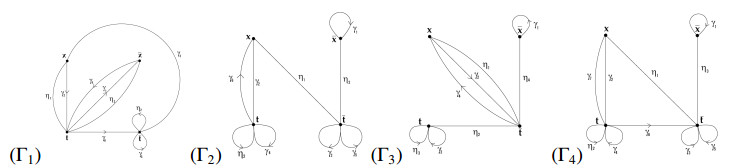
In this research, we constructed a class of nonlinear greedy average block Kaczmarz methods to solve nonlinear problems without computing the Moore-Penrose pseudoinverse of the Jacobian matrix. These kinds of methods adopt the average technique of the Gaussian Kaczmarz method and combine the greedy strategy, which greatly reduces the amount of computation. The local convergence analysis and numerical experiments of the proposed methods are given. The numerical results show the effectiveness of the proposed methods.
Citation: Ying Lv, Li-Li Xing, Wen-Di Bao, Wei-Guo Li, Zhi-Wei Guo. A class of pseudoinverse-free greedy block nonlinear Kaczmarz methods for nonlinear systems of equations[J]. Networks and Heterogeneous Media, 2024, 19(1): 305-323. doi: 10.3934/nhm.2024014
[1] | Cenap Özel, Habib Basbaydar, Yasar Sñzen, Erol Yilmaz, Jung Rye Lee, Choonkil Park . On Reidemeister torsion of flag manifolds of compact semisimple Lie groups. AIMS Mathematics, 2020, 5(6): 7562-7581. doi: 10.3934/math.2020484 |
[2] | Yunfeng Tang, Huixin Yin, Miaomiao Han . Star edge coloring of $ K_{2, t} $-free planar graphs. AIMS Mathematics, 2023, 8(6): 13154-13161. doi: 10.3934/math.2023664 |
[3] | Xiuhai Fei, Zhonghua Wang, Cuixian Lu, Haifang Zhang . Higher Jordan triple derivations on $ * $-type trivial extension algebras. AIMS Mathematics, 2024, 9(3): 6933-6950. doi: 10.3934/math.2024338 |
[4] | Gurninder S. Sandhu, Deepak Kumar . A note on derivations and Jordan ideals of prime rings. AIMS Mathematics, 2017, 2(4): 580-585. doi: 10.3934/Math.2017.4.580 |
[5] | Jung-Chao Ban, Chih-Hung Chang . Entropy dimension of shifts of finite type on free groups. AIMS Mathematics, 2020, 5(5): 5121-5139. doi: 10.3934/math.2020329 |
[6] | Limin Liu, Hongjin Liu . Consistent pairs of $ \mathfrak{s} $-torsion pairs in extriangulated categories with negative first extensions. AIMS Mathematics, 2024, 9(1): 1494-1508. doi: 10.3934/math.2024073 |
[7] | Jin Cai, Shuangliang Tian, Lizhen Peng . On star and acyclic coloring of generalized lexicographic product of graphs. AIMS Mathematics, 2022, 7(8): 14270-14281. doi: 10.3934/math.2022786 |
[8] | Rashad Abdel-Baky, Mohamed Khalifa Saad . Some characterizations of dual curves in dual 3-space $ \mathbb{D}^{3} $. AIMS Mathematics, 2021, 6(4): 3339-3351. doi: 10.3934/math.2021200 |
[9] | Jin Yang, Zhenzhen Wei . The optimal problems for torsional rigidity. AIMS Mathematics, 2021, 6(5): 4597-4613. doi: 10.3934/math.2021271 |
[10] | Hui Chen . Characterizations of normal cancellative monoids. AIMS Mathematics, 2024, 9(1): 302-318. doi: 10.3934/math.2024018 |
In this research, we constructed a class of nonlinear greedy average block Kaczmarz methods to solve nonlinear problems without computing the Moore-Penrose pseudoinverse of the Jacobian matrix. These kinds of methods adopt the average technique of the Gaussian Kaczmarz method and combine the greedy strategy, which greatly reduces the amount of computation. The local convergence analysis and numerical experiments of the proposed methods are given. The numerical results show the effectiveness of the proposed methods.
Consider a non trivial group A and take an element t not in A. Consider an equation,
s(t)=a1tp1a2tp2...antpn=1, |
where, ajϵA, pj = ±1 and aj≠ 1 if it appears between two cyclically adjacent t having exponents with different signs. The equation s(t)=1 is solvable over the group A if it has a solution in some group extension of A. Precisely, let G be a group containing A, if there exist an injective homomorphism, say λ:A→G, such that, g1gp1g2gp2⋯gngpn=1 in G, where gj=λ(aj) and g∈G then the equation s(t)=1 is solvable over A. A conjecture stated in [2] by Levin asserts that if A is torsion- free, then every equation over A is solvable. Bibi and Edjvet [1], using in particular results of Prishchepov [3], has shown that the conjecture is true for equations of length at most seven. Here we prove the following: Theorem: The singular equation,
s(t)=atbt−1ctdtet−1ftgt−1ht−1=1, |
of length eight is solvable over any torsion-free group.
Levin showed that any equation over a torsion-free group is solvable if the exponent sum p1+p2⋯+pn is same as length of the equation. Stallings proved that any equation of the form a1ta2t⋯amtb1t−1b2t−1⋯bnt−1=1, is solvable over torsion-free group. Klyachko showed that any equation with exponent sum ±1 is solvable over torsion free group.
All necessary definitions concerning relative presentations and pictures can be found in [4]. A relative group presentation P is a triplet <A,t|r> where A is a group, t is disjoint from A and r is a set of cyclically reduced words in A∗<t>. If the relative presentation P is orientable and aspherical then the natural homomorphism A→G is injective. P is orientable and aspherical implies s(t)=1 is solvable. We use weighttest to establish asphericity. The star graph Γ of a relative presentation P=<A,t|r> is a directed graph with vertex set {t,t−1} and edges set r∗, the set of all cyclic permutations of elements of {r,r−1} which begin with t or t−1. S∈r∗ write S=Tαj where αj∈A and T begins and ends with t or t−1. The initial function i(S) is the inverse of last symbol of T and terminal function τ(S) is the first symbol of T. The labeling function on the edges is defined by π(S)=αj and is extended to paths in the usual way. A weight function θ on the star graph Γ is a real valued function given to the edges of Γ. If d is an edge of Γ, then θ(d) = θ(ˉd). A weight function θ is aspherical if the following three conditions are satisfied:
(1) Let R ϵr be cyclically reduced word, say R=tϵ11a1...tϵnnan, where ϵi = ±1 and ai∈ A. Then,
n∑ι=1(1−θ(tϵιιaι...tϵnnantϵ11a1...tϵι−1ι−1aι−1))≥2. |
(2) Each admissible cycle in Γ has weight at least 2.
(3) Each edge of Γ has a non-negative weight.
The relative presentation P is aspherical if Γ admits an aspherical weight function. For convenience we write s(t)=1 as,
s(t)=atbt−1ctdtet−1ftgt−1ht−1=1, |
where a,b,c,e,f,g∈A/{1} and d,h∈A. Moreover, by applying the transformation y=td, if necessary, it can be assumed without any loss that d=1 in A.
