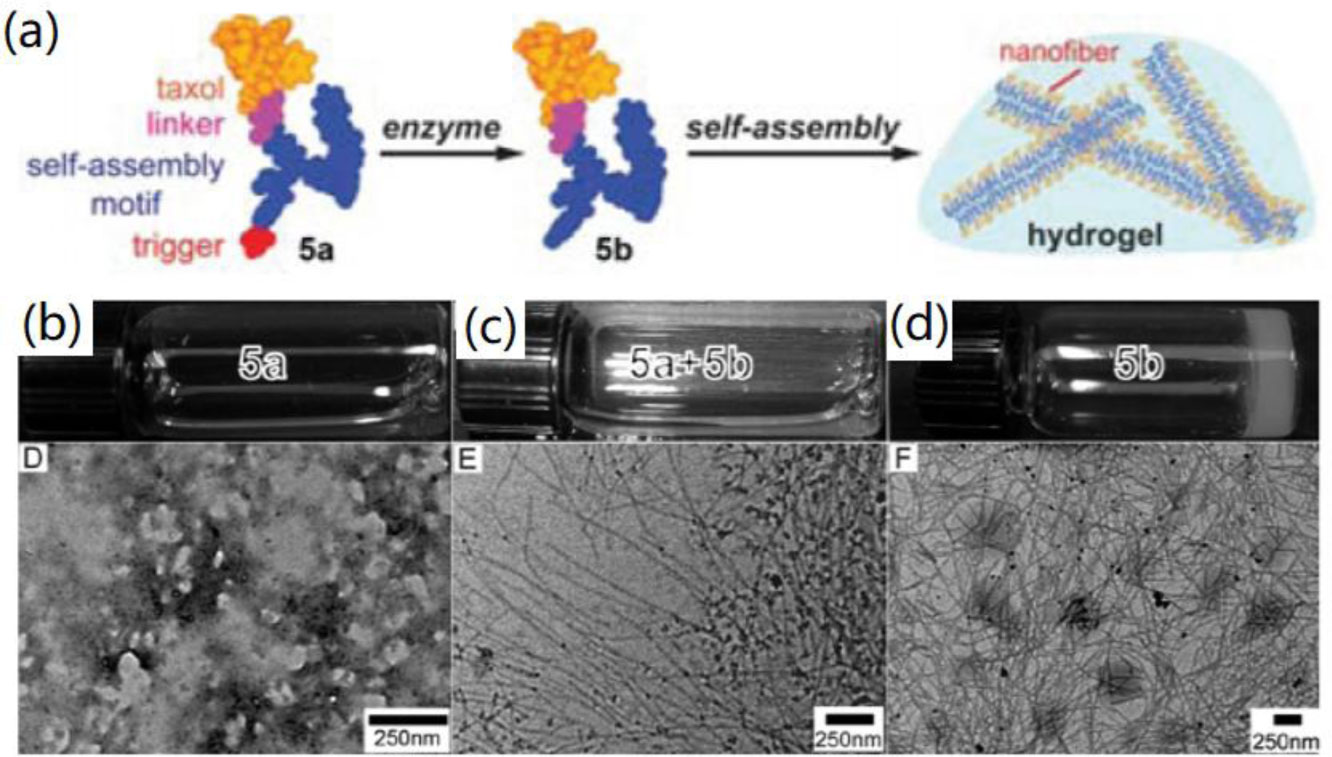
Citation: Yun Chen, Bin Liu, Lei Guo, Zhong Xiong, Gang Wei. Enzyme-instructed self-assembly of peptides: Process, dynamics, nanostructures, and biomedical applications[J]. AIMS Biophysics, 2020, 7(4): 411-428. doi: 10.3934/biophy.2020028
[1] | J. Dandurand, E. Dantras, C. Lacabanne, A. Pepe, B. Bochicchio, V. Samouillan . Thermal and dielectric fingerprints of self-assembling elastin peptides derived from exon30. AIMS Biophysics, 2021, 8(3): 236-247. doi: 10.3934/biophy.2021018 |
[2] | Nicola Gaetano Gatta, Gaetano Cammarota, Vittorio Gentile . Possible roles of transglutaminases in molecular mechanisms responsible for human neurodegenerative diseases. AIMS Biophysics, 2016, 3(4): 529-545. doi: 10.3934/biophy.2016.4.529 |
[3] | Raghvendra P Singh, Guillaume Brysbaert, Marc F Lensink, Fabrizio Cleri, Ralf Blossey . Kinetic proofreading of chromatin remodeling: from gene activation to gene repression and back. AIMS Biophysics, 2015, 2(4): 398-411. doi: 10.3934/biophy.2015.4.398 |
[4] | Aaron Lerner, Torsten Matthias . Don’t forget the exogenous microbial transglutaminases: it is immunogenic and potentially pathogenic. AIMS Biophysics, 2016, 3(4): 546-552. doi: 10.3934/biophy.2016.4.546 |
[5] | Enrica Serretiello, Martina Iannaccone, Federica Titta, Nicola G. Gatta, Vittorio Gentile . Possible pathophysiological roles of transglutaminase-catalyzed reactions in the pathogenesis of human neurodegenerative diseases. AIMS Biophysics, 2015, 2(4): 441-457. doi: 10.3934/biophy.2015.4.441 |
[6] | Domenico Lombardo, Pietro Calandra, Maria Teresa Caccamo, Salvatore Magazù, Luigi Pasqua, Mikhail A. Kiselev . Interdisciplinary approaches to the study of biological membranes. AIMS Biophysics, 2020, 7(4): 267-290. doi: 10.3934/biophy.2020020 |
[7] | Warin Rangubpit, Sunan Kitjaruwankul, Panisak Boonamnaj, Pornthep Sompornpisut, R.B. Pandey . Globular bundles and entangled network of proteins (CorA) by a coarse-grained Monte Carlo simulation. AIMS Biophysics, 2019, 6(2): 68-82. doi: 10.3934/biophy.2019.2.68 |
[8] | Carsten Sachse . Single-particle based helical reconstruction—how to make the most of real and Fourier space. AIMS Biophysics, 2015, 2(2): 219-244. doi: 10.3934/biophy.2015.2.219 |
[9] | Larisa I. Fedoreyeva, Boris F. Vanyushin . Regulation of DNA methyltransferase gene expression by short peptides in nicotiana tabacum regenerants. AIMS Biophysics, 2021, 8(1): 66-79. doi: 10.3934/biophy.2021005 |
[10] | Larisa I. Fedoreyeva, Boris F. Vanyushin, Ekaterina N. Baranova . Peptide AEDL alters chromatin conformation via histone binding. AIMS Biophysics, 2020, 7(1): 1-16. doi: 10.3934/biophy.2020001 |
The self-assembly of molecules is a simple, effective, and controllable process to form functional nanostructures and nanomaterials with various dimensions, in which molecules are combined each other spontaneously through both external and internal interactions [1]–[4]. In nature, a lot of biomolecules such as DNA, protein, peptide, virus, and biopolymers have shown unique characteristics to self-assemble into nanostructure, inspiring the design, synthesis, and applications of self-assembled biomaterials in the fields of nanotechnology, biomedicine, tissue engineering, energy storage, environmental science, and others [5]–[8].
