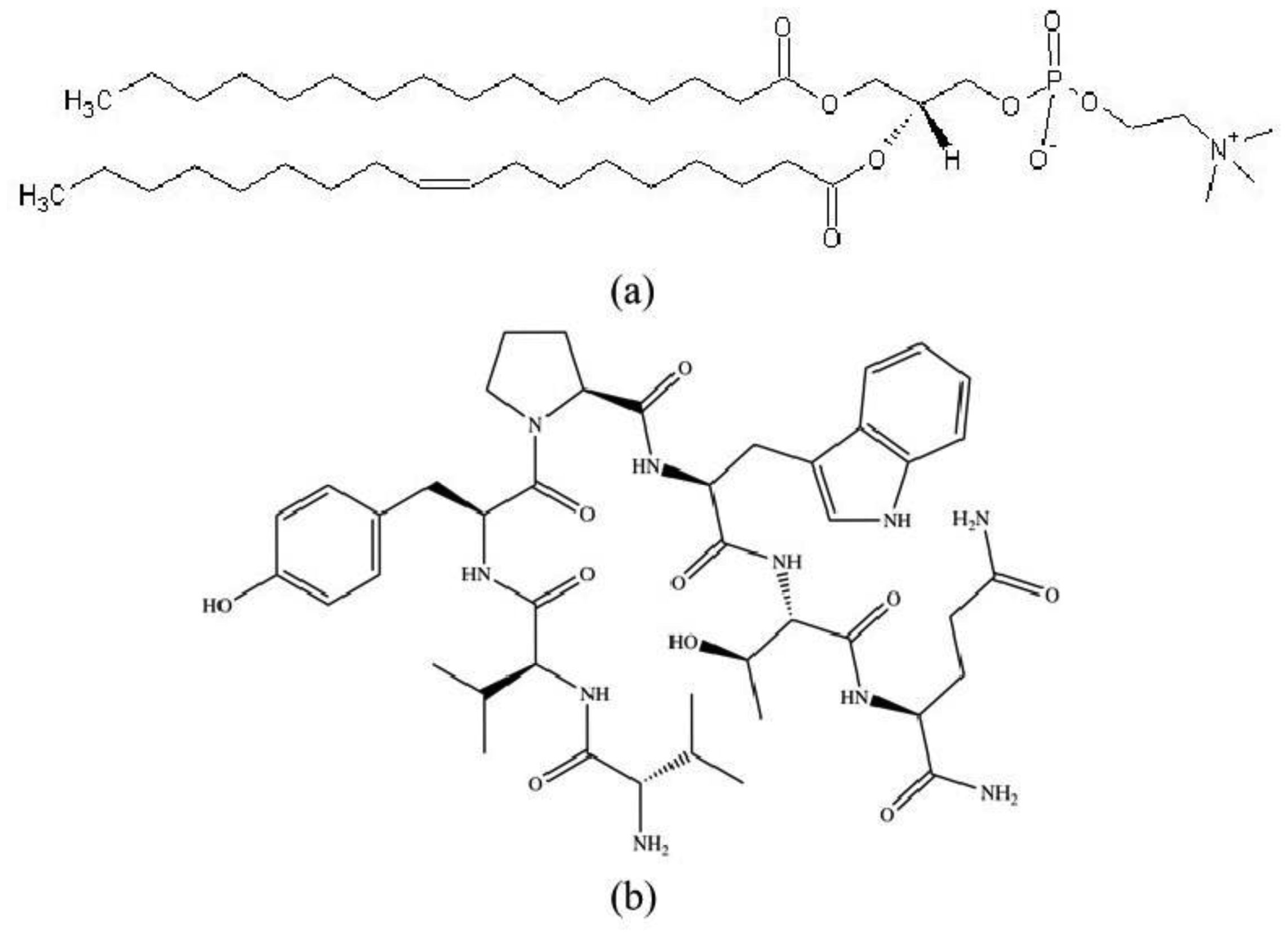
The morphinomimetic properties of hemorphins are intensively studied with regard to new peptide drug developments. In this respect, the investigation of mechanical properties and stability of lipid membranes provides a useful background for advancement in pharmacological applications of liposomes. Here we probed the effect of the endogenous heptapeptide VV-hemorphin-5 (valorphin) on the bending elasticity of biomimetic lipid membranes of 1-palmitoyl-2-oleoyl-sn-glycero-3-phosphocholine (POPC) by analysis of thermal shape fluctuations of nearly spherical giant unilamellar vesicles. In a wide concentration range covering valorphin concentrations applied in nociceptive screening in vivo, we report alterations of the bilayer bending rigidity in a concentration-dependent non-monotonic manner. We performed quantitative characterization of VV-hemorphin-5 association to POPC membranes by isothermal titration calorimetry in order to shed light on the partitioning of the amphiphilic hemorphin between the aqueous solution and membranes. The calorimetric results correlate with flicker spectroscopy findings and support the hypothesis about the strength of valorphin-membrane interaction related to the peptide bulk concentration. A higher strength of valorphin interaction with the bilayer corresponds to a more pronounced effect of the peptide on the membrane's mechanical properties. The presented study features the comprehensive analysis of membrane bending elasticity as a biomarker for physicochemical effects of peptides on lipid bilayers. The reported data on thermodynamic parameters of valorphin interactions with phosphatidylcholine bilayers and alterations of their mechanical properties is expected to be useful for applications of lipid membrane systems in pharmacology and biomedicine.