(1) Since c(2, 2, 3, 3, 3, 3, 3, 3) = 0, so at least 3 admissible cycles must be of order 2.
(2) ac and ac−1 implies c2 = 1, a contradiction.
(3) af and af−1 implies f2 = 1, a contradiction.
(4) be and be−1 implies e2 = 1, a contradiction.
(5) bg and bg−1 implies g2 = 1, a contradiction.
(6) If two of ac, af and cf−1 are admissible then so is the third.
(7) If two of ac−1, af−1 and cf−1 are admissible then so is the third.
(8) If two of ac,cf and af−1 are admissible then so is the third.
(9) If two of ac−1, af and cf are admissible then so is the third.
(10) If two of be,eg, bg−1 are admissible then so is the third.
(11) If two of be−1, eg and bg are admissible then so is the third.
(1) a=c−1,b=e−1
(2) a=c−1,b=g−1
(3) a=c−1,e=g−1
(4) a=c−1,d=h−1
(5) a=c−1,b=e
(6) a=c−1,b=g
(7) a=c,b=g−1
(8) a=c,e=g−1
(9) a=c,d=h−1
(10) a=c,b=e
(11) a=c,b=g
(12) a=c,e=g
(13) a=f−1,b=g−1
(14) a=f−1,d=h−1
(15) a=f−1,b=g
(16) c=f−1,b=e−1
(17) c=f−1,b=g−1
(18) c=f−1,d=h−1
(19) c=f−1,b=e
(20) c=f−1,b=g
(21) c=f−1,e=g
(22) a=f,d=h−1
(23) a=f,b=e
(24) a=f,b=g
(25) c=f,b=g−1
(26) c=f,e=g−1
(27) c=f,d=h−1
(28) c=f,b=e
(29) b=e,d=h−1
(30) a=c−1,a=f−1,d=h−1
(31) a=c−1,a=f−1,c=f
(32) a=c−1,a=f,c=f−1
(33) a=c−1,c=f−1,d=h−1
(34) a=c−1,c=f,d=h−1
(35) a=c,a=f,c=f
(36) a=c,a=f−1,c=f−1
(37) a=f−1,c=f−1,d=h−1
(38) a=f−1,c=f,d=h−1
(39) a=f,c=f−1,d=h−1
(40) a=f,c=f,d=h−1
(41) b=e−1,b=g−1,d=h−1
(42) b=e−1,b=g−1,e=g
(43) b=e,b=g−1,e=g−1
(44) b=e−1,b=g,e=g−1
(45) b=e−1,b=g,d=h−1
(46) b=g−1,e=g−1,d=h−1
(47) b=g−1,e=g,d=h−1
(48) b=g,e=g−1,d=h−1
(49) b=g,e=g,d=h−1
(50) a=c−1,a=f,c=f−1,d=h−1
(51) a=c−1,a=f−1,c=f,d=h−1
(52) a=c−1,b=e,b=g−1,d=h−1
(53) a=c,a=f−1,c=f−1,d=h−1
(54) a=c,a=f,c=f,d=h−1
(55) b=e−1,b=g−1,e=g,d=h−1
(56) a=c−1,b=e,b=g−1,e=g−1,d=h−1
(57) a=c,a=f,c=f,b=e−1,d=h−1
(58) a=c,a=f,c=f,b=e,d=h−1
(59) c=f,b=e,b=g−1,e=g−1,d=h−1
(60) a=c,a=f−1,c=f−1,b=e−1,b=g−1,d=h−1
(61) a=c,a=f,c=f,b=e−1,b=g−1,d=h−1
(62) a=f−1,c=f,b=e−1,b=g,e=g−1,d=h−1
(63) a=f,c=f−1,b=e,b=g−1,e=g−1,d=h−1
(64) a=f,c=f−1,b=e−1,b=g,e=g−1,d=h−1
(65) a=c−1,a=f,c=f−1,b=e,b=g−1,e=g−1,d=h−1
(66) a=c−1,a=f−1,c=f,b=e−1,b=g,e=g−1,d=h−1
(67) a=c,a=f,c=f,b=e−1,b=g,e=g−1,d=h−1
Recall that P=<A,t|s(t)>=1 where s(t)=atbt−1ctdtet−1ftgt−1ht−1=1 and a,b,c,e,f,g∈A/{1} and d,h∈A. We will show that some cases of the given equation are solvable by applying the weight test. Also the transformation y=td, leads to the assumption that d=1 in A.
In this case the relative presentation P is given as :
P=<A,t|s(t)=atbt−1ctdtet−1ftgt−1ht−1>
= <A,t|s(t)=c−1te−1t−1ct2et−1ftgt−1ht−1=1>.
Suppose, x=tet−1 which implies that x−1=te−1t−1.
Using above substitutions in relative presentation we have new presentation
Q=<A,t,x|t−1c−1x−1ctxftgt−1h=1=x−1tet−1>. Observe that in new presentation Q we have two relators R1=t−1c−1x−1ctxftgt−1h and R2=x−1tet−1. The star graph Γ1 for new presentation Q is given by the Figure 1.
Let γ1↔c−1,γ2↔c,γ3↔1,γ4↔f,γ5↔g,γ6↔h and η1↔1,η2↔e,η3↔1.
Assign weights to the edges of the star graph Γ1 as follows:
θ(γ1)=θ(γ2)=θ(η2)=1 and θ(γ3)=θ(γ4)=θ(γ5)=θ(γ5)=θ(γ6)=θ(η1)=θ(η3)=12. The given weight function θ is aspherical and all three conditions of weight test are satisfied.
(1) Observe that, ∑(1−θ(γj))=6−(1+1+12+12+12)+12)=2 and ∑(1−θ(ηj))=3−(0+1+0)=2.
(2) Each admissible cycle having weight less than 2 leads to a contraction. For example, γ3γ6η1 is a cycle of length 2 having weight less than 2, which implies h=1, a contradiction.
(3) Each edge has non-negative weight.
In this case we have two relators R1=t−1atbt−1a−1t2et−1ftb−1t−1h and R2=ta−1t−1. The star graph Γ2 for new presentation Q is given by the Figure 1.
(1) Observe that, ∑(1−θ(γj))=6−(0+1+1+12+1+12)=2 and ∑(1−θ(ηj))=3−(0+1+1)=2.
(2) Each admissible cycle having weight less than 2 leads to a contradiction. For example γ6γ3η1 is a cycle of length 2, having weight less than 2, which implies h=1, a contradiction.
(3) Each edge has non-negative weight.
In this case we have two relators R1=xbx−1tg−1t−1ftgt−1h and R2=x−1t−1at. The star graph Γ2 for new presentation Q is given by the Figure 1.
(1) Observe that, ∑(1−θ(γj))=6−(0+1+1+12+1+12)=2 and ∑(1−θ(ηj))=3−(0+1+0)=2.