Recently, more attentions have been attracted onto the structural design, functional tailoring, and applications of self-assembled peptide nanomaterials due to a few advantages of peptide-based nanomaterials [9]–[13]. For instance, the structure and function of peptides can be tailored easily by designing functional amino acid sequences, the self-assembly of peptides into nanofibers, nanotubes, nanosheets, and hydrogels can be controlled by adjusting experimental conditions, and the formed peptide nanomaterials have excellent bioactivity and biocompatibility [9].
Many strategies can be utilized for promoting the self-assembly of peptide molecules into desired nanomaterials [14]–[17]. Compared with physical and chemical regulations that initiate the self-assembly of peptides, including adjusting the concentration of self-assembly components, solvents, temperature, pH value, ionic strength, metal ions, physical stimulations, and ligand-receptor interactions [18], the enzyme-instructed self-assembly of peptides has exhibited obvious advantages and potentials due to its combination with bio-mediated self-assembly and highly related biomedical applications [19]–[23]. In addition, enzyme-instructed peptide self-assembly has other advantages of good biocompatibility and mild reaction conditions, which can convert monodispersed peptide precursors into self-assembled building blocks under constant conditions, which can better control the self-assembly process and carry out biomedical applications [21],[24]. Meanwhile, the enzymes produced by living cells have high specificity and catalytic performance on their substrates [25]. Therefore, the self-assembly systems with specific biological functions could be obtained by using specific enzymes to guide the self-assembly of peptides [26], which make it possible to prepare highly complex biomimetic nanomaterials through controlling the formation of nanostructures with enzymatic instruction.
Inspired by the naturally occurring self-assembly systems, peptides with self-assembly functions have been widely used to construct various functional nanomaterials [27]–[31]. Previously studies indicated that peptide with different conformations could self-assemble into different nanostructures under enzymatic guiding. For instance, α-helix is the most common conformation in peptides, and the peptides with α-helix conformation can form stable nanofibers and hydrogels by controllable self-assembly. Wang et al. found that the enzyme provided a unique way to assist peptides to self-assemble into α-helix conformation, and the self-assembly of the α-helix conformation resulted in the formation of peptide nanomaterials with stable nanostructure, strong cell uptake, and high inhibition efficiency towards cancer cells and tumors [32]. In another study, Yang et al. explored further the advantage of enzyme-induced peptide self-assembly to form polypeptide hydrogels, which could in-situ trigger the self-assembly of peptides [22]. Therefore, when a specific enzyme was overexpressed in the sites that needs medical treatment, it can immediately promote the synthesis of peptide nanomaterials, thus improving the selectivity and efficiency of therapy.
To present the progress of enzyme-instructed self-assembly of peptide and the formation of functional biomaterials for various applications, here we would like to contribute a review to summarize the enzyme-induced peptide self-assembly, kinetics/dynamics control, self-assembled structures, and bio-applications of the formed peptide nanomaterials. It is expected this work could provide useful information for readers to understand the unique properties of enzymes for assisting the self-assembly of peptide and other biomolecules to create novel bionanomaterials for biomedical and tissue engineering applications.
In this section, the self-assembly of peptides by using enzymes in both extracellular and intracellular situations is introduced and discussed.
Phosphatase is widely found in cells, tissues, and organs, and it can hydrolyze the phosphate groups of molecules and make them self-assemble to form various nanostructures through the cleavage of P-O bond [33]. Paclitaxel is a widely used antineoplastic drug, which shows significant activity towards the treatment of cancers of lung, breast, colorectal, ovarian, and bladder. However, because paclitaxel is a highly hydrophobic drug, it needs to be converted into water-active form without harming its biological activity. To overcome this problem, Gao et al. synthesized a hydrogel precursor (named as 5a) as shown in Figure 1a, which was composed of a self-assembly sequence, an enzyme cutting group, a linker, and a paclitaxel molecule [34]. Under the hydrolysis of alkaline phosphatase, the precursor was converted into a hydrogel-forming agent (named as 5b), which could self-assemble into nanofibers and provide supramolecular hydrogels of paclitaxel derivatives [34]. It was found that no peptide nanofibers were formed without the enzymatic treating (Figure 1b), however, the addition of enzyme into the peptide solution for 5 min induced the formation of peptide nanofibers quickly (Figure 1c). After overnight incubation, the enzyme-treated peptides formed hydrogels through the molecular linking between the formed self-assembled nanofibers, as shown in Figure 1d. The formed peptide nanofibers could slowly release paclitaxel derivatives into the water media, thus achieving in the purpose of cancer therapy. In another case, they demonstrated for the first time the self-assembly of phosphatase-hydrolyzed peptides containing serine phosphate. It was found that the supramolecular hydrogels could be used as potential soft biomaterials for cell culture and tissue engineering [35]. Besides, Gazit and co-workers have reported that the enzymatic activation of self-immolative dendrimers mediated the controlled assembly of peptide molecules to form uniform nanotubes [36].
