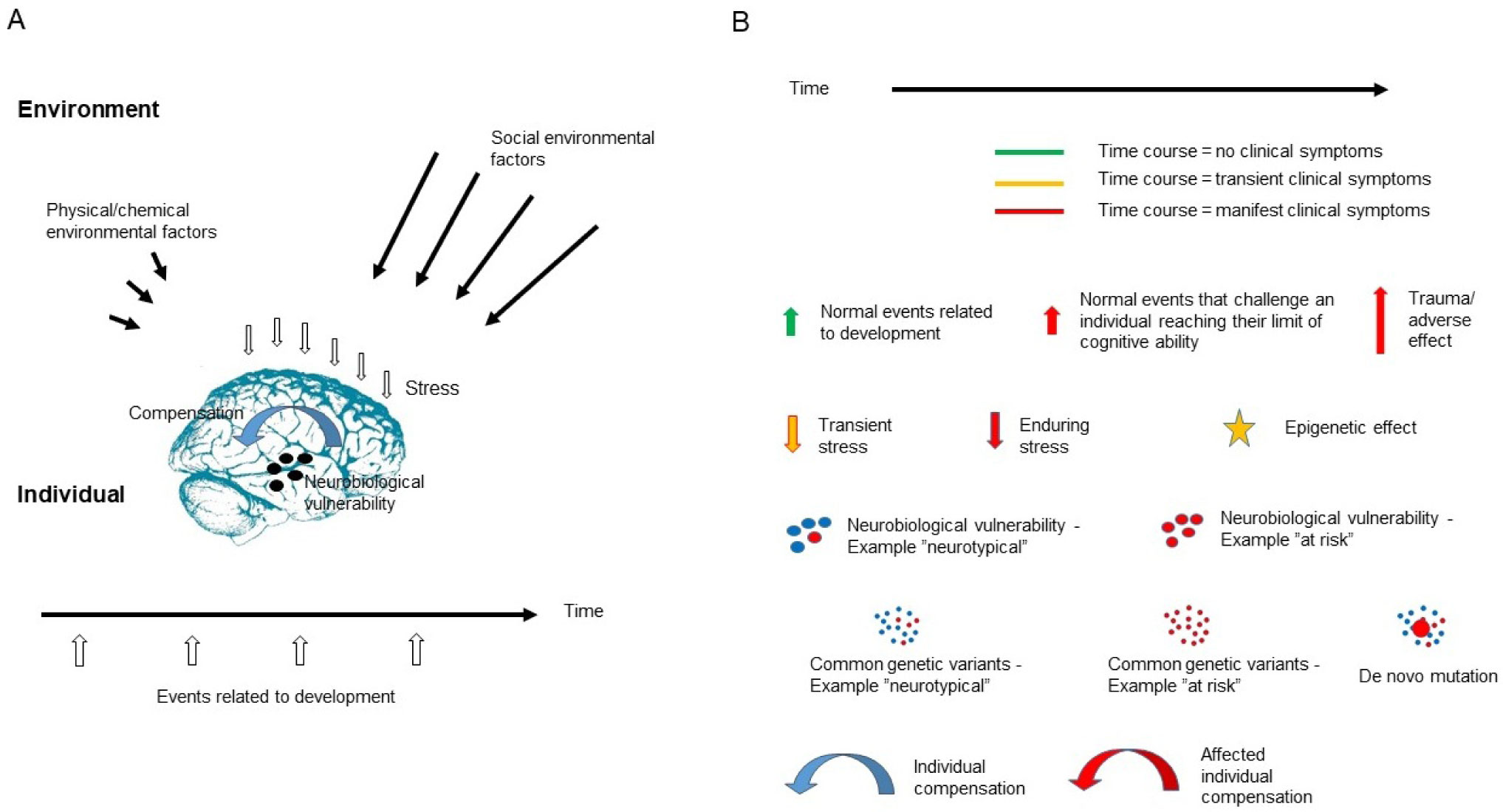
The present article is a theoretical contribution suggesting a simple model of the dynamics involved in the development of neurodevelopmental disorders and the phenomenon of autism offering an explanation of why different individuals have different onset of manifest disease. The model relates to present genetic and epigenetic evidence and previous theoretical models. The dynamic model applies an individualized transdiagnostic and dimensional approach integrating several levels of influence involved in the dynamic interaction between an individual and their environment across time. The dynamic model illustrates the interaction between a basic neurobiological susceptibility, compensating mechanisms, and stress-related releasing mechanisms involved in the development of manifest clinical illness. The model has a particular focus on the dynamics of neurocognitive processes and their relationship to more basic information, psychological and social processes. A basic assumption guiding the model is that even quite normal events related to typical development may increase the risk of enduring stress in cognitively vulnerable individuals, further increasing their risk of developing manifest clinical illness. The model suggests that genetic variation, endogenous epigenetic processes of development, and epigenetic changes influenced by physical/chemical and social environmental risk and resilience factors all may contribute to phenotypical expression. A genetic susceptibility may translate into a biological susceptibility reflected in cognitive impairments. A cognitively vulnerable individual may be at increased risk of misinterpreting sensory inputs, potentially resulting in psychopathological expressions, e.g., autistic symptoms, observed across several neurodevelopmental disorders. The genetically influenced experience of an individual relates to cognitive phenomena occurring at the interfaces between the brain, mind, and society. The severity of clinical illness may differ from the severity of a disorder of reasoning. The dynamic model may help guide future development of personalized medicine in psychiatry and identify relevant points of intervention. A discussion of the implications of the model relating to epigenetic evidence and to previous theoretical models is included at the end of the paper.
Citation: Bodil Aggernæs. Suggestion of a dynamic model of the development of neurodevelopmental disorders and the phenomenon of autism[J]. AIMS Molecular Science, 2020, 7(2): 122-182. doi: 10.3934/molsci.2020008
[1] | Tatjana Mijatovic, Dario Siniscalco, Krishnamurthy Subramanian, Eugene Bosmans, Vincent C. Lombardi, Kenny L. De Meirleir . Biomedical approach in autism spectrum disorders—the importance of assessing inflammation. AIMS Molecular Science, 2018, 5(3): 173-182. doi: 10.3934/molsci.2018.3.173 |
[2] | Rosanna Parlato, Holger Bierhoff . Role of nucleolar dysfunction in neurodegenerative disorders: a game of genes?. AIMS Molecular Science, 2015, 2(3): 211-224. doi: 10.3934/molsci.2015.3.211 |
[3] | Antonio Parisi, Sara Parisi . Autism, 75 years of history: From psychoanalysis to neurobiology. AIMS Molecular Science, 2019, 6(1): 20-26. doi: 10.3934/molsci.2019.1.20 |
[4] | Tsuyoshi Inoshita, Yuzuru Imai . Regulation of vesicular trafficking by Parkinson's disease-associated genes. AIMS Molecular Science, 2015, 2(4): 461-475. doi: 10.3934/molsci.2015.4.461 |
[5] | Alveena Batool Syed, James Robert Brašić . Nuclear neurotransmitter molecular imaging of autism spectrum disorder. AIMS Molecular Science, 2019, 6(4): 87-106. doi: 10.3934/molsci.2019.4.87 |
[6] | Amrit Krishna Mitra . Oxytocin and vasopressin: the social networking buttons of the body. AIMS Molecular Science, 2021, 8(1): 32-50. doi: 10.3934/molsci.2021003 |
[7] | Nikhita Bolar, Aline Verstraeten, Lut Van Laer, Bart Loeys . Molecular insights into bicuspid aortic valve development and the associated aortopathy. AIMS Molecular Science, 2017, 4(4): 478-508. doi: 10.3934/molsci.2017.4.478 |
[8] | Stephen Schultz, Dario Siniscalco . Endocannabinoid system involvement in autism spectrum disorder: An overview with potential therapeutic applications. AIMS Molecular Science, 2019, 6(1): 27-37. doi: 10.3934/molsci.2019.1.27 |
[9] | Sarah S. Knox . Gene x environment interactions as dynamical systems: clinical implications. AIMS Molecular Science, 2016, 3(1): 1-11. doi: 10.3934/molsci.2016.1.1 |
[10] | William A. Toscano, Jr., Hitakshi Sehgal, Emily Yang, Lindsey Spaude, A. Frank Bettmann . Environment-Gene interaction in common complex diseases: New approaches. AIMS Molecular Science, 2014, 1(4): 126-140. doi: 10.3934/molsci.2014.4.126 |
The present article is a theoretical contribution suggesting a simple model of the dynamics involved in the development of neurodevelopmental disorders and the phenomenon of autism offering an explanation of why different individuals have different onset of manifest disease. The model relates to present genetic and epigenetic evidence and previous theoretical models. The dynamic model applies an individualized transdiagnostic and dimensional approach integrating several levels of influence involved in the dynamic interaction between an individual and their environment across time. The dynamic model illustrates the interaction between a basic neurobiological susceptibility, compensating mechanisms, and stress-related releasing mechanisms involved in the development of manifest clinical illness. The model has a particular focus on the dynamics of neurocognitive processes and their relationship to more basic information, psychological and social processes. A basic assumption guiding the model is that even quite normal events related to typical development may increase the risk of enduring stress in cognitively vulnerable individuals, further increasing their risk of developing manifest clinical illness. The model suggests that genetic variation, endogenous epigenetic processes of development, and epigenetic changes influenced by physical/chemical and social environmental risk and resilience factors all may contribute to phenotypical expression. A genetic susceptibility may translate into a biological susceptibility reflected in cognitive impairments. A cognitively vulnerable individual may be at increased risk of misinterpreting sensory inputs, potentially resulting in psychopathological expressions, e.g., autistic symptoms, observed across several neurodevelopmental disorders. The genetically influenced experience of an individual relates to cognitive phenomena occurring at the interfaces between the brain, mind, and society. The severity of clinical illness may differ from the severity of a disorder of reasoning. The dynamic model may help guide future development of personalized medicine in psychiatry and identify relevant points of intervention. A discussion of the implications of the model relating to epigenetic evidence and to previous theoretical models is included at the end of the paper.
The complex transdiagnostic and dimensional nature of autism as a pathological phenomenon, and changes across time in the definition of autism as a clinical construct, are both reasons why a focus on autism may be a relevant starting point for a discussion of the basic dynamics involved in the development of neurodevelopmental disorders. The prevalence of autism spectrum disorders (ASD) has changed over time from approximately 0.1% at the beginning of the century to approximately 1% at present [1]–[4]. The reasons for this marked change may be attributable to several sources, including both true etiological factors and cultural factors [5]–[7]. The effort to identify and understand the reasons for this marked increase in prevalence is important. Potentially, such knowledge may challenge our present understanding of autism and the assumptions guiding autism research, thereby yielding an impact on the effort of identifying the etiological causes and molecular mechanisms of ASD and related neurodevelopmental disorders.
The task to identify mechanisms and etiological causes involved in the development of ASD is of major importance. Insights resulting from such an effort may have great impact on future treatment strategies. Potentially, such knowledge may improve the prognosis of affected individuals, increase their quality of life (QoL), and point to future strategies for prevention. The task, however, is complex and is complicated by the heterogeneous nature of ASD and the many coexisting or comorbid conditions that are often present together with ASD [8]–[11].
A major challenge for autism research is to explain why only some but not all at-risk individuals develop clinical symptoms surpassing the diagnostic threshold for an ASD. This represents a dimensional challenge. Genetic risk factors for ASD may be present not only in affected individuals but also in unaffected individuals, a finding observed in the family members of diagnosed individuals [12] as well as the general population [13]. Such evidence challenges the prevalent categorical diagnostic approach and indicates that a dimensional approach may be more relevant.
Furthermore, genetic markers for ASD can be observed across different neurodevelopmental disorders, indicating that these markers are associated with more than one disorder [9],[14],[15]. Such evidence reveals another major challenge for autism research, a transdiagnostic challenge. The transdiagnostic and dimensional challenges identified at the genetic level are also observable at the phenotypic level [8],[10]. Moreover, they can be observed at different translational levels of psychopathology, e.g., at different levels of information processing at the neurocognitive level, identified as neurocognitive impairments, or the neural level of activity, observed as psychophysiological deficits [16]. To what extent are differences in psychopathological expression explained by qualitative or quantitative differences in genetic vulnerability and to what extent do other factors contribute, including physical, chemical and social environmental risk and resilience factors?
The present paper for the special issue of AIMS Molecular Science on the molecular mechanisms of and therapies for ASD is a theoretical contribution. From a clinical perspective, the paper focuses on the dynamic, translational and transgenerational aspects of molecular mechanisms and offers an explanation of why different individuals have different onset of manifest disease. The aim of the present theoretical paper is to contribute with suggestions for how to develop a simple theoretical model of the complex dynamics involved in the development of neurodevelopmental disorders and the phenomenon of autism that may help explain the observed biological and clinical heterogeneity of ASD and their relation to other neurodevelopmental disorders, including schizophrenia. The ambition has been to sketch the design of a model that may account for the impact of neurobiological and psychological processes taking place at the individual level and their interactions with processes acting at the physical, chemical and social environmental levels. Assumptions guiding the model are that clinical symptoms are expressions of different phenomena related to the brain and that many different routes may lead towards the same overall clinical picture. Some clinical symptoms may more directly relate to basic brain phenomena—for example, anxiety, compulsive behavior and affective symptoms—whereas the relationship between the phenomena of autism and psychosis with the brain may be more indirect with these phenomena relating to cognitive phenomena taking place at the interface between the brain, the mind and society. Genetic and epigenetic mechanisms are suggested to be operating as the basic mechanisms integrating and translating information between all levels involved in psychodynamics across time. When designing the model, a central goal for the model was that it should be able to distinguish between processes related to a basic susceptibility, adaptive processes and decompensating factors involved in the development of manifest clinical illness. Furthermore, it should include illustrations of the dynamics over time embedded within a developmental context. Finally, the model should be able to distinguish between a neurobiological susceptibility and contributions from environmental risk factors (physical, chemical and social), discriminate among adaptive biological, psychological and social processes, and ultimately, separate biological, psychological and social decompensating factors.
The suggested dynamic model is the result of a further development of previously published theoretical ideas suggesting that autism may represent a transdiagnostic, dimensional construct of reasoning [16],[17]. These ideas—inspired by original ideas by Bleuler (1911) [18] and Piaget (1967) [19], and reinterpreted in light of present empirical evidence—include the suggestion of a neurodevelopmental approach, a cognitive link between ASD and schizophrenia, and revised concepts of autism and psychosis. The present author owes much inspiration to the sociologist Alberoni (1984) [20] and his concept of the nascent state. The nascent state is a psychotic-like state thought to be involved in the dynamics of the development of society. The concept has informed a central assumption included in the dynamic model, the idea that social dynamics may be an important driver in the psychopathological development of neurodevelopmental disorders. Furthermore, the concept has inspired a central hypothesis of the model suggesting that cognitive impairments with no measurable abnormal changes within the neurotransmitter systems may be involved in psychodynamics, see below. This hypothesis was also inspired by the results from psychological research conducted in the 1970's by a group of psychologists and psychiatrists exploring experiences of reality across early neurotypical development and different psychopathological states including psychotic and non-psychotic conditions [21],[22]. The results indicated changes in the experience of reality across early neurotypical development with a tendency to experience imaginary items as being real disappearing around the age of 6. Furthermore, these researchers found that psychotic patients compared with 6-year old children and non-psychotic patients had a tendency to experience non-existing items as being real, whereas the experience of the material world remained intact.
The basic hypotheses of the dynamic model assume that cognitively vulnerable individuals may be at risk for enduring stress resulting merely from events relating to typical development and that this may increase their risk of developing manifest clinical disease. At different time points and levels of cognitive complexity, cognitive impairments with no measurable abnormal changes within the neurotransmitter systems may distort the experience of sensory input and result in cognitive experiences that may reflect a distorted picture of reality. A basic disorder of reasoning may be common between ASD and schizophrenia, whereas differences in age-related symptom expression, the severity of cognitive impairments, comorbidity of clinical conditions, and focus on psychosis may be what separate ASD from schizophrenia at the clinical and phenomenological levels. Cognitive impairments may reflect information processing deficits resulting from a genetic susceptibility. An assumption guiding the dynamic model is that a basic disorder of reasoning associated with an inflexible way of thinking may be a common feature for the phenomena of autism and psychosis, with both phenomena relating to the experience of reality [16]. Whereas autism as a phenomenon relates to the reciprocal social behavioral and cognitive aspects of an experience, psychosis as a phenomenon focuses on how an experience perceptually relates to reality expressed in thought or behavior, with both phenomena expressing various, qualitatively different aspects of how an experience relates to reasoning. As such, both phenomena are expected to be observed across a clinical dimensional spectrum reflecting reasoning ranging from neurotypical expressions to pathological expressions such as those observed in ASD and intellectual disability. This suggestion may be consistent with findings from a recent population-based study of children focusing on alterations in mentalization and their association with psychotic experiences defined as psychotic symptoms in the absence of psychotic illness [23]. An exaggerated type of Theory-of-Mind, Hyper-Theory-of-Mind associated with psychotic disorders was related to psychotic experiences and delusional ideas. Hypo-Theory-of Mind associated with a diagnosis of ASD and negative symptoms was also associated with psychotic experiences indicating that both types of Theory-of-Mind may be involved in the development of psychotic phenomena. The authors concluded that different alterations of Theory-of-Mind may be suggested to impact different stages of development of psychosis.
From a basic assumption regarding autism as primarily an environmental condition, dating back to Bleuler (1911) [18], focus shifted towards a predominant view considering autism a neurobiological condition [1]. Today, there is an increasing awareness of the importance to integrate these two frameworks and to explore the significance of gene-environment interactions [7],[24],[25] and possible epigenetic mechanisms involved in the development of ASD [26]–[28]. Furthermore, there is an increasing awareness of the need to examine deviations in relation to expected normal variation across development [29]–[32]. Finally, culturally related factors may affect prevalence measures [5],[6]. The marked increase in the prevalence of ASD may be due to nonetiological factors, including reporting practices, increased awareness and availability of mental health services, as well as a broadening of the diagnostic criteria [5],[6]. However, a true increase in disease prevalence may also be due to etiological factors such as diverse environmental risk factors, e.g., exposure to toxins, maternal lifestyle, or parental age [5].
Epigenetic mechanisms are reversible [26],[27],[33]. Evidence for the involvement of epigenetic mechanisms in the development of neurodevelopmental disorders including ASD and the identification of relevant environmental factors that may be involved in epigenetic changes in these disorders are therefore potentially of great importance because they may turn out to be very relevant foci for future treatment and prevention. To the extent that experience is genetically influenced [34], and insofar the cognitive ability of an individual sets the limits for experience at the interface between brain and mind with social interaction taking place at the interface between an individual and their environment, a major implication of the suggested dynamic model is that genetically influenced individual experiences set the limits for social interaction.
Previous research on risk factors involved in the development of ASD have mainly focused on individual genetic or environmental risk factors, with only a few studies exploring the interactions between individual risk factors [11]. Twin studies have contributed with estimates of the contributions made by genetic and environmental risk factors, providing strong support for a major influence of genetic factors. A shared environmental risk for ASD, however, may also be important [24]. Furthermore, evidence from a large twin and family study indicates that nonshared environmental risk factors contribute to overall risk [12]. This finding is in line with the general assumption that most of the environmental variance in families is nonshared rather than shared, although little is known about the specific sources of nonshared environmental effects, in particular, the specific sources of nonshared experience outside the families [34].
Prenatal exposure to valproic acid and the rubella virus are examples of environmental factors strongly associated with ASD [11]. Other environmental risk factors for ASD, albeit with smaller effect sizes, include very low birth weight, extreme premature birth, and advanced paternal and maternal age. A family history of mental illness, birth in an urban area and diverse perinatal factors have also been associated with the risk of ASD [35],[36], although conclusions from studies on pregnancy-related exposures are inconsistent [37].
