä J, Lundqvist A, Virta LJ, et al. (2013) Mother's and offspring's use of antibiotics and infant allergy to cow's milk. Epidemiology 24: 303-309. https://doi.org/10.1097/EDE.0b013e31827f520f ![]() |
[95] |
Loh W, Tang MLK (2018) The epidemiology of food allergy in the global context. Int J Environ Res Public Health 15: 2043. https://doi.org/10.3390/ijerph15092043 ![]() |
[96] |
Simon D (2019) Recent advances in clinical allergy and immunology 2019. Int Arch Allergy Imm 180: 291-305. https://doi.org/10.1159/000504364 ![]() |
[97] |
Oro AS, Guarino TJ, Driver R, et al. (1996) Regulation of disease susceptibility: decreased prevalence of IgE-mediated allergic disease in patients with multiple sclerosis. J Allergy Clin Immunol 97: 1402-1408. https://doi.org/10.1016/S0091-6749(96)70210-5 ![]() |
[98] |
Cameron MH, Nilsagard Y (2018) Balance, gait, and falls in multiple sclerosis. Handb Clin Neurol 159: 237-250. https://doi.org/10.1016/B978-0-444-63916-5.00015-X ![]() |
[99] |
Rosiak K, Zagożdżon P (2017) Quality of life and social support in patients with multiple sclerosis. Psychiatr Pol 51: 923-935. https://doi.org/10.12740/PP/64709 ![]() |
[100] |
Beiske AG, Naess H, Aarseth JH, et al. (2007) Health-related quality of life in secondary progressive multiple sclerosis. Mult Scler 13: 386-392. https://doi.org/10.1177/13524585070130030101 ![]() |
[101] |
Lock C, Hermans G, Pedotti R, et al. (2002) Gene-microarray analysis of multiple sclerosis lesions yields new targets validated in autoimmune encephalomyelitis. Nat Med 8: 500-508. https://doi.org/10.1038/nm0502-500 ![]() |
[102] |
Pedotti R, DeVoss JJ, Youssef S, et al. (2003) Multiple elements of the allergic arm of the immune response modulate autoimmune demyelination. P Natl Acad Sci USA 100: 1867-1872. https://doi.org/10.1073/pnas.252777399 ![]() |
[103] |
Musio S, Gallo B, Scabeni S, et al. (2006) A key regulatory role for histamine in experimental autoimmune encephalomyelitis: disease exacerbation in histidine decarboxylase-deficient mice. J Immunol 176: 17-26. https://doi.org/10.4049/jimmunol.176.1.17 ![]() |
[104] |
Lapilla M, Gallo B, Martinello M, et al. (2011) Histamine regulates autoreactive T cell activation and adhesiveness in inflamed brain microcirculation. J Leukocyte Biol 89: 259-267. https://doi.org/10.1189/jlb.0910486 ![]() |
[105] |
Gregory GD, Robbie-Ryan M, Secor VH, et al. (2005) Mast cells are required for optimal autoreactive T cell responses in a murine model of multiple sclerosis. Eur J Immunol 35: 3478-3486. https://doi.org/10.1002/eji.200535271 ![]() |
[106] | Alonso A, Hernán MA, Ascherio A (2008) Allergy, family history of autoimmune diseases, and the risk of multiple sclerosis. Acta Neurol Scand 117: 15-20. |
[107] |
Alonso A, Jick SS, Hernán MA (2006) Allergy, histamine 1 receptor blockers, and the risk of multiple sclerosis. Neurology 66: 572-575. https://doi.org/10.1212/01.wnl.0000198507.13597.45 ![]() |
[108] | Karimi P, Modarresi SZ, Sahraian MA, et al. (2013) The relation of multiple sclerosis with allergy and atopy: a case control study. Iran J Allergy Asthma Immunol 12: 182-189. |
[109] |
Krishna MT, Subramanian A, Adderley NJ, et al. (2019) Allergic diseases and long-term risk of autoimmune disorders: longitudinal cohort study and cluster analysis. Eur Respir J 54: 1900476. https://doi.org/10.1183/13993003.00476-2019 ![]() |
[110] |
Mansouri B, Asadollahi S, Heidari K, et al. (2014) Risk factors for increased multiple sclerosis susceptibility in the Iranian population. J Clin Neurosci 21: 2207-2211. https://doi.org/10.1016/j.jocn.2014.04.020 ![]() |
[111] |
