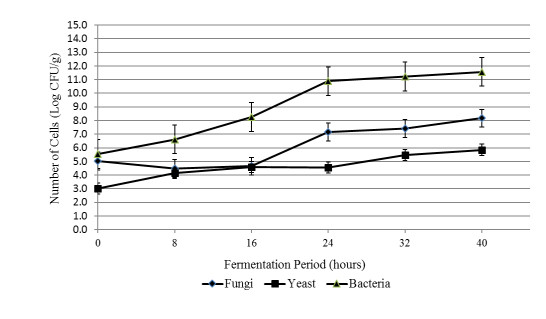
The aim of the research was to study the effect of inoculum type and fermentation time on microbial growth patterns (yeast, fungi and bacteria), β-glucan formation and antioxidant activity during soybean fermentation into tempe. The research was conducted using factorial Completely Randomized Block Design with 3 replications. The first factor was the types of inoculum: commercial inoculum of tempe, Raprima (3%), a single inoculum of S. cerevisiae (3%), a single inoculum of R. oligosporus (3%), and mixed inoculum of 1.5% S. cerevisiae and 1.5% R. oligosporus. The second factor was the length of fermentation which consisted of 0, 8, 16, 24, 32 and 40 hours at room temperature. Regarding the number of fungi, yeasts and bacteria, the observational data were presented descriptively in the form of graphs, while for the data from the analysis of β-glucan and antioxidant activity, the data obtained were analyzed for variance with analysis of variance (ANOVA) and then analyzed further by the Least Significant Difference (LSD) at the 5% significance level. The results showed that the type of inoculum and duration of fermentation had an effect on increasing the growth of fungi, yeasts and bacteria, as well as increasing β-glucan content and the antioxidant activity of tempe. Yeast growth had a more dominant effect on increasing β-glucan content and antioxidant activity compared to fungi and bacteria. Tempe inoculated with a mixed inoculum of 1.5% R. oligosporus + 1.5% S. cerevisiae, resulted in the highest β-glucan content of 0.58% and the highest antioxidant activity at 82.42%. In conclusion, a mixed inoculum of 1.5% R. oligosporus + 1.5% S. cerevisiae with 36−40 hours of fermentation produced tempe with the highest β-glucan content and antioxidant activity. Therefore, the β-glucan content causes tempe to have better potential health benefits than tempe without the addition of S. cerevisiae.
Citation: Samsul Rizal, Maria Erna Kustyawati, Murhadi, Udin Hasanudin, Subeki. The effect of inoculum types on microbial growth, β-glucan formation and antioxidant activity during tempe fermentation[J]. AIMS Agriculture and Food, 2022, 7(2): 370-386. doi: 10.3934/agrfood.2022024
[1] | Birabrata Nayak, Bibhu Prasad Panda . Modelling and optimization of texture profile of fermented soybean using response surface methodology. AIMS Agriculture and Food, 2016, 1(4): 409-418. doi: 10.3934/agrfood.2016.4.409 |
[2] | Budi Suarti, Sukarno, Ardiansyah, Slamet Budijanto . Bio-active compounds, their antioxidant activities, and the physicochemical and pasting properties of both pigmented and non-pigmented fermented de-husked rice flour. AIMS Agriculture and Food, 2021, 6(1): 49-64. doi: 10.3934/agrfood.2021004 |
[3] | Orbe Chamorro Mayra, Luis- Armando Manosalvas-Quiroz, Nicolás Pinto Mosquera, Iván Samaniego . Effect of fermentation parameters on the antioxidant activity of Ecuadorian cocoa (Theobroma cacao L.). AIMS Agriculture and Food, 2024, 9(3): 872-886. doi: 10.3934/agrfood.2024047 |
[4] | María Cámara-Ruiz, José María García Beltrán, Francisco Antonio Guardiola, María Ángeles Esteban . In vitro and in vivo effects of purslane (Portulaca oleracea L.) on gilthead seabream (Sparus aurata L.). AIMS Agriculture and Food, 2020, 5(4): 799-824. doi: 10.3934/agrfood.2020.4.799 |
[5] | Marlin Marlin, Marulak Simarmata, Umi Salamah, Waras Nurcholis . Effect of nitrogen and potassium application on growth, total phenolic, flavonoid contents, and antioxidant activity of Eleutherine palmifolia. AIMS Agriculture and Food, 2022, 7(3): 580-593. doi: 10.3934/agrfood.2022036 |
[6] | The Han Nguyen, Patrick Achiever Blamo Jr, Xiaoyong Liu, Thi Van Anh Tran, Sang Moo Kim . β-Glucuronidase inhibitory activity of bromophenol isolated from red alga Grateloupia lancifolia. AIMS Agriculture and Food, 2021, 6(2): 551-559. doi: 10.3934/agrfood.2021032 |
[7] | Abdul Basit M. Gaba, Mohamed A. Hassan, Ashraf A. Abd El-Tawab, Mohamed A. Abdelmonem, Mohamed K. Morsy . Impact of low energy electron beam on black pepper (Piper nigrum L.) microbial reduction, quality parameters, and antioxidant activity. AIMS Agriculture and Food, 2022, 7(3): 737-749. doi: 10.3934/agrfood.2022045 |
[8] | Albert Nugraha, Asadin Briliantama, M Umar Harun, Li Sing-Chung, Chin Xuan Tan, Vuanghao Lim, Amir Husni, Widiastuti Setyaningsih . Ultrasound-assisted extraction of phenolic compounds from ear mushrooms (Auricularia auricula-judae): Assessing composition and antioxidant activity during fruiting body development. AIMS Agriculture and Food, 2024, 9(4): 1134-1150. doi: 10.3934/agrfood.2024059 |
[9] | Nicolas Nagahama, Bruno Gastaldi, Michael N. Clifford, María M. Manifesto, Renée H. Fortunato . The influence of environmental variations on the phenolic compound profiles and antioxidant activity of two medicinal Patagonian valerians (Valeriana carnosa Sm. and V. clarionifolia Phil.). AIMS Agriculture and Food, 2021, 6(1): 106-124. doi: 10.3934/agrfood.2021007 |
[10] | Evi Mintowati Kuntorini, Laurentius Hartanto Nugroho, Maryani, Tri Rini Nuringtyas . Maturity effect on the antioxidant activity of leaves and fruits of Rhodomyrtus tomentosa (Aiton.) Hassk.. AIMS Agriculture and Food, 2022, 7(2): 282-296. doi: 10.3934/agrfood.2022018 |