Citation: Iva Valkova, Petar Todorov, Victoria Vitkova. VV-hemorphin-5 association to lipid bilayers and alterations of membrane bending rigidity[J]. AIMS Biophysics, 2022, 9(4): 294-307. doi: 10.3934/biophy.2022025
[1] | Rianne Bartelds, Jonathan Barnoud, Arnold J. Boersma, Siewert J. Marrink, Bert Poolman . Lipid phase separation in the presence of hydrocarbons in giant unilamellar vesicles. AIMS Biophysics, 2017, 4(4): 528-542. doi: 10.3934/biophy.2017.4.528 |
[2] | Nily Dan . Bilayer degradation in reactive environments. AIMS Biophysics, 2017, 4(1): 33-42. doi: 10.3934/biophy.2017.1.33 |
[3] | Sudarat Tharad, Chontida Tangsongcharoen, Panadda Boonserm, José L. Toca-Herrera, Kanokporn Srisucharitpanit . Local conformations affect the histidine tag-Ni2+ binding affinity of BinA and BinB proteins. AIMS Biophysics, 2020, 7(3): 133-143. doi: 10.3934/biophy.2020011 |
[4] | Thuy Hien Nguyen, Catherine C. Moore, Preston B. Moore, Zhiwei Liu . Molecular dynamics study of homo-oligomeric ion channels: Structures of the surrounding lipids and dynamics of water movement. AIMS Biophysics, 2018, 5(1): 50-76. doi: 10.3934/biophy.2018.1.50 |
[5] | Carl S. Helrich . Studies of cholesterol structures in phospholipid bilayers. AIMS Biophysics, 2017, 4(3): 415-437. doi: 10.3934/biophy.2017.3.415 |
[6] | Chia-Wen Wang, Meng-Han Lin, Wolfgang B. Fischer . Cholesterol affected dynamics of lipids in tailor-made vesicles by ArcVes software during multi micro second coarse grained molecular dynamics simulations. AIMS Biophysics, 2023, 10(4): 482-502. doi: 10.3934/biophy.2023027 |
[7] | Keleabetswe L. Mpye, Samantha Gildenhuys, Salerwe Mosebi . The effects of temperature on streptavidin-biotin binding using affinity isothermal titration calorimetry. AIMS Biophysics, 2020, 7(4): 236-247. doi: 10.3934/biophy.2020018 |
[8] |
István P. Sugár .
A generalization of the Shell Theorem. Electric potential of charged spheres and charged vesicles surrounded by electrolyte. AIMS Biophysics, 2020, 7(2): 76-89. doi: 10.3934/biophy.2020007 |
[9] | István P. Sugár, Parkson Lee-Gau Chong . Monte Carlo simulations of the distributions of intra- and extra-vesicular ions and membrane associated charges in hybrid liposomes composed of negatively charged tetraether and zwitterionic diester phospholipids. AIMS Biophysics, 2017, 4(2): 316-336. doi: 10.3934/biophy.2017.2.316 |
[10] | Nily Dan . Membrane-induced interactions between curvature-generating protein domains: the role of area perturbation. AIMS Biophysics, 2017, 4(1): 107-120. doi: 10.3934/biophy.2017.1.107 |
The morphinomimetic properties of hemorphins are intensively studied with regard to new peptide drug developments. In this respect, the investigation of mechanical properties and stability of lipid membranes provides a useful background for advancement in pharmacological applications of liposomes. Here we probed the effect of the endogenous heptapeptide VV-hemorphin-5 (valorphin) on the bending elasticity of biomimetic lipid membranes of 1-palmitoyl-2-oleoyl-sn-glycero-3-phosphocholine (POPC) by analysis of thermal shape fluctuations of nearly spherical giant unilamellar vesicles. In a wide concentration range covering valorphin concentrations applied in nociceptive screening in vivo, we report alterations of the bilayer bending rigidity in a concentration-dependent non-monotonic manner. We performed quantitative characterization of VV-hemorphin-5 association to POPC membranes by isothermal titration calorimetry in order to shed light on the partitioning of the amphiphilic hemorphin between the aqueous solution and membranes. The calorimetric results correlate with flicker spectroscopy findings and support the hypothesis about the strength of valorphin-membrane interaction related to the peptide bulk concentration. A higher strength of valorphin interaction with the bilayer corresponds to a more pronounced effect of the peptide on the membrane's mechanical properties. The presented study features the comprehensive analysis of membrane bending elasticity as a biomarker for physicochemical effects of peptides on lipid bilayers. The reported data on thermodynamic parameters of valorphin interactions with phosphatidylcholine bilayers and alterations of their mechanical properties is expected to be useful for applications of lipid membrane systems in pharmacology and biomedicine.
Endogenous hemoglobin-derived peptides (hemorphins) have been shown to exhibit pharmacological potential related to their numerous biological activities [1],[2]. Hemorphins are promising therapeutic agents and biomarkers in oncology, nephrology and vascular medicine and can be efficiently applied in inflammation and for mood regulation [3]–[8]. The detailed characterization of hemorphins' interaction mechanisms with biomembranes provides useful data in view of increasing expectations towards peptides in the prevention and treatment of various diseases and conditions [9]. The application of lipid vesicles as drug carriers motivates the investigation of their physical properties, dynamics, and stability in the presence of medicinal substances for vectorization. The bending rigidity, which characterizes the deformability of lipid bilayers, is relevant to the flow properties of vesicles in blood circulation. Thus, the investigation of membrane mechanical properties is of practical importance regarding biomedical applications of liposomes.