With the help of enzymes, peptide precursors without the self-assembly ability could be induced to form self-assembled nanostructures through the breakage of chemical bonds [37], which could promote the connection of two precursors through the formation of catalytic bonds to create a suitable hydrogel-forming agent under the catalysis of enzymes [38]. For instance, Toledano et al. used thermal hemolysin to couple two peptide derivatives into hydrogel precursors, which self-assembled into three-dimensional (3D) nanofiber network and peptide hydrogels [39].
The above introduced studies indicated that the peptide self-assembly can be triggered by using hydrolase to tailor the conformation and structure of peptide molecules. Gao and co-workers found the first example of using tyrosinase oxidase to trigger the gel-sol phase transition of small molecular hydrogels [40]. The tetra-peptides produced by the hydrolysis of phosphate in tetra-peptide derivatives by phosphatase could self-assemble into peptide nanofibers and hydrogels. In the preparation process of peptide hydrogels, targeted drug molecules could be incorporated into the peptide hydrogel firstly. Oxidative tyrosinase could convert tyrosine in tetrapeptides into quinones, which leads to the loss of π-π interactions between phenol rings, resulting in the gel-sol phase transition. As a result, the corresponding drug molecules were released with the solvation of hydrogels. In addition, the drug releasing was controllable by using different amounts of tyrosinase. Due to the increase of tyrosinase activity in malignant melanoma, the formed enzyme-triggered peptide hydrogels with reactive activity towards tyrosinase could be used potentially to treat the malignant melanoma [41].
In addition to extracellular enzyme-induced molecular self-assembly, people have also explored the intracellular enzyme-induced molecular self-assembly [42]–[44]. For peptides, the first step is to form non-self-assembled polypeptide precursors outside the cells. After that polypeptide precursors are transported into living cells through the cell membrane to form self-assembly construction under the action of enzymes, and then form self-assembled nanostructure such as nanofibers after self-assembly, thus affecting the cell functions [45]–[47].
For some peptides that are difficult to enter into cells, strategies have been utilized to increase their uptake by combining with other substances. For example, D-peptide is an important molecular platform for biomedical applications due to its high biological activity and stability. However, because of its hydrolytic resistance of protein, it is lack of interaction with endogenous transporters, so it is difficult to enter into cells [48]. Fortunately, D-peptide can combine with natural amino acid, taurine, to form ester conjugate, which could greatly increase the cellular uptake of small D-peptide by mammalian cells [49].
In order to solve the problem of the resistance of anti-tumor drugs, Xu and co-workers conducted the first study on the combination of intracellular enzyme-induced self-assembly and cisplatin for the treatment of drug-resistant ovarian cancer [50]. As shown in Figure 2, two peptide precursors with mirrored structure (L-1 ad D-1) were utilized to prove their hypothesis, in which the addition of carboxylesterase (CES) to transfer the precursors to L-2 and D-2 peptides with self-assembly abilities. After the self-assembly of both L-2 and D-2 in one system for the formation of peptide nanofibers, the created nanofibers could interact with actin to inhibit the growth of cancer cells. In addition, the co-culture of cancer cells and normal cells confirmed that due to the over-expression of carboxylesterase in cancer cells, the self-assembly process is selective to cancer cells. Therefore, by introducing non-cytotoxic components to combine with cisplatin, the enzyme-induced peptide nanomaterials not only inhibited the growth of tumor cells, but also exhibited neglected effects on normal cells.
In this section, the self-assembly control by the trigger of enzymes and the formation of corresponding peptide nanostructures will be introduced.
The self-assembly of peptide molecules can be controlled by both kinetics and dynamics to produce relatively stable components. Ulijn et al. have investigated the self-assembly of aromatic short peptide derivatives that catalyzed by nonspecific endoprotease by studying the change of the free energy of the amide hydrolysis process [51]. It was found that the formation of amide was not helpful for mediating the thermodynamic reaction, but the change of the free energy was very small. For the self-assembly of aromatic peptide derivatives, in the process of the formation of self-assembled nanostructures, the created free energies were followed as ΔGself-assembly-ΔGamide hydrolysis < 0. Therefore, the whole process is more conducive to the formation of amide structure, and this type of reversible system was carried out under the control of thermodynamics. In addition, because the system was completely reversible, the self-tuning could be carried out in the process of forming self-assembled structure, and the components for self-assembly could be selected to form stable self-assembled nanostructures. In another study, they further studied the sequence-structure relationships in the formation of aromatic dipeptide hydrogels, and found that the enzyme-induced self-assembly of peptides was controlled by the thermodynamics [52].
In addition, Li et al. analyzed the kinetics of intracellular enzymatic self-assembly [53]. Three stereoisomers of dipeptide precursors (LD-1-SO3, DL-1-SO3, and DD-1-SO3) were designed and synthesized for studying the self-assembly that controlled by kinetics. After the hydrolysis by carboxylesterase (CES), these precursors produced corresponding hydrogel agents (Figure 3a). Because the formed hydrogel agents have different stereochemistry, the morphology of the self-assembled peptide nanofibers was also different. The precursors of DL-1-SO3 and DD-1-SO3 have the lowest and highest inhibitory activities on cancer cells respectively, which was inversely proportional to their reaction rates on the formation of hydrogels in PBS buffer. To further understand the enzyme-instructed self-assembly of peptide molecules, they selected three key processes to reflect the interactions between peptide drug and cancer cells, including the exchange of enzymatic self-assembled molecules inside and outside the cell, the chemical mechanism of supramolecular assembly through carboxylesterase, and the interaction between self-assembled nanostructures and cancer cells. Correspondingly, three kinetic parameters, the molecular transport coefficients of in and out cells (Kin/Kout), the Michaelis constant (KM), and the hydrogelation rate constant (Kg/Ks), were analyzed, as indicated in Figure 3b. The obtained results proved that the stereochemistry of precursors affected the morphology of the final nanostructures, and the cytotoxicity of intracellular enzymatic self-assembly to cancer cells was mostly determined by the inherent characteristics of peptide molecules. This study provided a potential strategy to enhance the self-assembly ability of biomolecules by using the stereochemistry of precursors, and meanwhile proved that it is possible to optimize intracellular enzyme-induced self-assembly of peptides by weakening the extracellular hydrolysis of molecules.