The aim of the research was to study the effect of inoculum type and fermentation time on microbial growth patterns (yeast, fungi and bacteria), β-glucan formation and antioxidant activity during soybean fermentation into tempe. The research was conducted using factorial Completely Randomized Block Design with 3 replications. The first factor was the types of inoculum: commercial inoculum of tempe, Raprima (3%), a single inoculum of S. cerevisiae (3%), a single inoculum of R. oligosporus (3%), and mixed inoculum of 1.5% S. cerevisiae and 1.5% R. oligosporus. The second factor was the length of fermentation which consisted of 0, 8, 16, 24, 32 and 40 hours at room temperature. Regarding the number of fungi, yeasts and bacteria, the observational data were presented descriptively in the form of graphs, while for the data from the analysis of β-glucan and antioxidant activity, the data obtained were analyzed for variance with analysis of variance (ANOVA) and then analyzed further by the Least Significant Difference (LSD) at the 5% significance level. The results showed that the type of inoculum and duration of fermentation had an effect on increasing the growth of fungi, yeasts and bacteria, as well as increasing β-glucan content and the antioxidant activity of tempe. Yeast growth had a more dominant effect on increasing β-glucan content and antioxidant activity compared to fungi and bacteria. Tempe inoculated with a mixed inoculum of 1.5% R. oligosporus + 1.5% S. cerevisiae, resulted in the highest β-glucan content of 0.58% and the highest antioxidant activity at 82.42%. In conclusion, a mixed inoculum of 1.5% R. oligosporus + 1.5% S. cerevisiae with 36−40 hours of fermentation produced tempe with the highest β-glucan content and antioxidant activity. Therefore, the β-glucan content causes tempe to have better potential health benefits than tempe without the addition of S. cerevisiae.
The quality of tempe depends on the raw materials and starter culture or the type of inoculum used. In general, tempe is made using inoculum containing R. oligosporus. Besides R. oligosporus, other important microbes of the Rhizopus species involved in tempe fermentation are R. oryzae and R. stolonifer [1]. These three microorganisms ferment soybeans into tempe and R. oligosporus retains most of the nutrients in soybeans and increases protein digestibility in soybeans [2].
According to Kustyawati [3], yeast plays a role in tempe fermentation and is able to grow with R. oligosporus and indigenous bacteria during the tempe fermentation. The addition of Saccharomyces cerevisiae is expected to result in the content of β-glucan in tempe so that its potential for making tempe containing β-glucan needs to be explored in depth. However, the intentional addition of S. cerevisiae in tempe fermentation is predicted to affect the growth of other microorganisms, both fungi and bacteria during fermentation.
Tempe has a variety of nutrients needed by the human body. In addition, tempe also contains bioactive components that are beneficial to human health. Nurdini et al. [4] stated that the growth of fungi, yeasts and lactic acid bacteria during fermentation played a role in providing nutrients and bioactive components in tempe. Tempe has antibacterial and antioxidant compounds that are beneficial for health, namely genestein, daidzein, phytosterols and phytic acid [5]. Raw tempe extract is able to inhibit the growth of pathogenic bacteria such as Staphylococcus aureus [6], inhibit the growth of pathogens Escherichia coli, Salmonella typhimurium and Shigella flexneri [7]. The findings of Kuligowski et al. [8] showed that tempe extract can stimulate the growth of Lactobacillus and Bifidobacterium as normal microflora in the intestine.
Tempe with the addition of S. cerevisiae is predicted to contain β-glucan derived from S. cerevisiae, because the cell wall of S. cerevisiae is one of the most common sources of β-1, 3-glucan and β-1, 6-glucan production [9]. The addition of S. cerevisiae and the content of β-glucan in tempe is expected to improve the functional properties of tempe so that the health benefits of tempe are also increased. This is supported by the opinion of Hetland et al. [10], that β-glucan has various biological activities including as an anticholesterol and immune system enhancer.
Based on the results of previous studies, the addition of S. cerevisiae in tempe fermentation was proven to produce β-glucan content in tempe [11,12,13,14]. In their research, Rizal and Kustyawati [11] stated that the addition of S. cerevisiae up to 3% in tempe fermentation resulted in tempe with the highest β-glucan content (2.5%) and was organoleptically acceptable. Rizal et al. [14] added that tempe made with the addition of S. cerevisiae and R. oligosporus had a higher protein content (17.40%) than tempe without S. cerevisiae (17.11%). However, it is not known how big the contribution of S. cerevisiae in producing β-glucan in tempe is compared to R. oligosporus. The results of research by Rizal et al. [15] showed that R. oligosporus also had an effect on the formation of β-glucan in tempe. To find out, it is necessary to study the role of S. cerevisiae and R. oligosporus during the fermentation process of soybeans into tempe in producing β-glucan.