Quantitative characterization of valorphin association with LUVs could allow the determination of peptides partitioning between the aqueous solution and the lipid membrane [10]. Also, a thermodynamic description of the binding process would provide better insight into the mechanism of these interactions [11] and make a valuable impact on the investigation of peptide effects on the structural, mechanical and electrical properties of lipid membranes. Isothermal titration calorimetry (ITC) is a technique capable of measuring directly the binding energetics of biological processes and to generate information concerning the nature of interactions. In the present study it is considered as a suitable approach to confirm and thermodynamically characterize the binding of valorphin to phosphatidylcholine membranes [12].
The synthetic lipid 1-palmitoyl-2-oleoyl-sn-glycero-3-phosphocholine, POPC (Avanti Polar Lipids Inc. (AL, USA) was used for the preparation of model membranes. Bidistilled water (pH 5.5) was produced in a quartz distiller. Chloroform and methanol for lipid solutions were purchased analytical grade from Sigma-Aldrich (Germany). Polydimethylsiloxane (PDMS) was supplied by Dow Corning (Germany). VV-hemorphin-5 was obtained by solid phase synthesis as described previously [13]. Structural formulas of the peptide and phosphatidylcholine used in the study are shown in Figure 1 as drawn by ACD Labs (ACD Inc., Canada) and PerkinElmer ChemDraw Professional 16.0, CambridgeSoft (MA, USA).
LUVs were obtained by extrusion [14]. LUV suspensions were prepared from POPC dissolved in chloroform/methanol (1: 1 v/v). The solvent was completely removed from the samples after evaporation under a stream of oxygen-free dry nitrogen followed by desiccation under vacuum overnight. Lipid depositions were then hydrated with filtered (220 nm) bidistilled water to a lipid concentration of 1.3 mmol/L. The samples were vortexed for 1 min, then left in a sonication bath for 5 min. The multilamellar vesicles thus obtained were subsequently extruded with a LipoFast small-volume extruder equipped with polycarbonate filters (Avestin, Ottawa, Canada) by performing 11 extrusions through 800 nm, followed by 21 extrusions through 100 nm filters. LUV samples were studied the same day after equilibration for 20 min at 22 ± 1 °C.
Valorphin-containing quasispherical giant unilamellar vesicles (GUV) were produced by electroformation [15] on indium tin oxide (ITO)-coated glass plates separated by a polydimethylsiloxane (PDMS, Dow Corning, Germany) spacer following the procedure reported in [16]. Lipid depositions were formed by the uniform spreading of 50 µL of POPC-valorphin solution with the total concentration of 1 g/L in chloroform-methanol (9: 1 volume parts) on the ITO-coated side of each electrode. After the complete evaporation of the organic solvents under vacuum, the electroformation chamber (4 mL) was filled in with bidistilled water, thus achieving the final lipid concentration of 33 µM. Subsequently, AC electric field with a frequency of 10 Hz and 3 V peak-to-peak voltage was applied to the ITO-electrodes for a couple of hours in order to obtain a high yield of nearly spherical fluctuating GUVs appropriate for analysis of thermal fluctuations of their shape according to [17].
The analysis of thermal shape fluctuations (ATSF)
Here n ≥ 2, kB is the Boltzmann constant and T is the absolute temperature. The real mechanical tension of the bilayer
ATSF performed on visibly fluctuating quasispherical GUVs with diameters larger than 10 µm, exploits algorithms for quality control of the recorded vesicles as discussed in [17]. Thus, alterations of the calculated kc value by systematic artifacts (volume and surface changes during measurements, non-quasisphericity, blurred contours, membrane defects, etc.) are eliminated.
Observation chambers of two parallel glass slides, separated by a 0.5 mm-thick (CoverWell®) spacer (Sigma-Aldrich Inc., USA) were used containing ~400 µL of GUV suspension freshly prepared. GUVs' shape fluctuations were observed under Axiovert 100 (Zeiss, Germany) inverted microscope operating in phase contrast and equipped with an oil-immersed objective Zeiss N-Achroplan (Ph3, 100x, NA 1.25). The stroboscopic sample illumination, synchronized with the camera, makes it accessible for the acquisition and analysis of fast fluctuation modes [23]. The image record and processing were carried out through a CCD camera (C3077, Hamamatsu Photonics, Japan) connected to a frame grabber board (DT3155, Data Translation, USA, 768x576 8-bit pixels; 0.106 µm/pix) mounted in a computer for digitization and analysis.
The association of VV-hemorphin-5 to phosphatidylcholine membranes is assessed by high-sensitivity titration calorimetry [24],[25] The heat flow resulting from the peptide-lipid interaction was measured using a low-volume NanoITC calorimeter (TA Instruments, Lindon, UT, USA) with a reaction cell of 190 µL and 50 µL syringe. Prior to use, the device is electrically calibrated at 25 °C with both the sample and reference cells filled with distilled water.
We studied LUV suspensions prepared from POPC and bidistilled water following the procedure described above. The binding behavior of VV-hemorphin-5 was assessed in the concentration range between 1.75 and 3.5 µmol/L. All samples were tempered (25 °C) and thoroughly degassed under vacuum (84 kPa, 20 min with stirring) to prevent air bubble formation. In a series of measurements, 300 µL of peptide solutions were titrated with LUV suspensions. Aliquots of 2 µL were injected in 25 steps with a 300 s interval and a stirring speed of 250 rpm at 25 °C. The blank experiment, accounting for the heat of vesicle dilution in a pure solvent, is performed at the same conditions. Heat rate changes, detected during the interactions, were submitted to the calorimeter software for calculation of injection heats (ITCRun software) and thermodynamic parameters of binding (NanoAnalyze software, TA Instruments, Lindon, UT, USA), respectively.