(2) Each admissible cycle having weight less than 2 leads to a contradiction. For example, γ5η3γ1η3 is a cycles of length 2 having weight less than 2, which implies b=g−1, a contradiction.
(3) Each admissible cycle has non-negative weight.
In this case we have two relators R1=xbx−1et−1ftg and R2=t−1a−1t2. The star graph Γ3 for the relative presentation is given by the Figure 1.
(1) Observe that ∑(1−θ(γj))=4−(0+1+12+12)=2 and ∑(1−θ(ηj))=4−(0+12+1+12)=2.
(2) Each possible admissible cycle has weight atleast 2, so θ admits an aspherical weight function.
(3) Each edge has non-negative weight.
In this case we have two relators R1=xbx−1tbt−1ftgt−1ht−1 and R2=x−1t−1at. The star graph Γ4 for the relative presentation is given by the Figure 1.
(1) Observe that ∑(1−θ(γj))=7−(0+1+1+1+12+12+1)=2 and ∑(1−θ(ηj))=3−(0+12+1+12)=2.
(2) Each admissible cycle having weight less than 2 leads to a contradiction. For example, γ5η3γ1η3 is a cycle of length 2 having weight less than 2, which implies g=b−1 a contradiction.
(3) Each edge has non-negative weight.
In this case we have two relators R1=t−1axa−1t2et−1fxh and R2=x−1tb−1t−1. The star graph Γ5 for the relative presentation Q is given by the Figure 2.
(1) Observe that, ∑(1−θ(γj))=6−(12+1+12+1+12+12)=2 and ∑(1−θ(ηj))=3−(12+12+0)=2.
(2) Each admissible cycle having weight less than 2 leads to a contradiction. For example, γ6γ3η3 is a cycle of length 2 having weight less than 2, which implies h=1, a contradiction.
(3) Each edge has non-negative weight,
In this case we have two relators R1=xg−1xtet−1ftgt−1h and R2=x−1t−1ct. The star graph Γ6 for the relative presentation is given by the Figure 2.
(1) Observe that, ∑(1−θ(γj))=6−(32+12+1+12+12+0)=2 and ∑(1−θ(ηj))=3−(0+1+0)=2
(2) Each admissible cycle having weight less than 2 leads to a contradiction, For example, the cycle γ1η1η3, γ6η1η3γ2 and γ6η1η3γ1 are the cycles of length 2 having weight less than 2, which implies g=1, h=1 and h=g, a contradiction.
(3) Each edge has non-negative weight.
In this case we have two relators R1=xht−1atbt−1atx−1f and R2=x−1tgt−1. The star graph Γ7 for the relative presentation Q is given by the Figure 2.
(1) Observe that, ∑(1−θ(γj))=6−(12+1+1+1+12+0)=2 and ∑(1−θ(ηj))=3−(12+12+0)=2.
(2) Each possible admissible cycle having weight less than 2 leads to a contradiction. For Example η2γ5γ1 is a cycle of length 2 having weight less than 2, which implies g=h−1, a contradiction.
(3) Each edge has non-negative weight.
In this case we have two relators R1=t−1xbxtet−1ftg and R2=x−1t−1at. The star graph Γ8 for the relative presentation is given by the Figure 2.
(1) Observe that, ∑(1−θ(γj))=6−(12+32+0+12+12+1)=2 and ∑(1−θ(ηj))=3−(0+1+0)=2.
(2) Each edge having weight less than 2 leads to a contradiction. For example, γ2η1η3 is a cycle of length 2 having weight less than 2, which implies b=1, a contradiction.
(3) Each edge has non-negative weight.
In this case we have two relators R1=t−1axatxftgt−1h and R2=x−1tb−1t−1. The star graph Γ9 for the relative presentation is given by the Figure 3.
(1) Observe that, ∑(1−θ(γj))=6−(1+12+12+12+1+12)=2 and ∑(1−θ(ηj))=3−(0+12+12)=2.
(2) Each edge having weight less than 2 leads to a contradiction. For example, γ6γ5η1 is a cycles of length 2 having weight less than 2, which implies that h=1, a contradiction.
(3) Each edge has non-negative weight.
In this case we have two relators R1=t−1axat2et−1fxh and R2=x−1tgt−1. The star graph Γ10 for the relative presentation is given by the Figure 3.
(1) Observe that, ∑(1−θ(γj))=6−(12+1+12+1+12+12)=2 and ∑(1−θ(ηj))=3−(12+12+0)=2.
(2) Each edge having weight less than 2 leads to a contradiction. For example, γ6γ3η3 is a cycles of length 2 having weight less than 2, which implies that h=1, a contradiction.
(3) Each edge has non-negative weight.
In this case we have two relators R1=t−1ctbt−1axfx−1th and R2=x−1t2et−1. The star graph Γ11 for the relative presentation is given by the Figure 3.
(1) Observe that ∑(1−θ(γj))=6−(1+12+0+12+1+1)=2 and ∑(1−θ(ηj))=4−(1+0+12+12)=2.
(2) Each possible admissible cycle has weight atleast 2, so θ admits an aspherical weight function.
(3) Each edge has non-negative weight.
In this case we have two relators R1=xbt−1ct2ex−1b−1t−1h and R2=x−1t−1at. The star graph Γ12 for the relative presentation is given by the Figure 3.
(1) Observe that, ∑(1−θ(γj))=6−(12+12+0+1+1+1)=2 and ∑(1−θ(ηj))=3−(0+1+0)=2.
(2) Each edge having weight less than 2 leads to a contradiction. For example, γ6γ3η1 is a cycles of length 2 having weight less than 2, which implies that h=1, a contradiction.
(3) Each edge has non negative weight.
In this case we have two relators R1=t−1xbt−1ct2exg and R2=x−1t−1ft. The star graph Γ13 for the relative presentation is given by the Figure 4.
(1) ∑(1−θ(γj))=6−(12+12+1+1+1+0)=2 and ∑(1−θ(ηj))=3−(0+12+12)=2.
(2) Each edge having weight less than 2 leads to a contradiction. For example, γ6γ4γ1 amd γ6γ5η1η3 are the cycles of length 2 having weight less than 2, which implies g=1 and g=e−1, a contradiction.
(3) Each edge has non-negative weight.
In this case we have two relators R1=xbt−1ct2ex−1bt−1h and R2=x−1t−1at. The star graph Γ13 for the relative presentation Q is given by the Figure 4.
(1) Observe that, ∑(1−θ(γj))=6−(12+1+1+12+1+0)=2 and ∑(1−θ(ηj))=3−(0+12+12)=2.
(2) Each admissible cycle having weight less than 2 leads to a contradiction. For example, γ6η1γ3 is a cycle of length 2 having weight less than 2, which implies h=1, a contradiction.
(3) Each edge has non-negative weight.
In this case we have two relators R1=t−1ac−1tx−1ctxgt−1h and R2=x−1tb−1t−1c−1t. The star graph Γ14 for the relative presentation Q is given by the Figure 4.
(1) Observe that, ∑(1−θ(γj))=6−(1+12+1+1+12+0)=2 and ∑(1−θ(ηj))=4−(0+1+12+12)=2.