Chen J, Taylor B, Winzenberg T, et al. (2020) Comorbidities are prevalent and detrimental for employment outcomes in people of working age with multiple sclerosis. Mult Scler 26: 1550-1559. https://doi.org/10.1177/1352458519872644 ![]() |
[112] |
Lo LMP, Taylor BV, Winzenberg T, et al. (2021) Comorbidity patterns in people with multiple sclerosis: A latent class analysis of the Australian Multiple Sclerosis Longitudinal Study. Eur J Neurol 28: 2269-2279. https://doi.org/10.1111/ene.14887 ![]() |
[113] |
Hill E, Abboud H, Briggs FBS (2019) Prevalence of asthma in multiple sclerosis: A United States population-based study. Mult Scler Relat Disord 28: 69-74. https://doi.org/10.1016/j.msard.2018.12.012 ![]() |
[114] |
Sorensen A, Conway DS, Briggs FBS (2021) Characterizing relapsing remitting multiple sclerosis patients burdened with hypertension, hyperlipidemia, and asthma. Mult Scler Relat Disord 53: 103040. https://doi.org/10.1016/j.msard.2021.103040 ![]() |
[115] |
Tremlett HL, Evans J, Wiles CM, et al. (2002) Asthma and multiple sclerosis: an inverse association in a case-control general practice population. QJM 95: 753-756. https://doi.org/10.1093/qjmed/95.11.753 ![]() |
[116] |
Bergamaschi R, Villani S, Crabbio M, et al. (2009) Inverse relationship between multiple sclerosis and allergic respiratory diseases. Neurol Sci 30: 115-118. https://doi.org/10.1007/s10072-009-0036-8 ![]() |
[117] |
Sahraian MA, Jafarian S, Sheikhbahaei S, et al. (2013) Respiratory tract rather than cutaneous atopic allergy inversely associate with multiple sclerosis: a case-control study. Clin Neurol Neurosur 115: 2099-2102. https://doi.org/10.1016/j.clineuro.2013.07.028 ![]() |
[118] |
Pedotti R, Farinotti M, Falcone C, et al. (2009) Allergy and multiple sclerosis: a population-based case-control study. Mult Scler 15: 899-906. https://doi.org/10.1177/1352458509106211 ![]() |
[119] |
Ren J, Ni H, Kim M, et al. (2017) Allergies, antibiotics use, and multiple sclerosis. Curr Med Res Opin 33: 1451-1456. https://doi.org/10.1080/03007995.2017.1325575 ![]() |
[120] |
Skaaby T, Husemoen LL, Thuesen BH, et al. (2015) Specific IgE positivity against inhalant allergens and development of autoimmune disease. Autoimmunity 48: 282-288. https://doi.org/10.3109/08916934.2014.1003640 ![]() |
[121] |
Manouchehrinia A, Edwards LJ, Roshanisefat H, et al. (2015) Multiple sclerosis course and clinical outcomes in patients with comorbid asthma: a survey study. BMJ Open 5: e007806. https://doi.org/10.1136/bmjopen-2015-007806 ![]() |
[122] |
Ponsonby AL, Dwyer T, van der Mei I, et al. (2006) Asthma onset prior to multiple sclerosis and the contribution of sibling exposure in early life. Clin Exp Immunol 146: 463-470. https://doi.org/10.1111/j.1365-2249.2006.03235.x ![]() |
[123] |
Hughes AM, Lucas RM, McMichael AJ, et al. (2013) Early-life hygiene-related factors affect risk of central nervous system demyelination and asthma differentially. Clin Exp Immunol 172: 466-474. https://doi.org/10.1111/cei.12077 ![]() |
[124] | Lopez CM, Yarrarapu SNS, Mendez MD (2022) Food allergies In: StatPearls, Treasure Island (FL): StatPearls Publishing. |
[125] |
Yu JW, Pekeles G, Legault L, et al. (2006) Milk allergy and vitamin D deficiency rickets: a common disorder associated with an uncommon disease. Ann Allerg Asthma Im 96: 615-619. https://doi.org/10.1016/S1081-1206(10)63558-2 ![]() |
[126] |
Correale J, Ysrraelit MC, Gaitán MI (2009) Immunomodulatory effects of vitamin D in multiple sclerosis. Brain 132: 1146-1160. https://doi.org/10.1093/brain/awp033 ![]() |
[127] |