Tempe with the addition of yeast contains higher isoflavones than ordinary tempe [3]. The isoflavone content of tempe which is added with yeast consists of daidzein and genistein [16]. Isoflavones in tempe act as antioxidants, anticancer and antimicrobials [17]. Therefore, the addition of S. cerevisiae in the manufacture of tempe is predicted to increase its antioxidant and antimicrobial activity, so this also needs to be studied further. This research was aimed to study the effect of inoculum type and fermentation time on microbial growth patterns (yeasts, fungi and bacteria), β-glucan formation and antioxidant activity during soybean fermentation into tempe.
The starters used in this study included commercial tempe inoculum (Raprima brand), pure cultures of S. cerevisiae and R. oligosporus. Pure cultures include Rhizopus oligosporus FNCC (Food and Nutrition Culture Collection) 6010 and Saccharomyces cerevisiae FNCC (Food and Nutrition Culture Collection) 3012 were obtained from the Inter-University Center for Food and Nutrition, Gadjah Mada University, Indonesia. Soybeans were obtained from tempe craftsmen in Gunung Sulah Bandar Lampung. The study was conducted using Completely Randomized Block Design with 3 replications. The first treatment was the types of inoculum: 3% inoculum of commercial tempe Raprima brand (S1), 3% single inoculum of S. cerevisiae (S2), 3% single inoculum of R. oligosporus (S3), and mixed inoculum of S. cerevisiae and R. oligosporus (1.5%:1.5%) (S4). The second treatment was fermentation time which consisted of 0 (L1), 8 (L2), 16 (L3), 24 (L4), 32 (L5) and 40 (L6) hours at room temperature. Observations were made every 8 hours covering the number of fungi, yeasts and bacteria. The data obtained from the analysis of the number of microbes is then presented descriptively in the form of graphs. Meanwhile, the data from the analysis of β-glucan content and antioxidant activity were analyzed for variance with analysis of variance (ANOVA) and analyzed further with the Least Significant Difference (LSD) at a significant level of 5%.
Malt Extract Agar (MEA) as much as 5 g was dissolved in 100 mL of distilled water, mixed evenly by stirring while heated on a hotplate until the media was completely mixed, then sterilized at 121 ℃ for 15 minutes. The media was allowed to stand for a while, put into a 20 mL sterile dish and allowed to stand until the media was solid. The isolates of S. cerevisiae were taken by ose and then cultured into the solid MEA medium by the scratch method, then put into the incubator, left for 24−48 hours at 28−30 ℃ so that S. cerevisiae colonies were obtained on the surface of the MEA media. The colonies formed were then harvested by adding 10 mL of sterile distilled water and harvested using drygalski stems. The suspension of S. cerevisiae was put into a 50 mL centrifuge tube, centrifuged for 10 minutes at 3000 rpm for pure culture and separated from the supernatant. The supernatant was then discarded to obtain pure culture pellets of S. cerevisiae. To determine the number of S. cerevisiae cells, the haemacytometer was counted and adjustments were made to obtain the number of S. cerevisiae 107 cells/mL.
Potato Dextrose Agar (PDA) media as much as 3.9 g was added with 100 mL of distilled water and then mixed evenly by stirring while heated on a hotplate until the media was completely mixed. Next, the media was sterilized at 121 ℃ for 15 minutes. After that, the media was put into a 20 mL sterile dish, then allowed to stand until the media became solid. Pure isolates of Rhyzopus oligosporus were taken using a sterile ose needle and inoculated into PDA media, then spread over the entire surface of the media by the scratch method. Furthermore, the media containing the fungi was put into an incubator and then incubated at 28−30 ℃ for a week until pure R. oligosporus colonies were found on the surface of the media. Colonies of R. oligosporus formed were then harvested using drygalski stems by pouring 10 mL of sterile distilled water into the media. After that, R. oligosporus spores were centrifuged at 3000 rpm for 10 minutes to obtain supernatants and microbial pellets. The supernatant obtained was then discarded to obtain a pellet of R. oligosporus culture. The number of R. oligosporus cells was then counted using a haemacytometer and adjusted to obtain the number of R. oligosporus as much as 107 spores/mL.
Initially 400 g of soybeans were soaked overnight in clean water at room temperature, then the husks were removed. Soybeans are then boiled in water with a ratio of soybeans and water is 1:3, within 30 minutes, drained and left until the soybean temperature reaches room temperature. The next step is fermentation, which is done by mixing every 100 g of boiled soybeans with inoculum according to treatment (3% Raprima, 3% S. cerevisiae, 3% R. oligosporus and mixed inoculum 1.5% S. cerevisiae + 1.5% R oligosporus) with an inoculum concentration of 107−108 cells/mL according to the type of inoculum that had been prepared previously. After being stirred evenly, the soybean seeds were put into a perforated plastic packaging so that there was aeration, then incubated (fermented) with the appropriate fermentation time at room temperature. Tempe is then observed every 8 hours.