(2) Each admissible cycle having weight less than 2 leads to a contradiction. For example, γ6η1γ4 is a cycles of length 2, having weight less than 2, which implies h=1 a contradiction.
(3) Each edge has non-negative weight.
In this case we have two relators R1=t−1axtex−1h and R2=x−1tbt−1ct. The star graph Γ15 for the relative presentation Q is given by the Figure 4.
(1) Observe that, ∑(1−θ(γj))=4−(1+0+1+0)=2 and ∑(1−θ(ηj))=4−(1+0+0+1)=2.
(2) Each admissible cycle having weight less than 2 leads to a contradiction. For example, γ4η4γ2γ1 is a cycle of length 2 having weight less than 2, which implies h=1, a contradiction.
(3) Each edge has non-negative weight.
In this case we have two relators R1=t−2atbx−1texg and R2=x−1t−1et−1c−1t. The star graph Γ16 for the relative presentation Q is given by the Figure 4.
(1) ∑(1−θ(γj))=4−(1+1+12+12+12)=2 and ∑(1−θ(ηj))=4−(12+12+12+12)=2.
(2) Each admissible cycle has weight atleast 2, so θ admits an aspherical weight function.
(3) Each edge has non-negative weight.
In this case we have two relators R1=t−1atbxtbx−1gt−1h and R2=x−1t−1ct. The star graph Γ17 for the relative presentation Q is given by the Figure 5.
(1) ∑(1−θ(γj))=6−(1+12+1+12+1+12+12)=2 and ∑(1−θ(ηj))=3−(12+12+0)=2.
(2) Each admissible cycle having weight less than 2 leads to a contradiction. For example, γ6η3γ3 is a cycle of length 2 having weight less than 2, which implies h=1, a contradiction.
(3) Each edge has non-negative weight.
In this case we have two relators R1=t−1axct2et−1c−1tbt−1h and R2=x−1tbt−1. The star graph Γ18 for the relative presentation Q is given by the Figure 5.
(1) Observe that, ∑(1−θ(γj))=6−(12+1+12+1+12+12)=2 and ∑(1−θ(ηj))=3−(12+12+0)=2.
(2) Each admissible cycle having weight less than 2 leads to a contradiction. For example, γ6γ3η3 is a cycle of length 2 having weight less than 2, which implies h=1 a contradiction.
(3) Each edge has non-negative weight.
In this case we have two relators R1=t−1atbxtex−1et−1h and R2=x−1t−1ct. The star graph Γ19 for the relative presentation Q is given by the Figure 5.
(1) Observe that, ∑(1−θ(γj))=6−(12+1+12+1+12+12)=2 and ∑(1−θ(ηj))=3−(0+1+0)=2.
(2) Each admissible cycle having weight less than 2 leads to a contradiction. For example, γ6η3γ3 is a cycle of length 2 having weight less than 2, which implies h=1, a contradiction.
(3) Each edge has non-negative weight.
In this case we have two relators R1=t−1xbt−1ct2exg and R2=x−1t−1at. The star graph Γ20 for the Q relative presentation is given by the Figure 5.
(1) Observe that, ∑(1−θ(γj))=6−(1+12+1+1+0+12)=2 and ∑(1−θ(ηj))=3−(0+12+12)=2.
(2) Each possible admissible cycle has weight atleast 2, so θ admits an aspherical weight function.
(3) Each edge has non-negative weight.
In this case we have two relators R1=t−1axctxatget−1h and R2=x−1tbt−1.
The star graph Γ21 for the relative presentation Q is given by the Figure 6.
(1) Observe that, ∑(1−θ(γj))=6−(12+12+1+12+12+1)=2 and ∑(1−θ(ηj))=3−(0+12+12)=2.
(2) Each admissible cycle having weight less than 2 leads to a contradiction. For example, γ6γ3η1, is a cycle of length 2 having weight less than 2, which implies h=1 a contradiction.
(3) Each edge has non-negative weight.
In this case we have two relators R1=xct2exh and R2=x−1t−1atbt−1. The star graph Γ22 for the relative presentation Q is given by the Figure 6.
(1) Observe that, ∑(1−θ(γj))=4−(12+12+12+12)=2 and ∑(1−θ(ηj))=4−(12+12+12+12)=2.
(2) Each admissible cycle having weight less than 2 leads to a contradiction. For example, γ23η1 is a cycle of length 2, having weight less than 2, which implies e2=1, a contradiction.
(3) Each edge has non-negative weight.
In this case we have two relators R1=t−1atg−1xtexgt−1h and R2=x−1t−1ft>. The star graph Γ23 for the relative presentation is given by the Figure 6.
(1) Observe that, ∑(1−θ(γj))=6−(1+12+12+12+1+12)=2 and ∑(1−θ(ηj))=3−(12+12+0)=2.
(2) Each admissible cycle having weight less than 2 leads to a contradiction. For example, γ6η3γ3 is a cycle of length 2 having weight less than 2, which implies h=1, a contradiction.
(3) Each edge has non-negative weight.
In this case we have two relators R1=t−1atbxtexe−1t−1h and R2=x−1t−1ct. The star graph Γ23 for the relative presentation Q is given by the Figure 6.
(1) Observe that, ∑(1−θ(γj))=6−(1+12+12+12+1+12)=2 and ∑(1−θ(ηj))=3−(12+12+0)=2.
(2) Each admissible cycle having weight less than 2 leads to a contradiction. For example, γ6η3γ3 has weight less than 2, which implies h=1, a contradiction.
(3) Each edge has non-negative weight.
In this case we have two relators R1=t−2atbxtexg and R2=x−1t−1ct. The star graph Γ24 for the relative presentation Q is given by the Figure 6.
(1) Observe that, ∑(1−θ(γj))=6−(1+12+1+1+12+0)=2 and ∑(1−θ(ηj))=3−(0+1+0)=2.
(2) Each possible admissible cycle has weight at least 2, so θ admits an aspherical weight function.
(3) Each edge has non-negative weight.
In this case we have two relators R1=t−1axt−1cxctgt−1h and R2=x−1t2bt−1. The star graph Γ25 for the relative presentation Q is given by the Figure 7.
(1) ∑(1−θ(γj))=4−(12+12+12+12)=2 and ∑(1−θ(ηj))=4−(12+12+12+12)=2.
(2) Each possible admissible cycle has weight atleast 2, so θ admits an aspherical weight function.
(3) Each edge has non-negative weight.
In this case we have two relators R1=xcta−1t2xftg and R2=x−1t−2atbt−1. The star graph Γ26 for the relative presentation Q is given by the Figure 7.
(1) ∑(1−θ(γj))=6−(1+12+1+12+12+12)=2 and ∑(1−θ(ηj))=5−(12+1+12+12+12)=2.
(2) Each possible admissible cycle has weight atleast 2, so θ admits an aspherical weight function.
(3) Each edge has non-negative weight.