Despite very strong evidence for genetic involvement in the development of ASD and rapid progress in the identification of risk genes with new advanced genomic technologies, the majority of the genetic risk either remains unaccounted for or is estimated to derive from common inherited yet unidentified variants with an additive effect [38]. Multiple familial inheritance patterns exist, with etiological heterogeneity appearing to be the norm rather than the exception [9],[39]–[40]. Rare genetic risk factors with large effect sizes [41],[42] can only account for a very small number of cases of ASD, whereas common genetic variants with small effect sizes [9] likely account for most of the genetic risk in ASD [11],[43].
Common polygenic as well as rare de novo variation may both contribute to the risk for ASD, likely conferring risk in different ways [42]. Apparently, the genetic influences from both common variants and de novo mutation variants are additive. Whereas the de novo variant risk for ASD has a strong impact only within a limited number of cases, the common polygenic risk may be more consistently contributing to autism liability across all cases.
Increasing evidence suggests that ASD may share biological pathways with other neurodevelopmental disorders with variants in genes also found in these disorders, e.g., intellectual disability and schizophrenia [9],[14],[15],[44],[45]. Furthermore, ASD are strongly associated with social and communication trait variation in the population, with both common inherited and de novo variants influencing typical variation [13].
Epigenetic mechanisms may be the biological mechanisms that connect genetic and environmental factors, with most epigenetic evidence coming from preclinical animal research and studies on human brain cells [26],[28]. Increasing evidence from human population studies on epigenetics in neurodevelopmental disorders including ASD is now emerging [46],[47]. An original description of the concept of “epigenotype” comes from Waddington (1942), who defined epigenetics as the study of the developmental processes and the causal mechanisms by which genes of a genotype produce phenotypic effects [48].
Epigenetic regulation of gene transcription and expression involves processes modifying chromatin via deoxyribonucleic acid (DNA) methylation, histone modification and small noncoding ribonucleic acids (RNAs), so-called micro RNAs, without altering the DNA sequence [26],[27],[33]. Enzymatic processes modify the state of chromatin, with chromatin consisting of a histone protein-based structure with DNA wrapped around it. Several enzymatic processes, e.g., methylation, phosphorylation, acetylation, and DNA methylation reactions involving covalent modification of cytosines that are catalyzed by DNA methyltransferases, are involved in the modification of histone proteins [33]. Evidence suggests that DNA methylation is a dynamic, targeted and reversible process [33]. Histone modification enzymes interact with DNA methylating enzymes, thereby regulating gene expression. Gene expression only takes place in active regions of the chromatin associated with hypomethylated DNA. The pattern of methylation is gene- and tissue-specific. Suppression of gene expression via DNA methylation is associated with hypermethylated DNA and inactive chromatin.
To maintain the homeostasis of epigenetic information, a dynamic balance of the DNA methylation pattern and the chromatin structure is required [33]. Changes in epigenetic regulation caused by mutations in genes involved in epigenetic mechanisms and their regulation or caused by environmental factors or genetic programming affecting epigenetic regulation may affect this balance.
While the genome remains largely intact throughout the lifespan, the epigenome may change according to the developmental requirements and the physiological needs to adapt to the environment [25]. It is relevant to distinguish between two basic kinds of epigenetic changes that interact with each other, thereby affecting outcome [25]. Endogenously programmed changes include epigenetic changes related to developmental and aging processes, as well as genetic variation affecting the genes involved in epigenetic modification. Second, epigenetic changes occur in response to exogenous factors such as physical and chemical agents and a range of other environmental stressors, including psychological, behavioral and social stressors. The interactions between these processes are complex and may depend on developmental windows of sensitivity.
A study of volunteer monozygotic twin pairs has documented age-dependent epigenetic differences, with its results indicating that older twins are epigenetically different from younger twins, a difference not due to a general increase in the epigenetic variability in the older population [49]. The older twin pairs showed more than two times as many locus-specific DNA methylation differences compared to the younger twin-pairs. According to the authors, an explanation of the observed differences in epigenetic patterns in genetically identical individuals may relate to the influence of both external factors, e.g., lifestyle factors such as smoking and diet, and internal factors, e.g., aging [49].
Lifestyle factors, including substance abuse, and environmental factors affecting gametogenesis, reproduction, fetal life and parenting behavior may all potentially modify the epigenome [25]. Naturally occurring or synthetic endocrine-disrupting compounds, chemicals that affect endocrine function, hormones, receptors and signaling pathways, have potential or observed epigenetic effects.
Focusing on the epigenetic effects of prenatal environmental toxicants that may act as endocrine disruptors, Tran and Miake [47] report on findings from preclinical and human population studies on the epigenetic effects of maternal smoking, heavy metals, plastic-derived chemicals and organic pollutants. Preclinical studies suggest negative effects of environmental toxicants on neuronal cells and neurodevelopment with the molecular mechanisms not yet fully understood. Evidence from epidemiological studies may also indicate a relationship between maternal smoking, plastic-derived chemicals and heavy metals and an increased risk for neurodevelopmental conditions. Furthermore, alterations in DNA methylation related to maternal smoking, plastic-derived chemicals, and organic pollutants as well as heavy metals and the associated possible effects on genes involved in neurodevelopment suggest that epigenetic mechanisms may be involved in the observed associations between environmental toxicants and neurodevelopmental changes.
Nutrition, medication and prenatal infection are risk factors associated with neuropsychiatric disorder, for which epigenetic mechanisms are potentially involved; however, the relationship between the risk factors and epigenetics have not yet been established, and many of the factors account for only a small amount of the risk [50]. Furthermore, stress, trauma, and early life adversity are associated with effects on the hypothalamic–pituitary–adrenal axis (HPA axis) affecting the stress response and the homeostatic regulation of glucocorticoid levels. Preclinical, epidemiological and clinical studies suggest a strong link between exposure to stress and dysregulation of the HPA axis or susceptibility to neuropsychiatric illnesses. Glucocorticoids may directly influence DNA methylation and chromatin remodeling. Lee and Avramopoulos [50] suggest a model of the complex gene-environment interactions, focusing on circularity with genes determining phenotypes, phenotypes affecting behaviors, behaviors modifying environmental exposures, and environmental exposures modifying epigenetic marks and the function of genes.
Evidence from epidemiological studies and animal models suggests that gestational exposures to parental stress are strongly associated with neurodevelopmental disorders, including ASD, and that transmission of not only maternal but also paternal stress to offspring through epigenetic marks in the germ cell may be involved [51]. Although environmental exposure to prenatal stress and early life environment may influence long-term risk for a neurodevelopmental disorder, the risk for manifest clinical disease may depend on multiple interacting factors, combining a genetic predisposition with later environmental exposures experienced across a lifetime. Bale [51] suggests that a genetic susceptibility may be the first hit in a two- or three-hit model, with an environmental exposure to prenatal stress acting as a second hit, where the next stress-inducing event experienced during later points in life may tip the balance and trigger onset of manifest clinical disease. The model suggests that prenatal exposure to stress may trigger epigenetic programming in a context with increased genetic vulnerability, and together, the genetic vulnerability and epigenetic programming may increase sensitivity to later life events.
Several rare, severe neurodevelopmental conditions that are due to altered genetic imprinting or caused by mutations in genes that encode factors involved in epigenetic regulation are associated with autism spectrum behavior and intellectual disability [52]. Genomic imprinting refers to parent-specific epigenetic marks regulating the expression of genes via DNA methylation and histone modification in specific regions of the genome [52]. Angelman syndrome, a severe neurodevelopmental syndrome characterized by intellectual disability and developmental delay of motor skills and speech, results from an imprinting defect. Other examples of neurodevelopmental conditions associated with epigenetic alterations are Rett syndrome and Fragile X syndrome. Rett syndrome is an example of a group of disorders associated with intellectual impairment and ASD and caused by mutations in genes encoding different proteins involved in epigenetic regulation [52]. Gene mutations associated with Rett syndrome include mutations affecting a gene localized on the X chromosome encoding a protein binding to methylated DNA independent of the DNA sequence. This protein is responsible for the inhibition of transcription. Interestingly, the expression is especially high in the brain and rather specific for neuron maturation and, later, maintenance and modulation of synapses and dendritic complexity. Gene expression is associated with changes related to brain development with high expression during embryonic development, low expression at birth and increased expression thereafter. Fragile X syndrome is a clinical syndrome involving intellectual disability, delayed speech and psychomotor development, and autism spectrum behavior, which also results from a mutation in a gene located on the X chromosome [52]. Methylation of a polymorphic, unstable, noncoding repeated section of the gene and adjacent regulatory elements leads to repression of gene expression in this syndrome.
Clinical and preclinical evidence indicates that exposure to environmental chemicals may affect several proteins involved in normal brain maturation, e.g. Brain-Derived Neurotrophic Factor, with dysregulation of these proteins associated with ASD [28]. In animal models, organic chemicals have been associated with modulation of the expression of genes coding for oxytocin, and vasopressin, known to be involved in the modulation of social cognition and behavior [28]. Dysregulations in these brain neuropeptide systems have been suggested to underlie social dysfunctions in ASD. Epidemiological studies and preclinical research suggest that environmental factors including prenatal exposure to air pollution, vitamin D deficiency and anticonvulsant medication are associated with ASD, and observations of altered DNA methylation may indicate that epigenetic mechanisms are involved [28]. Vitamin D is associated with the regulation of genes involved in the control of inflammation, immunity, and cell development suggesting a possible role for these processes in the development of ASD. An abnormal metabolic profile of the methionine transmethylation and transsulfuration pathway associated with oxidative stress in ASD and related to susceptible genetic variants may indicate that alterations in gene expression due to multiple polymorphisms and environmental factors may interact and affect metabolic pathways, inducing a chronic metabolic imbalance [53]. A redox/methylation hypothesis of autism assuming that oxidative stress is initiated by environmental factors in genetically vulnerable individuals leading to impaired methylation and neurological deficits resulting from impaired synchronizing of neural networks has been proposed [54].
A large, recent case-control study aiming to identify DNA methylation biomarkers of ASD detectable at birth did not find any global differences in DNA methylation or identify any differentially methylated positions when comparing a group of neonates who later developed a diagnosis within the autism spectrum and a matched control group [45]. This study used DNA samples isolated from neonatal blood collected shortly after birth and included a case-control sample of 1316 individuals with equal numbers of ASD cases and controls. As an exploratory finding, the study found a significant association between increased polygenic burden for ASD and methylomic variation at specific loci.
A small cohort study of ASD patients with resulting rare variants examined in an independent, larger ASD sample aimed to identify methylation aberrations in an epigenome-wide association study of blood DNA and to explore the functional consequences on the blood transcriptome of a subset [55]. The results showed a significant association of ASD with the hypomethylation of rare genetic variants at several loci and identified a few clustered epimutations in single patients. The identified epimutations affected genes involved in neurodevelopment and function. The authors concluded that inherited alterations in DNA methylation from unaffected parents due to either genetic or epigenetic defects affect gene expression and contribute to ASD susceptibility, possibly in an additive manner. The study found no global methylation alterations, which is in line with the results from Hannon et al. [46] and consistent with the results from a small study on gene expression and DNA methylation levels in brain tissue from two brain regions conducted in a post mortem sample of subjects with ASD and matched controls [56]. This study found significant transcriptional heterogeneity in brains from autistic subjects and decreased expression of genes related to mitochondrial oxidative phosphorylation and protein synthesis.
In a recent study of monozygotic twin pairs, the results showed multiple differentially methylated regions associated with ASD, with variability appearing to be specific to ASD-discordant twin pairs. In this study, a genome-wide analysis of DNA methylation was conducted in a sample of twin pairs from a representative population cohort including twin pairs concordant and discordant for ASD, ASD-associated traits and no autistic phenotype. In line with the studies mentioned above, ASD was only associated with site-specific differences and not associated with systemic differences in global DNA methylation [57].
Another line of epigenetic research has focused on the task of unraveling the effects of maltreatment on the associated risks for mental problems including neurodevelopmental disorders and reactive attachment disorder [58]–[60]. In light of previous evidence indicating an overlap between individual disorders associated with maltreatment and their shared environmental and genetic predisposing factors, Minnis [58] suggests considering maltreatment-associated psychiatric problems (MAPP) a syndrome of overlapping complex neurodevelopmental problems in children who have experienced abuse or neglect early in life. The concept of MAPP may be an example of ESSENCE—Early Symptomatic Syndromes Eliciting Neurodevelopmental Clinical Examinations—a concept previously introduced by Gillberg [8]. In a cross-sectional twin study, this group of researchers, in line with their expectations, observed an association between childhood maltreatment and symptoms of neurodevelopmental disorders including ASD [60]. Interestingly, their data suggested that the association was mainly due to common genetic factors. Although childhood maltreatment was associated with an increased load of neurodevelopmental symptoms, the nature of the study design did not permit an investigation of directions of causality. Overall, the findings were not consistent with the assumption that maltreatment is responsible for the increased load of neurodevelopmental problems observed in children with a history of maltreatment.
Tissue-specific processes are influenced by cortisol signaling via transcription. Furthermore, cortisol affects genes directly by regulating and mediating the stress response of the HPA axis, thereby affecting the subsequent cortisol levels [50]. Glucocorticoids may directly influence DNA methylation and chromatin remodeling. Both intense traumatic stress and chronic stress may affect the HPA axis and glucocorticoid dynamics, leading to abnormal cortisol levels that can persist even in the absence of the initial stressor. Furthermore, individual perception and experience of a stressor may activate the HPA axis, resulting in the production and release of cortisol [50], and indicating a possible basis of a translational interaction between psychological and biological regulatory processes. The relationship between stress or cortisol-induced HPA-axis dysregulation and the resulting effect on cortisol dynamics may affect mood and emotions.
In general, evidence indicates that stress responses promote adaptation via responses of several interacting systems, neural, cardiovascular, autonomic, immune and metabolic systems, with most of the evidence coming from animal studies [61]. Complex, non-linear interactions take place with glucocorticoids, catecholamines (e.g., adrenaline, noradrenaline and dopamine), other hormones, pro- and anti-inflammatory cytokines and the parasympathetic nervous system all involved in regulation of stress responses. Stress hormones modulate function within the brain by changing the structure of neurons. Repeated stress causes changes in brain regions including hippocampus, prefrontal cortex and amygdala [61],[62]. The hippocampus is involved in cognitive function including memory and direction of attention and is a very sensitive and malleable region of the brain undergoing several adaptive changes in response to acute and chronic stress.
Evidence from epidemiological studies suggests that intrauterine exposures to stress are associated with an increased risk for neurodevelopmental disorders, including ASD [51]. According to the two- or three-hit model by Bale [51] this could be explained by the effects that prenatal stress may have on the fetal brain by triggering epigenetic processes that may modify the action of susceptible genes, thereby increasing sensitivity to later life events.
Evidence from animal models and in vivo experiments indicates that mechanisms of environmental programming exist, with scientific results demonstrating that persistent changes in behavior and physiology initiated by early fetal experiences may be mediated by epigenetic processes resulting in sustained changes in gene expression in the brain [63]. Differences in DNA methylation patterns may alter glucocorticoid receptor expression. Preclinical data further provide evidence that the molecular mechanisms underlying the effects of early experience on neural function may be reversible in adulthood, speaking to possible social and therapeutic implications [64]. Evidence supports the hypothesis stating that the DNA methylation pattern is dynamic even in postmitotic brain tissue with a steady state maintained by the state of chromatin acetylation [63].
In light of their findings from experiments on the effects of maternal stress on fetal experience and later behavioral phenotypic expressions, Meaney and Szyf [63] suggest that epigenetic processes may imprint dynamic environmental experiences on the genome, resulting in stable changes in the phenotype. They relate their results to biological evidence from similar findings of maternal effects on defensive responses to threat observed in plants and various animals. Such effects may follow exposure of the mother to the same or a similar kind of threat, indicating a possible translation of the environmental experience of the mother via epigenetic mechanisms of inheritance into phenotypic variation in the offspring. If this is the case, then maternal effects could transmit adaptive responses across generations.
In addition to epigenetic effects transmitted directly to the embryo and fetus, recent evidence indicates that both maternal and paternal adversity as well as glucocorticoid exposure before conception may have profound effects on the development and subsequent function of the HPA axis and related behaviors in offspring [65]. The effects depend on the timing and duration of exposure and are sex- and age-specific. Interestingly, emerging evidence suggests that the effects of both early maternal and paternal exposures can last across multiple generations with modifications of epigenetic mechanisms, e.g., DNA methylation, observed in gametes and peripheral and brain tissues of offspring transmitted via both maternal and paternal lineage [65]. Nevertheless, detailed knowledge about the mechanisms of epigenetic developmental programming is lacking.
The evidence from human studies on the epigenetic effects related to early life stress is sparse. A few studies document transmission of abnormal HPA-axis activity from mother to offspring many years after initial exposure to a traumatic event, e.g., war, hunger or genocide [66]. According to Stenz and coauthors [66], a challenge of this type of studies is how to interpret whether or not the transmission of traits from parents to offspring are acquired by learning from the traumatized parents or via biological transmission by epi-mutated gametes or an altered intrauterine environment [66].
In humans, prenatal exposure to maternal stress may affect epigenetic modification of the regulation of stress. A human study of prenatal exposure to increased maternal depressed mood identified an association between the methylation status in newborns of a human glucocorticoid receptor gene and increased stress-reactivity in the exposed infants at three months of age [67].
Studies of stress-sensitivity in ASD have convergent findings showing disordered HPA-axis regulation of stress in both high-functioning autism [68]–[71] and low-functioning autism [72]. More variability of salivary cortisol levels are found in children and adolescents with ASD compared to neurotypical controls [69],[71],[72]. Furthermore, higher levels of salivary cortisol with significantly flatter daytime and nighttime slopes are observed in autism [68],[69],[72]. According to the authors, possible interpretations of their results may include the suggestion that these findings relate to alterations in circadian rhythms possibly reflecting abnormalities in subcortical structures engaged in stress responsivity to novel stimuli and memory formation and retrieval related to personal experiences [70],[72]. The cortisol variability may result from heightened sensitivity to daily changes and unpredictable events in the environment, with elevated evening values perhaps reflecting the cumulative effects of daily stress [69].
The results show increased stress response in anticipation of stressful situations in both high-functioning and low-functioning autism [68],[72], a finding also observed in neurotypical controls [68]. Social stress may increase with age [70]. Not only children but also adults with ASD experience more stress compared to neurotypical controls [73],[74]. Observations have shown that perception of life as stressful may be associated with greater social disability without being directly related to the biological response to stress [74]. Such evidence may reflect the complex interaction of factors involved in social stress responses with the resulting adaptation or contribution to clinical symptoms possibly involving a psychological/biological connection.
With regard to the epigenetic nature of development, Courchesne [30] proposes a growth dysregulation hypothesis of autism suggesting that a pathologic dysregulation of growth during early life, yielding abnormal anatomical brain development with different brain structures following different schedules of pathological growth, ultimately may lead to the changes in behavior observed in autism.
Consistent with the growth dysregulation hypothesis, convergent genetic evidence from several lines of research relating to large data consortia all point to early neurodevelopment as a target for pathological processes involved in the development of ASD and related disorders, with a few examples given below.
A recent brain MRI study of the impact of the 16p11.2 distal copy number variants (CNV) associated with ASD and schizophrenia examined gene dose-response effects on measures of brain structure [75]. Independent of clinical and family history, the researchers observed negative dose-response associations with CNV on intracranial and basal ganglia volumes.
Another recent study, focusing on simplex autism (i.e., the sole family member with ASD), analyzed the contribution of variants in the noncoding, putatively regulatory portions of the genome after whole-genome sequencing (WGS) comparing probands negative for known pathogenic mutations and unaffected siblings [76]. The results showed trends for several de novo mutations in genes associated with nervous system development.
Furthermore, recent evidence from a nationwide cross-disorder association study suggests that a dysregulation of genes associated with common variants involved in neurodevelopment may contribute to a general liability for several different psychiatric outcomes, including ASD [77]. The authors identified novel variants predicted to regulate genes expressed in the developing neocortex. Their results drew on the analysis of DNA extracted from neonatal dried blood spot samples.