Isothermal titration calorimetry (ITC) was applied to probe the association of VV-hemorphin-5 to phosphatidylcholine membranes. Thermodynamic parameters of valorphin-membrane interactions were studied for model systems comprised of POPC vesicle suspensions and diluted (3.5 µmol/L) valorphin aqueous solutions. The heat of LUV dilution in hemorphin solutions was measured in multiple injection modes. The inner panels in Figure 2 represent the heat flow during the ITC experiments in which 2 µL aliquots of lipid vesicles (1.3 mmol/L lipid concentration) were repeatedly injected into the sample cell containing valorphin solution. The detected peaks were exothermic for all investigated peptide concentrations and indicated that binding interactions were accompanied by the release of heat from the system. In the course of titration, the heat flow decreased because of the free peptide depletion in the calorimeter cell but even at the end of titration peaks did not diminish completely to the baseline. This was attributed to the dilution heat of LUVs since the injection heats after about 10 aliquots were comparable to those measured in the blank experiment. Therefore, the signals were corrected by the average area of dilution peaks before the evaluation of reaction enthalpies and binding isotherms [26]. The calorimetric data were analyzed using the NanoAnalyze software provided with the instrument.
Thermodynamic characterization of the studied interactions required applying of a theoretical model consistent with the experimental results. In our case the model for one set of binding sites the most satisfactorily fitted the data. It assumed the existence of N independent and thermodynamically identical binding sites on lipid vesicles. Interaction of VV-hemorphin-5 with these sites is an equilibrium process dependent on the unbound peptide concentration and free lipids from LUVs external monolayers [26],[27]. As thoroughly discussed by Seelig [28] peptide-membrane interactions represent partitioning and/or adsorption rather than the specific binding between peptide and lipid molecules.
Partitioning of the amphiphilic VV-hemorphin-5 between the bulk solution and the hydrophobic core or interface of LUVs is quantified by the equilibrium constant [29],[30]:
where ΔG0/NA stands for the standard free energy of peptide transfer from the bulk phase into the membrane
From the last two relations the fraction Φ can be presented as a function of the lipid concentration. The heat release
As far as the ITC experiment measures the heat change Q(i) – Q(i – 1) is measured, ΔQ(i) for the i-th injection is given by Eq 4. The expression is corrected for the displaced volume dVi at the i-th titration step.
Nonlinear regression analysis of experimental data with
P/L (mol%) | Kp (105 L/mol) | N | ΔH (kJ/mol) | ΔG (kJ/mol) | TΔS (kJ/mol) | |
1.75 | 0.571 | 9.302 ± 4.195 | 0.134 ± 0.001 | –17.60 ± 0.32 | –39.04 | –21.44 |
2.60 | 0.849 | 0.297 ± 0.049 | 0.187 ± 0.025 | –2.06 ± 0.77 | –29.69 | –27.63 |
3.50 | 1.142 | 0.050 ± 0.007 | 0.100 ± 0.027 | –0.76 ± 0.08 | –26.83 | –26.07 |
ITC measurements were performed at three different valorphin concentrations as shown in Table 1. The peptide concentration range was chosen not to exceed valorphin concentrations applied in nociceptive screening in vivo [13].
Peptide-membrane interactions possess exothermic nature and suggest an association by van der Waals forces, intra- and/or intermolecular hydrogen bonds. Since ITC only generates information for the overall enthalpy they cannot be distinguished. The heat release during the experiment indicates the transfer of the system to formation of associates between the peptides and lipid bilayers.
An inverse relationship was observed between the strength of peptide-to-lipid binding and peptide concentrations in solution, possibly due to the steric hindrance between molecules. The calculated Gibbs free energy ΔG was negative in the range of investigated valorphin concentrations and verified the spontaneous peptide-membrane association via partitioning and adsorption. Both enthalpy and entropy terms had a favorable impact on Gibbs free energy with a more pronounced effect of entropy (Table 1). Usually, the entropy increase is attributed to hydrophobic interactions, desolvation of molecular surfaces and conformational changes of the interacting species [28].