Ramagopalan SV, Dyment DA, Guimond C, et al. (2010) Childhood cow's milk allergy and the risk of multiple sclerosis: a population based study. J Neurol Sci 291: 86-88. https://doi.org/10.1016/j.jns.2009.10.021 ![]() |
[128] | Pichler J, Gerstmayr M, Szepfalusi Z, et al. (2002) 1 alpha,25(OH)2D3 inhibits not only Th1 but also Th2 differentiation in human cord blood T cells. Pediatr Res 52: 12-18. https://doi.org/10.1203/00006450-200207000-00005 |
[129] |
Sakaguchi S, Yamaguchi T, Nomura T, et al. (2008) Regulatory T cells and immune tolerance. Cell 133: 775-787. https://doi.org/10.1016/j.cell.2008.05.009 ![]() |
[130] |
Mirzakhani H, Al-Garawi A, Weiss ST, et al. (2015) Vitamin D and the development of allergic disease: how important is it?. Clin Exp Allergy 45: 114-125. https://doi.org/10.1111/cea.12430 ![]() |
[131] |
Danikowski KM, Jayaraman S, Prabhakar BS (2017) Regulatory T cells in multiple sclerosis and myasthenia gravis. J Neuroinflammation 14: 117. https://doi.org/10.1186/s12974-017-0892-8 ![]() |
[132] | Ashtari F, Jamshidi F, Shoormasti RS, et al. (2013) Cow's milk allergy in multiple sclerosis patients. J Res Med Sci 18: S62-S65. |
[133] | Ashtari F, Jamshidi F, Shoormasti RS, et al. (2013) Fish and egg specific immunoglobin e in multiple sclerosis patients. Int J Prev Med 4: S185-S188. |
[134] |
Bourne T, Waltz M, Casper TC, et al. (2017) Evaluating the association of allergies with multiple sclerosis susceptibility risk and disease activity in a pediatric population. J Neurol Sci 375: 371-375. https://doi.org/10.1016/j.jns.2017.02.041 ![]() |
[135] |
Fakih R, Diaz-Cruz C, Chua AS, et al. (2019) Food allergies are associated with increased disease activity in multiple sclerosis. J Neurol Neurosur Ps 90: 629-635. https://doi.org/10.1136/jnnp-2018-319301 ![]() |
[136] |
Albatineh AN, Alroughani R, Al-Temaimi R (2020) Predictors of multiple sclerosis severity score in patients with multiple sclerosis. Int J MS Care 22: 233-238. https://doi.org/10.7224/1537-2073.2019-054 ![]() |
[137] |
Parodi B, de Rosbo NK (2021) The gut-brain axis in multiple sclerosis. Is its dysfunction a pathological trigger or a consequence of the disease?. Front Immunol 12: 718220. https://doi.org/10.3389/fimmu.2021.718220 ![]() |
[138] |
Agirman G, Yu KB, Hsiao EY (2021) Signaling inflammation across the gut-brain axis. Science 374: 1087-1092. https://doi.org/10.1126/science.abi6087 ![]() |
1. | Clara Ureña-Paniego, Trinidad Montero-Vílchez, Salvador Arias-Santiago, Tralokinumab for the Treatment of Atopic Dermatitis in a Patient with Multiple Sclerosis, 2024, 1710-3568, 10.1089/derm.2024.0038 |
Reference | Type of study | Specimens | Main findings |
Pedotti R et al. (2003) [102] | Experimental study | EAE mice (brain tissue) | Increase in prostaglandin D synthase and tryptase; H1R-H2R expression; Reduction of EAE severity through: H1R and PAFR antagonists, FcγRIII and FcϵRI gene inhibition |
Musio S et al. (2006) [103] | Experimental study | EAE HDC−/− (histamine-deficient) mice vs EAE WT mice | Increase development of autoimmune disease in HDC−/− |
Lapilla M et al. (2011) [104] | Experimental study | PLP-activated mouse T cells | Inhibition of T cells proliferation, IFNγ production and adhesion on ICAM1, by histamine agonist |
Gregory GD et al. (2005) [105] | Experimental study | EAE W/Wv (mast cell-deficient) mice vs EAE WT mice | Worsening of disease by mast cells |
EAE: experimental autoimmune encephalomyelitis; FcϵRI: high-affinity IgE receptor; FcγRIII: Fc receptor for immune-complexed IgG; H1R: histamine receptor 1; H2R: histamine receptor 2; ICAM1: intercellular adhesion molecule 1; IFNγ: interferon γ; PAFR: platelet-activating factor receptor; PLP: myelin proteolipid protein; WT: wild type.