In this case we have two relators R1=t−1xbx−1tex−1g and R2=x−1t−1at. The star graph Γ27 for the relative presentation Q is given by the Figure 7.
(1) ∑(1−θ(γj))=5−(1+0+1+1+0)=2 and ∑(1−θ(ηj))=3−(0+0+1)=2.
(2) Each possible admissible cycle has weight atleast 2, so θ admits an aspherical weight function.
(3) Each edge has non-negative weight.
In this case we have two relators R1=t−1axctx−1fte−1t−1h and R2=x−1tbt−1. The star graph Γ27 for the new presentation Q is given by the Figure 7.
(1) Observe that, ∑(1−θ(γj))=5−(1+0+1+1+0)=2 and ∑(1−θ(ηj))=3−(0+0+1)=2.
(2) Each possible admissible cycle has weight atleast 2 so θ admits an aspherical weight function.
(3) Each edge has non-negative weight.
In this case we have two relators R1=xbx−1tex−1gt−1h and R2=x−1t−1at. The star graph Γ27 for the relative presentation Q is given by the Figure 7.
(1) Observe that, ∑(1−θ(γj))=5−(0+1+1+0+1)=2 and ∑(1−θ(ηj))=3−(0+0+1)=2.
(2) Each possible admissible cycle has weight atleast 2, so θ admits an aspherical weight function.
(3) Each edge has non-negative watch.
In this case we have two relators: R1=xbx−1tet−1c−1tgt−1h and R2=x−1t−1at. The star graph Γ28 for the relative presentation Q is given by the Figure 7.
(1) Observe that, ∑(1−θ(γj))=5−(0+1+1+1+12+12)=2 and ∑(1−θ(ηj))=3−(0+12+12)=2.
(2) Each possible admissible cycle having weight less than 2 leads to a contrdiction. For example, γ5η3γ1 is a cycle of length 2 having weight less than 2, which implies g=b−1, a contradiction.
(3) Each edge has non-negative weight.
In this case we have two relators R1=t−1xbx−1texg and R2=x−1t−1at. The star graph Γ29 for the relative presentation Q is given by the Figure 8.
(1) Observe that, ∑(1−θ(γj))=5−(1+0+1+0+1)=2 and ∑(1−θ(ηj))=3−(0+0+1)=2.
(2) Each possible admissible cycle has weight atleast 2, so θ admits an aspherical weight function.
(3) Each edge has non-negative weight.
In this case we have two relators R1=t−1xbx−1tex−1g and R2=x−1t−1at. The star graph Γ30 for the relative presentation Q is given by the Figure 8.
(1) Observe that, ∑(1−θ(γj))=5−(1+0+1+1+0)=2 and ∑(1−θ(ηj))=3−(0+0+1)=2.
(2) Each possible admissible cycle has weight atleast 2, so θ admits an aspherical weight function.
(3) Each edge has non-negative weight.
In this case we have two relators R1=xbxtexgt−1h and R2=x−1t−1at. The star graph Γ31 for the relative presentation Q is given by the Figure 8.
(1) Observe that, ∑(1−θ(γj))=5−(1+0+12+12+1)=2 and ∑(1−θ(ηj))=3−(12+0+12)=2.
(2) Each possible admissible cycle has weight atleast 2, so θ admits an aspherical weight function.
(3) Each edge has non-negative weight.
In this case we have two relators R1=xbxtex−1gt−1h and R2=x−1t−1at. The star graph Γ32 for the relative presentation Q is given by the Figure 8.
(1) Observe that, ∑(1−θ(γj))=5−(0+1+0+1+1)=2 and ∑(1−θ(ηj))=3−(1+0+0)=2.
(2) Each possible admissible cycle has weight atleast 2, so θ admits an aspherical weight function.
(3) Each edge has non-negative weight.
In this case we have two relators R1=t−1xbxtex−1g and R2=x−1t−1at. The star graph Γ33 for the relative presentation Q is given by the Figure 9.
(1) Observe that ∑(1−θ(γj))=5−(1+1+0+12+12)=2 and ∑(1−θ(ηj))=3−(12+0+12)=2.
(2) Each possible admissible cycle has weight atleast 2, so θ admits an aspherical weight function.
(3) Each edge has non-negative weight.
In this case we have two relators R1=t−1xbt−1ct2ex−1g and R2=x−1t−1at. The star graph Γ38 for the relative presentation Q is given by the Figure 9.
(1) Observe that, ∑(1−θ(γj))=6−(1+12+1+1+12+0)=2 and ∑(1−θ(ηj))=3−(0+12+12)=2.
(2) Each admissible cycle having weight less than 2 leads to a contradiction. For example, γ2γ−16η1η3γ5 is the cycle of length 2 having weight less than 2, which leads to a contradiction.
(3) Each edge has non-negative weight.
In this case we have two relators R1=t−1xbx−1texg and R2=x−1t−1at. The star graph Γ35 for the relative presentation Q is given by the Figure 9.
(1) Observe that, ∑(1−θ(γj))=5−(0+1+1+1+0)=2 and ∑(1−θ(ηj))=3−(1+0+0)=2.
(2) Each possible admissible cycle has weight atleast 2, so θ admits an aspherical weight function.
(3) Each edge has non-negative weight.
In this case we have two relators R1=t−1xbx−1tbxb−1 and R2=x−1t−1at. The star graph Γ35 for the relative presentation Q is given by the Figure 9.
(1) Observe that, ∑(1−θ(γj))=5−(1+0+1+0+1)=2 and ∑(1−θ(ηj))=3−(0+0+1)=2.
(2) Each possible admissible cycle has weight atleast 2 so θ admits an aspherical weight function.
(3) Each edge has non-negative weight.
In this case we have two relators R1=t−1xbxtexg and R2=x−1t−1at. The star graph Γ36 for the relative presentation Q is given by the Figure 9.
(1) Observe that, ∑(1−θ(γj))=5−(0+1+1+1+0)=2 and ∑(1−θ(ηj))=3−(1+0+0)=2.
(2) Each possible admissible cycle has weight atleast 2, so θ admits an aspherical weight function.
(3) Each edge has non-negative weight.
In this case we have two relators R1=t−1axctx−1fx−1 and R2=x−1tbt−1. The star graph Γ37 for the relative presentation Q is given by the Figure 10.
(1) Observe that, ∑(1−θ(γj))=5−(12+12+1+1+0)=2 and ∑(1−θ(ηj))=3−(12+12+0)=2.
(2) Each possible admissible cycle has weight atleast 2, so θ admits an aspherical weight function.
(3) Each edge has non-negative weight.
In this case we have two relators R1=t−1axctx−1fx−1h and R2=x−1tbt−1. The star graph Γ37 for the relative presentation Q is given by the Figure 9.
(1) Observe that, ∑(1−θ(γj))=5−(12+12+1+1+0)=2 and ∑(1−θ(ηj))=3−(12+0+12)=2.
(2) Each possible admissible cycle has weight atleast 2, so θ admits an aspherical weight function
(3) Each edge has non-negative weight.