The exothermic nature of membrane-peptide interaction (
The effect of valorphin on the membrane mechanical properties was assessed by measuring the bending rigidity of POPC bilayers containing various concentrations of the opioid peptide. Several hundred frames were acquired once per second for every studied GUV [18]. Taking into account that the time lapse between two adjacent images was 1 s, we performed a correlation test of each image sequence. Consequently, only vesicles with a correlation time of the second fluctuation mode, n = 2 in Eq 1 [32],[33], lower than half a second, τ2 ≤ 0.5 s were used for the calculation of the membrane bending modulus. The values of the bending modulus reported here, represent the weighted average of kc with standard deviations, calculated over the vesicles, meeting all requirements for goodness [17] as shown in Table 2 and Figure 3 (black closed circles). At P/L ~ 0.035 mol% we report a considerable increase (~40%) of the bending constant testifying to more rigid lipid bilayers in the presence of valorphin. As it has been established theoretically and experimentally [22],[34]–[40] the bending elasticity of lipid membranes is related to structural parameters of the bilayer such as chemical composition, phase state, thickness, lipid packing, unsaturation of the aliphatic chains, surface charge, etc. It has been revealed that the interaction of amphipathic peptide molecules with lipid bilayers may significantly change the membrane bending rigidity. The effect of amphiphilic peptides such as alamethicin, magainin, melittin and other antimicrobial peptides on the mechanical properties of lipid bilayers has been reported in previous studies of ours and other authors [41]–[45]. Most of them lead to membrane softening as a result of the lateral perturbation of lipid molecules in the bilayer by the presence of adsorbed peptide molecules inducing spontaneous curvature and membrane thinning [46]. In the case of valorphin studied here, an interesting non-monotonic behavior of the membrane bending elasticity is reported as a function of the peptide concentration. The above finding suggests that the origin of membrane stiffening may be related to changes in the membrane thickness and/or packing density of the bilayer in the presence of 0.035 mol% of valorphin. For example, similar stiffening has been reported previously for polymer-grafted [47] or cholesterol-containing bilayers [48],[49]. A previous study on native gramicidin/phosphatidylcholine interactions has revealed a biphasic change in membrane behavior at a critical peptide-to-lipid molar ratio [50]. In the case of the highly hydrophobic long-chain gramicidin the observations have been related to the condensing effect of the polypeptide on the surrounding lipid molecules with a local maximum of the densely packed area around a critical molar concentration. In this densely packed area, an ordered arrangement of gramicidin dimers have been obtained together with a disordered arrangement of gramicidin monomers in the fluid membrane regions. In our study we correlate results from mechanical and calorimetric measurements, both yielding similar non-monotonic dependence on valorphin concentration (Figure 3). Since data on valorphin conformation in the phosphatidylcholine membrane from experiments or molecular dynamic simulations are not available, only assumptions can be made about the molecular mechanisms involved. In order to explore the molecular mechanisms leading to the observed non-monotonic behavior of kc with the peptide concentration, further investigations need to be performed such as circular dichroism spectroscopy for characterization of valorphin conformation and aggregation.
P/L (mol%) | kc (10–19 J) | m | GF |
0 | 1.46 ± 0.05 | 10 | 0.82 |
0.005 | 1.33 ± 0.11 | 7 | 0.15 |
0.010 | 1.50 ± 0.16 | 6 | 0.77 |
0.035 | 2.07 ± 0.14 | 11 | 0.82 |
1 | 1.67 ± 0.08 | 13 | 0.98 |
10 | 1.47 ± 0.09 | 8 | 0.41 |
Thermodynamic parameters of valorphin interaction with POPC membranes were studied at peptide bulk concentrations up to
The higher strength of valorphin-membrane interactions obtained from the calorimetric measurements at total peptide concentration P/L ~ 0.8 mol% is coherent with the stronger effect of VV-hemorphin-5 on POPC membrane elasticity revealed by thermal shape fluctuation analysis of GUVs.
Limited solubility and concentration-dependent conformation of valorphin in water have not been systematically investigated yet. VV-hemorphin-5 is a heptapeptide consisting of hydrophobic non-polar and hydrophilic polar amino acids as shown in Figure 1b. The presence of nonpolar valine, proline and tryptophan in the structure usually involves peptides in hydrophobic-effect-based interactions, while the polar threonine, tyrosine and glutamine residues participate in hydrogen bonding to water and lipid molecules, which is important in the mechanisms of peptide interaction with membranes. The non-polar residues contribute mainly to the detected entropy change of the system. From general considerations, apart from hydrophobic interactions, they are also supposed to be engaged in van der Waals interactions that decrease the enthalpy. Another source of enthalpy decrease is the possibility of hydrogen bond formation between the hydroxyl groups in the side chains of tyrosine and threonine and water molecules solvating LUV surfaces. Formation of intramolecular hydrogen bonds between skeletal amino and carbonyl groups of the peptide is also possible since they stabilize the conformation of a peptide chain. Tyrosine is the only ionizable amino acid in valorphin structure. It behaves as a very weak acid but at pH 5.5 is fully protonated. At the same pH, the N-terminus of glutamine is positively charged and can be electrostatically attracted by the lipid phosphate group. The membrane stiffening at very low valorphin concentrations indicates a peptide-induced increase of the lipid packing density and thickening until a ‘critical’ total peptide concentration is achieved, above which membrane softening is measured. The observed phenomena may be due to a concentration-dependent valorphin aggregation changing the peptide-membrane interaction above this ‘threshold’ valorphin concentration. This assumption may underlie further investigations by structural methods on valorphin-phosphatidylcholine membranes. The latter is intended to be performed as an objective of a future study. In further investigations of molecular mechanisms of valorphin/POPC interactions the application of a number of experimental techniques such as circular dichroism spectroscopy, and in silico methods may be considered. The answer to the question regarding the origin of the effect may be found by correlating the obtained results on the number of binding sites and membrane stiffening with circular dichroism measurements and numerical simulations of the peptide conformation and aggregation in solution and in the membrane environment.
The results reported above contribute to the physicochemical description of valorphin-containing lipid bilayers. The bending modulus of POPC membranes was measured in a wide range of VV-hemorpin-5 concentrations revealing a considerable increase in its value at a given valorphin concentration. Similar behavior was obtained for the strength of valorphin interaction with the bilayer quantified by the number of binding sites considering partitioning and/or adsorption rather than the specific binding between peptide and lipid molecules. Future efforts to study the conformation of the opioid peptide as a function of its concentration in the bulk solution would provide useful insight regarding the concentration-related valorphin effect on the membrane mechanics.