The measurement results of β-glucan levels in tempeh are expressed in terms of glucose equivalent glucan levels, so glucose is used as the standard for measuring β-glucan. A total of 3 g of tempe samples were added with 30 mL of NaOH 0.7 M, then hydrolyzed for 6 hours at a temperature of 75 ℃ to obtain a cloudy solution, then centrifuged at 10,000 rpm for 30 minutes at 25 ℃. The resulting supernatant was then discarded, the resulting residue was then washed with 30 mL 0.5 M acetic acid solution and then centrifuged again at 10,000 rpm for 30 minutes at 25 ℃. The resulting supernatant was discarded. Washing using acetic acid was repeated 3 times. The resulting residue was washed with 20 mL of distilled water and then centrifuged again at 5000 rpm for 10 minutes. This is followed by washing with distilled water again up to two times. The resulting residue was added with 20 mL of ethanol, then centrifuged at 5000 rpm for 10 minutes to produce crude β-glucan. The biomass was heated in an oven for 1 day at 45 ℃ and then weighed as dry weight of β-glucan. The dry residue was added with 4 mL of 1 M NaOH then allowed to stand for 1 hour. Then, 2 mL of Pb-Acetate was added and allowed to stand for about 30 minutes. The solution was then added with 1 g of sodium oxalate until a clear solution was obtained. Then 2 mL of the solution was taken and 0.5 mL of 5% phenol and 2.5 mL of 5 M sulfuric acid were added and then measured with a UV-Vis spectrophotometer at a wavelength (λ) of 490 nm. The standard solution used was a standard solution of glucose in several concentrations which was added with 0.5 mL of 5% phenol and 2.5 mL of 5 M sulfuric acid in a test tube, shaken homogeneously, allowed to stand for 10 minutes, then heated for 15 minutes at 100 ℃. Absorption each concentration of glucose standard solution was measured by spectrophotometer at a wavelength of 490 nm. The blank solution was 0.5 mL of distilled water mixed with 0.5 mL of 5% phenol and 2.5 mL of 5 M sulfuric acid.
The calculation of the number of bacteria, fungi and yeasts in tempe samples was carried out using the TPC (Total Plate Count) method. Analysis of total bacteria, total yeast and total fungi was carried out every 8 hours during fermentation from 0−48 hours. Samples of each tempe were taken and serial dilutions were made up to 10-8 in duplicate. A total of 1 mL of the last three dilution levels of 1 mL each was poured into a petri dish containing the appropriate media (MEA medium for yeast, PDA for fungi and Nutrient Agar medium Merck for bacteria) and then incubated for 24−48 hours at 37 ℃ to grow bacteria and for 3−5 days at 28−30 ℃ to grow fungi and yeasts.
The antioxidant activity of tempe samples was tested based on the procedure of Ismail et al. [19]. A total of 0.5 mL of tempe extract sample was given 2 mL of 0.2 mM DPPH solution. The blank solution was prepared in the following way: 0.2 mM DPPH solution was taken with a pipette of 2 mL and then put into a test tube and added 0.5 mL of ethanol. Samples were incubated for 30 minutes in a dark room without light. The absorbance of DPPH was measured using a UV-Vis spectrophotometer at 517 nm. Antioxidant activity was measured as a decrease in the absorption of the DPPH solution caused by the addition of the sample solution. The value of DPPH absorption before and after the addition of the sample solution, expressed as a percent of antioxidant activity with the formula:
(1) |
Note: A sample = Absorbance sample; A control = Absorbance does not contain sample.
The growth of fungi, yeasts and bacteria during the fermentation of boiled soybeans into tempe with the addition of various types of inoculum according to the treatment is presented in Figures 1−4. Figure 1 shows the growth of yeast, fungi and bacteria during the fermentation of soybean inoculated with Raprima inoculum. During tempe fermentation with Raprima inoculum, bacterial growth was the most dominant, followed by fungi, while yeast growth was the lowest. The presence of yeast growth during tempe fermentation with Raprima inoculum indicated that in the manufacture of tempe using tempe inoculum commonly used by tempe producers, there was growth and involvement of other microbes other than R. oligosporus as the main microbe. The presence of yeast and bacteria, especially lactic acid bacteria (LAB), in tempe has been reported by a number of researchers. Roubous and Nout [2] stated that both types of microbe were found in tempe.
This result is in line with the findings of Kustyawati [3] which showed that during the fermentation of tempe with commercial tempe inoculum (Raprima), bacteria grew most dominantly followed by fungi. However, in Kustyawati's study [3], yeast did not grow during soybean fermentation inoculated by commercial tempe inoculum. The presence of bacteria is predicted to come from the fermentation environment or from within the tempe inoculum itself, namely bacteria that contribute to tempe fermentation such as lactic acid bacteria and spore-forming bacteria such as Bacillus substilis. In the study by Moreno et al. [20], lactic acid bacteria were found during the production of tempe in the home industry in Malaysia, both in the raw material, at the stage of soaking soybeans, boiling soybeans and in fresh tempe. Andayani et al. [21] even found 25 microbial isolates from chocolate sorghum tempe, of which there were lactic acid bacteria such as Lactococcus sp., Streptococcus sp. and yeast genus Saccharomyces sp. The presence of yeast in the process of tempe fermentation made with Raprima inoculum is also predicted to come from the tempe inoculum itself, so that the amount of yeast is less than the amount of fungi. Feng et al. [22] reported many yeast species growing on barley tempe. The role of yeast during tempe fermentation is generally related to its contribution to the interaction between microorganisms, changes in texture and biosynthesis of flavor components.