An analysis of published gene-expression microarray studies across five major neuropsychiatric disorders using brain transcriptomic profiling, a method analyzing quantitative, genome-wide molecular phenotypes across disorders, identified patterns of both shared and distinct gene expression across the conditions [78]. The authors found shared transcriptional dysregulation related to a polygenic single-nucleotide polymorphism (SNP)-based) overlap among ASD, schizophrenia and bipolar disorder, indicating that a major component of the observed gene-expression patterns may reflect biological processes coupled with underlying genetic variation. The degree of transcriptomic severity was larger in ASD compared to schizophrenia and bipolar disorder. Furthermore, the results showed a distinct pattern in ASD, with upregulated microglial markers indicating microglia involvement in the dysregulation of synaptic connectivity during neurodevelopment [78]. When extending their analysis, the authors identified disease-associated rare variants: rare, nonsynonymous de novo mutations in both autism- and schizophrenia-related cases.
Results from a human postmortem study suggest that development of the prefrontal cortex is associated with widespread changes in the cortical transcriptome across development, indicating that epigenetic changes occurring not solely during fetal life but also after birth and later across development may take place [79]. The study used genome-wide profiling of a histone mark associated with transcriptional regulation in neuronal chromatin in a small sample of postmortem brains from the late gestational period to 80 years of age. The results showed a majority of developmentally regulated peaks of rapid gains or losses of histone methylation during the transitions from prenatal to postnatal development, with slower changes observed during early and later childhood but only minimal changes observed later in life.
According to Menon [31], network analysis may contribute evidence about changes in the overall organization of the brain associated with neuropsychiatric disorders and may help identify affected subnetworks and networks compensating for dysfunction. Multiple brain regions interact over time. A triple neurocognitive network model on psychopathology may help relate findings into a common framework for understanding dysfunction in core neurocognitive networks across multiple neurodevelopmental disorders, including ASD [31]. This model predicts that a change in one core network may have an impact on the other networks, resulting in clinical manifestations that may extend beyond the primary deficit. Three core neurocognitive functional networks involved in higher cognitive functioning are included in the model. First, the central executive network rooted in the dorsolateral prefrontal cortex and the posterior parietal cortex is important in working memory and attention. Second, the salience network rooted in the frontoinsular cortex and dorsal anterior cingulate cortex is important for the detection and mapping of salient external inputs and internal brain events. The salience network also comprises subcortical and limbic areas involved in reward and motivation, including the amygdala, the substantia nigra and the ventral tegmental area. Finally, the default mode network rooted in the posterior cingulate cortex and medial prefrontal cortex is important for self-referential mental activity. Menon [31] suggests that changes affecting the balance among these three core neurocognitive networks are the fundamental mechanisms underlying cognitive dysfunction in many psychiatric disorders and report that changes in all three core networks have been associated with ASD.
Neurobiological evidence indicates that the phenotypic expressions of autism may result from an interaction of normal development with abnormal constraints at the cellular and molecular level reflected at the cognitive level [29],[80]. Some of the observed symptoms in ASD may reflect compensatory changes resulting from the interaction of normal cognitive development with abnormal neural information processing [29],[80]. Clinical symptoms of ASD may change over time and across individuals.
Evidence about the mechanisms responsible for changes in behavioral presentation during development and about how these changes relate to underlying cognitive and neural dysfunctions is sparse [81]. To be able to measure compensation and disentangle the contributions of compensation and cognitive impairments to behavior, it may be necessary to develop sensitive cognitive tools that exist independent of behavioral symptoms. Livingston and Happé [81] have created a working definition of compensation, defining compensation as the processes that contribute to an improved behavioral presentation of a neurodevelopmental disorder in the presence of persisting cognitive or neural core deficits. They point to evidence for compensation's improvement of social symptoms despite persistent theory of mind deficits in four subetaoups of ASD: individuals with good outcomes, at-risk individuals who transition away from an ASD phenotype, individuals with late diagnosis, and the presentation of autism in females. Several neurocognitive mechanisms are potentially involved in compensation, including greater intelligence quotient (IQ), with higher IQ in childhood being a strong predictor for good outcomes later in life. Another mechanism may be intact executive functions for those individuals who are not impaired. Finally, neural mechanisms that may involve recruitment of alternative networks, e.g., the prefrontal cortex, or hyperactivation of already involved networks are other possible mechanisms [81]. Compensatory resources may be limited under stress, anxiety or mental fatigue. Modulation of compensation by the environment is designated by Livingston and Happé [81] as “environmental scaffolding”.
How are the epigenetically mediated gene-environment interactions translated into the observed phenotypic expressions? To what extent do genetic and epigenetic mechanisms contribute to the development of manifest clinical illness? To what extent are psychopathological manifestations of illness preprogrammed from an early stage? What kind of mechanisms trigger the onset of illness? How are clinical appearance and time of onset related to how and when genetic and epigenetic events occur, e.g., preconception, intrauterine life, neonatal life and early development, childhood, adolescence, and life later on?
Theoretical models may potentially help guide research in the effort to answer such questions. Diathesis-stress models assume that adversity early in life may alter the development of genetically or biologically predisposed individuals by increasing their risk for disease later in life [63]. The relationship between early environment and later health may be mediated by parental effects on the development of neural systems underlying the expression of behavioral and endocrine responses to stress [63]. During perinatal life, adversity may alter development by promoting vulnerability, particularly for stress-related diseases such as depression and anxiety. Prolonged activation of the HPA axis and autonomic systems in response to stress are associated with chronic stress and persistent activation of stress hormones, thereby increasing clinical risk. The actions of glucocorticoids and catecholamines increase the availability of energy substrates in cells, a relevant response during acute stress conditions, yet one that is inadequate and destructive in high-risk conditions associated with chronic stress and persistent activation of stress hormones [63]. The brain plays a central role in perceiving, responding and adapting to experiences throughout life determining potentially stressful events, and controlling the behavioral and physiological responses, and the resulting lifestyle [61]. This is reflected in the two concepts “allostasis” and “allostatic load”, with the first concept referring to the active process by which an individual responds to daily events and maintains homeostasis, and the second concept referring to the long term effects of multiple interacting mediators acting on the body and brain resulting from adapting to the demands of daily life over time, and from the behavioral and physiological consequences affecting how an individual responds to stressors [61]. The “allostatic load” may result from inefficient management of allostasis, e.g. an inadequate response or lack of habituation to a recurrent stressor, or from enduring stress with genetic differences also playing an important role.
To help predict susceptibility to negative fear-response system reactions after trauma (disaster exposure), Weems [82] has developed a bioecological model of risk and resilience to traumatic stress relating to biological markers of the fear system. The model includes three critical components that together may predict the response of an individual and assumes that it is possible to understand biological correlates of disaster reactions in terms of basic biological markers of the fear-response system. The basic components of the model comprise (1) the qualities of exposure; (2) the genetic susceptibility to environmental influences, which may involve age-related critical periods; and (3) the degree to which the trauma is enduring coupled with the quality of the post-trauma environment [82]. Weems et al. [83] emphasize the importance of applying a developmental approach to understand the neurobiological response to traumatic stress. The investigators argue that the brain undergoes marked changes in childhood and that typical developmental changes may become affected by traumatic stress. The authors suggest an integration of approaches focusing on the symptom network with brain network approaches, and they refer to evidence indicating that changes in structural connections and distributed functional networks including the salience network, default mode network, and central executive network are associated with traumatic and severe early life stress.
Common features of the epigenetic models suggested by both Bale [51] and Lee and Avramopoulos [50] and the theoretical models of psychopathology focusing on the dynamics of stress referenced in the previous section [63],[82] are the inclusion of both molecular mechanisms and the effects of environmental factors. Several researchers acknowledge the need to integrate evidence from different fields in order to be able to understand and predict psychopathological courses and grasp the complexity involved in psychodynamics. This is apparent from several theoretical models. A few examples may illustrate how researchers have integrated evidence related to processes at different psychopathological levels (the genetic, neurobiological, cognitive, psychological, and social levels) in their explanatory models [14],[84]–[87].
The model of the complex relationship between biological variation and some major forms of psychopathology suggested by Craddock and Owen [14] is an example of a comprehensive model including several translational levels of psychopathology, genetic variation, biological systems, neural processes and several domains of psychopathology (cognitive impairments, negative symptoms, positive symptoms and mood symptoms). These authors suggest that future development of models of mental illness should integrate evidence from various levels across scientific disciplines. They base this suggestion on genetic evidence indicating that rare genetic variants with large effects may be involved in several different mental disorders across a disease spectrum ranging from intellectual disability, ASD and schizophrenia to bipolar and unipolar mood disorders [14]. They encourage the use of dimensional measures of key domains of psychopathology in addition to diagnostic categories and recognize that a single axis of psychopathological conditions in light of observed substantial individual variation is a simplification, e.g., individuals with ASD may also be suffering from mood pathology. Furthermore, they suggest research to focus on the relationship between psychotic disorders and neurodevelopmental disorders such as ASD.
Another example of a theoretical model is that developed by Ouss-Ryngaert and Golse [84]. According to these authors [84], complex models have a great influence on the way we conceive of and study development [84]. Their paradigm of “double reading” provides a new way to approach psychopathology, assumptions about etiology and whether clinical expressions are related to the mind or brain (thereby reflecting a psychic defense versus an impairment or defect, respectively). The paradigm assumes that for many developmental disorders, including ASD, the disorders both affect the constitution of the inner world and have a brain origin. Their framework makes it possible to consider a symptom as both reflecting an impairment and serving as a psychic defense. They apply the principle in their clinical evaluations of developmental disorders, where they propose an integrative clinical view [84]. According to Ouss-Ryngaert and Golse [84], there are several reasons for connecting neuroscience and psychoanalysis. They argue that contemporary concepts of development, in contrast to previous developmental models that were predictive, i.e., moving from a gene to a phenotype, now include early environmental influences and that psychoanalytic models have changed to become more rooted in early development. While referring to Meaney and Szyf [63] and Sameroff and Mackenzie [88], they state that new models of neuroscience focus on two major processes, epigenetics and plasticity, with development understood as a complex transactional system.
A third example of a comprehensive model is the developmental model of transgenerational transmission of psychopathology developed by Hosman et al. [85] The purpose of this model was to establish a scientific basis for a science- and practice-based preventive program for children of parents with a mental illness. These children are at high risk of developing a mental disorder themselves, with evidence indicating that the risk may depend on the presence of accumulated risk factors weighed against protective factors (resilience factors) that reduce said risk. The model describes the main domains of risk and the protective factors involved in the development of psychopathology relating to different stages of development [85]. The model differentiates between several interacting social domains and influences. Mechanisms of transgenerational risk include genetic risk, prenatal influences, parent-child interactions, family processes and social influences, all possibly affecting the outcomes.
A fourth example of a comprehensive theoretical model is a framework for conceptualizing the pathogenesis of neurodevelopmental disorders suggested by Sarovic [86]. This model includes endogenous and exogenous factors and their interaction focusing in particular on genetic susceptibility, cognitive compensation and environmental risk factors. Three interacting features are suggested to determine the observable behavioral phenotype: (1) a genetic susceptibility operationalized through a broader phenotype representing a spectrum of behaviors typical for a given disorder; (2) cognitive compensation operationalized through cognitive capacity representing the ability of an individual to recognize, respond to and learn from environmental cues and demands, and to cope; (3) risk factors operationalized through the neuropathological burden reflecting risk factors undermining brain development and function [86]. Risk factors include malnutrition, infection or neuroinflammation, pre- and postnatal stress, obstetric complications, toxins, somatic comorbidity and mechanical brain trauma [86]. Etiologies are suggested to be nonspecific and somatic insults to activate the HPA-axis and immune system. The model suggests that immune function and autonomic regulation modulate the effects of environmental insults, or induce insults of their own, potentially increasing the effects from environmental insults decreasing cognitive compensatory ability [86].
A final example of how evidence may give rise to theory comes from a study exploring the structure of psychopathology in data from a complete birth cohort [87]. The results indicate that one general psychopathology dimension, the p factor best explains psychiatric disorders across a period of 20 years spanning from adolescence to midlife [87]. Caspi et al. [87] point to four hypotheses that potentially may explain their findings: (1) a dimensional severity hypothesis suggesting that thought disorder symptoms are central as a dimension of severity; (2) a developmental hypothesis suggesting a developmental progression of severity; (3) an etiological hypothesis suggesting that the origins of p begin with genetic liability; and (4) a hypothesis about dubious specificity of etiological variables and other variables, e.g. biomarkers, consequences, treatments etc. Their analyses take into account dimensionality, persistence, co-occurrence, and sequential comorbidity of mental disorders. Higher p-scores were associated with worse developmental histories, more compromised brain function early in life, more life impairment and greater familiarity. An extremely high correlation between a thought disorder dimension and the general psychopathology dimension suggest that the thought disorder factor to a great extent may reflect general psychopathology indicating that a full description of the structure of common psychiatric disorders may have to include the dimension of disordered thought. Caspi et al. [87] suggest that the p dimension may represent low-to-high psychopathology and for future research to apply a translational and transdiagnostic approach to uncover the etiology of p.
Depending on their perspective, the five exemplified models emphasize different aspects of the psychodynamics involved in the development of psychopathology. Craddock and Owen [14] primarily focus on the molecular, biological and neural processes, although they acknowledge both the role that environmental factors may play in etiology and the importance of including a developmental perspective. Hosman et al. [85] primarily focus on the significance of psychological and social environmental risk and resilience factors related to developmental stages, yet they acknowledge the importance of genetic influences. Ouss-Ryngaert and Golse [84] emphasize the need to continuously bridge psychological and neurobiological approaches and to integrate evidence from genetic science while applying a developmental context, with development recognized as a complex transactional system. Sarovic [86] focuses on the impact of polygenic risk and of pregnancy-related and early postnatal risk factors and their effects on brain development, including a focus on how risk factors modulate diagnostic risk through their effects on cognitive compensation. The model includes immune function and autonomic regulation as factors modulating the impact of environmental factors, or by themselves acting as risk factors. A major strength of this model is the focus on operationalization. Finally, Caspi et al. [87] emphasize the need for an open-minded transdiagnostic, dimensional approach that ignores the idea of specificity. They acknowledge the importance of a translational approach including measurements across genetic, neural, cognitive and environmental domains, and the need to follow measures across time focusing on developmental progression of severity with thought disorder symptoms suggested to be the core symptoms of psychopathology.
The present dynamic model may add to this pool of theoretical literature by suggesting frameworks within which to understand the psychodynamics involved in the development of mental illness as well as by searching for a common language with which to guide the collaborative effort between the scientific communities engaged in this task. The present contribution may provide yet another perspective that may inspire future research engaged in the task of unravelling the dynamics of the development of ASD and related neurodevelopmental disorders.
Results from preclinical research indicate that environmentally programmed effects reflected in altered DNA methylation early in life can be reversed by pharmacological activation later in life and may therefore be modified by environmental factors [63]. The reversible nature of epigenetic mechanisms makes them relevant to consider as possible targets for both the treatment and prevention of psychiatric disorders. Two overall approaches to treatment and prevention are prevalent: (1) an approach focusing on the options to develop molecular and pharmacological interventions based on the insights from genetic science, and (2) an approach focusing on how to apply environmental actions in therapy and prevention.
An example of a discussion on how to develop interventions based on evidence for molecular mechanisms is given by Shork et al. [77]. These researchers reflect on possible implications for the development of pharmacological intervention related to increasing evidence suggesting that genetic variants associated with neurodevelopmental disorders are involved in early neurodevelopment, with susceptible genes affecting synapse and calcium channel biology during neuronal proliferation, migration and the establishment of brain circuits [77]. According to these researchers, such observation makes it critical to consider the developmental stages during which the susceptible genes are expressed in order to be able to target the molecular pathologies.
Epigenetic drugs targeting DNA methylation and histone deacetylation enzymes may have potential in the treatment of psychiatric disorders [33]. Their use, however, may not be without challenges, with specificity and risk of unintended global side effects being a main challenge [33]. Knowledge about the complex epigenetic machinery and how epigenetic mechanisms are involved in specific psychopathological processes leading to disease is essential in order to be able to develop targeted epigenetic therapy [33].
An aim of the epigenetic autism research by Ouss-Ryngaert and Golse [84] is to examine how pharmacological treatment on the one hand and environmental action on the other may change the brain's state. They hypothesize the different treatments, such as psychotherapy and medication, may have similar effects, even though they work through different mechanisms [84]. These researchers assume that epigenetic processes include the effect of relational actions and predict that new findings in neuroscience will change the way psychoanalysts think and treat their patients; they further hope that their own approach will change the way neuroscientists think and affect neuroscientists' choice of experimental paradigms.
Studies with the aim of identifying relevant environmental interventions aiming to reduce stress—and, as such, possibly affecting epigenetic processes—has been performed by Bishop-Fitzpatrick and coresearchers [89],[90]. These authors have examined the association between perceived stress, QoL and reports of social support, and recreational activity in autism. Results showed significantly lower levels of social support and QoL in adults with ASD compared to neurotypical controls, with direct effects of social support and perceived stress on QoL in adults with autism [89]. Furthermore, results indicated that self-reported recreational activities moderated the relationship between perceived stress and QoL, whereas social activities did not [90]. Based on their findings, the authors expect that interventions that focus on teaching adults with ASD to manage stress may help improve QoL [89],[90], and they suggest creating opportunities for participation in recreational activities [90].
The question of whether epigenetic drugs targeting DNA methylation or environmental actions possibly affecting altered DNA methylation may be relevant for the treatment and prevention of ASD cannot be answered in light of the present evidence.
Of possible relevance for future choices in which approach to apply, Yehuda et al. [91] point out that it is important to distinguish between whether epigenetic alterations are associated with preconception effects or result from the contribution of in utero effects on fetal development associated with the maternal state. Although these researchers focus on the effects of trauma on later development of Post Traumatic Stress Disorder (PTSD), their reflections may be of potential interest also for neurodevelopmental disorders including ASD, as their focus on the effects of trauma relates to the possible effects of stress on offspring behavior, which are also relevant for these disorders [91]. In contrast to epigenetic effects resulting from direct maternal transmission that may affect fetal development, the epigenetic alterations associated with preconception transmission are unassociated with maternal state during gestation or later parenting influences. Therefore, they may be susceptible to control and change. As such, transgenerational epigenetic markers transmitted through germ cells may be malleable, and epigenetic influences may be able to contribute to resilience as an alternative to vulnerability. Moreover, learning and changing experiences across a lifetime may have an effect on observed epigenetic changes in offspring and are likely to vary across the lifespan depending on environmental influences. Therefore, according to Yehuda et al. [91], in addition to research that focus on how molecular mechanisms influence individuals and their offspring, it is also important to focus on the effects of human experience and growth [91].
In light of the presented evidence, both pharmacological agents as well as environmental interventions have potential roles in the future treatment of ASD and related neurodevelopmental disorders. If the suggested dynamic model and associated hypotheses are able to predict psychopathological courses of illness, time of onset, and the dynamics involved, then the model may contribute with new knowledge of possible significance for the effort of developing personalized medicine in psychiatry.
The present article is a theoretical contribution representing a further development of previously reported theoretical ideas suggesting that autism may represent a transdiagnostic, dimensional construct of reasoning [16],[17]. In light of the present genetic and epigenetic evidence, previously suggested hypotheses are included in a simple dynamic model illustrating the dynamics involved in the development of neurodevelopmental disorders and the phenomenon og autism. Examples of various possible courses of illness suggested by the model, and how they may depend on etiology and the mechanisms involved, are illustrated. Finally, a discussion of the implications of the dynamic model is included in the last part of the paper relating to epigenetic evidence and to previous theoretical models of the complex mechanisms involved in the development of neurodevelopmental disorders.
The dynamic model illustrates the dynamics involved in the development of clinical symptoms across time, and how clinical symptoms at any given time relate to an environmental context and a given developmental stage and history. Depending on approach, clinical symptoms may either be classified as belonging to specific disorders, sorting the clinical symptoms and behavior into clinical syndromes having prognostic value, or symptoms may be assumed to reflect expressions of different more basic brain related phenomena possible to identify at any given time and expressed in behavior depending on the genetic set up and associated neurobiological vulnerability, compensating mechanisms and environmental risk, and as such reflecting transdiagnostic and dimensional phenomena independent of descriptive categorical diagnoses. In both cases, the clinical symptoms develop and are expressed as the result of a dynamic interaction between a basic genetically related vulnerability affecting the development and function of the brain, the ability of an individual to compensate, and the effects of the physical, chemical and social environmental influence on development.