Knowledge attained on the interplay between the endogenous hemoglobin-derived heptapeptide VV-hemorphin-5 with phosphatidylcholine bilayers may be useful in the development of various applications of lipid membrane systems in pharmacology and biomedicine. The determination of material parameters of lipid bilayers in the presence of peptide and protein molecules is relevant also to characterization of mechanical properties of cell membranes, which are highly dynamic, fluid structures, made functional by complex lipid–lipid, lipid–protein and protein–protein interactions.
[1] |
Karelin AA, Philippova MM, Karelina EV, et al. (1994) Isolation of endogenous hemorphin-related hemoglobin fragments from bovine brain. Biochem Bioph Res Co 202: 410-415. https://doi.org/10.1006/bbrc.1994.1943 ![]() |
[2] |
Sanderson K, Nyberg F, Khalil Z (1998) Modulation of peripheral inflammation by locally administered hemorphin-7. Inflamm Res 47: 49-55. https://doi.org/10.1007/s000110050266 ![]() |
[3] |
Altinoz MA, Elmaci I, Ince B, et al. (2016) Hemoglobins, hemorphins, and 11p15.5 chromosomal region in cancer biology and immunity with special emphasis for brain tumors. J Neurol Surg Part A 77: 247-257. https://doi.org/10.1055/s-0035-1566120 ![]() |
[4] |
Altinoz MA, Guloksuz S, Schmidt-Kastner R, et al. (2019) Involvement of hemoglobins in the pathophysiology of Alzheimer's disease. Exp Gerontol 126: 110680. https://doi.org/10.1016/j.exger.2019.110680 ![]() |
[5] |
Blishchenko EY, Sazonova OV, Kalinina OA, et al. (2005) Antitumor effect of valorphin in vitro and in vivo: combined action with cytostatic drugs. Cancer Biol Ther 4: 125-131. https://doi.org/10.4161/cbt.4.1.1474 ![]() |
[6] |
Blishchenko EY, Sazonova OV, Kalinina OA, et al. (2002) Family of hemorphins: co-relations between amino acid sequences and effects in cell cultures. Peptides 23: 903-910. https://doi.org/10.1016/S0196-9781(02)00017-7 ![]() |
[7] |
Wei F, Zhao L, Jing Y (2020) Hemoglobin-derived peptides and mood regulation. Peptides 127: 170268. https://doi.org/10.1016/j.peptides.2020.170268 ![]() |
[8] |
Ali A, Alzeyoudi SAR, Almutawa SA, et al. (2020) Molecular basis of the therapeutic properties of hemorphins. Pharmacol Res 158: 104855. https://doi.org/10.1016/j.phrs.2020.104855 ![]() |
[9] |
Mielczarek P, Hartman K, Drabik A, et al. (2021) Hemorphins—From discovery to functions and pharmacology. Molecules 26: 3879. https://doi.org/10.3390/molecules26133879 ![]() |
[10] |
Wieprecht T, Seelig J (2002) Isothermal titration calorimetry for studying interactions between peptides and lipid membranes. Curr Top Membr 52: 31-56. https://doi.org/10.1016/S1063-5823(02)52004-4 ![]() |
[11] |
Wiseman T, Williston S, Brandts JF, et al. (1989) Rapid measurement of binding constants and heats of binding using a new titration calorimeter. Anal Biochem 179: 131-137. https://doi.org/10.1016/0003-2697(89)90213-3 ![]() |
[12] | Velázquez-Campoy A, Ohtaka H, Nezami A, et al. (2004) Isothermal titration calorimetry. Curr Protoc Cell Biol : 17.18.1-17.18.24. https://doi.org/10.1002/0471143030.cb1708s23 |
[13] |
Todorov P, Peneva P, Pechlivanova D, et al. (2018) Synthesis, characterization and nociceptive screening of new VV-hemorphin-5 analogues. Bioorg Med Chem Lett 28: 3073-3079. https://doi.org/10.1016/j.bmcl.2018.07.040 ![]() |
[14] |
Vitkova V, Yordanova V, Staneva G, et al. (2021) Dielectric properties of phosphatidylcholine membranes and the effect of sugars. Membranes-Basel 11: 847. https://doi.org/10.3390/membranes11110847 ![]() |
[15] |
Angelova MI, Dimitrov DS (1986) Liposome electroformation. Faraday Discuss Chem Soc 81: 303-311. https://doi.org/10.1039/DC9868100303 ![]() |
[16] |
Vitkova V, Antonova K, Popkirov G, et al. (2010) Electrical resistivity of the liquid phase of vesicular suspensions prepared by different methods. J Phys Conf Ser 253: 012059. https://doi.org/10.1088/1742-6596/253/1/012059 ![]() |
[17] |
Genova J, Vitkova V, Bivas I (2013) Registration and analysis of the shape fluctuations of nearly spherical lipid vesicles. Phys Rev E 88: 022707. https://doi.org/10.1103/PhysRevE.88.022707 ![]() |
[18] | Mitov MD, Faucon JF, Méléard P, et al. (1992) Thermal fluctuations of membranes, In: Gokel, G.W., Advances in Supramolecular Chemistry, 1 Eds., Greenwich: JAI Press Inc., 93–139. |