Figure 2 shows microbial growth during soybean fermentation inoculated with S. cerevisiae as the sole inoculum. During the fermentation of soybeans inoculated with a single culture of S. cerevisiae, bacteria still grew dominantly than yeast, but there was no growth of fungi during fermentation. The growth of bacteria during fermentation is predicted to come from the fermentation environment itself, during soybean soaking, boiling soybeans and the fermentation process, as reported by Moreno et al. [19]. This is supported by the results of research by Kustyawati [3] which showed that in all tempeh with various types of inoculum, bacteria always grew dominantly. Meanwhile, R. oligosporus in tempe is not a contaminant microorganism but must be added intentionally to soybeans, so that it cannot grow in fermented tempe which is only given a single inoculum of S. cerevisiae. Although it was possible that there was growth of R. oligosporus during fermentation, it was suspected that its growth was unable to compete with S. cerevisiae which was intentionally added. Because there was no fungi growth during soybean fermentation which was only inoculated with a single inoculum of S. cerevisiae, the formation of tempe did not occur. According to Handoyo and Morita [23], it is possible that R. oligosporus plays a role in supporting the growth of other microorganisms in soybean fermentation, because during fermentation, R. oligosporus produces several enzymes such as lipase, protease and phytase which hydrolyze carbohydrates, lipids and proteins in soybeans to produce amino acids and amino acids which are further utilized by bacteria and yeasts.
On the other hand, in soybean fermentation using only R. oligosporus as the sole inoculum (Figure 3), there was no yeast growth, while fungi could grow well and produce tempe formation, although bacterial growth was still more dominant. This indicates that yeast is not predicted to be present in the fermentation environment. Even if there is yeast growth during fermentation, the amount of yeast is thought to be very small so that it cannot grow and compete with fungi growth. This result is in accordance with the results of Kustyawati's study [3] which showed that there was a dominant growth by bacteria, while yeast did not grow during tempe fermentation which was inoculated by a single inoculum of R. oligosporus.
In making tempe using a combination of R. oligosporus and S. cerevisiae inoculum, fungi and yeast side by side were able to grow and reproduce well during fermentation (Figure 4). In Figure 4, it can be seen that bacteria still grew dominantly compared to fungi and yeasts, while yeast had more dominant growth compared to fungi. This result has similarities with the results of Kustyawati [3] which showed the growth of similar types of microbes during tempe fermentation inoculated by R. oligosporus together with several types of yeast. During the tempe fermentation, the inoculum of R. oligosporus with yeast, both S. boulardii and Y. Lipolytica, the growth of bacteria was dominant, followed by yeast and finally by fungi. This is different from the growth of fungi and yeast in soybean fermentation using Raprima inoculum, where the growth of fungi is more dominant than yeast (Figure 1). Yeast growth was higher than fungi in tempe fermentation with mixed inoculum due to the addition of S. cerevisiae inoculum intentionally into soybean fermentation with R. oligosporus as the main microbe for tempe formation. Meanwhile, in tempe made with Raprima inoculum, the presence of yeast was predicted only from the Raprima inoculum itself. This indicated that the addition of S. cerevisiae to tempe fermentation did not interfere with the growth of fungi in the formation of hyphal threads that could glue soybean seeds to form tempe. Yeast can grow side by side with fungi and is thought to have a beneficial effect on the tempe produced.
The presence of bacteria and yeast and their role in tempe fermentation has been discovered by several previous researchers. Barus et al. [24] showed the presence of several bacteria in soybean soaking water and in fresh tempe which have an important role in tempe fermentation. According to Barus et al. [24], in addition to R. oligosporus, the presence of bacteria has an important role in the manufacture of tempe, because some of them play a role in improving the quality of tempe. For example, Klebsiella pneumoniae contributes to the production of vitamin B12 in tempe [25], Micrococcus or Arthrobacter has a role in the formation of isoflavones [26]. The findings of Barus et al. [24] showed the presence of several types of bacteria in fresh soybean and tempe soaking water, including Bacillus subtilis at 3.1×106 CFU/g, Klebsiella pneumoniae at 3.7×107 CFU/g and Flavobacterium sp. a total of 5.9×108 CFU/g, with the total bacteria reaching 9.4×108 CFU/g in fresh tempe. Based on Figures 1−3, for all types of inoculum inoculated on boiled soybeans, the growth patterns of fungi during tempe fermentation were similar. The fungi undergo an adaptation phase with an incubation period of 8 hours to 16 hours. Fungi growth began to be significant after incubation of more than 16 hours and growth began to slightly slop after 24 hours of incubation, and finally reached constant growth at 32 hours of incubation with the number of fungi ranging from 106–108 CFU/g until the end of fermentation at 40 hours. The growth of fungi in this study was also similar to the growth of fungi in Kustyawati's study [3] which showed that fungi underwent adaptation to 12 hours of incubation, then experienced significant growth after 12 hours of incubation until they passed the 24-hour incubation period and peaked at 36 hours of incubation, with the highest number of fungi reached 106 CFU/g.
The results of the study on the effect of adding several types of inoculum and fermentation time on the content of β-glucan in tempe are presented in Figures 5−7. Figure 5 shows that the average amount of β-glucan increased during the tempe fermentation process in all of inoculum types. The β-glucan content in soybean (0 hours fermentation) was 0.17%, then it started to increase at 8 and 16 hours of fermentation, and increased sharply at 24 to 40 hours of fermentation. This is understandable because the amount of β-glucan is influenced by the number of microbes, especially the amount of yeast, during fermentation. Because β-glucan is found in yeast cell walls, the more the amount of yeast, the greater the amount of β-glucan in the fermentation substrate. Significant growth of fungi and yeasts occurred after 16 to 24 hours of fermentation and after that microbial growth occurred constantly up to 40 hours of fermentation, as shown in Figures 1 and 4.