Reference | Type of study | Study group | Allergies/Symptoms | Main findings |
Alonso A et al. (2008) [106] | Case-control study (questionnaire on allergy history) | US population (298 MS women and 1248 controls) | Pollens, house dust, animal dander, foods, drugs, other | No correlation allergy-MS |
Alonso A et al. (2006) [107] | Case-control study (medical records on allergy history and H1R antagonist use) | UK population (163 MS and 1523 controls) | Rhinitis/hay fever, asthma, urticaria/angioedema, eczema/atopic dermatitis, other | No correlation allergy-MS; negative correlation H1R antagonist-MS |
Karimi P et al. (2013) [108] | Case-control study (questionnaire on allergy history and IgE measure on serum sample) | Iranian population (40 MS and 40 controls) | Conjunctivitis, rhinitis, urticaria and eczema, asthma; plant and animal allergies | No differences in allergy history and IgE levels between MS and controls |
Krishna MT et al. (2019) [109] | Retrospective cohort study (database on autoimmune disorders) | UK population (782320 allergic rhinitis/conjunctivitis, 1393570 atopic eczema, 1049868 asthma patients) | Rhinitis/conjunctivitis, eczema, asthma | No correlation allergy-MS |
Mansouri B et al. (2014) [110] | Multicenter case-control study (questionnaire on environmental and familial factors) | Iranian population (1403 MS and 883 controls) | Foods, drugs, pollens, house dust, animal dander, other | Positive correlation allergy-MS |
Chen J et al. (2020) [111] | Cohort study (questionnaire on comorbidities and employment outcomes) | Australian population (929 MS working-aged) | Not reported | Positive correlation allergy-MS; worsening of MS symptoms; reduction of work performance; increase of annual costs |
Lo LMP et al. (2021) [112] | Cohort study (medical records on comorbidities) | Australian population (1518 MS) | Not reported | No correlation allergy-MS; allergy comorbidity (37.4% self-reported, 28.6% diagnosed) |
Hill E at al. (2019) [113] | Retrospective study (records on asthma) | US population (141880 MS and 56416790 controls) | Asthma | Asthma prevalence among young and elderly MS |
Sorensen A et al. (2021) [114] | Cross-sectional study (medical records on asthma and other comorbidities) | US population (2012 RRMS) | Asthma | Characterization of asthmatic RRMS patients: women, obese, hypertensive; with a medium/low income; no treated with DMT |
Tremlett HL et al. (2002) [115] | Retrospective case-control study (medical records on asthma, eczema and dermatitis) | Wales population (320 MS and 320 controls) | Asthma | Asthma lower prevalence among MS |
Bergamaschi R et al. (2009) [116] | Case-control study (questionnaire on ARD) | Italian population (200 MS and 200 controls) | ARD | Negative correlation allergy-MS; less MS severity in coexistence with ARD |
Sahraian MA et al. (2013) [117] | Case-control study (questionnaire on RTA, CA and FDA) | Iranian population (195 MS and 195 controls) | RTA, CA and FDA | RTA and FDA protective role against MS onset (negative correlation) |
Pedotti R et al. (2009) [118] | Case-control study (questionnaire on allergy history) | Italian population (423 MS and 643 controls) | Allergic asthma, rhinitis, conjunctivitis, atopic dermatitis and eczema, contact dermatitis, food, drug and insect allergies | Negative correlation atopic allergies-MS |
Ren J et al. (2017) [119] | Case-control study (database on AD) | American population (829 MS and 2441 controls) | Respiratory tract allergies and other | Protective role of allergies for MS onset (negative correlation) |
Skaaby T et al. (2015) [120] | Cohort study (detection of allergy specific IgE) | Danish population (14849 persons including 834 autoimmune diseases with 35 MS) | Respiratory allergens | No correlation allergy-MS |
Manouchehrinia A et al. (2015) [121] | Cohort study (questionnaire on asthma and MS grade) | England population (680 MS) | Asthma | No correlation allergy-MS; worsening of psychological conditions in asthmatic MS |