Under the control of kinetics and dynamics, peptides with designed sequences could be catalyzed under enzymes to form various self-assembled nanostructures such as nanofibers, nanotubes, vesicles, networks, and hydrogels. Here we would like to make a brief introduction on these self-assembled nanomaterials.
The Stupp group studied the formation of amphiphilic peptide nanofibers with enzyme response nanostructures for the first time [54], in which a pair of reversible enzymes were utilized to promote the assembly and disassembly of peptide amphiphilic (PA) nanostructures (Figure 4a). In their study, the used PA contained a common substrate sequence that is specific to the protein kinase A (PKA). After the treating with PKA, the PA molecules were phosphorylated, resulting the disassembly of PA nanofibers with high aspect ratio. After adding alkaline phosphatase (AP) into the disassembled peptide solution, filamentous nanostructures were reorganized by removing phosphate groups, as shown in Figure 4b. The interesting properties of the designed peptide could be utilized to fabricate enzymatic biosensors with high sensitivity and selectivity. In addition, the formed peptide nanofibers could be used for targeted drug delivery. PKA is mainly concentrated in the environment of cancer cell lines and it is a biomarker of extracellular cancers. Therefore, anticancer drugs could be integrated into PA to release drugs in the presence of cancer cells.
Aromatic dipeptide nanotubes are a kind of unique organic nanostructures. Under mild conditions, peptide nanotubes with high water-solubility and biocompatibility could be formed by using cheap starting materials, and the formed nanotubes had good chemical and thermal stability as well as high mechanical strength, and therefore peptide nanotubes have been widely used in biological and nanotechnological fields [55]–[57]. To enhance the control over the assembly process in the preparation of self-assembly of peptide nanotubes, Adler-Abramovich et al. proposed to use self-immolative dendritic system as a platform for controllable assembly of peptide nanotubes [36]. As shown in Figure 5a, a dendritic macromolecule 1 (AB3) was catalyzed by the penicillin G amidase (PGA) enzyme to disassemble to form amine intermediate (1a), which was further disassembled through triple elimination to release three drug units. Based on this mechanism, a novel self-immolative dendritic system by combining diphenylalanine (FF) and a PGA-specific trigger was designed for the formation of peptide nanotubes (Figure 5b). When the content of specific activating enzyme in malignant tissue was low, the advantage of this system was especially significant [58]. The designed AB3 dendritic system had also a significant advantage as an effective carrier for controlled formation of FF nanotubes. When the peptide was attached, it prevented the formation of any tissue structure. After the activation by enzyme, the dendritic peptide disassembled into three FF groups, which could be released quickly when the trigger was broken. In addition, the system allowed the release of terminal groups through a variety of triggers, and finally peptide nanotubes were formed through the self-assembly of terminal groups.
Peptide vesicles play an important role as drug delivery carriers and stimulus response materials [59]–[61]. For example, for the fat-soluble anti-cancer drug doxorubicin (DOX), an amphiphilic peptide A6K2 with the self-assembly properties has been designed to form peptide vesicles for drug delivery application [62]. As the out membrane of most tumor cells is negatively charges, the designed positively charged peptide could interact with the cell membrane via the electrostatic interaction, promoting the transport of DOX-coated peptide vesicles into tumor cells through the endocytosis, as indicated in Figure 5c. It was found that the plasma amine oxidase (PAO) and lysine oxidase (LO) can oxidize two charged lysine residues in the designed peptide molecule, inducing the degradation of self-assembled peptide vesicles to release DOX, and the vesicles were dissembled into nanofibers. The drug loading and releasing experiments indicated that the formed peptide vesicles were useful for long-term slow releasing of drugs and could be used as high-performance nanocarriers for treating diseases that needs long period.
In addition to causing cancer cell apoptosis through the self-assembly of enzymatic peptides, Kuang et al. observed that small D-peptide derivatives in the space around the cells could be phosphorylated by phosphatase on the cell surface, thus triggering the self-assembly of peptides to form nanoscale networks on the cell surface, as shown in Figure 5d [63]. Due to the over-expression of phosphatase around cancer cells, the formed hydrogels and nano-networks were formed on the surface of cancer cells selectively. In addition, the created nano-networks prevented the cancer cells from exchanging substances, thus inhibiting the growth of cancer cells and eventually inducing the death of cancer cells. In another study, Criado-Gonzalez and co-workers found that enzyme could catalyze the activity of a tripeptide to generate chemical hydrogels by self-assembly, resulting the formation of a supramolecular nano-network structure inside the covalent host material [64].
Besides the self-assembled nanostructures introduced above, peptide can also be instructed by the activation of enzymes to form 3D hydrogels, and the corresponding studies can be found in a very recent review by us [13].
In this part, the biomedical applications of enzyme-instructed self-assembly of peptide molecules for the cancer diagnostics, cancer therapy, bioelectronic devices, and biosensors are demonstrated.
How to achieve selective inhibition of cancer cells is still a major challenge in chemotherapy. Recently, a new concept based on enzyme-instructed self-assembly of peptides has been applied in supramolecular chemistry and chemical biology, especially in the development of a multi-step cancer treatment. As a new scheme, enzyme-instructed self-assembly of peptides consists of two processes: enzymatic reaction and self-assembly, which can achieve selective targeting of cancer cells. This process can achieve the combination of enzyme catalysis and molecular self-assembly, relying on local molecular self-assembly, thus disrupting multiple cellular processes [63].