Embedded in a developmental context, the dynamic model distinguishes among three different kinds of mechanisms involved in the development of manifest clinical disease: (1) a basic neurobiological susceptibility; (2) individual compensation or compensating mechanisms by the social environment; and (3) releasing mechanisms, including physical, chemical and social environmental stress-related factors.
In the following section, examples of different clinical courses across development predicted by the dynamic model are illustrated, and a simple translational model illustrating brain dynamics, gene-environment interactions, and the transdiagnostic and dimensional challenge is presented. For the applicable symbols, see Figure 1.
Transient clinical symptoms may occur within the normal range across the course of neurotypical development, e.g., feeling tense, anxious or having discrete compulsive behavior. An illustration of a model of the development of transient clinical symptoms within the normal range across the course of normal development is included in Figure 2.
More enduring clinical symptoms, outside the normal range of what may be expected according to neurotypical development, may result from pathological processes.
Traumatic or adverse events may initiate processes resulting in clinical manifestations across development, as shown in Figures 3a and 3b. Different stages across each course are illustrated. Across a neurotypical course of development, transient stress resulting from adverse events or trauma may result in either no or transient clinical symptoms (see Figure 3a). Recurrent and enduring stress may increase stress reactivity, possibly via epigenetic processes, and may initiate pathological courses resulting in clinical symptoms or manifest illness, e.g., anxiety disorders or depression (see Figure 3b). The dynamics illustrated are simple and do not include involved molecular mechanisms, potentially compensating mechanisms, or possible effects of stress on cognition.
A basic assumption of the dynamic model is that normal events related to development may initiate processes resulting in clinical manifestations in cognitively vulnerable individuals who reach the limits of their cognitive ability. According to the model, the diagnostic categories of ASD and schizophrenia are reflecting clinical conditions in which the rationale of an individual's behavior may differ qualitatively from that of the social environment due to different experiences resulting from a basic neurobiological susceptibility reflected in cognitive impairments [16],[17]. Cognitive and social challenges increase across the course of typical development. The dynamic model predicts that some individuals at different levels of cognitive complexity may reach the limits of their cognitive abilities, increasing their risk of experiencing enduring stress, which further increases their risk of developing manifest clinical illness above the diagnostic cut off for a disorder (see Figure 4a). This figure illustrates the basic psychodynamics involved in the development of neurodevelopmental disorders including ASD and schizophrenia. The neurobiological susceptibility may affect an individual's ability to readjust to new expectations, and other requirements related to development. Subtler cognitive deficits may go unnoticed. Recognition of an underlying vulnerability may not occur until after manifest disease appears in the form of obvious clinical manifestations, such as compulsive behavior, anxiety, depressive symptoms, changes in social behavior or psychotic symptoms, resulting from enduring stress. Furthermore, stress triggered by major life events and trauma may worsen already present cognitive impairments. A combination of the mechanisms illustrated in Figures 3a, 3b and 4a are therefore likely to be involved in the development and course of mental illness across a lifespan.
Depending on the course of neurodevelopment and on individual cognitive resources, some individuals may be able to compensate for underlying cognitive and neural dysfunctions (see Figure 4b). Social and cognitive challenges, however, increase across the course of development, further increasing the risk for clinical symptoms. Individual compensation may reduce the risk for clinical manifestations for some time. Along with an increase in cognitive and social challenges, however, transient stress may arise and may cause transient clinical symptoms outside the normal range, e.g., anxiety, depressive symptoms, and social withdrawal. If stress continues, this may increase the risk for more enduring clinical symptoms and the development of manifest clinical illness. Enduring stress may affect the ability to compensate and result in more enduring clinical symptoms, ultimately resulting in manifest clinical illness. In addition to events related to normal development, major life events and trauma may contribute to the psychopathological course.
Rough illustrations with suggestions about gene-environment interaction and epigenetic effects over time and how they may act as modifiers or mediators when modeling the effects on the brain and affecting the psychopathological courses of neurodevelopmental disorders are shown in Figures 5a, 5b, 5c, 6, and Tables 1 and 2. As illustrated in these figures and tables, different courses of illness may depend not only on the nature of a more basic genetic vulnerability, be it either inherited or acquired. Environmental influences across development and associated epigenetic modification affecting brain development and function or altering stress-sensitivity may affect the risk of developing manifest clinical symptoms and illness. Genetic variation and the endogenous epigenetic processes of development and aging, as well as exogenous epigenetic changes affected by the influence of environmental risk and resilience factors, may all contribute to phenotypical expression.
An individual interacts with their social and physical/chemical environment (see Figure 5a). The brain may act as a filter of information as it translates environmental and internal sensory stimuli into experiences of the mind. Epigenetic modulation is at the core of this translation with common gene polymorphism moderating the influence of environmental risk factors (see Tables 1 and 2). The resulting epigenetic effects depend on time of exposure and may involve effects on neurodevelopment, and function, modulation of stress-sensitivity, effects on social cognition and behavior, as well as direct tissue related effects on behavior, e.g., effects resulting from adaptative stress responses involving the neural, cardiovascular, autonomic, immune and metabolic systems. A genetic susceptibility may translate into a biological susceptibility, e.g., information processing deficits reflected in cognitive impairments. A cognitively vulnerable individual may be at increased risk of misinterpreting sensory inputs. This risk may depend on both environmental risk and resilience factors and on genetic influences related to an individual, with the severity of disordered reasoning predicted to increase with increases in the neurodevelopmental pathological load. Misinterpretations related to a disordered reasoning, e.g., deficits in social cognition or psychotic experiences, may represent psychopathological expressions of a reasoning disorder. Depending on the neurobiological susceptibility, developmental course and clinical state, clinical symptoms reflecting such misinterpretations may develop, e.g., deficits in Theory-of-Mind such as Hypo-Theory-of-Mind or Hyper-Theory-of-Mind, or positive or negative psychotic symptoms. According to the dynamic model, development of manifest clinical illness requires that clinical symptoms are recurrent or enduring and that they cause suffering.
The dynamic model suggests that clinical symptoms associated with stress, resulting from activation of the HPA-axis, e.g., anxiety, compulsive behavior and affective symptoms are predicted to be present from early on, and recurrently or continuously across the course of neurodevelopmental illness depending on the severity of clinical illness. Furthermore, cognitive phenomena, e.g. deficits of mentalization, impairments in the ability to integrate inputs coherently or in regulation of attention, or executive dysfunctions may all reflect information processing deficits resulting from a neurodevelopmental genetic susceptibility predicted to contribute to the core symptoms of neurodevelopmental disorders. The cognitive impairments are present and may be observed before the development of manifest clinical illness. The cognitive phenomena take place at the interface between the brain, the mind and society (see Figure 5a). The phenomena of autism and psychosis are both clinical expressions of qualitatively different aspects reflecting how an experience relates to reality with both phenomena predicted to result from a basic disorder of reasoning associated with an inflexible way of thinking [16]. Autism as a phenomenon relates to the social cognitive and behavioral aspects of an experience. Psychosis focuses on how an experience perceptually relates to reality expressed in thought and behavior. Both phenomena can be observed across a clinical dimensional spectrum reflecting reasoning ranging from neurotypical expressions to pathological expressions (see Figure 5a and 5b).
Clinical psychopathological expressions of misinterpretations are present across several developmental disorders including intellectual disability and are, as such, transdiagnostic, psychopathological expressions (see Figure 5b). From a categorical point of view, observations of concrete misinterpretations as reflected in negative psychotic symptoms and Hypo-Theory-of-Mind are frequent in ASD, whereas elaborated abstract misinterpretations as reflected in positive symptoms and Hyper-Theory-of-Mind are frequent in psychotic disorders, including schizophrenia (see Figure 5c).
Environmental stressors | Mechanisms |
Physical/chemical agents:
|
|
Social stressors:
|
|
Mechanisms | Type of effects |
Preconception exposure transgenerational genomic transmission |
|
|
|
|
|
Intrauterine exposure maternal transmission of environmental risk |
|
|
|
|
|
|
|
Infant exposure transmission of environmental risk |
|
|
|
|
|
|
|
|
|
Childhood exposure Adolescent exposure Adult exposure transmission of environmental risk |
|
|
|
|
|
|
|
|
|
|
|
|
|
The severity of illness may differ from the severity of a disorder of reasoning reflected in the dynamic model by the distinction between a basic neurobiological susceptibility and manifest clinical illness. In addition to the cognitive symptoms resulting from a basic neurobiological susceptibility and reflected in a disorder of reasoning, further cognitive symptoms, e.g., impairments of working memory or executive dysfunctions, may develop as a result of the effects of recurrent and enduring stress on brain cognitive function. The contribution of these stress-associated cognitive impairments added to an already present cognitive vulnerability is predicted to be involved in the psychopathological processes finally resulting in manifest neurodevelopmental clinical illness. They may worsen already present cognitive impairments and affect the ability to compensate.
The dynamic model illustrates the dynamics of interactions among processes taking place at and across several different translational levels: the genetic, biological, cognitive, psychological and social levels. The model has a particular focus on the dynamics of neurocognitive processes and their relationships with more basic information processes related to brain structure and function on the one hand and their relations to the psychological and social level on the other.
Epigenetic effects initiated by physical, chemical and/or social environmental risk factors may affect brain development and function, modify the level of stress reactivity, or directly affect behavior via direct effects on tissue, and may depending on the genetic and environmental risk load affect the time of onset of clinical manifestations (see Figure 6). At different stages across development, environmental risk factors may trigger epigenetic mechanisms that may affect neurodevelopment, alter stress-sensitivity or affect behavior (see Tables 1 and 2). Genetic variation and epigenetic processes related to preconception exposure, intrauterine exposure, and environmental exposures early and later in life may all affect the psychopathological course of illness, thereby contributing in various ways. The relative contribution from physical/chemical risk factors and social environmental risk factors may change across time with the relative importance of social risk factors suggested to increase across time. Lifestyle related behaviors influenced by stress associated behavioral responses contribute to the clinical manifestations.
Several factors related to the genetic and environmental risk load may affect time of onset. The genetic load may be reflected in both the quality and quantity of the genetic susceptibility with Loss of Function mutations and a large polygenic risk score predicted to increase the risk of clinical manifestations and early time of onset. The environmental risk load may be reflected in epigenetic marks associated with susceptible genes with effects depending on time of exposure as well as the quality and quantity of the environmental risk factors and the presence of resilience factors counteracting the effect of risk. Prior to conception, transgenerational transmission may affect future stress-sensitivity via epigenetic programming. Across development, prior epigenetic modification altering stress-sensitivity and modifying the effect of risk genes may be expressed and moderate the influence of environmental risk factors. Across time, the accumulated epigenetic effects of environmental risk factors may increase stress reactivity and the risk for manifest clinical illness. Prior epigenetic modification combined with an increase in environmental risk factors may result in early manifestation, e.g., of high-functioning ASD. On the other hand, environmental resilience factors may decrease stress reactivity and the risk for manifest illness with environmental resilience factors postponing clinical manifestation, e.g., of high-functioning ASD. Furthermore, mechanisms of individual compensation or social mechanisms of support, including scaffolding and environmental social support are predicted to postpone the onset of manifest neurodevelopmental clinical illness. Some examples of environmental influences predicted to affect the development of manifest clinical symptoms follows below. Toxicants may induce rare de novo mutations involved in early neurodevelopment during fetal life or induce epigenetic effects that alter stress sensitivity during fetal life. Pre- and postnatal stress, obstetric complications and early maltreatment may activate the HPA axis, initiating epigenetic processes that in turn modify the action of susceptible genes. Enduring social challenges, e.g., bullying may activate the HPA axis and initiate epigenetic processes. Finally, the dynamic model suggests that even innocuous effects, e.g. of unrealistic expectations related to development, or reaching the level of cognitive ability and being unable to adjust may activate the HPA-axis and result in epigenetic effects contributing to the risk of developing manifest neurodevelopmental illness. The parent-child interaction is only one of several social interaction contexts that may affect an individual across a lifetime.
Figure 6 illustrates how epigenetic effects may modify the course of illness depending on gene-environment interactions resulting from the combined contributions of genetic factors and environmental risk factors across the course of development. In most cases, for manifest clinical illness to occur, several events have to take place. This may be characteristic in most of the cases where polygenic risk from genes associated with common variants is prevalent. In contrast, in the rarer cases resulting from genetic syndromes and gene mutations with large effects, manifest clinical illness may be present right after birth.
The suggested dynamic model is an example of a deductive approach to neurodevelopmental research guided by several integrated hypotheses that may be tested in future research. The model includes neurobiological aspects of mental illness as well as cognitive, psychological and social dynamics. Mechanisms working at different levels assumed to be involved in the development of manifest clinical illness are integrated into an overall coherent theoretical framework that also includes hypotheses about the significance of neurodevelopment, development across time and interaction with the environment.
As suggested by the dynamic model, the association between a neurodevelopmental genetic load and clinical psychopathological expression may not be straightforward. Rather, the association may depend on the complex interaction of factors acting at and across many different levels over time. The dynamic model may be consistent with several models of epigenetic dynamics involved in the complex interaction among genetic, epigenetic and environmental factors previously suggested by several authors [25],[50],[51]. Consistent with the idea of a three-hit model [51], the dynamic model distinguishes between a more basic vulnerability and later events that trigger the development of manifest clinical illness. As with the three-hit model, the dynamic model assumes that the mechanisms by which early life events contribute to the development of neurodevelopmental disorders may involve complex interactions between a more basic genetic susceptibility, environmental factors, and epigenetic programming [51]. In line with Latham et al. [25], the dynamic model suggests that various courses of illness are possible with several possible routes, and the disease threshold and courses of illness may depend on changes in the dynamic relationship between an epigenetic state and environmental factors across time. Furthermore, the dynamics involve complex gene-environment interactions that include interacting endogenous processes of genetic variation, development and aging, as well as exogenous processes of environmental influences affecting phenotypic expression across the lifespan, per Latham et al. [25]. The dynamic model emphasizes that an individual engages with their social and physical/chemical environment in mutual interaction (see Figure 5a). This may be consistent with Lee and Avramopoulos's [50] assumption that not only is the phenotype affected by environmental factors but also the phenotype itself may affect the behaviors of an individual, thereby influencing how an individual modifies their environment. According to these authors, the environment may alter genes through mutation or, more commonly, through epigenetic modification [50].
As previously suggested and as reflected in the dynamic model, there may be a need to distinguish between psychopathology representing manifest clinical illness and psychopathology representing a more basic neurobiological susceptibility [16].
Overall, convergent evidence from preclinical, epidemiological and genetic studies suggests that both common and rare genetic variants and associated genes are involved in fetal neurodevelopment. Despite different approaches and foci, various analyses of the functional implications of gene loci associated with ASD suggests that risk variants and associated genes may be involved in processes relating to brain development and neuronal function [9],[76]–[78]. Such findings may indicate that a genetic susceptibility translates into a biological susceptibility, thereby increasing the risk for the later development of mental disorders. Furthermore, the observed association between susceptible genes related to early neurodevelopmental processes and neurodevelopmental disorders appears to be consistent with preclinical evidence indicating that genetic and epigenetic processes affecting neurodevelopment may depend on developmental windows of sensitivity [25].
The exploratory finding by Hannon et al. [46] of an association between methylomic variation at specific loci in the blood at birth and an increased polygenic burden for ASD may indicate that epigenetic modifications of susceptible common variants are involved in the early stages of the development of ASD, potentially contributing to susceptibility. Even so, the mechanisms linking DNA sequence variation to alterations in DNA methylation and other epigenetic mechanisms are not well understood [46].
The results by Hannon et al. [46] showing little evidence of an association between global DNA methylation at birth and later ASD may be in line with the suggestion that a genetic and associated biological vulnerability—even in absence of manifest clinical illness—can be present at birth. This suggestion is consistent with the assumption of the three-hit model by Bale [51] and the prediction suggested by the dynamic model that postnatal future events possibly involving epigenetic processes may occur throughout life and may further contribute to dynamics resulting in later development of manifest clinical illness.
The findings by Schork et al. [77] indicating that molecular mechanisms affecting neurodevelopment are involved in the early development of ASD may be consistent with the growth dysregulation hypothesis proposed by Courchesne [30]. Shork et al. [77] suggest targeting future investigations of gene-environment interactions by focusing on the overlap in timing for the actions of genetic and environmental susceptibility factors and considering the developmental course of pathological susceptibility implied by associated germline variants partitioning the risk; these are a critical next step in translational research.
In light of the present genetic and epigenetic evidence, the answers to several questions appear to be important in order to be able to predict how and when various genetic and epigenetic processes contribute to a psychopathological course of illness and time of clinical onset.
How does the balance between the relative contributions from genetic variance and epigenetic processes affect the clinical course of illness and the time of clinical onset? To what extent do the genetic load and type of genetic susceptibility affect a clinical course of illness? To what extent do morphological changes in the brain related to genetic effects on early neurodevelopment affect the time of clinical onset? How does the continuous development of the brain across time affect brain changes related to those genetic effects that affect early neurodevelopment? To what extent do preprogrammed epigenetic processes, e.g., those related to development and aging, contribute to the development of manifest clinical illness and to the time of clinical onset?
The finding by Fraga and coauthors [49] suggesting that age-dependent epigenetic differences in monozygotic twins are not solely explained by intrinsic, preprogrammed epigenetic processes of aging is important. The possible indication that external factors across time can also modify phenotypical expression may imply that external factors across time are also likely to be involved in psychopathological processes. Insofar as this is the case, it may be reasonable to assume that external factors can affect phenotypic behavioral expression in vulnerable individuals, potentially resulting in manifest clinical illness.
How are the processes of DNA methylation and other epigenetic processes related to pathogenesis involved in the development of a prior neurobiological susceptibility and to the time of first clinical appearance? Is it possible to distinguish between the contributions of intrinsic epigenetic processes modifying the expression of susceptible genes and the contribution of extrinsic epigenetic processes influenced by external factors? How do these processes interact across time, and how do they relate to morphological changes of the brain, or to stress-related reactions mediated by the HPA axis or other hormonal systems, and their associated functional disturbances?
To be able to understand the biological predictors of ASD in order to predict clinical trajectories, the answers to such questions appear to be essential. In the following sections, a discussion of the dynamic model in light of the reported genetic and epigenetic evidence will follow, focusing on psychopathological dynamics in general and the significance of molecular mechanisms in particular.
An increasing pool of convergent genetic evidence from several lines of research suggests that complex gene-environment interactions and epigenetic mechanisms are likely to be involved in the development of manifest clinical illness.
In contrast to the strong impact of de novo variant risk present in a relatively small number of cases, the common genetic variants associated with ASD may each contribute with only small effects, yet together, they may contribute substantially to autism liability, with most of the heritability likely due to common variants [43].
Most likely, multiple types of genetic risk for ASD are associated with a continuum of behavioral and developmental traits across a spectrum, with only the most severe cases at the end of the spectrum resulting in an ASD condition or some other, related neurodevelopmental disorder [13]. Much in line with the model of the complex relationship between biological variation and some major forms of psychopathology espoused by Craddock and Owen [14], Robinson and coauthors [13] suggest a continuum model to be used in future studies of the biology of neurodevelopmental disorders. Their suggestion refers to evidence from genome-wide genetic linkage analysis focusing on variations in social behavior and adaptive functioning relating to data from several large ASD consortia, as well as population-based data.
Increasing evidence suggests that both quantitative and qualitative differences in genetic profiles may be associated with the heterogeneous clinical expression across subetaoups [9],[42]. De novo and common polygenic variation probably confer risk in different ways [13],[42]. Consistent with the hypotheses of the dynamic model, such evidence may indicate that different routes and mechanisms may be involved in the development of the various clinical expressions of the phenomenon of autism and neurodevelopmental disorders including ASD.
How are the relationships among genetic vulnerability, cognitive vulnerability and manifest clinical illness reflective of the present evidence, and how do these fit with the predictions suggested by the dynamic model?