β-glucan is mainly found in yeast cell walls, but can also be obtained from fungi and bacteria. Therefore, increasing the number of microbes, especially yeast, can increase the amount of β-glucan. According to Sudiana et al. [27], β-glucan can be produced from various sources such as cereals, algae, bacteria, fungi, and nowadays it is often produced from the yeast S. cerevisiae. Saccharomyces cerevisiae is known as a producer of β-glucan. Saccharomyces cerevisiae has a cell wall structure that contains glucose and functions as a glycoprotein [28,29]. The cell wall structure of S. cerevisiae consists mostly of glucan polysaccharides with β-1, 3-glucan and β-1, 6-glucan bonds [30]. Another component is a mannoprotein located on the outer cell wall and which binds to β-(1, 6)-glucan [31]. The dry weight of the cell wall of S. cerevisiae is about 10%−25% of the total cell biomass, where the dry weight of the cell wall contains 35%−40% mannoprotein, 5%−10% β-1, 6 glucan, more than half or 50%−55% consists of β-1, 3 glucans, 2%−14% lipids and 1%−2% chitin [32]. The type of β-glucan in the cell wall of S. cerevisiae is β-(1, 3) with β-(1, 6)-glucan branch. The β-(1, 3)-glucan and chitin complexes are the main constituents of the inner cell wall, β-(1, 6)-glucan connects the inner and outer cell wall components bound to mannoproteins on the outer surface of the cell wall [33].
Figure 6 shows the effect of the types of inoculum on the β-glucan content of tempe. The results showed that the type of inoculum significantly affected the β-glucan content of tempe. The lowest levels of glucans were found in tempe inoculated with a single inoculum of R. oligosporus, which was 0.17% (S3), while the highest levels of glucans were found in tempe inoculated with a mixture of inoculums of R. oligosporus and S. cerevisiae which reached 0.41% (S4). The difference in the amount of β-glucan in tempe made with different types of inoculum is closely related to the number of microbes in each tempe. Based on the results of the calculation of the number of microbes in each tempe with different types of inoculum as shown in Figures 1−4, it can be concluded that S. cerevisiae is the most important microbe in the formation of β-glucan in tempe.
Figure 7 shows the changes in β-glucan levels during tempe fermentation in various types of inoculum. The highest amount of β-glucan was found in soybeans fermented with a single inoculum of S. cerevisiae, but tempe was not formed because R. oligosporus as a tempe-forming agent was not available. Because the main goal in this study was the formation of tempe containing β-glucan, soybean fermentation by single inoculum S. cerevisiae was not used as the best treatment even though the amount of β-glucan produced was the highest (0.66%). The highest amount of β-glucan which was chosen as the best treatment was tempe which was made with the addition of a mixed inoculum of R. oligiosporus and S. cerevisiae with a fermentation time of 40 hours (S4L6), which was 0.58%.
The increase in the amount of β-glucan in tempe during fermentation inoculated with mixed inoculum of R. oligosporus and S. cerevisiae was influenced by the amount of fungi and yeast in each tempe. The β-glucan content of tempe shows the potential for health benefits of tempe compared to tempe without the addition of S. cerevisiae. This is supported by Andriani [34] who showed that the administration of β-glucan extract was able to significantly suppress the blood cholesterol levels of rats. Andriani's study [34] concluded that β-glucan extract has a function as a cholesterol-lowering drug. Nicolasi [35] previously also reported that plasma cholesterol levels can be reduced by about 8%−26% by β-glucan in obese hypercholesterolemic patients. According to Ferdiansyah [36], β-glucan acts as an antihypercholesterolemic through the mechanism of reducing cholesterol absorption in the intestine. Therefore, tempe produced with the addition of S. cerevisiae has better potential health benefits than tempe without the addition of S. cerevisiae due to the presence of beta glucan in the tempe.
Based on the results of the analysis of variance, it is known that the antioxidant activity of tempe is influenced by the type of inoculum and the fermentation time of tempe. The effect of inoculum type and fermentation time on the antioxidant activity of tempe is presented in Figures 8−10. Further tests using BNT showed that the antioxidant activity increased with increasing fermentation time (Figure 8). The highest antioxidant activity was found in tempe after 40 hours of fermentation, which was 73.14%. The antioxidant activity of tempe is caused by the content of isoflavones in soybeans and tempe. The results of Banobe et al. [37] showed that soybean tempe had antioxidant activity of 76.10% with an isoflavone content of 0.33%. The content of isoflavones in soy tempe comes from the soybean seeds themselves.
Figure 8 shows an increase in antioxidant activity during the fermentation of soybeans into tempe. The antioxidant activity of tempe at fermentation times of 24, 32 and 40 hours, respectively, was 61.51%, 69.09% and 73.143%. This is due to an increase in the percentage of free radical inhibition (DPPH) during fermentation. That is, the antioxidants in tempe at each fermentation time were able to capture free radicals (DPPH) of 61.51%, 69.09% and 73.14%. These results are in line with the research of Widoyo et al. [38] which showed an increase in the antioxidant activity of soy tempe along with the increase in fermentation time. The antioxidant activity of soy tempe research by Widoyo et al. [38] for 30, 42 and 54 hours respectively were 36.35%, 39.03% and 42.12%. The antioxidant activity of edamame tempe also increased after fermentation for 1, 2 and 3 days with antioxidant activity of 95%, 97%, 100%, respectively, while the antioxidant activity of unfermented edemame soybean was 24% [39]. Research conducted by Maryati et al. [40] also showed an increase in the antioxidant activity of the functional drink of dragon fruit along with the increase in the duration of fermentation.
The results of the analysis also showed that the type of inoculum affected the antioxidant activity of tempe (Figure 9). Figure 9 shows that the highest antioxidant activity was found in tempe inoculated with a mixed inoculum of R. oligosporus and S. cerevisiae (S4), which was 62.05%, followed by a single inoculum of S. cerevisiae (S2) of 54.53%, tempe with the inoculum Raprima (S1) of 48.62%, then tempe with a single inoculum of R. oligosporus (S3) with antioxidant activity of 45.82%.