Based on this, Zhou and co-workers synthesized two tetrapeptides, which contain one or two phosphate tyrosine residues, whose N-terminal is blocked by naphthyl [21]. Tetra-peptides form self-assembled molecules after enzymatic dephosphorylation. In their experiment, it was found that the phosphorylated D-tetrapeptide showed excellent inhibitory effect on cancer cells compared with the unphosphorylated D-tetrapeptide. Moreover, the comparison of monophosphorylated D-tetrapeptide and bisphosphorylated D-tetrapeptide also showed a better effect. The obtained results suggested that cancer cells with higher levels of expression are more likely to be inhibited by precursors, as shown in Figure 6. This strategy can also be used in other enzymes and self-assembly molecules or systems to explore supramolecular assembly in the cellular environment [65], exhibiting a potential highly effective method for cancer therapy.
Alkaline phosphatase (AP) is an enzyme overexpressed in stem cells and some cancer cells. In the body, it can convert ATP into adenosine, causing diseases such as cancer. In the previous research methods, the method of inhibiting the activity of AP was usually used to organize the occurrence of this process, but the efficiency was not very satisfactory. It has been found that enzyme-guided peptide self-assembly can effectively kill cancer cells that overexpress AP [66],[67]. Peptide assemblies were formed selectively in cancer cells and within cells, inducing the death of cancer cells by activating exogenous cell death receptors or producing intracellular cellular stress. For instance, Feng et al. further confirmed that AP guidance could effectively inhibit the peptide self-assembly of osteosarcoma tumors in vivo [68]. In animal experiments, it was found that AP produced peptide assemblies through the process of dephosphorylation catalyzed by precursors. This process inhibited the tumor growth in in-situ osteosarcoma mouse model without damaging other normal tissues of the body, thus would be highly valuable for developing an enzyme-guided peptide assembly as functional nanomaterials for in vivo cancer therapy.
In addition to direct killing cancer cells, self-assembled peptide nanostructures can also be utilized as drug carriers to deliver anti-tumor drug to kill the cancer cells. Small molecules can be self-assembled to form some molecular hydrogels through noncovalent interactions, which makes them have potential applications in drug delivery and tissue engineering [69]. Especially in drug delivery, small molecules that can respond to external stimuli have great potential. Gao et al. reported the synthesis of small molecular hydrogel of Ac-YYYY-OMe and speculated that the gel-sol phase transition may be triggered by tyrosinase oxidase [40]. Three structural compounds were further synthesized using phenylalanine (F) instead of tyrosine (Y) as a comparison. In the process of synthesizing supramolecular hydrogels, the drug molecules were added into the structure and the encapsulated drugs were released by different amount of tyrosinase to achieve in the cancer therapy in this way.
The stacking structure of aromatic rings in some organic molecules can self-assembled to form nanoscale wires, which makes it possible to be used in optical and bioelectronic devices [70]–[72]. Although researchers have synthesized some electronic materials based on the self-assembly of peptides [73], the electrical conductivity of peptide-based nanomaterials has not been measured directly. The measurement of the charge transport through some other methods, such as spectroscopy, undoubtedly leads to errors in the measurement of electrical conductivity.
It has been found that the enzyme-triggered peptide self-assembly was easy to control and can better transform the unassembled premise into self-assembled construction modules. Different conditions in the process of self-assembly, changing its chemical composition or the properties of self-assembled components can not only change the morphology of its nanostructure, but also further affect its electrical conductivity [74]. Xu et al. further studied the formation of π-β peptide nanotube network by enzyme-triggered self-assembly initiated by aromatic peptide amphiphiles, which showed significant charge transfer [75]. The team further measured the minimum thin layer resistance of macromolecular peptides that can achieve 0.1 MΩ/sq in air and 500 MΩ/sq in vacuum at room temperature. The synthesized nanomaterials are expected to be used in biology, such as biosensors, intelligent biomaterials and some biological photovoltaic devices.
In addition to the applications in electronic nanomaterials, the enzyme-induced peptide nanomaterials can also be used for the fabrication of biosensors. For instance, Zhang and co-workers reported the glucose metabolism-induced peptide self-assembly for colorimetric detection of glucose [76]. As shown in Figure 7a, glucose oxidase (GOx) mediated the glucose metabolism and promoted the self-assembly of peptide-based building blocks. When glucose and GOx were added into the peptide-based gelator 1 and gelator 2 systems, the glucose metabolism systems were created and the metabolic products triggered the formation and decomposition of hydrogels (Figure 7b). Therefore, the phase transition of gelator 1 (sol-gel) and 2 (gel-sol) could be used as indicator for colorimetric sensing glucose. This method was simple, highly sensitive, and selective for the detection of glucose, as indicated in Figure 7c and 7d, the addition of GOx and fructose into the gelator 1 and 2 did not cause their phase transition within 24 h.
Ulijn et al. developed the synthesis of peptide hydrogels through the enzyme-triggered reverse hydrolysis by using thermolysin [39]. In their experimental process, they found that the enzyme-triggered gelation of peptides could be utilized for the detection of protease activity. In another study, Bremmmer and co-workers further demonstrated that artificial blood clots could be triggered in human blood plasma by using enzyme-induced peptide gel formation [77].
Overall, in this review we presented the case studies on the self-assembly of peptide molecules triggered by enzymatic catalysis. It is clear that enzyme-instructed peptide self-assembly exhibited advantages for controllable formation of various peptide nanostructures and function-specific biomedical applications, especially for the in-situ cancer diagnostic and therapy. To help readers to understand the enzyme-induced formation of peptide nanostructures, we introduced the self-assembly kinetics and dynamics of peptides in both extracellular and intracellular conditions. In addition, the formation mechanisms of enzyme-induced peptide nanostructures from nanofibers to nanotubes, nanovesicles, network, and hydrogels are introduced and discussed.