Common genetic variants appear to be associated with normal to high IQ and high-functioning ASD [9],[92],[93], whereas single-gene and de novo mutations are associated with severe intellectual disability and rarer syndromes [93],[94]. In addition to the observations of lower IQ in the presence of a de novo mutation, de novo mutations occur across the entire IQ distribution [94]. Furthermore, evidence indicates that common polygenic risk may more consistently contribute to autism liability across cases with common variant risk associated with ASD not only in individuals with high IQ but also in those with low IQ, sometimes acting together with a de novo mutation [42]. Overall, common variants are associated with better educational and cognitive outcomes in the population.
Focusing on the relationships among cognitive impairment, symptom severity and ASD-associated de novo mutations, or familial inherited variants, Robinson and coauthors [93] analyzed the association between measures of intelligence and of language and behavior, and the rate of de novo loss-of-function (LoF) mutations and family history of mental disease. They identified subetaoups with different probabilities of case severity related to de novo and inherited variants [93]. Their results showed high rates of LoF mutations and low frequencies of family history of psychiatric illness in intellectually impaired individuals, with this group also being more impaired with regard to language and behavior. In contrast, a higher IQ was associated with a family history of psychiatric disease and low rates of de novo LoF, indicating that the inherited risk for mental disorders may be more relevant in ASD etiology for cases of higher functioning. These results are consistent with Sanders et al. [94]. Insofar as intellectual disability and time of early onset are likely to be associated, higher IQ is likely to be associated with later onset, all other factors being equal; the predictions offered by the dynamic model may also be consistent with these results.
Clarke et al. [92] investigated the relationship between common genetic risk for ASD and cognitive function in the general population using polygenic risk profiling to investigate the genetic overlap. Consistent with Robinson [93], the authors found a positive correlation between polygenic risk for ASD and general cognitive ability, a finding replicated in an independent sample. Their result indicates that the relationship between genetic risk for ASD and intelligence may be partly independent of clinical state, suggesting that common genetic variants associated with ASD affect general cognitive ability [83]. Clarke et al. [92] acknowledge that the existence of distinct classes of genetic risk may complicate the relationship between autism and intelligence.
Interestingly, and apparently consistent with the findings by Robinson et al. [93] and Clarke et al. [92], an association between autism risk and common polygenic effects on greater educational attainment suggests that individuals with ASD and intellectual disability have inherited more IQ-increasing alleles compared with their typically developing siblings [42]. This finding, replicated in independent ASD cohorts, was not associated with de novo events. The authors raise the question whether an IQ below 70 in an individual with ASD may differ somehow from an IQ below 70 in someone without and suggest examining the mechanisms through which genetic risk is conferred by distinguishing between how and when risks are deleterious or beneficial [42].
From a clinical perspective, the results suggesting that the relationship between genetic risk for ASD and intelligence may be partly independent of clinical state and that individuals with ASD compared with their typically developing siblings respond differently may not be surprising. The results of a cognitive test depend on motivation and mutual understanding. It is a common observation that test behavior may differ in individuals with ASD compared to the behavior of typically developed individuals. How do such behavioral differences affect a cognitive test result? If test performance understates real cognitive potential due to a lack of motivation or a lack of ability to understand the purpose of a test, then the test result may not be representative of the true cognitive potential or comparable to standard expectations. This may explain the observed differences in educational attainment in ASD compared with typical development, all other factors being equal. Another possible explanation emerges from the clinical observation that an uneven cognitive profile possibly contributes to a different cognitive style, with an average IQ not being representative of the true cognitive potential.
Previously, the present author has suggested that a cognitive vulnerability may influence the experience of reality in ASD [16] and that a tendency to experience the imaginary world as being real, reflected in a rigidity of thinking, may reflect the core link between the phenomena of autism and psychosis and the clinical diagnostic constructs ASD and schizophrenia [16],[17]. These hypotheses, included in the dynamic model, are central for the vulnerability model that illustrates how a basic genetic and neurobiological susceptibility may translate into a disorder of reasoning (see Figures 5a, 5b and 5c). From a cognitive perspective, cognitive impairments comparable in nature may operate at different levels of complexity, resulting in the reduced cognitive flexibilities frequently observed in both ASD and schizophrenia [17]. The different levels of complexity of thought and reasoning are comparable to those distinguished by Piaget, which he suggested relate to different cognitive stages of development [19]. As illustrated by the vulnerability model, it is possible to identify different levels of severity of disordered reasoning that are assumed to be associated with neurodevelopmental pathological load (see Figure 5a). Although there may be an overlap between severity of disordered reasoning and severity of illness, according to the dynamic model, this may not necessarily be the case. The risk may depend on both environmental risk and resilience factors, as well as on genetic influences related to the individual. These may change during development. In addition to the contribution from genetically related causes, and dependent on the neurodevelopmental pathological load, more enduring or recurrent clinical symptoms may also depend on the presence of enduring or recurrent stress (see Figures 4a, 4b, 6 and tables 1 and 2).
From a neurobiological perspective, the particular focus of the dynamic model on the dynamics of interactions between processes taking place at and across several different translational levels, including the genetic, biological and cognitive levels, may be consistent with the model of biological variation and psychopathology proposed by Craddock and Owen [14]. It appears reasonable to assume that cognitively vulnerable individuals may be at greater risk of influence by environmental risk factors compared with individuals having normal cognitive abilities. Increased stress reactivity resulting from epigenetic processes activated by early life events may further contribute to basic neurobiological susceptibility, thereby increasing the risk for manifest clinical illness. Preclinical evidence indicates that maternal stress may result in increased stress responsiveness and cognitive deficits in offspring [51]. Such evidence may be consistent with the suggestions of the dynamic model that maternal stress may affect a fetus and may alter sensitivity of the hypothalamic-pituitary-adrenal (HPA)-stress axis in offspring, in addition to possibly affecting neurodevelopment and potentially resulting in altered behavioral stress reactivity and cognitive deficits.
How do environmental risk and resilience factors modify risk across development in genetically susceptible children and adolescents? Children may be differentially susceptible to environmental influences depending on gene-environment interactions. Studies suggest that common gene polymorphisms may moderate the influence of environmental risk factors on psychopathological outcomes [95],[96]. Importantly, epigenetic processes may not only activate susceptible genes but also activate protective genes, thereby affecting risk or resilience [63],[91]. Such evidence may help elucidate why only some but not all at-risk individuals develop manifest clinical illness, e.g., genetically susceptible individuals.
How do the effects of stress initiated by epigenetic processes that occur later in life balance with the significance of the molecular and brain morphological changes affecting neurodevelopment that are established early in life? Information about this balance has potentially important therapeutic consequences. In particular, for those cases of high-functioning ASD where genetic causes are primarily associated with common genetic variants having a late time of onset, it may be relevant to consider whether a focus on prevention and treatment should target stress-releasing factors, rather than factors related to neurodevelopment.
According to the dynamic model, the time of clinical onset depends on genetic influences, neurodevelopmental pathological load, environmental risk and resilience factors, and compensating mechanisms (see Figures 4a, 4b, 6 and Tables 1 and 2). Consistent with Latham et al. [25], endogenous epigenetic processes and exogenous epigenetic processes modify gene expression and mediate the complex gene-environment interactions across time. The dynamic model suggests that a large and severe neurodevelopmental pathological load may be visible from the beginning of life. Normal events related to development may cause enduring stress that contributes to the clinical expression of manifest clinical illness. In contrast to this example of early onset, the dynamic model predicts that the neurodevelopmental pathological load in psychopathological courses of ASD with a late time of onset may be smaller and less severe, consistent with the observation of either no or only a few clinical symptoms early in life. In this case, the dynamic model predicts that clinical expressions only occur in the presence of recurrent or enduring stress occurring later across the developmental course, either those resulting from predictable challenges related to normal development or those related to adverse events or trauma. The dynamic model may be in line with the concept of “allostatic load” insofar as this concept focuses on the significance of the accumulated effects of stressors throughout life and acknowledge the importance of genetic differences [61]. A strength of this concept is the emphasis on how behavioral and physiological consequences of recurrent or chronic stress may affect lifestyle, with lifestyle factors by themselves contributing to the course.
In light of present genetic and epigenetic evidence, it appears that the effects of stress may be at least twofold. First, stress mediated via epigenetic effects may modulate processes occurring at the molecular level, e.g., the effects on neurodevelopment initiated by the modulation of expression of susceptible genes or the effects resulting from epigenetic programming of future stress sensitivity. Second, stress mediated via epigenetic effects may affect behavior through direct effects on tissues via hormone release or via regulation of endocrine function, e.g., direct effects of cortisol release on the brain that may affect neurocognitive function or result in clinical symptoms such as anxiety, compulsivity or depressive symptoms observed clinically.
Already emphasized above is the importance of epigenetic mechanisms that affect the neurodevelopment and stress sensitivity involved in the development of ASD. The convergent evidence showing disordered HPA-axis regulation of stress with increased cortisol variability and higher levels of salivary cortisol and flatter slopes across time observed in both high- and low-functioning autism [68]–[74] may indicate that stress is involved not only in the development but also in the maintenance of clinical symptoms. Such evidence may be consistent with the predictions made by the dynamic model and with the suggestions offered by stress researchers that characteristic alterations in circadian rhythms observed in ASD may result from heightened sensitivity to daily changes and unpredictable events and to cumulative effects of daily stress [69],[70],[72]. The observation of higher levels of psychological measures of stress and affected sensory functioning related to lower levels of cortisol may indicate chronic stress in ASD, with lower levels of cortisol observed in conditions of chronic stress and social situations characterized by unstable social relationships [69],[71].
A recent study focusing on the relationships among cortisol, serotonin, oxytocin and repetitive behaviors found that individuals with ASD had higher levels of cortisol areas under the curve and of serotonin, as well as lower levels of oxytocin, compared with a group of typically developing controls [97]. The levels of cortisol, serotonin and oxytocin were significantly associated with total scores on the Repetitive Behavior Scales-Revised in individuals with ASD, and the cortisol levels were positively associated with the stereotyped and restricted behavior subscale scores. The authors concluded that cortisol, serotonin and oxytocin are associated with repetitive behaviors in ASD and are possibly involved in the occurrence of these symptoms [97]. The clinical description of the ASD group showed that all individuals had a comorbid intellectual disability. Stereotyped behavior is a common clinical symptom in intellectual disability. The authors, however, did not discuss to what extent factors related to comorbid intellectual disability may have contributed to their results. Another study exploring the effect of a prior diagnosis of OCD in children and their parents on susceptibility to ASD, or the other way around, found that ASD with a comorbid diagnosis of mental disability did not increase the risk of OCD [98]. The authors aimed to determine the patterns of comorbidity, longitudinal risk, and shared familial risk between OCDe and ASD in a large population-based, nationwide prospective cohort study based on a younger cohort of children. Records of the exposures were independent of outcome. The results showed that the risk for a comorbid OCD in individuals with an ASD and the agammaegation of ASD in offspring of parents with OCD were increased [98]. Individuals first diagnosed with an ASD had a 2-fold higher risk of a later OCD, whereas individuals first diagnosed with an OCD had an almost 4-fold higher risk of a diagnosis within the autism spectrum later in life. Interestingly, the observed associations were stronger for less severe types of ASD. In light of the observed high comorbidity, longitudinal risk, and shared familial risk between the two disorders, the authors concluded that their results indicate partially shared etiological mechanisms between OCD and ASD.
To what extent are repetitive behaviors, e.g., stereotyped and restricted behaviors, or obsessive and compulsive behaviors associated with stress and to what extent are they associated with genetic influences? How does the contribution to repetitive behaviors from stress-related respectively genetically influenced factors vary with IQ and over time?
According to Valla and Belmonte [99], ritualistic behavior may result from compensatory mechanisms for interactions between cognitive inflexibility and sensory abnormalities related to development. Their developmental dynamic interactionist model assumes that initially independent social and nonsocial autistic traits may covary because of dynamic trait interactions over the course of development [99]. The emphasis of the dynamic model on the significance of impaired cognitive flexibility and of possible compensatory mechanisms involved in the development of neurodevelopmental disorders and the phenomenon of autism may be in line with Valla and Belmonte's model [99]. Their model could also be consistent with the two- or three hit model proposed by Bale [51] and to the suggestion that disordered HPA-axis regulation of stress in ASD may reflect abnormalities in subcortical structures engaged in stress responsivity to novel stimuli, as well as to those structures implicated in memory formation and recall related to individual experiences [70];[72].
According to Livingston and Happé [81], compensatory resources may be limited under stress, anxiety or mental fatigue. Under such circumstances, in line with Livingston and Háppe [81], the dynamic model predicts that transient stress may cause transient clinical symptoms (see Figure 4b). With more enduring stress, the risk for more enduring clinical symptoms and development of manifest clinical illness arises. The dynamic model suggests that enduring stress affects the ability to compensate, thereby contributing to the development and maintenance of more enduring clinical symptoms. It may be reasonable to assume that stress mediated via epigenetic effects can affect neurocognitive function, e.g., via direct effects of cortisol release on the brain, thereby affecting the neurocognitive mechanisms potentially involved in compensation. This would be consistent with evidence from animal models of maternal stress demonstrating that maternal stress may alter the development and sensitivity of an offspring's HPA axis and behavioral stress reactivity, potentially resulting in cognitive deficits [51], and with results from animal and human studies indicating functional and structural changes in brain areas involved in cognitive function such as the hippocampus and prefrontal areas [61],[62].
Basic assumptions of the dynamic model are the suggestions that secondary stress-related processes may affect time-demanding cognitive processes involved in adaptive coping and that adaptive or compensatory mechanisms should be distinguished from more basic processes involved in the development of manifest clinical illness [16]. According to Belmonte et al. [29], and in line with the assumptions guiding the dynamic model, secondary compensatory processes may mask primary abnormalities, thereby increasing the risk of overlooking cognitive and perceptual features closer to the core dysfunction than the diagnostic features. In line with Szatmari [32] and Belmonte et al. [29], the dynamic model assumes that more basic phenomena relating to brain structure and function and their deviations in relation to expected normal variation across development may contribute to the development of manifest clinical illness [16]. Consistent with the prediction by Belmonte et al. [29], the model predicts that subtler cognitive deficits may not be recognized until after manifest illness appears with overt changes in behavior, e.g., stress-related symptoms, or social withdrawal.
Evidence indicates that individuals with ASD show enhanced and sustained social stress increasing with age [70],[73],[74]. Such evidence may be consistent with a previous suggestion included in the dynamic model that a discrepancy between the cognitive ability of an individual and social and cognitive challenges may give rise to stress, potentially worsening already present cognitive impairments while further contributing to symptom appearance and increasing the risk of developing manifest clinical illness [16],[17].
The impact of stress in the dynamics resulting in the development of neurodevelopmental disorders including ASD, and how events related to normal development may trigger stress as suggested by the dynamic model, may be underestimated. In addition to the neurocognitive mechanisms (e.g., higher IQ, intact executive functions, or neural mechanisms involving recruitment of alternative networks) suggested by Livingston and Happé [81] to potentially be involved in compensation, Lai et al. [100] have suggested that some individuals may use learned strategies to hide or camouflage social and communicative difficulties. According to these authors, such coping strategies may require considerable cognitive effort and may lead to increased stress, anxiety and depression [100].
Recognition of the importance of including mechanisms for compensation when trying to understand the relationship between behavioral presentations and an underlying neurobiological susceptibility in neurodevelopmental disorders cannot be underestimated. As stated by Livingston and Happé [81], a transdiagnostic compensation framework may have important implications for clinical practice and research. Compensation may help explain an observed mismatch between behavior and cognition in high-functioning subetaoups. The framework of compensation may help guide research in the effort of investigating mechanisms involved in changes in symptom severity across development and unravelling the mechanisms involved in the development of neurodevelopmental disorders across time.
To what extent is ASD the combined result of deviant processes and normal processes? If deviant behavior results from the combined action of normal processes and deviant conditions, what does that imply for our understanding of autism and associated psychopathological processes? How does such knowledge affect strategies for treatment and prevention?
Natural or synthetic physical and chemical compounds, including toxicants, medication and nutrition, may affect brain maturation, endocrine function and signaling pathways, with epigenetic mechanisms possibly being involved [25],[28],[47],[50]. Physical and chemical environmental risk factors are included in the dynamic model, although the mechanisms involved and the extent to which they contribute to the development of ASD still remain unknown. Associations between ASD and infection, despite having been observed, may not be specific to an infection or to ASD [101], with recent findings suggesting a genetic risk for infections and a genetic correlation with mental disorders, as well as a high degree of comorbidity between the two disorders [102]. The suggestion that chemical environmental risk factors may affect modulation of the expression of genes coding for neuropeptides and result in dysregulation in brain neuropeptide systems underlying social dysfunctions in ASD [28] is interesting and warrant further investigation. The hypothesis assuming that oxidative stress is initiated by environmental factors in genetically vulnerable individuals [54] appears to be consistent with the concept of “allostatic load” [61], and may be in line with the suggestion that oxidative stress observed in ASD related to susceptible gene variants may be due to multiple polymorphisms and environmental factors interacting and affecting metabolic pathways, thereby inducing a chronic metabolic imbalance [53]. In light of the of the many physiological systems, e.g., neural, cardiovascular, autonomic, immune and metabolic systems that are affected by the multiple interacting mediators activated in response to stress [61], concurrent findings of cardiovascular, autonomic and immune dysfunction as well as metabolic imbalance and affected brain function may not be surprising in clinical conditions involving recurrent or enduring stress. To what extent this may explain concurrent findings of autonomic and immune dysfunction in neurodevelopmental disorders including ASD remains an open question. Such a view is reflected in the dynamic model and also expressed in the model by Sarovic [86] with this model also suggesting that immune function and autonomic regulation have an independent role modulating the effects of environmental risk factors, or inducing insults of their own, potentially increasing the effects.
In general, evidence indicating that common gene polymorphisms may moderate the influence of environmental risk factors on psychopathological outcome and that children are differentially susceptible to environmental risk factors, including stressful life events [95],[96], may be important to and of potential use in the development of future strategies for treatment and prevention.
Social environmental risk factors include stressful life events and trauma. Convergent evidence indicates that epigenetic processes may be involved in the transmission of maternal and paternal adversity to offspring before conception [65] and the transmission of maternal stress to the fetus [51], with observed effects on stress sensitivity and potential effects on neurodevelopment. Furthermore, HPA-axis dysregulation related to social stressors is observed in children, adolescents and adults with ASD [69],[72],[89]. Since epigenetic processes may be reversible [63], knowledge about the stress-related processes and gene-environment interactions involved in the development and maintenance of clinical symptoms in autism may have important implications for future choices about relevant approaches to treatment and prevention. In this light, the findings by Dinkler et al. [60] indicating that the effects of childhood maltreatment, and the associated risk for mental problems, including neurodevelopmental disorders, are mainly due to possible common genetic factors offer an important contribution to the field's knowledge. Although maltreated children appear to be at higher risk of having multiple neurodevelopmental problems, the findings were not consistent with the assumption that maltreatment causes these problems [60]. The authors concluded that maltreated children may carry a double burden of both having extremely adverse experiences in childhood as well as a high load of neurodevelopmental problems, with their data suggesting that the association between maltreatment and neurodevelopmental disorders may have a common genetic origin [60]. This suggestion may be consistent with the conclusions from a sound study on the origins of cognitive deficits in victimized children including data from two longitudinal population-based birth cohorts [103]. The study included scores for twins discordant for victimization and found that observed cognitive deficits in victimized individuals were largely explained by cognitive deficits already present prior to victimization and by confounding genetic and environmental risk factors indicating that the association between childhood maltreatment and later cognition is largely non-causal.