[19] |
Faucon JF, Mitov MD, Méléard P, et al. (1989) Bending elasticity and thermal fluctuations of lipid membranes. Theoretical and experimental requirements. J Phys 50: 2389-2414. https://doi.org/10.1051/jphys:0198900500170238900 ![]() |
[20] |
Bivas I, Hanusse P, Bothorel P, et al. (1987) An application of the optical microscopy to the determination of the curvature elastic modulus of biological and model membranes. J Phys 48: 855-867. https://doi.org/10.1051/jphys:01987004805085500 ![]() |
[21] |
Schneider MB, Jenkins JT, Webb WW (1984) Thermal fluctuations of large quasi-spherical bimolecular phospholipid vesicles. J Phys 45: 1457-1472. https://doi.org/10.1051/jphys:019840045090145700 ![]() |
[22] |
Helfrich W (1973) Elastic properties of lipid bilayers: theory and possible experiments. Z Naturforsch C 28: 693-703. https://doi.org/10.1515/znc-1973-11-1209 ![]() |
[23] | Genova J, Vitkova V, Aladgem L, et al. (2005) Stroboscopic illumination gives new opportunities and improves the precision of bending elastic modulus measurements. J Optoelectron Adv M 7: 257-260. |
[24] | Seelig J (1997) Titration calorimetry of lipid–peptide interactions. BBA-Biomembranes 1331: 103-116. https://doi.org/10.1016/S0304-4157(97)00002-6 |
[25] |
Freyer MW, Lewis EA (2008) Isothermal titration calorimetry: experimental design, data analysis, and probing macromolecule/ligand binding and kinetic interactions. Method Cell Biol 84: 79-113. https://doi.org/10.1016/s0091-679x(07)84004-0 ![]() |
[26] | Damian L (2013) Isothermal titration calorimetry for studying protein-ligand interactions, In: Williams, M., Daviter, T., Protein-Ligand Interactions, Methods in Molecular Biology, New Jersey: Humana Press, 1008, 103–118. https://doi.org/10.1007/978-1-62703-398-5_4 |
[27] |
Turner DC, Straume M, Kasimova MR, et al. (1995) Thermodynamics of interaction of the fusion-inhibiting peptide Z-D-Phe-L-Phe-Gly with dioleoylphosphatidylcholine vesicles: direct calorimetric determination. Biochemistry-US 34: 9517-9525. https://doi.org/10.1021/bi00029a028 ![]() |
[28] |
Seelig J (2004) Thermodynamics of lipid–peptide interactions. BBA-Biomembranes 1666: 40-50. https://doi.org/10.1016/j.bbamem.2004.08.004 ![]() |
[29] | Tamm LK (1994) Physical studies of peptide—bilayer interactions, In: White, S.H., Membrane Protein Structure, Methods in Physiology Series, New York: Springer, New York. https://doi.org/10.1007/978-1-4614-7515-6_13 |
[30] |
Ben-Shaul A, Ben-Tal N, Honig B (1996) Statistical thermodynamic analysis of peptide and protein insertion into lipid membranes. Biophys J 71: 130-137. https://doi.org/10.1016/S0006-3495(96)79208-1 ![]() |
[31] |
Mertins O, Dimova R (2011) Binding of chitosan to phospholipid vesicles studied with isothermal titration calorimetry. Langmuir 27: 5506-5515. https://doi.org/10.1021/la200553t ![]() |
[32] |
Milner ST, Safran SA (1987) Dynamical fluctuations of droplet microemulsions and vesicles. Phys Rev A 36: 4371-4379. https://doi.org/10.1103/PhysRevA.36.4371 ![]() |
[33] |
Bivas I (2010) Shape fluctuations of nearly spherical lipid vesicles and emulsion droplets. Phys Rev E 81: 061911. https://doi.org/10.1103/PhysRevE.81.061911 ![]() |
[34] |
Fernandez-Puente L, Bivas I, Mitov MD, et al. (1994) Temperature and chain length effects on bending elasticity of phosphatidylcholine bilayers. Europhysics Lett 28: 181-186. https://doi.org/10.1209/0295-5075/28/3/005 ![]() |
[35] |
Rawicz W, Olbrich KC, Mclntosh T, et al. (2000) Effect of chain length and unsaturation on elasticity of lipid bilayers. Biophys J 79: 328-339. https://doi.org/10.1016/S0006-3495(00)76295-3 ![]() |
[36] |
Marsh D (2006) Elastic curvature constants of lipid monolayers and bilayers. Chem Phys Lipids 144: 146-159. https://doi.org/10.1016/j.chemphyslip.2006.08.004 ![]() |
[37] | Vitkova V, Petrov AG (2013) Lipid bilayers and membranes: material properties, In: Aleš, I., Genova, J., Advances in Planar Lipid Bilayers and Liposomes, Academic Press, 17, 89–138. https://doi.org/10.1016/B978-0-12-411516-3.00005-X |
[38] |
Dimova R (2014) Recent developments in the field of bending rigidity measurements on membranes. Adv Colloid Interfac 208: 225-234. https://doi.org/10.1016/j.cis.2014.03.003 ![]() |