Ponsonby AL et al. (2006) [122] | Case-control study (questionnaire on asthma and sibling exposure) | Australian population (136 MS and 272 controls) | Asthma | Positive correlation MS-allergy (strengthened in MS with reduced sibling exposure) |
Hughes AM et al. (2013) [123] | Multicenter case-control study (questionnaire on comorbidities and sibship structure) | Australian population (282 FCD adults and 588 control) | Asthma, hayfever, eczema | No correlation allergy-FCD; exposure to younger siblings reduced FCD but not asthma risk |
Ramagopalan SV et al. (2010) [127] | Cohort study (questionnaire on CMA) | Canadian population (6638 MS and 2509 controls) | CMA | No correlation allergy-MS |
Ashtari F et al. (2013) [132],[133] | Case-control study (detection of allergy specific IgE) | Iranian population (48 MS and 48 controls) | Cow's milk, eggs and fish allergies | No correlation allergy-MS |
Bourne T et al. (2017) [134] | Case-control study (questionnaire on allergy history) | US pediatric population (271 MS and 418 controls) | Environmental and food allergies | No correlation environmental allergy-MS; negative correlation food allergy-MS activity |
Fakih R et al. (2019) [135] | Cohort study (questionnaire on allergy history) | US population (1349 MS) | Environmental, food and drug allergies | Positive correlation food allergy-MS activity (worsening effect) |
Albatineh AN et al. (2020) [136] | Cross-sectional study (questionnaire on lifestyle habits) | Kuwaiti population (128 MS) | Food allergy | No correlation allergy-MSSS |
AD: allergic diseases; ARD: allergic respiratory diseases; CA: coetaneous allergy; CMA: cow's milk allergy; DMT: disease-modifying therapies; FCD: first clinical diagnosis of central nervous system demyelination; FDA: food/drug allergy; H1R: histamine receptor 1; IgE: immunoglobulin E; MS: multiple sclerosis; MSSS: MS severity score; RRMS: relapsing-remitting MS; RTA: respiratory tract allergy.
Reference | Type of study | Specimens | Main findings |
Pedotti R et al. (2003) [102] | Experimental study | EAE mice (brain tissue) | Increase in prostaglandin D synthase and tryptase; H1R-H2R expression; Reduction of EAE severity through: H1R and PAFR antagonists, FcγRIII and FcϵRI gene inhibition |
Musio S et al. (2006) [103] | Experimental study | EAE HDC−/− (histamine-deficient) mice vs EAE WT mice | Increase development of autoimmune disease in HDC−/− |
Lapilla M et al. (2011) [104] | Experimental study | PLP-activated mouse T cells | Inhibition of T cells proliferation, IFNγ production and adhesion on ICAM1, by histamine agonist |
Gregory GD et al. (2005) [105] | Experimental study | EAE W/Wv (mast cell-deficient) mice vs EAE WT mice | Worsening of disease by mast cells |
Reference | Type of study | Study group | Allergies/Symptoms | Main findings |
Alonso A et al. (2008) [106] | Case-control study (questionnaire on allergy history) | US population (298 MS women and 1248 controls) | Pollens, house dust, animal dander, foods, drugs, other | No correlation allergy-MS |
Alonso A et al. (2006) [107] | Case-control study (medical records on allergy history and H1R antagonist use) | UK population (163 MS and 1523 controls) | Rhinitis/hay fever, asthma, urticaria/angioedema, eczema/atopic dermatitis, other | No correlation allergy-MS; negative correlation H1R antagonist-MS |
Karimi P et al. (2013) [108] | Case-control study (questionnaire on allergy history and IgE measure on serum sample) | Iranian population (40 MS and 40 controls) | Conjunctivitis, rhinitis, urticaria and eczema, asthma; plant and animal allergies | No differences in allergy history and IgE levels between MS and controls |
Krishna MT et al. (2019) [109] | Retrospective cohort study (database on autoimmune disorders) | UK population (782320 allergic rhinitis/conjunctivitis, 1393570 atopic eczema, 1049868 asthma patients) | Rhinitis/conjunctivitis, eczema, asthma | No correlation allergy-MS |
Mansouri B et al. (2014) [110] | Multicenter case-control study (questionnaire on environmental and familial factors) | Iranian population (1403 MS and 883 controls) | Foods, drugs, pollens, house dust, animal dander, other | Positive correlation allergy-MS |