According to the dynamic model, social expectations may be important social environmental stressors if an individual is unable to fulfill them due to unrecognized cognitive impairments or an impaired ability to readjust to new expectations. In general, the significance of cognitive impairments and their involvement in psychodynamics may be underestimated. In particular, cognitive dysfunctions that go unnoticed may contribute to more enduring stress triggered by cognitive and social challenges, either because of compensation or the use of learned strategies or because the cognitive impairments are subtle or overshadowed by other symptoms. As illustrated in the vulnerability model (Figure 5a), the cognitive ability of an individual set limits for experience at the interface between the brain and mind. Translation of sensory inputs into interpretations take place in the brain. Individual experience results from the translation of sensory information via basic information processing and psychological processes. As illustrated in the vulnerability model, a disorder of reasoning may affect the experienced interpretations of reality. In parallel, at the interface between an individual and their environment, social interaction takes place, consisting of the interaction between two or more individuals influenced by their experiences. Mechanisms that govern social dynamics set the limits for social interaction at the interface between the mind and society. Insofar as the cognitive ability of an individual sets the limits for experience, individual experience may likewise limit social interaction.
The dynamic model and the vulnerability model emphasize the importance of social aspects of cognitive impairment in the development of clinical symptoms (see Figures 4a, 4b, 5a, 6 and Tables 1 and 2). The dynamic model suggests (1) that genetic influences affect cognition, thereby affecting the ability to engage in social interaction; and (2) that stress resulting from cognitive and social challenges across development may affect the brain, further contributing to cognitive impairments and/or disrupting compensatory strategies and thereby worsening social capacity. The focus of the dynamic model on the dynamics of interaction between cognitive processes and their relation to the psychological and social level may be consistent with work by Sameroff and Mackenzie [88].
Compensation by the social environment, also called “environmental scaffolding” [81], may support cognitively vulnerable individuals by reducing stress and reducing the visible effects of cognitive impairments on behavior. According to the dynamic model, scaffolding may reduce the risk of manifest clinical illness, thereby postponing the time of clinical onset.
Parent-child interaction, the attachment dimension, may be only one of several social interaction contexts that may affect an individual across a lifetime. In line with Sameroff and Mackenzie [88] and Plomin [34], the dynamic model suggests analyzing the potential psychopathological impact of changing social interaction contexts across time, not only early in life but also across development into adulthood.
To understand the psychodynamics involved in the development of neurodevelopmental disorders and the phenomenon of autism, in light of present evidence, it appears important to distinguish between genetic influences, epigenetic processes, environmental exposures and how gene-environment interactions across time affect gene expression. Furthermore, information about the kind of environmental exposure and the timing of said exposure, whether it occurs prior to conception, during the fetal state, after birth, and earlier or later across the developmental course, are important pieces of information needed to be able to disentangle the complex processes involved in the development and maintenance of the psychopathological expressions of autism and neurodevelopmental disorders.
Should the epigenetic changes observed by Shulha et al. [79] as developmentally regulated peaks across a lifetime be replicated in future studies, it will be interesting to see whether such changes are associated with social and cognitive adjustment related to normal challenges occurring across a typical developmental course, as predicted by the dynamic model. Whether the findings by Shulha et al. [79] relate to normal events across development, e.g., social and cognitive challenges across childhood, adolescence and adulthood, remains to be seen. Furthermore, the issue of whether neurodevelopmental disorders are associated with endogenous epigenetic regulation of susceptible genes, deviances in epigenetic regulation, and/or exogenous epigenetic processes related to normal or deviant events across development remains an open question. According to the dynamic model, several routes are possible, and several mechanisms may be involved across time.
With reference to trauma research, Weems et al. [83] suggest investigating the impact of stress exposure on age-related changes in structural or functional connectivity and linking information about effects on connectivity to the symptom-expression network. In addition, these authors suggest clarifying the role of cumulative stress and the timing of trauma on connectivity [83]. The dynamic model may be consistent with suggestions by Weems et al. [83] to apply a developmental approach in order to be able to understand the neurobiological response to stress. They argue that traumatic stress may affect the typical developmental changes of the brain.
The bioecological model of risk and resilience to traumatic stress by Weems et al. [82] focuses on the dynamics involved in the development of trauma-associated disorders, and PTSD in particular. The basic components of the Weems et al. [82] model—namely, quality and duration of environmental exposure (trauma), genetic susceptibility and quality of the social environment—are also components included in the dynamic model. To what extent does traumatic stress differ from stress associated with neurodevelopmental disorders including ASD? Is it relevant to distinguish between different environmental exposures associated with stress, or are the epigenetic mechanisms identical independent of the kind of exposure, e.g., trauma or social stress? In addition to having a secondary focus on the dynamics involved in the development of disorders associated with trauma, the dynamic model primarily focuses on the dynamics involved in psychopathology associated with neurodevelopmental disorders, including ASD. In contrast to the model by Weems et al. [82], the dynamic model suggests that not only trauma or adverse events, including maltreatment but also quite normal events across development may trigger the development of clinical symptoms in cognitively susceptible individuals.
The dynamic model may be compatible with Menon [31], who suggests that network analysis results may contribute to evidence about changes in the overall organization of the brain that are associated with neuropsychiatric disorders. Consistent with Belmonte et al. [29],[80], Menon [31] distinguishes between affected subnetworks and networks compensating for dysfunction. The triple neurocognitive network model on psychopathology focuses on the dynamic interaction of multiple brain regions across development, assuming that such an approach may help relate findings into a common framework for understanding dysfunction in core neurocognitive networks across multiple neurodevelopmental disorders, including ASD [31]. Changes in one core network may affect the other networks, resulting in clinical manifestations that go beyond the primary deficit.
The impact of social environment on the development and maintenance of clinical symptoms after the early years of life may be underestimated. It is reasonable to assume that the quality of social relations not only is important in early childhood but also may be important later in life, potentially contributing to epigenetic modification of susceptible genes. This assumption of the dynamic model may be in line with evidence indicating that, in addition to preprogrammed epigenetic changes related to developmental and aging processes, epigenetic status also may change across a lifetime depending on environmental influences [49]. Furthermore, the assumption is consistent with the theoretical suggestion that while the influence of family decreases across time, life events outside the family become more important in shaping experience [34],[88].
From a theoretical point of view, the transactional model emphasizes the significance of the active interplay between an individual and their context in shaping experience [88]. This model views developmental outcomes as the results of individual differences in the regulation of experience. As such, they represent relational constructs, the products of a combination of an individual and their experience in a given context. Individuals engage in complex dynamic reciprocal interchanges and modify social and biological experiences.
According to Plomin [34], and consistent with Sameroff and Mackenzie [88], the contributions of nonshared life events among siblings increase across a lifetime, shaping the experience of individuals and potentially affecting outcomes. Nonshared environmental factors, however, can only account for a small degree of the effects of experience on outcomes. Individuals actively contribute to their experiences [34]. They affect the environment, implying that environmental measures depend on the individual making measures of genetically influenced experiences. This suggestion could be in line with the assumption by Lee and Avramopoulos [50] that the phenotype may affect the behaviors of an individual, thereby influencing how an individual modifies the environment. Furthermore, the suggestion may be consistent with the findings by Dinkler et al. [60], who suggest that the association between maltreatment and neurodevelopmental disorders may have a common genetic origin and the evidence suggesting that common gene polymorphisms can modify the effects of environmental risk factors [95],[96]. The suggestion by Plomin [34] that measures of experience are genetically influenced may also be consistent with the dynamics illustrated by the vulnerability model (Figure 5a). As illustrated by this model, the cognitive ability of an individual sets the limits for experience at the interface between the brain and mind, and social interaction takes place at the interface between an individual and their environment, with individual experience setting limits for social interaction.
Insofar as experience is genetically influenced [34], to what extent is the genetic influence stable and to what extent is it malleable?
As previously mentioned, the results from studies on animal models indicate that early experience related to mother-infant interaction affects behavior and physiology, e.g., stress sensitivity, in offspring mediated by epigenetic processes [63]. Despite the stable nature of the resulting DNA methylation patterns observed in selected brain regions, e.g., the hippocampus, these changes are reversible. Such findings may have important therapeutic consequences, indicating that environmental programming potentially is reversible by pharmacological treatment or environmental intervention. Possibly related, although focusing on epigenetic alterations associated with preconception transmission, is the suggestion by Yehuda et al. [91] that experiences and learning across time may affect epigenetic processes in offspring and may vary across a lifespan depending on environmental influences. Insofar as epigenetic processes are malleable and involved in the development of manifest clinical illness, the opportunity for environmental intervention becomes a relevant option to consider.
Results from studies in adults with ASD show that perceived stress and stressful events are significantly associated with social disability and that social stress is associated with social but not global functioning [73],[74]. Consistent with this finding, a recent meta-regression analysis found statistically significant associations of moderate effect size between measures of quality of life (QoL) and social functioning in adults with ASD, with only small and insignificant associations observed between QoL and age, IQ, and autism severity [104]. Social functioning explained only approximately 10% of the variation in QoL in adults with autism. The authors mention other potentially significant QoL correlates reported in prior research, such as perceived stress, self-esteem, depression, anxiety, and psychological empowerment. In light of their findings, they suggest either supporting social functioning in adults with ASD as a means to increase QoL or educating the social environment regarding the importance of understanding and meeting adults with ASD, thereby increasing their QoL regardless of their level of social functioning [104], a suggestion in line with Bishop-Fitzpatrick et al. [89].
Associated psychiatric disorders may contribute to impairment. An epidemiological study exploring the rates and types of psychiatric comorbidity associated with ASD, as well as the associations with variables identified as risk factors for child psychiatric disorders, found that 70% of their participants had at least one comorbid disorder and approximately 40% had two or more [10]. These results are consistent with recent solid evidence showing that comorbidity within mental disorders is common, with risk persisting over time [105]. The most common comorbid diagnoses identified by Simonoff and coauthors [10] were social anxiety disorder, attention-deficit/hyperactivity disorder and oppositional defiant disorder. While epilepsy was associated with any main disorder, autism severity was not a significant predictor of other disorders, and neither IQ nor the Vineland Adaptive Behavior Scale score was associated with any other psychiatric disorder category [10]. The authors concluded that delineation of psychiatric comorbidity could identify targets for specific intervention that have the potential to reduce overall impairment and improve QoL [10], a suggestion in line with Gillberg [8].
The results by several researchers [92]–[94] indicating that the relationship between genetic risk for ASD and intelligence may be partly independent on clinical state may be consistent with both Simonoff et al. [10] and the findings by Kim and Bottema-Beutel [104] that IQ is independent of clinical state in ASD. These observations may be in line with the distinction between a basic neurobiological susceptibility and manifest clinical illness reflected in the dynamic model and the prediction suggested by the vulnerability model that severity of illness may differ from the severity of a disorder of reasoning.
A potential challenge for relevant treatment—which may be particularly relevant for clinical cases complicated with social adversities—may be that the complexity of clinical problems is so overwhelming that the social environment, including parents and clinicians, do not recognize neurodevelopmental problems [58],[60]. The results by Dinkler et al. [60] showing that maltreated children display more neurodevelopmental disorders than children who have not experienced childhood maltreatment may indicate that individuals presenting with trauma-related disorders may have undiagnosed neurodevelopmental disorders. Such evidence may point to the relevance of applying a multidisciplinary clinical approach, in line with the suggestions by Simonoff [10] and Gillberg [8] and possibly in line with the implications of the dynamic model.
To understand the specific psychodynamics involved in the development of neurodevelopmental disorders and the phenomenon of autism, for each individual course of illness, the dynamic model suggests that it may be possible—at least in theory—to disentangle the contribution from susceptible gene variants (e.g., rare mutations, CNVs, common variants), intrinsic and extrinsic epigenetic processes affecting gene expression and modifying stress sensitivity across development and to identify the environmental factors affecting extrinsic epigenetic processes. Although in theory, overall contributing mechanisms and different routes may be predicted, to be able to predict an individual course and a specific route of disease across time would demand having a crystal ball and being able to look into the future. On the other hand, for each individual case, while looking backwards in time, it is suggested that the dynamic model can be applied as a tool guiding the task of disentangling how the different contributing mechanisms across time may have contributed to clinical symptoms of possible relevance for treatment. From a universal perspective, the dynamic model offers an explanation for why different individuals have different onsets of disease. The model visualizes overall universal psychodynamics including different possible contributory mechanisms. Even though the model suggests that there are several routes to disease, some overall generalizable patterns may be predicted. According to the dynamic model, environmental risk and resilience factors and common gene polymorphisms may modify risk across development in genetically susceptible individuals such that they are differentially susceptible to environmental influences depending on gene-environment interactions. The model emphasizes the importance of seemingly natural and innocuous events such as normal challenges related to development and the importance of social risk factors. According to the model, the relative contribution from physical and chemical environmental factors may decrease across time, whereas the relative contribution from social environmental risk factors may increase.
To conceptualize a phenotypic outcome as truly transgenerational, according to Bale [51], an epigenetic mark must be present in the germ cell. Evidence from preclinical research indicates that epigenetic marks can be erased and re-established in the absence of re-exposure to the original environmental stimuli [51]. This ability determines whether future generations will be impacted [51]. Today, growing evidence from animal models, in vivo experiments and epidemiological studies indicates that transgenerational passage of parental stress experiences may take place and involves changes in germ cell epigenetic marks. How and when epigenetic marks can be programmed during germ cell development is still not clear [51]. Importantly, previous preclinical evidence indicates that epigenetic processes may be reversible [63]. The molecular mechanisms underlying the relationship between environment and neurodevelopmental disorders remain unclear [47].
According to the dynamic model, cognitive impairments comparable in nature may operate at different levels of complexity, thereby influencing the experience of reality, and such impairments may be reflected in a reduced cognitive flexibility representing a core link between ASD and schizophrenia [16],[17]. The vulnerability model illustrates how a basic genetic and neurobiological susceptibility may translate into a disorder of reasoning, with different levels of severity of disordered reasoning assumed to be associated with neurodevelopmental pathological load (Figure 5a). Although there may be an overlap between severity of disordered reasoning and severity of illness, according to the dynamic model, this may not necessarily be the case. The risk may depend both on environmental risk and resilience factors and on genetic influences related to an individual. These may change across development. In addition to contributions from genetically related causes and the neurodevelopmental pathological load, more enduring or recurrent clinical symptoms may depend on the presence of enduring or recurrent stress.
From a scientific point of view, the effort of unravelling the dynamics involved in the development of autism spectrum and related disorders will require collaboration between different scientific disciplines and an agreement on the definitions of concepts as well as a clear distinction between clinical phenomena and clinical diagnoses [16]. Although clinical experience should inform the development of theory, as suggested by Cuthbert and Insel [106], it may be of paramount importance to challenge the inherent assumptions of present categorical diagnostic classifications. The dynamic model presumes an open-minded dimensional and transdiagnostic approach that is symptom-focused, albeit independent of the inherent assumptions implied in the present symptom-based categorical diagnostic classifications of mental illness provided by the Diagnostic and Statistical Manual of Mental Disorders, Fifth Edition (DSM-5) and the Tenth Revision of the International Classification of Diseases and Related Health Problems (ICD-10) [107],[108]. As such, the dynamic model may be in line with the hypothesis about dubious specificity by Caspi et al. [87], and with the ESSENCE approach suggested by Gillberg [8], although extended beyond preschool age into adulthood. Similar to the concept of ESSENCE, the dynamic model assumes that neurodevelopmental disorders express themselves differently at different timepoints depending on environmental demands, and as such it does not make sense to distinguish between specific disorders. Furthermore, the dynamic model may be consistent with the model of the complex relationship between biological variation and some major forms of psychopathology proposed by Craddock and Owen [14], although the dynamic model presumes that the gradient of affective pathology may be equally contributing to psychopathology along the whole spectrum of mental disorders. The relatively large contribution of a neurodevelopmental pathological gradient to clinical symptoms in ASD and other neurodevelopmental disorders may blur the contribution from the gradient of affective pathology. Such a view may be consistent with the dimensional severity hypothesis suggested by Caspi et al. [87], and their suggestion that thought disorder symptoms may represent core symptoms of general psychopathology. Insofar as their thought disorder dimension may reflect a basic neurobiological susceptibility associated with genetic liability this may be comparable to the suggestion by the dynamic model that a disorder of reasoning may reflect the basic neurobiological susceptibility at the core of neurodevelopmental disorders.
According to the dynamic model, affective symptoms, including anxiety, obsessive or compulsive symptoms, and depressive symptoms may result from the epigenetic dynamics of stress involved in the development of manifest clinical illness with symptoms varying across time depending on environmental requirements. This view may be consistent with the developmental extension of the dimensional hypothesis by Caspi et al. [87] suggesting a developmental progression of severity. In addition to the thought disorder dimension, these authors identified two other dimensions of psychopathology, an externalizing liability to antisocial and substance-abuse disorders, and an internalizing liability to depression and anxiety also highly correlated with the p factor suggesting that these factors also to some extent may reflect general psychopathology [87]. Whereas no sex differences were observed in the thought disorder dimension, in contrast this was observed for the internalizing and externalizing factors. Males were more likely to exhibit externalizing and females more likely to exhibit internalizing symptom related behavior indicating that these two dimensions may represent gendered personality styles. In light of the suggestions by the dynamic model it is tempting to speculate whether these two dimensions reflect stress associated gender related symptom styles.As proposed by Ouss-Ryngaert and Golse [84], it will be necessary to bridge the gap between the different and sometimes conflicting approaches that hitherto have guided our understanding and clinical practice. They emphasize the importance of theoretical models to guide research and become aware of the assumptions guiding the models [84]. In line with previous models, the present dynamic model attempts to integrate present evidence in order to predict clinical illness. What the present model may add to previous models is an individualized and dynamic approach embedded in a developmental context integrating several levels of influence (genetic, neurocognitive, psychological and social) involved in the dynamic interaction between an individual and their physical, chemical and social environment across time. In addition, the model emphasizes that even quite normal events related to typical development, e.g., starting school, may increase the risk for enduring stress in cognitively vulnerable individuals, further increasing their risk of developing manifest clinical illness.
The association between a more basic disorder of reasoning and severity of illness may not be simple. Compensation may blur the presence of an underlying neurobiological susceptibility. Not only ASD but also other neurodevelopmental disorders, OCD, anxiety disorders, and depression may all represent transdiagnostic, dimensional phenomena marked by heterogeneity and comorbidity commonly observed across time [10],[98],[105].
The suggested model may assist in the interpretation of clinical data and may help predict time of clinical appearance and clinical course of illness. The model suggests that basic impairments in reasoning resulting from characteristic cognitive impairments may be common for the phenomena of autism and psychosis, with both phenomena relating to the experience of reality; autism to the social cognitive and behavioral aspects; psychosis to the perceptual aspects and how these may be reflected in thought and behavior [16],[17].
Insofar as epigenetic effects are reversible, it may be important to investigate how general or specific the epigenetic mechanisms involved in the psychopathological processes of neurodevelopmental disorders and the phenomenon of autism are. Such insight may have consequences for the prospect of inventing epigenetic tools for the treatment of ASD and related disorders compared to the alternative of removing environmental causes.
Despite the very simple nature of the suggested dynamic model, it may serve as an example of a possible way to create a common language to grasp and communicate the complexity of the mechanisms and the causes involved in the development of neurodevelopmental disorders and to help understand their nature and predict their time of onset.
There are several limitations of the present theoretical contribution. In its present form, the dynamic model appears to be a sketch rather than a comprehensive model. The model is clinically informed and does not in its present form illustrate the more detailed interactions taking place at the molecular level or the interactions between different regions of the brain that are assumed to be important. Neither does the present model illustrate the complex social and psychological dynamics also assumed to be important. The hypotheses guiding the model refer to scientific evidence from different fields, with the present paper focusing in particular on genetic and epigenetic mechanisms and how they may relate to other translational levels involved in psychodynamics, including basic information processing, cognitive and psychological processes, and social dynamics. Since the content of the paper relates to selected evidence, rather than to an extensive review of the literature, potentially relevant and important evidence is not included.
An important implication of the dynamic model is the need to integrate evidence from different fields of research. Researchers from molecular science, neuroscience, clinical science and social science should collaborate on the development of a comprehensive theoretical model. The present dynamic model may contribute as a skeleton for such an endeavor and suggests one possible direction to follow. In light of present genetic and epigenetic evidence, a future challenge appears to be to design studies that can distinguish between the different contributions from genetic and epigenetic processes across development and between normal and deviant processes involved in the psychopathological processes across time. The dynamic model represents a framework that may be consistent with the suggestion by Simonoff [7] that future causal research in autism needs to focus on both genetic and environmental factors, as well as to identify models of gene–environment interplay.