Peptide self-assembly has been a very interesting and hot research field currently, however the enzyme-triggered peptide self-assembly was only conducted by several famous groups, which should attract more attentions by more researchers as this topic is showing high potential in a few aspects. First, more attention can be focused on the application of enzyme-induced peptide nanomaterials for tissue engineering, for instance the in-situ enzyme-mediated formation of peptide 2D or 3D nanomaterials for tissue repair and hemostasis. Second, more efforts could be done to synthesize other novel peptide nanostructures by using enzymatic catalysis, such as nanobelts and nanosheets. Meanwhile, the created peptide nanomaterials can be further fabricated to functional hybrid nanomaterials via biomimetic and bioinspired synthesis strategies, which will be useful for energy and environmental science applications. Third, it is also interesting to use enzyme to trigger the assembly and disassembly of peptide-drug conjugates with reversible reactions in vivo, in order to achieve in controllable, highly effective drug loading and releasing. Fourth, other kind of biomimetic enzymes such as artificial nanozymes could be used to replace natural enzymes to induce the peptide self-assembly, which could promote the exploration of novel synthesis techniques and the development of functional hybrid nanomaterials through a one-step reaction.
[1] | Whitesides GM, Grzybowski B (2002) Self-assembly at all scales. Science 295: 2418-2421. |
[2] | Zhou Y, Yan D (2009) Supramolecular self-assembly of amphiphilic hyperbranched polymers at all scales and dimensions: progress, characteristics and perspectives. Chem Commun 2009: 1172-1188. |
[3] | Müller WT, Klein DL, Lee T, et al. (1995) A strategy for the chemical synthesis of nanostructures. Science 268: 272-273. |
[4] | Ariga K, Nishikawa M, Mori T, et al. (2019) Self-assembly as a key player for materials nanoarchitectonics. Sci Technol Adv Mater 20: 51-95. |
[5] | Wang L, Sun Y, Li Z, et al. (2016) Bottom-up synthesis and sensor applications of biomimetic nanostructures. Materials 9: 53. |
[6] | Helbing C, Deckert-Gaudig T, Firkowska-Boden I, et al. (2018) Protein handshake on the nanoscale: How albumin and hemoglobin self-assemble into nanohybrid fibers. ACS Nano 12: 1211-1219. |
[7] | Zhang X, Gong C, Akakuru OU, et al. (2019) The design and biomedical applications of self-assembled two-dimensional organic biomaterials. Chem Soc Rev 48: 5564-5595. |
[8] | Gong C, Sun S, Zhang Y, et al. (2019) Hierarchical nanomaterials via biomolecular self-assembly and bioinspiration for energy and environmental applications. Nanoscale 11: 4147-4182. |
[9] | Gong C, Sun S, Zhang Y, et al. (2017) Self-assembling peptide and protein amyloids: from structure to tailored function in nanotechnology. Chem Soc Rev 46: 4661-4708. |
[10] | Wang J, Liu K, Xing R, et al. (2016) Peptide self-assembly: thermodynamics and kinetics. Chem Soc Rev 45: 5589-5604. |
[11] | Mason TO, Buell AK (2019) The kinetics, thermodynamics and mechanisms of short aromatic peptide self-assembly. Adv Exp Med Biol 1174: 61-112. |
[12] | Zhao YR, Yang W, Chen CX, et al. (2018) Rational design and self-assembly of short amphiphilic peptides and applications. Curr Opin Colloid Interf Sci 35: 112-123. |
[13] |
Wang Y, Zhang W, Gong C, et al. (2020) Recent advance in the fabrication, functionalization, and bioapplications of peptide hydrogels. Soft Matter . doi: 10.1039/D0SM00966K
![]() |
[14] | Draper ER, Su H, Brasnett C, et al. (2017) Opening a can of worm(-like micelle)s: the effect of temperature of solutions of functionalized dipeptides. Angew Chem Int Ed 56: 10467-10470. |
[15] | Ghosh A, Haverick M, Stump K, et al. (2012) Fine-tuning the pH trigger of self-assembly. J Am Chem Soc 134: 3647-3650. |
[16] | Carrick LM, Aggeli A, Boden N, et al. (2007) Effect of ionic strength on the self-assembly, morphology and gelation of pH responsive beta-sheet tape-forming peptides. Tetrahedron 63: 7457-7467. |
[17] | Li KH, Zhang ZF, Li DP, et al. (2018) Biomimetic ultralight, highly porous, shape-adjustable, and biocompatible 3D graphene minerals via incorporation of self-assembled peptide nanosheets. Adv Funct Mater 28: 1801056. |
[18] | Wang L, Gong CC, Yuan XZ, et al. (2019) Controlling the self-assembly of biomolecules into functional nanomaterials through internal interactions and external stimulations: A review. Nanomaterials 9: 285. |
[19] | Chen Y, Liang GL (2012) Enzymatic self-assembly of nanostructures for theranostics. Theranostics 2: 139-147. |
[20] | Gao Y, Yang ZM, Kuang Y, et al. (2010) Enzyme-instructed self-assembly of peptide derivatives to form nanofibers and hydrogels. Biopolymers 94: 19-31. |
[21] | Zhou J, Du XW, Yamagata N, et al. (2016) Enzyme-instructed self-assembly of small D-peptides as a multiple-step process for selectively killing cancer cells. J Am Chem Soc 138: 3813-3823. |
[22] | Gao J, Zhan J, Yang ZM (2020) Enzyme-instructed self-assembly (EISA) and hydrogelation of peptides. Adv Mater 32: 1805798. |
[23] | Criado-Gonzalez M, Loftin B, Fores JR, et al. (2020) Enzyme assisted peptide self-assemblies trigger cell adhesion in high density oxime based host gels. J Mater Chem B 8: 4419-4427. |