Chen J et al. (2020) [111] | Cohort study (questionnaire on comorbidities and employment outcomes) | Australian population (929 MS working-aged) | Not reported | Positive correlation allergy-MS; worsening of MS symptoms; reduction of work performance; increase of annual costs |
Lo LMP et al. (2021) [112] | Cohort study (medical records on comorbidities) | Australian population (1518 MS) | Not reported | No correlation allergy-MS; allergy comorbidity (37.4% self-reported, 28.6% diagnosed) |
Hill E at al. (2019) [113] | Retrospective study (records on asthma) | US population (141880 MS and 56416790 controls) | Asthma | Asthma prevalence among young and elderly MS |
Sorensen A et al. (2021) [114] | Cross-sectional study (medical records on asthma and other comorbidities) | US population (2012 RRMS) | Asthma | Characterization of asthmatic RRMS patients: women, obese, hypertensive; with a medium/low income; no treated with DMT |
Tremlett HL et al. (2002) [115] | Retrospective case-control study (medical records on asthma, eczema and dermatitis) | Wales population (320 MS and 320 controls) | Asthma | Asthma lower prevalence among MS |
Bergamaschi R et al. (2009) [116] | Case-control study (questionnaire on ARD) | Italian population (200 MS and 200 controls) | ARD | Negative correlation allergy-MS; less MS severity in coexistence with ARD |
Sahraian MA et al. (2013) [117] | Case-control study (questionnaire on RTA, CA and FDA) | Iranian population (195 MS and 195 controls) | RTA, CA and FDA | RTA and FDA protective role against MS onset (negative correlation) |
Pedotti R et al. (2009) [118] | Case-control study (questionnaire on allergy history) | Italian population (423 MS and 643 controls) | Allergic asthma, rhinitis, conjunctivitis, atopic dermatitis and eczema, contact dermatitis, food, drug and insect allergies | Negative correlation atopic allergies-MS |
Ren J et al. (2017) [119] | Case-control study (database on AD) | American population (829 MS and 2441 controls) | Respiratory tract allergies and other | Protective role of allergies for MS onset (negative correlation) |
Skaaby T et al. (2015) [120] | Cohort study (detection of allergy specific IgE) | Danish population (14849 persons including 834 autoimmune diseases with 35 MS) | Respiratory allergens | No correlation allergy-MS |
Manouchehrinia A et al. (2015) [121] | Cohort study (questionnaire on asthma and MS grade) | England population (680 MS) | Asthma | No correlation allergy-MS; worsening of psychological conditions in asthmatic MS |
Ponsonby AL et al. (2006) [122] | Case-control study (questionnaire on asthma and sibling exposure) | Australian population (136 MS and 272 controls) | Asthma | Positive correlation MS-allergy (strengthened in MS with reduced sibling exposure) |
Hughes AM et al. (2013) [123] | Multicenter case-control study (questionnaire on comorbidities and sibship structure) | Australian population (282 FCD adults and 588 control) | Asthma, hayfever, eczema | No correlation allergy-FCD; exposure to younger siblings reduced FCD but not asthma risk |
Ramagopalan SV et al. (2010) [127] | Cohort study (questionnaire on CMA) | Canadian population (6638 MS and 2509 controls) | CMA | No correlation allergy-MS |
Ashtari F et al. (2013) [132],[133] | Case-control study (detection of allergy specific IgE) | Iranian population (48 MS and 48 controls) | Cow's milk, eggs and fish allergies | No correlation allergy-MS |
Bourne T et al. (2017) [134] | Case-control study (questionnaire on allergy history) | US pediatric population (271 MS and 418 controls) | Environmental and food allergies | No correlation environmental allergy-MS; negative correlation food allergy-MS activity |
Fakih R et al. (2019) [135] | Cohort study (questionnaire on allergy history) | US population (1349 MS) | Environmental, food and drug allergies | Positive correlation food allergy-MS activity (worsening effect) |
Albatineh AN et al. (2020) [136] | Cross-sectional study (questionnaire on lifestyle habits) | Kuwaiti population (128 MS) | Food allergy | No correlation allergy-MSSS |