Recent evidence challenges the hitherto prevalent categorical diagnostic approach to research on mental illness [87],[105],[106],[109]. To understand the complex interactions between the levels of biopsychosocial functioning involved in human regulation, according to Sameroff and Mackenzie [88], there is a need for longitudinal study designs able to assess these reciprocal processes. It is important to design studies in a way where it is possible to distinguish contributions from distinct psychopathological processes involved in pathogenesis across time. Several authors mention the need to construct and use research designs that can distinguish between genetic and environmental influences over time [34],[60],[110], and the need to collaborate across disciplines [14],[84],[111]. Lee and Avramopoulos [50] point to an important challenge of epigenetic studies, the dependence on tissue specificity and developmental processes wherein epigenetic information is dynamic and related to context in time and space. For neuropsychiatric disorders, this problem becomes particularly relevant given the difficulties faced in accessing the human brain. The use of animal models in studies of several environmental factors are relevant insofar as similar physiological processes and signal pathways exist among mammals [50]. According to Tran and Miake [47], in order to clarify etiological pathways, it is important for future studies to focus on epigenetic alterations of specific genes related to neurodevelopmental disorders and to perform such studies in humans, because the underlying biologies of animals and humans potentially differ with regard to epigenetic alterations after environmental exposure.
As a scientific tool, the suggested dynamic model will have to prove its value. To do so, the hypotheses guiding the model need to be operationalized and tested in future research. This task is a next step, outside the scope of the present paper. Like the neurodevelopmental model by Sarovic [86], the dynamic model distinguishes between individual risk factors related to a genetic susceptibility, environmental risk factors and protective factors. In contrast to the model by Sarovic [86], the dynamic model in addition to physical and chemical environmental risk factors emphasizes the importance of social risk and resilience factors, and the dynamic model does not include immune function and autonomic regulation as contributing factors of their own. Still, comparable to the model by Sarovic, an operationalization of the dynamic model may be considered possible following a similar track. Assessment involves the option—at any given time within a developmental context—to be able to identify a phenotypic profile, neuroprotective aid including cognitive capacity and social support, and neuropathological pressure including all possible environmental risk factors. A distinction between quantification of measures relating to a behavioral phenotype and a clinical phenotype appears to be relevant. Quantification of a neurodevelopmental behavioral phenotype could include measures of endophenotypes, polygenic scores and other measures relating to a basic neurobiological susceptibility. Quantification of a clinical phenotype, in addition, should include measures of severity of illness reflecting suffering, e.g. QoL, measures of social adaptive function, symptom rating scores etc. Quantification of neuroprotective aid could include cognitive measures and measures of social support. Finally, quantification of neuropathological pressure could include measures of epigenetic changes and relevant measures of stress, e.g., cortisol measures, heart rate variability, etc.
The dynamic model has potentially important therapeutic implications. As implied by the dynamic model, and in line with Latham et al. [25], many different routes may lead towards the same overall clinical picture. The dynamic model reveals several points of possible intervention: (1) a primary neurobiological vulnerability of genetic origin and derived consequences with associated primary effects on neurocognitive function; (2) an endogenous epigenetic modification of genetic variants and associated primary effects on neurocognitive function; (3) the genetic and epigenetic modification resulting from physical and chemical environmental factors and associated secondary effects on neurocognitive functioning; (4) the exogenous epigenetic effects of social environment and associated secondary effects on neurocognitive and social functioning; (5) the direct effects of stress on brain tissue and the associated secondary effects on neurocognitive functioning via epigenetic modification; (6) psychological processes affecting interpretation of sensory information; and finally; (7) compensating mechanisms.
The treatment effects may depend on the reversible nature of the epigenetic mechanisms involved in the development of manifest illness. As such, treatment courses may vary dependent on the character of the genetic, epigenetic and environmental factors involved. The choice of strategy for intervention may depend on information about the relative contributions from genetic, epigenetic processes and environmental exposures to the observed psychopathological expressions. Insofar as epigenetic processes are malleable, environmental factors have the potential to modify these processes [63],[91].
In each individual case, treatment strategies may be adapted according to individual needs in light of developmental history, including the genetic and epigenetic profile, as well a thorough description of individual risk and resilience factors that may change across the developmental course. In light of present genetic and epigenetic evidence, it appears relevant to distinguish between how contributions to psychopathology may depend on the quality and quantity of affected genes, epigenetic and environmental factors and gene-environment interactions across time. Relevant therapeutic approaches may depend on the involvement of rare or common genetic variants. In light of present evidence, both pharmacological agents as well as environmental interventions potentially have a role in the future treatment of ASD.
From a molecular genetic perspective, to the extent that susceptible genetic variants are involved in early neurodevelopment, it may be relevant to develop molecular and pharmacological interventions, as has been suggested by several researchers, e.g., Siniscalco et al. [28], Szyf et al. [33], and Shork et al. [77]. DNA methylation and histone deacetylation enzymes are possible targets for epigenetic drugs that may offer potential in the treatment of psychiatric disorders [33]. Developing targeted epigenetic therapy based on the insights from genetic science on how epigenetic mechanisms are involved in pathogenesis and on knowledge about complex epigenetic mechanisms may not be without challenges, with specificity being a main challenge [33].
From a clinical therapeutic perspective, a primary focus of treatment may be to adjust expectations and thereby relieve stress. Of relevance is to identify and, if possible, adjust or eliminate factors that cause stress and strengthen resilience factors, e.g., individual compensation, scaffolding and social support. Such an approach may be consistent with the approach taken by Bishop-Fitzpatrick et al. [89] and Kim and Bottema-Beutel [104] on environmental intervention and the suggestion by Yehuda et al. [91] to focus on the effects of human experience and growth, in addition to a focus on how molecular mechanisms influences individuals and their offspring.
In light of the heterogeneous nature and high risk of comorbidity [8],[10],[98],[105], it may be important to apply multiple strategies in the treatment of ASD, including symptomatic treatment of comorbid disorders, e.g., anxiety disorders, compulsive behavior, depression, attention deficits, sleep disorders, immune system dysfunction, seizures and gastrointestinal problems. It may also be important to distinguish between whether the comorbid conditions are directly related to the molecular mechanisms involved in the development of neurodevelopmental disorders and the phenomena of autism and to understand to what extent they are secondary, i.e., related to compensatory efforts as suggested by Belmonte and coauthors [29],[80] or to stress-related phenomena.
From a clinical perspective, the introduction of dynamic multilevel models may increase opportunities to diagnose and treat ASD, and related neurodevelopmental disorders in light of their complex and heterogeneous nature. New insight into the complex nature of neurodevelopmental disorders may improve the basis of evidence that clinicians rely on. Such insight could allow them to be able to apply multiple strategies in the treatment of ASD and other neurodevelopmental disorders and to differentiate treatment according to more specific etiological knowledge. With big data and personalized medicine entering the arena of psychiatry, application of such knowledge has the potential to help improve the treatment of complex, heterogeneous neurodevelopmental disorders such as ASD. According to Latham et al. [25], the use of genetic information in personalized medicine will require access to updated data on past and current environmental exposures and current epigenetic states. Personalized medicine may support clinicians when handling and combining the enormous amount of clinical and scientific data available today, e.g., genetic and epigenetic evidence, neurobiological, neurocognitive, psychological and social evidence. A major premise for the success of such an endeavor is the successful development of algorithms to guide the machine learning models applied in personalized medicine. To avoid misinterpretation of arbitrary associations as etiologically related, such algorithms will have to refer to coherent theory.
A simple theoretical model of the complex dynamics involved in the development of neurodevelopmental disorders and the phenomenon of autism may help explain the observed biological and clinical heterogeneity of ASD and related neurodevelopmental disorders. The suggested dynamic model illustrates how psychodynamics may involve the interaction between a basic neurobiological susceptibility, compensating mechanisms, and stress-related releasing factors. The model applies an individualized and dynamic approach embedded in a developmental context integrating several levels of influence—genetic, biological, cognitive, psychological, and social—involved in the dynamic interaction between an individual and their environment across time.
The model suggests that at different stages across development, environmental risk factors may affect genetic and epigenetic mechanisms, with potential effects on neurodevelopment and stress-sensitivity influencing the time of clinical onset. Environmental exposures across time, genetic variation and epigenetic processes related to preconception exposure, intrauterine exposure, and environmental exposures early and later in life may all affect the psychopathological course of illness.
The molecular mechanisms involved in the development of neurodevelopmental disorders including ASD are complex. Across time, accumulated epigenetic effects of environmental risk factors increase stress reactivity and the risk for manifest neurodevelopmental illness. At different stages across development, physical, chemical and social environmental risk factors may trigger epigenetic mechanisms that may affect neurodevelopment, alter stress-sensitivity or affect behavior. Depending on the genetic and environmental risk load, gene-environment interactions affect the time of onset of clinical illness. The genetic load reflects both the quality and quantity of a genetic susceptibility. The environmental risk load is reflected in epigenetic marks associated with susceptible genes with epigenetic effects depending on time of exposure as well as the quality and quantity of the environmental risk factors and the presence of resilience factors counteracting the effect of risk.
In light of present genetic and epigenetic evidence, major future challenges for neurodevelopmental research appear to be identifying and understanding the regulation of and interactions between rare and common genetic variants, how these interactions result in the heterogeneous clinical expressions of neurodevelopmental disorders, and how environmental factors modulate the overall risk via epigenetic mechanisms. Such knowledge may have important therapeutic implications.
As suggested by the dynamic model, many routes are possible, and in most cases, several events must take place for manifest clinical illness to occur. In particular, in the cases where polygenic risk from genes associated with common variants is prevalent, this may be characteristic, whereas manifest clinical illness may be present right after birth in the rarer cases resulting from genetic syndromes and gene mutations having large effects.
The processes are complex. According to the predictions of the dynamic model, it is relevant to distinguish between neurobiological susceptibility, which is associated with neurodevelopmental pathological load and stress sensitivity, and the severity of illness, which is related to manifest clinical illness and persistent stress. An observed discrepancy between behavioral expression and underlying cognitive or neurobiological susceptibility may be due to compensation. The psychodynamics may involve stress-related releasing factors, including normal events across development found challenging by cognitively vulnerable individuals.
In order to understand fully the psychodynamics involved in the pathogenesis of neurodevelopmental disorders and the phenomenon of autism, future theoretical models guiding research may need to apply a transdiagnostic dimensional translational approach including molecular mechanisms, cognitive and psychological processes as well as social dynamics, as emphasized by the dynamic model. Relevant choices of intervention and prevention may depend on etiology and ethical considerations.
Application of the suggested model in future psychopathological research may contribute new knowledge about the pathogenesis of mental illness and the dynamics involved in the development of ASD and related neurodevelopmental disorders, including schizophrenia, as well as of the phenomena lying behind these disorders.
The illustrated dynamics suggested by the model may help guide future development of personalized medicine in neurodevelopmental disorders. Furthermore, the model may be useful as a pedagogical tool for communication and psychoeducation to help disentangle the different mechanisms involved in development of manifest clinical illness. The model may help identify relevant points of intervention in the treatment of neurodevelopmental disorders including ASD and prevention of future progression in the course of illness. As a therapeutic tool, the model may shed light on the different mechanisms involved in the development of illness, and how these mechanisms work and interact at different time points depending on multiple factors unique to the individual, thereby contributing to the development of manifest disease. As illustrated by the model, the course of illness may depend not only on the development of the brain but also on the ability of an individual to cope in a complex world. In addition, the course may depend on social environmental challenges encountered by an individual across time.
Theoretical contributions to research may add to the overall scientific effort of unravelling the mechanisms involved in the pathogenesis of neurodevelopmental disorders, which at some time in the future may help augment our understanding of these disorders and may help alleviate or eliminate the stress and suffering related to these conditions.
[1] |
Baird G, Simonoff E, Pickles A, et al. (2006) Prevalence of disorders of the autism spectrum in a population cohort of children in South Thames: the Special Needs and Autism Project (SNAP). Lancet 368: 210-215. doi: 10.1016/S0140-6736(06)69041-7
![]() |
[2] |
Baxter AJ, Brugha TS, Erskine HE, et al. (2015) The epidemiology and global burden of autism spectrum disorders. Psychol Med 45: 601-613. doi: 10.1017/S003329171400172X
![]() |
[3] |
Brugha TS, Spiers N, Bankart J, et al. (2016) Epidemiology of autism in adults across age groups and ability levels. Br J Psychiatry 209: 498-503. doi: 10.1192/bjp.bp.115.174649
![]() |
[4] |
Elsabbagh M, Divan G, Koh Y-J, et al. (2012) Global prevalence of autism and other pervasive developmental disorders. Autism Res 5: 160-179. doi: 10.1002/aur.239
![]() |
[5] |
Atladottir HO, Gyllenberg D, Langridge A, et al. (2015) The increasing prevalence of reported diagnoses of childhood psychiatric disorders: a descriptive multinational comparison. Eur Child Adolesc Psychiatry 24: 173-183. doi: 10.1007/s00787-014-0553-8
![]() |
[6] |
Hansen SN, Schendel DE, Parner ET (2015) Explaining the increase in the prevalence of autism spectrum disorders. The proportion attributable to changes in reporting practices. JAMA Pediatr 169: 56-62. doi: 10.1001/jamapediatrics.2014.1893
![]() |
[7] |
Simonoff E (2012) Autism spectrum disorder: prevalence and cause may be bound together. Br J Psych 201: 88-89. doi: 10.1192/bjp.bp.111.104703
![]() |
[8] |
Gillberg C (2010) The ESSENCE in child psychiatry: Early Symptomatic Syndromes Eliciting Neurodevelopmental Clinical Examinations. Res Dev Dis 31: 1543-1551. doi: 10.1016/j.ridd.2010.06.002
![]() |
[9] |
Grove J, Ripke S, Als TD, et al. (2019) Identification of common genetic risk variants for autism spectrum disorder. Nat Genet 51: 431-444. doi: 10.1038/s41588-019-0344-8
![]() |
[10] |
Simonoff E, Pickles A, Charman, et al. (2008) Psychiatric disorders in children with autism spectrum disorders: Prevalence, comorbidity, and associated factors in a population-derived sample. J Am Acad Child Adolesc Psychiatry 47: 921-929. doi: 10.1097/CHI.0b013e318179964f
![]() |
[11] |
Wender CLA, Veenstra-VanderWeele J (2017) Challenge and Potential for Research on Gene-Environment Interactions in Autism Spectrum Disorder. Gene-Environment Transactions in Developmental Psychopathology, Advances in Development and Psychopathology: Brain Research Foundation Symposium Series 2 Springer International Publishing AG, 157-176. doi: 10.1007/978-3-319-49227-8_9
![]() |
[12] |
Sandin S, Lichtenstein P, Kuja-Halkola R, et al. (2014) The familial risk of autism. JAMA 311: 1770-1777. doi: 10.1001/jama.2014.4144
![]() |
[13] |
Robinson EB, St Pourcain B, Anttila V, et al. (2016) Genetic risk for autism spectrum disorders and neuropsychiatric variation in the general population. Nat Genet 48: 552-555. doi: 10.1038/ng.3529
![]() |
[14] |
Craddock N, Owen M (2010) The Kraepelinian dichotomy – going, going…but still not gone. Br J Psychiatry 196: 92-95. doi: 10.1192/bjp.bp.109.073429
![]() |
[15] | Cross-Disorder Group of the Psychiatric Genomics Consortium (2013) Identification of risk loci with shared effects on five major psychiatric disorders: a genome-wide analysis. Lancet 381: 1371-1379. |
[16] |
Aggernæs B (2018) Autism: A transdiagnostic, dimensional construct of reasoning? Eur J Neurosci 47: 515-533. doi: 10.1111/ejn.13599
![]() |
[17] | Aggernæs B (2016) Rethinking the concept of psychosis and the link between autism and Schizophrenia. Scand J Child Adol Psychiat Psychol 4: 4-11. |
[18] | Bleuler E (1911) Dementia Praecox oder Gruppe der Schizophrenien Leipzig: Deuticke. |
[19] | Piaget J (1967) La psychologie de l'intelligence Paris: A. Colin. |
[20] | Alberoni F (1984) Movement and Institution New York: Columbia University Press. |
[21] |
Aggernæs A, Haugsted R (1976) Experienced reality in three to six year old children: A study of direct reality testing. J Child Psychol Psychiat 17: 323-335. doi: 10.1111/j.1469-7610.1976.tb00407.x
![]() |
[22] |
Aggernæs A, Paikin H, Vitger J (1981) Experienced reality in schizophrenia: How diffuse is the defect in reality testing? Indian J Psychol Med 4: 1-13. doi: 10.1177/0975156419810101
![]() |
[23] |