[24] | Shy AN, Li J, Shi JF, et al. (2020) Enzyme-instructed self-assembly of the stereoisomers of pentapeptides to form biocompatible supramolecular hydrogels. J Drug Target 28: 760-765. |
[25] | Walsh C (2001) Enabling the chemistry of life. Nature 409: 226-231. |
[26] |
Gao Y, Gao J, Mu G, et al. (2020) Selectively enhancing radiosensitivity of cancer cells via in situ enzyme-instructed peptide self-assembly. Acta Pharmaceutica Sinica B . doi: 10.1016/j.apsb.2020.07.022
![]() |
[27] | Liu L, Klausen LH, Dong MD (2018) Two-dimensional peptide based functional nanomaterials. Nano Today 23: 40-58. |
[28] | Janairo JIB, Sakaguchi T, Mine K, et al. (2018) Synergic strategies for the enhanced self-assembly of biomineralization peptides for the synthesis of functional nanomaterials. Protein Peptide Lett 25: 4-14. |
[29] | Zhang WS, Lin DM, Wang HX, et al. (2017) Supramolecular self-assembly bioinspired synthesis of luminescent gold nanocluster-embedded peptide nanofibers for temperature sensing and cellular imaging. Bioconjugate Chem 28: 2224-2229. |
[30] | Li Y, Zhang WS, Zhang L, et al. (2017) Sequence-designed peptide nanofibers bridged conjugation of graphene quantum dots with graphene oxide for high performance electrochemical hydrogen peroxide biosensor. Adv Mater Interfaces 4: 1600895. |
[31] | Zhang WS, Yu XQ, Li Y, et al. (2018) Protein-mimetic peptide nanofibers: Motif design, self-assembly synthesis, and sequence-specific biomedical applications. Prog Polym Sci 80: 94-124. |
[32] | Liang CH, Zheng DB, Shi F, et al. (2017) Enzyme-assisted peptide folding, assembly and anti-cancer properties. Nanoscale 9: 11987-11993. |
[33] | Huang P, Gao Y, Lin J, et al. (2015) Tumor-specific formation of enzyme-instructed supramolecular self-assemblies as cancer theranostics. ACS Nano 9: 9517-9527. |
[34] | Gao Y, Kuang Y, Guo ZF, et al. (2009) Enzyme-instructed molecular, self-assembly confers nanofibers and a supramolecular hydrogel of taxol derivative. J Am Chem Soc 131: 13576-13577. |
[35] | Zhou J, Du XW, Wang JQ, et al. (2017) Enzyme-instructed self-assembly of peptides containing phosphoserine to form supramolecular hydrogels as potential soft biomaterials. Front Chem Sci Eng 11: 509-515. |
[36] | Adler-Abramovich L, Perry R, Sagi A, et al. (2007) Controlled assembly of peptide nanotubes triggered by enzymatic activation of self-immolative dendrimers. ChemBioChem 8: 859-862. |
[37] | Yang ZM, Ho PL, Liang GL, et al. (2007) Using beta-lactamase to trigger supramolecular hydrogelation. J Am Chem Soc 129: 266-267. |
[38] | Das AK, Collins R, Ulijn RV (2008) Exploiting enzymatic (reversed) hydrolysis in directed setf-assembly of peptide nanostructures. Small 4: 279-287. |
[39] | Toledano S, Williams RJ, Jayawarna V, et al. (2006) Enzyme-triggered self-assembly of peptide hydrogels via reversed hydrolysis. J Am Chem Soc 128: 1070-1071. |
[40] | Gao J, Zheng WT, Kong DL, et al. (2011) Dual enzymes regulate the molecular self-assembly of tetra-peptide derivatives. Soft Matter 7: 10443-10448. |
[41] | Li J, Shi JF, Medina JE, et al. (2017) Selectively inducing cancer cell death by intracellular enzyme-instructed self-assembly (EISA) of dipeptide derivatives. Adv Healthc Mater 6: 1601400. |
[42] | Gao Y, Berciu C, Kuang Y, et al. (2013) Probing nanoscale self-assembly of nonfluorescent small molecules inside live mammalian cells. ACS Nano 7: 9055-9063. |
[43] | Gao Y, Kuang Y, Du XW, et al. (2013) Imaging self-assembly dependent spatial distribution of small molecules in a cellular environment. Langmuir 29: 15191-15200. |
[44] | Gao Y, Shi JF, Yuan D, et al. (2012) Imaging enzyme-triggered self-assembly of small molecules inside live cells. Nat Commun 3: 1033. |
[45] | Pappas CG, Sasselli IR, Ulijn RV (2015) Biocatalytic pathway selection in transient tripeptide nanostructur,es. Angew Chem Int Ed 54: 8119-8123. |
[46] | Yang Z, Liang G, Xu B (2008) Enzymatic hydrogelation of small molecules. Acc Chem Res 41: 315-326. |
[47] | Yang ZM, Xu KM, Guo ZF, et al. (2007) Intracellular enzymatic formation of nanofibers results in hydrogelation and regulated cell death. Adv Mater 19: 3152-3156. |
[48] | Yang Z, Liang G, Guo Z, et al. (2007) Intracellular hydrogelation of small molecules inhibits bacterial growth. Angew Chem Int Ed 46: 8216-8219. |
[49] | Zhou J, Du XW, Li J, et al. (2015) Taurine boosts cellular uptake of small D-peptides for enzyme-instructed intracellular molecular self-assembly. J Am Chem Soc 137: 10040-10043. |
[50] | Li J, Kuang Y, Shi JF, et al. (2015) Enzyme-instructed intracellular molecular self-assembly to boost activity of cisplatin against drug-resistant ovarian cancer cells. Angew Chem Int Ed 54: 13307-13311. |
[51] | Williams RJ, Smith AM, Collins R, et al. (2009) Enzyme-assisted self-assembly under thermodynamic control. Nat Nanotechnol 4: 19-24. |
[52] | Hughes M, Frederix PWJM, Raeburn J, et al. (2012) Sequence/structure relationships in aromatic dipeptide hydrogels formed under thermodynamic control by enzyme-assisted self-assembly. Soft Matter 8: 5595-5602. |
[53] | Li J, Bullara D, Du XW, et al. (2018) Kinetic analysis of nanostructures formed by enzyme-instructed intracellular assemblies against cancer cells. ACS Nano 12: 3804-3815. |