The results showed that the highest antioxidant activity was found in tempe produced with mixed inoculum of R. oligosporus and S. cerevisiae with 40 hours of fermentation (S4L6) (Figure 10). This is in accordance with the results of the calculation of the number of microbes in tempe with various types of inoculum used. Tempe produced by cultures of R. oligosporus and S. cerevisiae showed the highest number of yeasts and fungi, namely fungi reaching 107 CFU/g, while yeasts reaching 108 CFU/g. The increase in antioxidant activity was influenced by the amount of fungi and yeast which increased with the increase in tempe fermentation time. The increase in the number of fungi affects the density of spores in tempe, while according to Mojsov [41], the density of spores affects the increase in cell biomass which in turn affects the activity of the enzymes it produces. Thus, the increasing number of R. oligosporus cells in tempe caused the activity of the β-glucosidase enzyme produced to also increase. This β-glucosidase enzyme will catalyze the hydrolysis reaction of isoflavone glycosides into aglycones, an insoflavone compound which is active as an antioxidant. Thus, it can be understood that the mixed inoculum of R. oligosporus and S. cerevisiae with a fermentation time of 40 hours (S4L6) produced the highest antioxidant activity, which was 84.42%.
The types of inoculum and duration of fermentation had an effect on increasing the growth of fungi, yeasts and bacteria, as well as increasing the antioxidant activity of tempe. Yeast growth had a more dominant effect on increasing β-glucan content and antioxidant activity compared to fungi and bacteria. Tempe inoculated with a mixed inoculum of 1.5% R. oligosporus + 1.5% S. cerevisiae resulted in the highest β-glucan content of 0.58% and the highest antioxidant at 82.42%. To produce tempe with high β-glucan content, it is recommended to use a mixed inoculum of 1.5% R. oligosporus + 1.5% S. cerevisiae with a fermentation time of 36−40 hours. These results indicate that tempe made with a mixture of S. cerevisiae and R. oligosporus has the potential to become tempe which has better health benefits than tempe in general, due to the high β-glucan content.
This research was partly funded by the University of Lampung through the BLU-Fundamental Research, University of Lampung, 2020.
The authors declare no conflict of interest.
[1] |
Bintari SH, Anisa DP, Veronika EJ, et al. (2008) Effect inoculation of Micrococcus luteus to growth of mold and content isoflavone at tempe processing. Biosaintifika: J Bio Bio Edu 1: 1–8. https://doi.org/10.15294/biosaintifika.v1i1.43 doi: 10.15294/biosaintifika.v1i1.43
![]() |
[2] | Roubous HPJ, Nout MJR (2011) Anti-diarrhoeal aspect of fermented soya beans, In: El-Shemy H. (Eds), Soybean and Health, London: Intech Open, 383–406. |
[3] |
Kustyawati ME (2009) Study on the role of yeast in tempe production. Agritech 29: 64–70. https://doi.org/10.22146/agritech.9765 doi: 10.22146/agritech.9765
![]() |
[4] | Nurdini AL, Nuraida L, Suwanto A, et al. (2015) Microbial growth dynamics during tempe fermentation in two different home industries. Food Res Int 22: 1668–1674. |
[5] | Astawan M, Wresdiyati T, Widowati S, et al. (2013) Phsyco-chemical characteristics and functional properties of tempeh made from different soybeans varieties. Pangan 22: 241–252. |
[6] |
Mambang DEP, Rosidah, Suryanto D (2014) Antibacterial activity of tempe extracts on Bacillus subtilis and Staphylococcus aureus. J Teknol dan Industri Pangan 25: 115–118. https://doi.org/10.6066/jtip.2014.25.1.115 doi: 10.6066/jtip.2014.25.1.115
![]() |
[7] |
Virgianti DP (2015) Uji antagonis jamur tempe (Rhizopus sp.) terhadap bakteri patogen enterik. Majalah Ilmiah Biologi Biosfera: A Sci J 32: 162–168. https://doi.org/10.20884/1.mib.2015.32.3.339 doi: 10.20884/1.mib.2015.32.3.339
![]() |
[8] |
Kuligowski M, Kuligowska IJ, Nowak J (2013) Evaluation of bean and soy tempeh influence on intestinal bacteria and estimation of antibacterial properties of bean tempeh. Pol J Microbiol 62: 189–194. https://doi.org/10.33073/pjm-2013-024 doi: 10.33073/pjm-2013-024
![]() |
[9] |
Pengkumsri N, Sivamaruthi BS, Sirilun S, et al. (2017) Extraction of β-glukan from Saccharomyces cerevisiae: Comparison of different extraction methods and in vivo assessment of immunomodulatory effect in mice. Food Sci Tech 37: 124–130. https://doi.org/10.1590/1678-457X.10716 doi: 10.1590/1678-457X.10716
![]() |
[10] | Hetland G, Johnson E, Eide DM, et al. (2013) Antimicrobial effects of β-glucans and pectin and of the Agaricus blazei-based mushroom extract, AndoSanTM. Examples of mouse models for pneumococcal, fecal bacterial, and mycobacterial infections. Microb Pathog Strategies Combating Them: Sci, Technol Edu 1: 889–898. |