In this case we have two relators R1=t−1axctxfx−1h and R2=x−1tbt−1. The star graph Γ38 for the relative presentation Q is given by the Figure 10.
(1) Observe that, ∑(1−θ(γj))=5−(0+1+1+0+1)=2 and ∑(1−θ(ηj))=3−(0+0+1)=2.
(2) Each possible admissible cycle has weight atleast 2, so θ admits an aspherical weight function.
(3) Each edge has non-negative weight.
In this case we have two relators R1=t−1axctx−1fte−1t−1h and R2=x−1tbt−1. The star graph Γ39 for the relative presentation Q is given by the Figure 10.
(1) Observe that, ∑(1−θ(γj))=6−(12+1+0+12+1+1)=2 and ∑(1−θ(ηj))=3−(12+12+0)=2.
(2) Each admissible cycle having weight less than 2 leads to a contradiction. For example, γ5η1η3 is the cycle of length 2 having weight less than 2, which implies h=1, a contradiction.
(3) Each edge has non-negative weight.
In this case we have two relators R1=t−1axctx−1fx and R2=x−1tbt−1. The star graph Γ40 for the relative presentation Q is given by the Figure 10.
(1) Observe that, ∑(1−θ(γj))=6−(12+1+0+12+1+1)=2 and ∑(1−θ(ηj))=3−(12+12+0)=2.
(2) Each possible admissible cycle has weight atleast 2 so θ admits an aspherical weight function.
(3) Each edge has non-negative weight.
In this case we have two relators R1=|t−1axctxfx−1 and R2=x−1tbt−1. The star graph Γ41 for the relative presentation Q is given by the Figure 11.
(1) Observe that, ∑(1−θ(γj))=6−(12+1+0+12+1+1)=2 and ∑(1−θ(ηj))=3−(12+12+0)=2.
(2) Each possible admissible cycle has weight atleast 2 so θ admits an aspherical weight function.
(3) Each edge has non-negative weight.
In this case we have two relators R1=t−1axa−1txfx−1 and R2=x−1tbt−1. The star graph Γ41 for the relative presentation Q is given by the Figure 11.
(1) Observe that, ∑(1−θ(γj))=5−(0+1+1+0+1)=2 and ∑(1−θ(ηj))=3−(0+0+1)=2.
(2) Each possible admissible cycle has weight atleast 2 so θ admits an aspherical weight function.
(3) Each edge has non-negative weight.
In this case we have two relators R1=x−1t−1axctx−1f and R2=x−1tbt−1. The star graph Γ42 for the relative presentation Q is given by the Figure 11.
(1) Observe that, ∑(1−θ(γj))=5−(0+12+12+1+1)=2 and ∑(1−θ(ηj))=3−(12+0+12)=2.
(2) Each admissible cycle has weight atleast 2 so θ admits an aspherical weight function.
(3) each edge has non-negative weight.
In this case we have two relators R1=xatbt−1cx−1f and R2=x−1tbt−2. The star graph Γ43 for the relative presentation Q is given by the Figure 11.
(1) Observe that, ∑(1−θ(γj))=6−(12+1+0+12+1+1)=2 and ∑(1−θ(ηj))=3−(12+12+0)=2.
(2) Each possible admissible cycle has weight atleast 2 so θ admits an aspherical weight function.
(3) Each edge has non-negative weight.
In this case we have two relators R1=xt−1axctxf and R2=x−1tbt−1. The star graph Γ44 for the relative presentation Q is given by the Figure 11.
(1) Observe that, ∑(1−θ(γj))=5−(12+12+12+12+1)=2 and ∑(1−θ(ηj))=3−(12+0+12)=2.
(2) Each possible admissible cycle has weight atleast 2 so θ admits an aspherical weight function.
(3) Each edge has non-negative weight.
In this case we have two relators R1=xbx−1etxg and R2=x−1t−2c−1t. The star graph Γ45 for the relative presentation Q is given by the Figure 12.
(1) Observe that, ∑(1−θ(γj))=4−(0+1+1+0)=2 and ∑(1−θ(ηj))=4−(1+0+0+1)=2.
(2) Each possible admissible cycle has weight atleast 2 so θ admits an aspherical weight function.
(3) Each edge has non-negative weight.
In this case we have two relators R1=t−1xbxtex−1g and R2=x−1t−1at. The star graph Γ46 for the relative presentation Q is given by the Figure 12.
(1) Observe that, ∑(1−θ(γj))=5−(1+1+0+0+1)=2 and ∑(1−θ(ηj))=3−(1+0+0)=2.
(2) Each possible admissible cycle has weight atleast 2 so θ admits an aspherical weight function.
(3) Each edge has non-negative weight.
In this case we have two relators R1=t−1xbxtexg and R2=x−1t−1at. The star graph Γ47 for the relative presentation Q is given by the Figure 12.
(1) Observe that, ∑(1−θ(γj))=5−(1+1+0+1+0)=2 and ∑(1−θ(ηj))=3−(1+0+0)=2.
(2) Each possible admissible cycle has weight atleast 2 so θ admits an aspherical weight function.
(3) Each edge has non-negative weight.
In this case we have two relators R1=x−1t−1axctx−1f and R2=x−1tbt−1. The star graph Γ48 for the relative presentation Q is given by the Figure 12.
(1) Observe that, ∑(1−θ(γj))=5−(0+0+1+1+1)=2 and ∑(1−θ(ηj))=3−(0+0+0)=2.
(2) Each possible admissible cycle has weight atleast 2 so θ admits an aspherical weight function.
(3) Each edge has non-negative weight.
In this case we have two relators R1=xc−1x−1tcx−1f and R2=x−1tb−1t−2. The star graph Γ49 for the relative presentation Q is given by the Figure 13.
(1) Observe that, ∑(1−θ(γj))=4−(0+1+1+0)=2 and ∑(1−θ(ηj))=4−(1+0+0+1)=2.
(2) Each possible admissible cycle has weight atleast 2 so θ admits an aspherical weight function.
(3) Each edge has non-negative weight.
In this case we have two relators R1=t−1xbxtb−1xg and R2=x−1t−1at. The star graph Γ50 for the relative presentation Q is given by the Figure 13.
(1) Observe that, ∑(1−θ(γj))=5−(12+1+12+1+0)=2 and ∑(1−θ(ηj))=3−(12+0+12=2.
(2) Each admissible cycle having weight less than 2 leads to a contradiction. For example γ2η1γ−15 is a cycle of length 2 having weight less than 2 which implies b=g, a contradiction.
(3) Each edge has non-negative weight.
In this case we have two relators R1=|t−1xbxtbxg and R2=x−1t−1at. The star graph Γ50 for the relative presentation Q is given by the Figure 12.
(1) Observe that, ∑(1−θ(γj))=5−(12+1+12+1+0)=2 and ∑(1−θ(ηj))=3−(12+0+12)=2.
(2) Each admissible cycle having weight less than 2 leads to a contradiction. For example γ2η1γ−15 is a cycle of length 2 having weight less than 2 which implies b=g, a contradiction.
(3) Each edge has non-negative weight.