[39] |
Faizi HA, Tsui A, Dimova R, et al. (2022) Bending rigidity, capacitance, and shear viscosity of giant vesicle membranes prepared by spontaneous swelling, electroformation, gel-assisted, and phase transfer methods: a comparative study. Langmuir 38: 10548-10557. https://doi.org/10.1021/acs.langmuir.2c01402 ![]() |
[40] |
Dimova R (2019) Giant vesicles and their use in assays for assessing membrane phase state, curvature, mechanics, and electrical properties. Annu Rev Biophys 48: 93-119. https://doi.org/10.1146/annurev-biophys-052118-115342 ![]() |
[41] |
Bivas I, Méléard P (2003) Bending elasticity of a lipid bilayer containing an additive. Phys Rev E 67: 012901. https://doi.org/10.1103/PhysRevE.67.012901 ![]() |
[42] |
Vitkova V, Méléard P, Pott T, et al. (2006) Alamethicin influence on the membrane bending elasticity. Eur Biophys J 35: 281-286. https://doi.org/10.1007/s00249-005-0019-5 ![]() |
[43] |
Shchelokovskyy P, Tristram-Nagle S, Dimova R (2011) Effect of the HIV-1 fusion peptide on the mechanical properties and leaflet coupling of lipid bilayers. New J Phys 13: 025004. https://doi.org/10.1088/1367-2630/13/2/025004 ![]() |
[44] |
Bouvrais H, Méléard P, Pott T, et al. (2008) Softening of POPC membranes by magainin. Biophys Chem 137: 7-12. https://doi.org/10.1016/j.bpc.2008.06.004 ![]() |
[45] |
Pott T, Gerbeaud C, Barbier N, et al. (2015) Melittin modifies bending elasticity in an unexpected way. Chem Phys Lipids 185: 99-108. https://doi.org/10.1016/j.chemphyslip.2014.05.004 ![]() |
[46] |
Zemel A, Ben-Shaul A, May S (2008) Modulation of the spontaneous curvature and bending rigidity of lipid membranes by interfacially adsorbed amphipathic peptides. J Phys Chem B 112: 6988-6996. https://doi.org/10.1021/jp711107y ![]() |
[47] |
Evans E, Rawicz W (1997) Elasticity of “Fuzzy” Biomembranes. Phys Rev Lett 79: 2379-2382. https://doi.org/10.1103/PhysRevLett.79.2379 ![]() |
[48] |
Méléard P, Gerbeaud C, Pott T, et al. (1997) Bending elasticities of model membranes: influences of temperature and sterol content. Biophys J 72: 2616-2629. https://doi.org/10.1016/S0006-3495(97)78905-7 ![]() |
[49] |
Gracià RS, Bezlyepkina N, Knorr RL, et al. (2010) Effect of cholesterol on the rigidity of saturated and unsaturated membranes: fluctuation and electrodeformation analysis of giant vesicles. Soft Matter 6: 1472-1482. https://doi.org/10.1039/b920629a ![]() |
[50] |
Sugár IP, Bonanno AP, Chong PLG (2018) Gramicidin lateral distribution in phospholipid membranes: fluorescence phasor plots and statistical mechanical model. Int J Mol Sci 19: 3690. https://doi.org/10.3390/ijms19113690 ![]() |
[51] |
Wieprecht T, Beyermann M, Seelig J (2002) Thermodynamics of the coil-ɑ-helix transition of amphipathic peptides in a membrane environment: the role of vesicle curvature. Biophys Chem 96: 191-201. https://doi.org/10.1016/s0301-4622(02)00025-x ![]() |
1. | Mohammad Abu Sayem Karal, Md. Masum Billah, Marzuk Ahmed, Md. Kabir Ahamed, A review on the measurement of the bending rigidity of lipid membranes, 2023, 19, 1744-683X, 8285, 10.1039/D3SM00882G |
P/L (mol%) | Kp (105 L/mol) | N | ΔH (kJ/mol) | ΔG (kJ/mol) | TΔS (kJ/mol) | |
1.75 | 0.571 | 9.302 ± 4.195 | 0.134 ± 0.001 | –17.60 ± 0.32 | –39.04 | –21.44 |
2.60 | 0.849 | 0.297 ± 0.049 | 0.187 ± 0.025 | –2.06 ± 0.77 | –29.69 | –27.63 |
3.50 | 1.142 | 0.050 ± 0.007 | 0.100 ± 0.027 | –0.76 ± 0.08 | –26.83 | –26.07 |
P/L (mol%) | kc (10–19 J) | m | GF |
0 | 1.46 ± 0.05 | 10 | 0.82 |
0.005 | 1.33 ± 0.11 | 7 | 0.15 |
0.010 | 1.50 ± 0.16 | 6 | 0.77 |
0.035 | 2.07 ± 0.14 | 11 | 0.82 |
1 | 1.67 ± 0.08 | 13 | 0.98 |
10 | 1.47 ± 0.09 | 8 | 0.41 |
P/L (mol%) | Kp (105 L/mol) | N | ΔH (kJ/mol) | ΔG (kJ/mol) | TΔS (kJ/mol) | |
1.75 | 0.571 | 9.302 ± 4.195 | 0.134 ± 0.001 | –17.60 ± 0.32 | –39.04 | –21.44 |
2.60 | 0.849 | 0.297 ± 0.049 | 0.187 ± 0.025 | –2.06 ± 0.77 | –29.69 | –27.63 |
3.50 | 1.142 | 0.050 ± 0.007 | 0.100 ± 0.027 | –0.76 ± 0.08 | –26.83 | –26.07 |
P/L (mol%) | kc (10–19 J) | m | GF |
0 | 1.46 ± 0.05 | 10 | 0.82 |
0.005 | 1.33 ± 0.11 | 7 | 0.15 |
0.010 | 1.50 ± 0.16 | 6 | 0.77 |
0.035 | 2.07 ± 0.14 | 11 | 0.82 |
1 | 1.67 ± 0.08 | 13 | 0.98 |
10 | 1.47 ± 0.09 | 8 | 0.41 |