[54] | Webber MJ, Newcomb CJ, Bitton R, et al. (2011) Switching of self-assembly in a peptide nanostructure with a specific enzyme. Soft Matter 7: 9665-9672. |
[55] | Adler-Abramovich L, Reches M, Sedman VL, et al. (2006) Thermal and chemical stability of diphenylalanine peptide nanotubes: Implications for nanotechnological applications. Langmuir 22: 1313-1320. |
[56] | Kol N, Adler-Abramovich L, Barlam D, et al. (2005) Self-assembled peptide nanotubes are uniquely rigid bioinspired supramolecular structures. Nano Lett 5: 1343-1346. |
[57] | Sedman VL, Adler-Abramovich L, Allen S, et al. (2006) Direct observation of the release of phenylalanine from diphenylalanine nanotubes. J Am Chem Soc 128: 6903-6908. |
[58] | Gopin A, Ebner S, Attali B, et al. (2006) Enzymatic activation of second-generation dendritic prodrugs: Conjugation of self-immolative dendrimers with poly(ethylene glycol) via click chemistry. Bioconjugate Chem 17: 1432-1440. |
[59] | Kim IK, Kim SH, Choi SM, et al. (2016) Extracellular vesicles as drug delivery vehicles for rheumatoid arthritis. Curr Stem Cell Res Thera 11: 329-342. |
[60] | Jyothi NVN, Prasanna PM, Sakarkar SN, et al. (2010) Microencapsulation techniques, factors influencing encapsulation efficiency. J Microencapsul 27: 187-197. |
[61] | Fonseca SB, Pereira MP, Kelley SO (2009) Recent advances in the use of cell-penetrating peptides for medical and biological applications. Adv Drug Deliver Rev 61: 953-964. |
[62] | Gong ZY, Liu XY, Dong JH, et al. (2019) Transition from vesicles to nanofibres in the enzymatic self-assemblies of an amphiphilic peptide as an antitumour drug carrier. Nanoscale 11: 15479-15486. |
[63] | Kuang Y, Shi JF, Li J, et al. (2014) Pericellular hydrogel/nanonets inhibit cancer cells. Angew Chem Int Ed 53: 8104-8107. |
[64] | Criado-Gonzalez M, Rodon Fores J, Wagner D, et al. (2019) Enzyme-assisted self-assembly within a hydrogel induced by peptide diffusion. Chem Commun 55: 1156-1159. |
[65] | Maeda Y, Javid N, Duncan K, et al. (2014) Discovery of catalytic phages by biocatalytic self-assembly. J Am Chem Soc 136: 15893-15896. |
[66] | Wang HM, Feng ZQQ, Wang YZ, et al. (2016) Integrating enzymatic self-assembly and mitochondria targeting for selectively killing cancer cells without acquired drug resistance. J Am Chem Soc 138: 16046-16055. |
[67] | Pires RA, Abul-Haija YM, Costa DS, et al. (2015) Controlling cancer cell fate using localized biocatalytic self-assembly of an aromatic carbohydrate amphiphile. J Am Chem Soc 137: 576-579. |
[68] | Feng ZQQ, Han XG, Wang HM, et al. (2019) Enzyme-instructed peptide assemblies selectively inhibit bone tumors. Chem 5: 2442-2449. |
[69] | Piepenbrock MOM, Lloyd GO, Clarke N, et al. (2010) Metal-and anion-binding supramolecular gels. Chem Rev 110: 1960-2004. |
[70] | Yamamoto Y, Fukushima T, Suna Y, et al. (2006) Photoconductive coaxial nanotubes of molecularly connected electron donor and acceptor layers. Science 314: 1761-1764. |
[71] | Daniel C, Makereel F, Herz LM, et al. (2005) The effects of supramolecular assembly on exciton decay rates in organic semiconductors. J Chem Phys 123: 084902. |
[72] | Stone DA, Hsu L, Stupp SI (2009) Self-assembling quinquethiophene-oligopeptide hydrogelators. Soft Matter 5: 1990-1993. |
[73] | Ghadiri MR, Granja JR, Milligan RA, et al. (1993) Self-assembling organic nanotubes based on a cyclic peptide architecture. Nature 366: 324-327. |
[74] | Li C, Ly J, Lei B, et al. (2004) Data storage studies on nanowire transistors with self-assembled porphyrin molecules. J Phys Chem B 108: 9646-9649. |
[75] | Xu HX, Das AK, Horie M, et al. (2010) An investigation of the conductivity of peptide nanotube networks prepared by enzyme-triggered self-assembly. Nanoscale 2: 960-966. |
[76] | Xu XD, Lin BB, Feng J, et al. (2012) Biological glucose metabolism regulated peptide self-assembly as a simple visual biosensor for glucose detection. Macromol Rapid Comm 33: 426-431. |
[77] | Bremmer SC, Chen J, McNeil AJ, et al. (2012) A general method for detecting protease activity via gelation and its application to artificial clotting. Chem Commun 48: 5482-5484. |
1. | Uttam Ghosh, Goutam Ghosh, 2022, Chapter 10, 978-3-031-21899-6, 241, 10.1007/978-3-031-21900-9_10 | |
2. | Marilisa Pia Dimmito, Lisa Marinelli, Ivana Cacciatore, Anna Lucia Valeri, Alessandra Rapino, Antonio Di Stefano, Self-assembling Peptides (SAPs) as Powerful Tools for the Preparation of Antimicrobial and Wound-Healing Nanostructures, 2024, 21, 15701808, 2232, 10.2174/1570180820666230726164112 | |
3. | Manas Kumar Pradhan, Surya Suresh Puthenpurackal, Aasheesh Srivastava, Enzymatic Dimerization‐Induced Self‐Assembly of Alanine‐Tyramine Conjugates into Versatile, Uniform, Enzyme‐Loaded Organic Nanoparticles, 2024, 63, 1433-7851, 10.1002/anie.202314960 | |
4. | Manas Kumar Pradhan, Surya Suresh Puthenpurackal, Aasheesh Srivastava, Enzymatic Dimerization‐Induced Self‐Assembly of Alanine‐Tyramine Conjugates into Versatile, Uniform, Enzyme‐Loaded Organic Nanoparticles, 2024, 136, 0044-8249, 10.1002/ange.202314960 | |
5. | K. Sivasankari, R. Naveenkumar, B. Karthikeyan, Photobleaching of face mask dyes BB and CR using novel BPPNTs-V2O5 nanotube composites: DFT studies of self-assembly and nanoclusters, 2024, 19, 1751-231X, 281, 10.2166/wpt.2024.026 |