In this case we have two relators R1=x−1t−1axctxc and R2=x−1tbt−1. The star graph Γ51 for the relative presentation Q is given by the Figure 13.
(1) Observe that, ∑(1−θ(γj))=5−(1+0+0+1+1)=2 and ∑(1−θ(ηj))=3−(0+0+1)=2.
(2) Each possible admissible cycle has weight atleast 2 so θ admits an aspherical weight function.
(3) Each edge has non-negative weight.
In this case we have two relators R1=xat−1x−1atxta−1 and R2=x−1tbt−1t−2. The star graph Γ52 for the relative presentation Q is given by the Figure 13.
(1) Observe that, ∑(1−θ(γj))=6−(0+1+0+1+1+1)=2 and ∑(1−θ(ηj))=4−(0+0+1+1)=2.
(2) Each possible admissible cycle has weight atleast 2 so θ admits an aspherical weight function.
(3) Each edge has non-negative weight.
In this case we have two relators R1=t−1xbxtb−1xb−1 and R2=x−1t−1at. The star graph Γ53 for the relative presentation Q is given by the Figure 14.
(1) Observe that, ∑(1−θ(γj))=5−(12+1+12+1+0)=2 and ∑(1−θ(ηj))=3−(12+0+12)=2.
(2) Each possible admissible cycle has weight atleast 2 so θ admits an aspherical weight function.
(3) Each edge has non-negative weight.
In this case we have two relators R1=xbt−1x−1bxtb−1tb−1 and R2=x−1t−1a−1tbt−1. The star graph Γ54 for the relative presentation Q is given by the Figure 14.
(1) Observe that, ∑(1−θ(γj))=6−(12+1+0+1+12+1)=2 and ∑(1−θ(ηj))=4−(1+0+0+1)=2.
(2) Each possible admissible cycle has weight atleast 2 so θ admits an aspherical weight function.
(3) Each edge has non-negative weight.
In this case we have two relators R1=t−1axa−1txax−1 and R2=x−1tbt−1. The star graph Γ55 for the relative presentation Q is given by the Figure 14.
(1) Observe that, ∑(1−θ(γj))=5−(0+1+1+0+1)=2 and ∑(1−θ(ηj))=3−(0+0+1)=2.
(2) Each possible admissible cycle has weight atleast 2 so θ admits an aspherical weight function.
(3) Each edge has non-negative weight.
In this case we have two relators R1=t−1xbx−1tb−1xb and R2=x−1t−1at. The star graph Γ56 for the relative presentation Q is given by the Figure 14.
(1) Observe that, ∑(1−θ(γj))=5−(1+0+1+0+1)=2 and ∑(1−θ(ηj))=3−(0+0+1)=2.
(2) Each possible admissible cycle has weight atleast 2 so θ admits an aspherical weight function.
(3) Each edge has non-negative weight.
In this case we have two relators R1=t−1xbx−1tb−1x−1b and R2=x−1t−1at. The star graph Γ57 for the relative presentation Q is given by the Figure 14.
(1) Observe that, ∑(1−θ(γj))=5−(1+0+1+1+0)=2 and ∑(1−θ(ηj))=3−(0+0+1)=2.
(2) Each possible admissible cycle has weight atleast 2 so θ admits an aspherical weight function.
(3) Each edge has non-negative weight.
In this case we have two relators R1=t−1axatx−1ax and R2=x−1tbt−1. The star graph Γ58 for the relative presentation Q is given by the Figure 15.
(1) Observe that, ∑(1−θ(γj))=5−(1+0+1+0+1)=2 and ∑(1−θ(ηj))=3−(1+0+0)=2.
(2) Each possible admissible cycle has weight atleast 2 so θ admits an aspherical weight function.
(3) Each edge has non-negative weight.
In all the cases, 3 conditions of weight test are satisfied.
Hence the equation s(t)=atbt−1ctdtet−1ftgt−1ht−1=1 where a,b,c,e,f,g∈A/{1} and d,h∈A is solvable.
In this article, we reviewed some basic concepts of combinatorial group theory (like torsion-free group, equations over groups, relative presentation, weight test) and discussed the two main conjectures in equations over torsion-free groups. We investigated all possible cases and solved the singular equation of length eight over torsion free group by using weight test. This result will be useful in dealing with equations over torsion-free groups.
The authors wish to express their gratitude to Prince Sultan University for facilitating the publication of this article through the Theoretical and Applied Sciences Lab.
All authors declare no conflicts of interest in this paper.
[1] | H. B. An, Z. Z. Bai, Broyden method for nonlinear equation in several variables, Mathematica Numerica Sinica (Chinese Journal), 26 (2004), 385–400. |
[2] |
H. B. An, Z. Z. Bai, Directional secant method for nonlinear equations, J. Comput. Appl. Math., 175 (2005), 291–304. https://doi.org/10.1016/j.cam.2004.05.013 doi: 10.1016/j.cam.2004.05.013
![]() |
[3] |
Z. Z. Bai, L. Wang, On convergence rates of Kaczmarz-type methods with different selection rules of working rows, Appl. Numer. Math., 186 (2023), 289–319. https://doi.org/10.1016/j.apnum.2023.01.013 doi: 10.1016/j.apnum.2023.01.013
![]() |
[4] |
Z. Z. Bai, W. T. Wu, On greedy randomized Kaczmarz method for solving large sparse linear systems, SIAM J. Sci. Comput., 40 (2018), A592–A606. https://doi.org/10.1137/17M1137747 doi: 10.1137/17M1137747
![]() |
[5] |
J. Q. Chen, Z. D. Huang, On the error estimate of the randomized double block Kaczmarz method, Appl. Math. Comput., 370 (2020), 124907. https://doi.org/10.1016/j.amc.2019.124907 doi: 10.1016/j.amc.2019.124907
![]() |
[6] |
Q. P. Chen, W. R. Hao, A homotopy training algorithm for fully connected neural networks, P. Roy. Soc. A-Math. Phy., 475 (2019), 20190662. https://doi.org/10.1098/rspa.2019.0662 doi: 10.1098/rspa.2019.0662
![]() |
[7] |
K. Du, W. T. Si, X. H. Sun, Randomized extended average block Kaczmarz for solving least squares, SIAM J. Sci. Comput., 42 (2020), A3541–A3559. https://doi.org/10.1137/20M1312629 doi: 10.1137/20M1312629
![]() |
[8] | K. Du, X. H. Sun, Pseudoinverse-free randomized block iterative algorithms for consistent and inconsistent linear systems, arXiv: 2011.10353 [preprint], (2020), [cited 2024 March 27]. Available from: https://doi.org/10.48550/arXiv.2011.10353 |
[9] |