Clemmensen L, van Os J, Skovgaard AM, et al. (2014) Hyper-Theory-of-Mind in children with psychotic experiences. PLoS ONE 9: e113082. doi: 10.1371/journal.pone.0113082
![]() |
[24] |
Hallmayer J, Cleveland S, Torres A, et al. (2011) Genetic heritability and shared environmental factors among twin pairs with autism. Arch Gen Psychiatry 68: 1095-1102. doi: 10.1001/archgenpsychiatry.2011.76
![]() |
[25] |
Latham KE, Sapienza C, Engel N (2012) The epigenetic lorax: gene–environment interactions in human health. Epigenomics 4: 383-402. doi: 10.2217/epi.12.31
![]() |
[26] |
Miake K, Hirasawa T, Koide T, et al. (2012) Epigenetics in autism and other neurodevelopmental diseases. Adv Exp Med Biol 724: 91-98. doi: 10.1007/978-1-4614-0653-2_7
![]() |
[27] |
Shanen NC (2006) Epigenetics of autism spectrum disorders. Human Molecular Genetics 15: R138-R150. doi: 10.1093/hmg/ddl213
![]() |
[28] |
Siniscalco D, Cirillo A, Bradstreet JJ, et al. (2013) Epigenetic findings in autism: New perspectives for therapy. Int J Environ Res Public Health 10: 4261-4273. doi: 10.3390/ijerph10094261
![]() |
[29] |
Belmonte MK, Cook EH, Anderson GM, et al. (2004) Autism as a disorder of neural information processing: directions for research and targets for therapy. Mol Psychiatry 9: 646-663. doi: 10.1038/sj.mp.4001499
![]() |
[30] |
Courchesne E (2002) Abnormal early brain development in autism. Mol Psychiatry 7: S21-S23. doi: 10.1038/sj.mp.4001169
![]() |
[31] |
Menon V (2011) Large-scale brain networks and psychopathology: A unifying triple network model. Trends Cogn Sci 15: 483-506. doi: 10.1016/j.tics.2011.08.003
![]() |
[32] |
Szatmari P (2000) The classification of autism, Asperger's syndrome, and pervasive developmental disorder. Can J Psychiat 45: 731-738. doi: 10.1177/070674370004500806
![]() |
[33] |
Szyf M (2009) Epigenetics, DNA methylation, and chromatin modifying drugs. Annu Rev Pharmacol Toxicol 49: 243-263. doi: 10.1146/annurev-pharmtox-061008-103102
![]() |
[34] |
Plomin R (2011) Commentary: Why are children in the same family so different? Non-shared environment three decades later. Int J Epidemiol 40: 582-592. doi: 10.1093/ije/dyq144
![]() |
[35] |
Larsson HJ, Eaton WW, Madsen KM, et al. (2005) Risk factors for autism: perinatal factors, parental psychiatric history, and socioeconomic status. Am J Epidemiol 161: 916-925. doi: 10.1093/aje/kwi123
![]() |
[36] |
Lauritsen MB, Pedersen CB, Mortensen PB (2005) Effects of familial risk factors and place of birth on the risk of autism: a nationwide registerbased study. J Child Psychol Psyc 46: 963-971. doi: 10.1111/j.1469-7610.2004.00391.x
![]() |
[37] |
Gardener H, Spiegelman D, Buka SL (2009) Prenatal risk factors for autism: comprehensive meta-analysis. Br J Psychiatry 195: 7-14. doi: 10.1192/bjp.bp.108.051672
![]() |
[38] | Ramaswami G, Geschwind DH (2018) Chapter 21 Genetics of autism spectrum disorder. Handbook of Clinical Neurology, Vol. 147 (3rd series) Neurogenetics, Part I Elsevier, 321-329. |
[39] |
Betancur C (2011) Etiological heterogeneity in autism spectrum disorders: More than 100 genetic and genomic disorders and still counting. Brain Res 1380: 42-77. doi: 10.1016/j.brainres.2010.11.078
![]() |
[40] |
Nava C, Keren B, Mignot C, et al. (2014) Prospective diagnostic analysis of copy number variants using SNP microarrays in individuals with autism spectrum disorders. Eur J Hum Genet 22: 71-78. doi: 10.1038/ejhg.2013.88
![]() |
[41] | The Autism Genome Project Consortium (2007) Mapping autism risk loci using genetic linkage and chromosomal rearrangements. Nat Genet 39: 319-328. |
[42] |
Weiner DJ, Wigdor EM, Ripke S, et al. (2017) Polygenic transmission disequilibrium confirms that common and rare variation act additively to create risk for autism spectrum disorders. Nat Genet 49: 978-985. doi: 10.1038/ng.3863
![]() |
[43] |
Gaugler T, Klei L, Sanders SJ, et al. (2014) Most genetic risk for autism resides with common variation. Nat Genet 46: 881-885. doi: 10.1038/ng.3039
![]() |
[44] |
Guilmatre A, Dubourg C, Mosca AL, et al. (2009) Recurrent rearrangements in synaptic and neurodevelopmental genes and shared biologic pathways in schizophrenia, autism, and mental retardation. Arch Gen Psychiat 66: 947-956. doi: 10.1001/archgenpsychiatry.2009.80
![]() |
[45] |
McCarthy SE, Gillis J, Kramer M, et al. (2014) De novo mutations in schizophrenia implicate chromatin remodeling and support a genetic overlap with autism and intellectual disability. Mol Psychiatry 19: 652-658. doi: 10.1038/mp.2014.29
![]() |
[46] |
Hannon E, Schendel D, Ladd-Acosta C, et al. (2018) Elevated polygenic burden for autism is associated with differential DNA methylation at birth. Genome Med 10: 19. doi: 10.1186/s13073-018-0527-4
![]() |
[47] | Tran NQV, Miyake K (2017) Neurodevelopmental disorders and environmental toxicants: epigenetics as an underlying mechanism. Int J Genomics 2017: 7526592. |
[48] |
Waddington CH (2012) The epigenotype. Int J Epidemiol 41: 10-13. doi: 10.1093/ije/dyr184
![]() |
[49] |
Fraga MF, Ballestar E, Paz MF, et al. (2005) Epigenetic differences arise during the lifetime of monozygotic twins. Proc Natl Acad Sci USA 102: 10604-10609. doi: 10.1073/pnas.0500398102
![]() |
[50] |
Lee R, Avramopoulos D (2014) Introduction to Epigenetics in Psychiatry. Epigenetics in Psychiatry Elsevier Inc., 3-25. doi: 10.1016/B978-0-12-417114-5.00001-2
![]() |
[51] |
Bale TL (2014) Lifetime stress experience: transgenerational epigenetics and germ cell programming. Dialogues Clin Neurosci 16: 297-305. doi: 10.31887/DCNS.2014.16.3/tbale
![]() |
[52] | Gos M (2013) Epigenetic mechanisms of gene expression regulation in neurological diseases. Acta Neurobiol Exp 73: 19-37. |
[53] |
James SJ, Melnyk S, Jernigan S, et al. (2006) Metabolic endophenotype and related genotypes are associated with oxidative stress in children with autism. Am J Med Genet Part B 141B: 947-956. doi: 10.1002/ajmg.b.30366
![]() |
[54] |
Deth R, Muratore C, Benzecry J, et al. (2008) How environmental and genetic factors combine to cause autism: A redox/methylation hypothesis. Neurotoxicology 29: 190-201. doi: 10.1016/j.neuro.2007.09.010
![]() |
[55] |
Homs A, Codina-Solà M, Rodríguez-Santiago B, et al. (2016) Genetic and epigenetic methylation defects and implication of the ERMN gene in autism spectrum disorders. Transl Psychiatry 6: e855. doi: 10.1038/tp.2016.120
![]() |
[56] |
Ginsberg MR, Rubin RA, Falcone T, et al. (2012) Brain transcriptional and epigenetic associations with autism. PLoS ONE 7: e44736. doi: 10.1371/journal.pone.0044736
![]() |
[57] |
Wong CCY, Meaburn EL, Ronald A, et al. (2014) Methylomic analysis of monozygotic twins discordant for autism spectrum disorder and related behavioural traits. Mol Psychiatry 19: 495-503. doi: 10.1038/mp.2013.41
![]() |
[58] |
Minnis H (2013) Maltreatment-Associated Psychiatric Problems: An Example of Environmentally Triggered ESSENCE? Sci World J 2013: 148468. doi: 10.1155/2013/148468
![]() |
[59] | Pritchett R, Pritchett J, Marshall E, et al. (2013) Reactive attachment disorder in the general population: a hidden ESSENCE disorder. Sci World J 2013: 81815. |
[60] |
Dinkler L, Lundström S, Gajwani R, et al. (2017) Maltreatment-associated neurodevelopmental disorders: a co-twin control analysis. J Child Psychol Psychiatr 58: 691-701. doi: 10.1111/jcpp.12682
![]() |
[61] |
McEwen BS (2008) Central effects of stress hormones in health and disease: understanding the protective and damaging effects of stress and stress mediators. Eur J Pharmacol 583: 174-185. doi: 10.1016/j.ejphar.2007.11.071
![]() |
[62] | Rostène W, Sarrieau A, Nicot A, et al. (1995) Steroid effects on brain functions: An example of the action of glucocorticoids on central dopaminergic and neurotensinergic systems. J Psychiatry Neurosci 20: 349-356. |
[63] |
Meaney MJ, Szyf M (2005) Environmental programming of stress responses through DNA methylation: life at the interface between a dynamic environment and a fixed genome. Dialogues Clin Neurosci 7: 103-123. doi: 10.31887/DCNS.2005.7.2/mmeaney
![]() |
[64] |
Weaver ICG, Champagne FA, Brown SE, et al. (2005) Reversal of maternal programming of stress responses in adult offspring through methyl supplementation: Altering epigenetic marking later in life. J Neurosci 25: 11045-11054. doi: 10.1523/JNEUROSCI.3652-05.2005
![]() |
[65] |
McGowan PO, Matthews SG (2018) Prenatal stress, glucocorticoids, and developmental programming of the stress response. Endocrinology 159: 69-82. doi: 10.1210/en.2017-00896
![]() |
[66] |
Stenz L, Schechter DS, Serpa SR, et al. (2018) Intergenerational transmission of DNA methylation signatures associated with early life stress. Curr Genomics 19: 665-675. doi: 10.2174/1389202919666171229145656
![]() |
[67] |
Oberlander TF, Weinberg J, Papsdorf M, et al. (2008) Prenatal exposure to maternal depression, neonatal methylation of human glucocorticoid receptor gene (NR3C1) and infant cortisol stress responses. Epigenetics 3: 97-106. doi: 10.4161/epi.3.2.6034
![]() |
[68] | Corbett BA, Mendoza S, Wegelin JA, et al. (2008) Variable cortisol circadian rhythms in children with autism and anticipatory stress. J Psychiatry Neurosci 33: 227-234. |
[69] |
Corbett BA, Schupp CW, Levine S, et al. (2009) Comparing cortisol, stress, and sensory sensitivity in children with autism. Autism Res 2: 39-49. doi: 10.1002/aur.64
![]() |
[70] |
Corbett BA, Schupp CW, Lanni KE (2012) Comparing biobehavioral profiles across two social stress paradigms in children with and without autism spectrum disorders. Mol Autism 3: 13. doi: 10.1186/2040-2392-3-13
![]() |
[71] |
Corbett BA, Muscatello RA, Blain SD (2016) Impact of sensory sensitivity on physiological stress response and novel peer interaction in children with and without autism spectrum disorder. Front Neurosci 10: 278. doi: 10.3389/fnins.2016.00278
![]() |
[72] |
Tordjman S, Anderson GM, Kermarrec S, et al. (2014) Altered circadian patterns of salivary cortisol in low-functioning children and adolescents with autism. Psychoneuroendocrinology 50: 227-245. doi: 10.1016/j.psyneuen.2014.08.010
![]() |
[73] |
Bishop-Fitzpatrick L, Mazefsky CA, Minshew NJ, et al. (2015) The relationship between stress and social functioning in adults with autism spectrum disorder and without intellectual disability. Autism Res 8: 164-173. doi: 10.1002/aur.1433
![]() |
[74] |
Bishop-Fitzpatrick L, Minshew NJ, Mazefsky CA, et al. (2017) Perception of life as stressful, not biological response to stress, is associated with greater social disability in adults with autism spectrum disorder. Autism Dev Disord 47: 1-16. doi: 10.1007/s10803-016-2910-6
![]() |
[75] |
Sønderby IE, Gústafsson Ó, Doan NT, et al. (2018) Dose response of the 16p11.2 distal copy number variant on intracranial volume and basal ganglia. Mol Psychiatry 25: 584-602. doi: 10.1038/s41380-018-0118-1
![]() |
[76] |
Turner TN, Coe BP, Dickel DE, et al. (2017) Genomic patterns of de novo mutation in simplex autism. Cell 171: 710-722. doi: 10.1016/j.cell.2017.08.047
![]() |
[77] |
Schork AJ, Won H, Appadurai V, et al. (2019) A genome-wide association study of shared risk across psychiatric disorders implicates gene regulation during fetal neurodevelopment. Nat Neurosci 22: 353-361. doi: 10.1038/s41593-018-0320-0
![]() |
[78] |
Gandal MJ, Haney JR, Parikshak NN, et al. (2018) Shared molecular neuropathology across major psychiatric disorders parallels polygenic overlap. Science 359: 693-697. doi: 10.1126/science.aad6469
![]() |
[79] |
Shulha HP, Cheung I, Guo Y, et al. (2013) Coordinated cell type–specific epigenetic remodeling in prefrontal cortex begins before birth and continues into early adulthood. PLoS Genet 9: e1003433. doi: 10.1371/journal.pgen.1003433
![]() |
[80] |
Belmonte MK, Yurgelun-Todd DA (2003) Functional anatomy of impaired selective attention and compensatory processing in autism. Brain Res Cogn Res 17: 651-664. doi: 10.1016/S0926-6410(03)00189-7
![]() |
[81] |
Livingston LA, Happé F (2017) Conceptualising compensation in neurodevelopmental disorders: Reflections from autism spectrum disorders. Neurosci Biobehav Rev 80: 729-742. doi: 10.1016/j.neubiorev.2017.06.005
![]() |
[82] |
Weems CF (2015) Biological correlates of child and adolescent responses to disaster exposure: A bio-ecological model. Curr Psychiatry Rep 17: 1-7. doi: 10.1007/s11920-015-0588-7
![]() |
[83] |
Weems CF, Russel JD, Neill EL, et al. (2019) Annual Research Review: Pediatric posttraumatic stress disorder from a neurodevelopmental network perspective. J Child Psychol Psychiatr 60: 395-408. doi: 10.1111/jcpp.12996
![]() |
[84] | Ouss-Ryngaert L, Golse B (2010) Linking neuroscience and psychoanalysis from a developmental perspective: Why and how? J Physiol 104: 303-308. |
[85] |
Hosman CMH, van Doesum KTM, van Santvoort F (2009) Prevention of emotional problems and psychiatric risks in children of parents with a mental illness in the Netherlands: I. The scientific basis to a comprehensive approach. Aust e-J Advancement Ment Health 8: 250-263. doi: 10.5172/jamh.8.3.250
![]() |
[86] | Sarovic D (2018) A framework for neurodevelopmental disorders: Operationalization of a pathogenetic triad for clinical and research use. Gillberg Neuropsychiatry Centre, Institute of Neuroscience and Physiology, Sahlgrenska Academy University of Gothenburg Available from: https://psyarxiv.com/mbeqh/. |
[87] |
Caspi A, Houts RM, Belsky DW, et al. (2014) The p factor: One general psychopathology factor in the structure of psychiatric disorders? Clin Psychol Sci 2: 119-137. doi: 10.1177/2167702613497473
![]() |
[88] |
Sameroff AJ, Mackenzie MJ (2003) Research strategies for capturing transactional models of development: The limits of the possible. Dev Psychopathol 15: 613-640. doi: 10.1017/S0954579403000312
![]() |
[89] |
Bishop-Fitzpatrick L, Mazefsky CA, Eack SM (2018) The combined impact of social support and perceived stress on quality of life in adults with autism spectrum disorder and without intellectual disability. Autism 22: 703-711. doi: 10.1177/1362361317703090
![]() |
[90] |
Bishop-Fitzpatrick L, Smith LE, Greenberg JS, et al. (2017) Participation in recreational activities buffers the impact of perceived stress on quality of life in adults with autism spectrum disorder. Autism Res 10: 973-982. doi: 10.1002/aur.1753
![]() |
[91] |
Yehuda R, Lehrner A, Bierer LM (2018) The public reception of putative epigenetic mechanisms in the transgenerational effects of trauma. Environ Epigenet 4: 1-7. doi: 10.1093/eep/dvy018
![]() |
[92] |
Clarke T-K, Lupton MK, Fernandez-Pujals AM, et al. (2016) Common polygenic risk for autism spectrum disorder (ASD) is associated with cognitive ability in the general population. Mol Psychiatry 21: 419-425. doi: 10.1038/mp.2015.12
![]() |
[93] |
Robinson EB, Samocha KE, Kosmicki JA, et al. (2014) Autism spectrum disorder severity reflects the average contribution of de novo and familial influences. Proc Natl Acad Sci USA 111: 15161-15165. doi: 10.1073/pnas.1409204111
![]() |
[94] |
Sanders SJ, He X, Willsey AJ, et al. (2015) Insights into autism spectrum disorder genomic architecture and biology from 71 risk loci. Neuron 87: 1215-1233. doi: 10.1016/j.neuron.2015.09.016
![]() |
[95] |
Bakermans-Kranenburg MJ, van Ijzendoorn MH (2006) Gene-environment interaction of the dopamine D4 receptor (DRD4) and observed maternal insensitivity predicting externalizing behavior in preschoolers. Dev Psychobiol 48: 406-409. doi: 10.1002/dev.20152
![]() |
[96] |
Kumsta R, Stevens S, Brookes K, et al. (2010) 5HTT genotype moderates the influence of early institutional deprivation on emotional problems in adolescence: evidence from the English and Romanian Adoptee (ERA) study. J Child Psychol Psychiatry 51: 755-762. doi: 10.1111/j.1469-7610.2010.02249.x
![]() |
[97] |
Yang C-J, Tan H-P, Yang F-Y (2015) The cortisol, serotonin and oxytocin are associated with repetitive behavior in autism spectrum disorder. Res Autism Spectr Disord 18: 12-20. doi: 10.1016/j.rasd.2015.07.002
![]() |
[98] |
Meier SM, Petersen L, Schendel DE, et al. (2015) Obsessive-compulsive disorder and autism spectrum disorders: Longitudinal and offspring risk. PLoS ONE 10: e0141703. doi: 10.1371/journal.pone.0141703
![]() |
[99] |
Valla JM, Belmonte MK (2013) Detail-oriented cognitive style and social communicative deficits, within and beyond the autism spectrum: independent traits that grow into developmental interdependence. Dev Rev 33: 371-398. doi: 10.1016/j.dr.2013.08.004
![]() |
[100] |
Lai MC, Lombardo MV, Ruigrok AN, et al. (2017) Quantifying and exploring camouflaging in men and women with autism. Autism 21: 690-702. doi: 10.1177/1362361316671012
![]() |
[101] |
Atladóttir HO, Thorsen P, Schendel DE, et al. (2010) Association of hospitalization for infection in childhood with diagnosis of autism spectrum disorders. Arch Pediatr Adolesc Med 164: 470-477. doi: 10.1001/archpediatrics.2010.9
![]() |
[102] |
Nudel R, Wang Y, Appadurai V, et al. (2019) A large-scale genomic investigation of susceptibility to infection and its association with mental disorders in the Danish population. Transl Psychiatry 9: 283. doi: 10.1038/s41398-019-0622-3
![]() |
[103] |
Danese A, Moffitt TE, Arseneault L, et al. (2017) The origins of cognitive deficits in victimized children: Implications for neuroscientists and clinicians. Am J Psychiatry 174: 349-361. doi: 10.1176/appi.ajp.2016.16030333
![]() |
[104] |
Kim SY, Bottema-Beutel K (2019) A meta regression analysis of quality of life correlates in adults with ASD. Res Autism Spectr Disord 63: 23-33. doi: 10.1016/j.rasd.2018.11.004
![]() |
[105] |
Plana-Ripoll O, Pedersen CB, Holtz Y, et al. (2019) Exploring comorbidity within mental disorders among a danish national population. JAMA Psychiatry 76: 259-270. doi: 10.1001/jamapsychiatry.2018.3658
![]() |
[106] |
Cuthbert N, Insel R (2013) Toward the future of psychiatric diagnosis: the seven pillars of RDoC. BMC Med 11: 126. doi: 10.1186/1741-7015-11-126
![]() |
[107] | (2013) American Psychiatric AssociationDiagnostic and Statistical Manual of Mental Disorders.American Psychiatric Publishing. |
[108] | (1993) WHOThe ICD-10 Classification of Mental and Behavioural Disorders: Diagnostic Criteria for Research. Geneva: World Health Organization. |
[109] |
Polanczyk GV, Casella C, Jaffee SR (2019) Commentary: ADHD lifetime trajectories and the relevance of the developmental perspective to Psychiatry: reflections on Asherson and Agnew-Blais. J Child Psychol Psychiatry 60: 353-355. doi: 10.1111/jcpp.13050
![]() |
[110] |
Narusyte J, Neiderhiser JM, D'Onofrio BM, et al. (2008) Testing different types of genotype–environment correlation: An extended children-of-twins model. Dev Psychol 44: 1591-1603. doi: 10.1037/a0013911
![]() |
[111] |
Sykes NH, Lamb JA (2007) Autism: The quest for the genes. Expert Rev Mol Med 9: 1-15. doi: 10.1017/S1462399407000452
![]() |
1. | Darko Sarovic, A Unifying Theory for Autism: The Pathogenetic Triad as a Theoretical Framework, 2021, 12, 1664-0640, 10.3389/fpsyt.2021.767075 | |
2. | Jonathan Lassen, Bob Oranje, Martin Vestergaard, Malene Foldager, Troels W. Kjær, Sidse Arnfred, Bodil Aggernæs, Reduced mismatch negativity in children and adolescents with autism spectrum disorder is associated with their impaired adaptive functioning, 2022, 15, 1939-3792, 1469, 10.1002/aur.2738 |
Environmental stressors | Mechanisms |
Physical/chemical agents:
|
|
Social stressors:
|
|
Mechanisms | Type of effects |
Preconception exposure transgenerational genomic transmission |
|
|
|
|
|
Intrauterine exposure maternal transmission of environmental risk |
|
|
|
|
|
|
|
Infant exposure transmission of environmental risk |
|
|
|
|
|
|
|
|
|
Childhood exposure Adolescent exposure Adult exposure transmission of environmental risk |
|
|
|
|
|
|
|
|
|
|
|
|
|
Environmental stressors | Mechanisms |
Physical/chemical agents:
|
|
Social stressors:
|
|
Mechanisms | Type of effects |
Preconception exposure transgenerational genomic transmission |
|
|
|
|
|
Intrauterine exposure maternal transmission of environmental risk |
|
|
|
|
|
|
|
Infant exposure transmission of environmental risk |
|
|
|
|
|
|
|
|
|
Childhood exposure Adolescent exposure Adult exposure transmission of environmental risk |
|
|
|
|
|
|
|
|
|
|
|
|
|