K. Du, X. H. Sun, A doubly stochastic block Gauss–Seidel algorithm for solving linear equations, Appl. Math. Comput., 408 (2021), 126373. https://doi.org/10.1016/j.amc.2021.126373 doi: 10.1016/j.amc.2021.126373
![]() |
[10] |
Y. C. Eldar, D. Needell, Acceleration of randomized Kaczmarz method via the Johnson-Lindenstrauss lemma, Numer. Algorithms, 58 (2011), 163–177. https://doi.org/10.1007/s11075-011-9451-z doi: 10.1007/s11075-011-9451-z
![]() |
[11] |
T. Elfving, Block-iterative methods for consistent and inconsistent linear equations, Numer. Math., 35 (1980), 1–12. https://doi.org/10.1007/BF01396365 doi: 10.1007/BF01396365
![]() |
[12] |
M. A. Gomes-Ruggiero, J. M. Martínez, A. C. Moretti, Comparing algorithms for solving sparse nonlinear systems of equations, SIAM J. Sci. Stat. Comput., 13 (1992), 459–483. https://doi.org/10.1137/0913025 doi: 10.1137/0913025
![]() |
[13] |
R. M. Gower, P. Richtárik, Randomized iterative methods for linear systems, SIAM J. Matrix Anal. A., 36 (2015), 1660–1690. https://doi.org/10.1137/15M1025487 doi: 10.1137/15M1025487
![]() |
[14] | S. Karczmarz, Angenaherte auflosung von systemen linearer glei-chungen, Bull. Int. Acad. Pol. Sic. Let., Cl. Sci. Math. Nat., 35 (1937), 355–357. |
[15] | K. Kawaguchi, Deep learning without poor local minima, In: D. D. Lee, M. Sugiyama, U. Luxburg, I. Guyon, R. Garnett, Advances in neural information processing systems, New York: Curran Associates Inc., 29 (2016), 586–594. |
[16] |
J. Liu, S. J. Wright, An accelerated randomized Kaczmarz algorithm, Math. Comput., 85 (2016), 153–178. http://dx.doi.org/10.1090/mcom/2971 doi: 10.1090/mcom/2971
![]() |
[17] | L. Lukšan, Hybrid methods for large sparse nonlinear least squares, J. Optimiz. Theory App., 89 (1996), 575–595. |
[18] |
A. Ma, D. Needell, A. Ramdas, Convergence properties of the randomized extended Gauss–Seidel and Kaczmarz methods, SIAM J. Matrix Anal. A., 36 (2015), 1590–1604. https://doi.org/10.1137/15M1014425 doi: 10.1137/15M1014425
![]() |
[19] |
L. Mirsky, Symmetric gauge functions and unitarily invariant norms, Q. J. Math., 11 (1960), 50–59. https://doi.org/10.1093/qmath/11.1.50 doi: 10.1093/qmath/11.1.50
![]() |
[20] | J. J. Moré, B. S. Garbow, K. E. Hillstrom, Testing unconstrained optimization software, ACM T. Math. Software, 7 (1981), 17–41. |
[21] |
M. S. Morshed, M. S. Islam, M. Noor-E-Alam, Accelerated sampling Kaczmarz Motzkin algorithm for the linear feasibility problem, J. Global Optim., 77 (2020), 361–382. https://doi.org/10.1007/s10898-019-00850-6 doi: 10.1007/s10898-019-00850-6
![]() |
[22] |
I. Necoara, Faster randomized block Kaczmarz algorithms, SIAM J. Matrix Anal. A., 40 (2019), 1425–1452. https://doi.org/10.1137/19M1251643 doi: 10.1137/19M1251643
![]() |
[23] |
D. Needell, Randomized Kaczmarz solver for noisy linear systems, BIT, 50 (2010), 395–403. https://doi.org/10.1007/s10543-010-0265-5 doi: 10.1007/s10543-010-0265-5
![]() |
[24] |
D. Needell, J. A. Tropp, Paved with good intentions: Analysis of a randomized block Kaczmarz method, Linear Algebra Appl., 441 (2014), 199–221. https://doi.org/10.1016/j.laa.2012.12.022 doi: 10.1016/j.laa.2012.12.022
![]() |
[25] |
D. Needell, R. Zhao, A. Zouzias, Randomized block Kaczmarz method with projection for solving least squares, Linear Algebra Appl, 484 (2015), 322–343. https://doi.org/10.1016/j.laa.2015.06.027 doi: 10.1016/j.laa.2015.06.027
![]() |
[26] | J. M. Ortega, W. C. Rheinboldt, Iterative Solution of Nonlinear Equations in Several Variables, Philadelphia: Society for Industrial and Applied Mathematics, 2000. https://doi.org/10.1137/1.9780898719468 |
[27] |
T. Strohmer, R. Vershynin, A randomized Kaczmarz algorithm with exponential convergence, J. Fourier Anal. Appl., 15 (2009) 262–278. https://doi.org/10.1007/s00041-008-9030-4 doi: 10.1007/s00041-008-9030-4
![]() |
[28] |
Q. F. Wang, W. G. Li, W. D. Bao, X. Q. Gao, Nonlinear Kaczmarz algorithms and their convergence, J. Comput. Appl. Math., 399 (2022), 113720. https://doi.org/10.1016/j.cam.2021.113720 doi: 10.1016/j.cam.2021.113720
![]() |
[29] |
X. Z. Wang, M. L. Che, C. X. Mo, Y. M. Wei, Solving the system of nonsingular tensor equations via randomized Kaczmarz-like method, J. Comput. Appl. Math., 421 (2023), 114856. https://doi.org/10.1016/j.cam.2022.114856 doi: 10.1016/j.cam.2022.114856
![]() |
[30] |
A. Q. Xiao, J. F. Yin, N. Zheng, On fast greedy block Kaczmarz methods for solving large consistent linear systems, Comput. Appl. Math., 42 (2023), 119. https://doi.org/10.1007/s40314-023-02232-x doi: 10.1007/s40314-023-02232-x
![]() |
[31] |
R. Yuan, A. Lazaric, R. M. Gower, Sketched Newton-Raphson, SIAM J. Optimiz., 32 (2022), 1555–1583. https://doi.org/10.1137/21M139788X doi: 10.1137/21M139788X
![]() |
[32] |
J. H. Zhang, Y. Q. Wang, J. Zhao, On maximum residual nonlinear Kaczmarz-type algorithms for large nonlinear systems of equations, J. Comput. Appl. Math., 425 (2023), 115065. https://doi.org/10.1016/j.cam.2023.115065 doi: 10.1016/j.cam.2023.115065
![]() |
[33] | Y. J. Zhang, H. Y. Li, Greedy capped nonlinear Kaczmarz methods, arXiv: 2210.00653 [preprint], (2022), [cited 2024 March 27]. Available from: https://doi.org/10.48550/arXiv.2210.00653 |
[34] | Y. J. Zhang, H. Y. Li, L. Tang, Greedy randomized sampling nonlinear Kaczmarz methods, arXiv: 2209.06082 [preprint], (2022), [cited 2024 March 27]. Available from: https://doi.org/10.48550/arXiv.2209.06082 |
[35] |
A. Zouzias, N. M. Freris, Randomized extended Kaczmarz for solving least squares, SIAM J. Matrix Anal. A., 34 (2013), 773–793. https://doi.org/10.1137/120889897 doi: 10.1137/120889897
![]() |