[11] |
Rizal S, Kustyawati ME (2019) Characteristics of sensory and Beta-glucan content of soybean tempe with addition of Saccharomyces cerevisiae. J Teknol Pertanian 20: 127–138. https://doi.org/10.21776/ub.jtp.2019.020.02.6 doi: 10.21776/ub.jtp.2019.020.02.6
![]() |
[12] |
Rizal S, Kustyawati ME, Murhadi, et al. (2020) Growth optimization of Saccharomyces cerevisiae and Rhizopus oligosporus during fermentation to produce tempe with high β-glucan content. Biodiversitas J Biol Diversity 21: 2667–2673. https://doi.org/10.13057/biodiv/d210639 doi: 10.13057/biodiv/d210639
![]() |
[13] |
Rizal S, Kustyawati ME, Murhadi, et al. (2021) The growth of yeast and fungi, the formation of β-glucan, and the antibacterial activities during soybean fermentation in producing tempe. Int J Food Sci. 6: 1–8. https://doi.org/10.1155/2021/6676042 doi: 10.1155/2021/6676042
![]() |
[14] |
Rizal S, Kustyawati ME, Suharyono, et al. (2022) Changes of nutritional composition of tempeh during fermentation with the addition of Saccharomyces cerevisiae. Biodiversitas J Biol Diversity 23: 1553–1559. https://doi.org/10.13057/biodiv/d230345 doi: 10.13057/biodiv/d230345
![]() |
[15] | Rizal S, Kustyawati ME, Murhadi, et al. (2018) Pengaruh konsentrasi Saccharomyces cerevisiae pada kandungan abu, protein, lemak dan β-glukan tempe. Prosiding Seminar Nasional Fakultas Pertanian UNS 2: 96–103. |
[16] |
Kustyawati ME, Subeki, Murhadi, et al. (2020) Vitamin B12 production in soybean fermentation for tempeh. AIMS Agric Food 5: 262–271. https://doi.org/10.3934/agrfood.2020.2.262 doi: 10.3934/agrfood.2020.2.262
![]() |
[17] |
Bavia ACF, Silva CE, Ferreira MP, et al. (2012) Chemical composition of tempeh from soybean cultivars specially developed for human consumption. Food Sci Tech 32: 613–620. https://doi.org/10.1590/S0101-20612012005000085 doi: 10.1590/S0101-20612012005000085
![]() |
[18] |
Kusmiati, Tamat SR, Jusuf E, et al. (2007) Beta-glucan production from two strains of Agrobacterium sp. in medium containing of molases and uracil combine. Biodiversivitas 8: 253–256. https://doi.org/10.13057/biodiv/d080210 doi: 10.13057/biodiv/d080210
![]() |
[19] |
Ismail J, Runtuwene MRJ, Fatimah F (2012) Total phenolic compounds and antioxidant activity of the seed and skin of pinang yaki (areca vestiaria giseke) fruits (Areca vetiaria Giseke). J Ilmiah Sains 12: 84–85. https://doi.org/10.35799/jis.12.2.2012.557 doi: 10.35799/jis.12.2.2012.557
![]() |
[20] |
Moreno MRF, Leisner JJ, Tee LK, et al. (2002) Microbial analysis of Malaysian tempeh, and characterization of two bacteriocins produced by isolates of Enterococcus faecium. J Appl Microbiol 92: 147–157. https://doi.org/10.1046/j.1365-2672.2002.01509.x doi: 10.1046/j.1365-2672.2002.01509.x
![]() |
[21] | Andayani P, Wardani AK, Murtini ES (2008) Isolation and identification of microorganism in sorghum tempeh (sorghum bicolor) and its potency for degrading starch and protein. J Teknol Pertanian 9: 95–105. |
[22] |
Feng XM, Larsen TO, Schnürer J (2007) Production of volatile compounds by Rhizopus oligosporus during soybean and barley tempeh fermentation. Int J Food Microbiol 113: 133–141. https://doi.org/10.1016/j.ijfoodmicro.2006.06.025 doi: 10.1016/j.ijfoodmicro.2006.06.025
![]() |
[23] |
Handoyo T, Morita N (2006) Structural and functional properties of fermented soybean (tempe) by using Rhizopus oligosporus. Int J Food Prop 9: 347–355. https://doi.org/10.1080/10942910500224746 doi: 10.1080/10942910500224746
![]() |
[24] |
Barus T, Suwanto A, Wahyudi A., et al. (2008) Role of bacteria in tempe bitter taste formation: Microbiological and molecular biological analysis based on 16S rRNA gene. Microbiol Indones 2: 17–21. https://doi.org/10.5454/mi.2.1.4 doi: 10.5454/mi.2.1.4
![]() |
[25] |
Keuth S, Bisping B (1994) Vitamin B12 production by Citrobacter freundiior and Klebsiella pneumoniae during tempe fermentation and proof of enterotoxin absence by PCR. Appl Environ Microb 60: 1495–1499. https://doi.org/10.1128/aem.60.5.1495-1499.1994 doi: 10.1128/aem.60.5.1495-1499.1994
![]() |
[26] |
Klus K, Borger-Papendorf G, Barz W (1993) Formation of 6, 7, 4'-trihydroxyisoflavone (factor 2) from soybean seed isoflavones by bacteria isolated from tempe. Phytochemistry 34: 979–981. https://doi.org/10.1016/s0031-9422(00)90697-6 doi: 10.1016/s0031-9422(00)90697-6
![]() |
[27] |
Sudiana ADG, Kuswendi H, Dewi VYK, et al. (2021) The potential of β-glucan from Saccharomyces cerevisiae cell wall as anticholesterol. J Anim Health Prod 9: 72–77. http://dx.doi.org/10.17582/journal.jahp/2021/9.1.72.77 doi: 10.17582/journal.jahp/2021/9.1.72.77
![]() |
[28] |
Siwicki AK, Patrycja S, Stanisl£ aw R, et al. (2015) Influence of β-glukan Leiber®Βeta-S on selected innate immunity parameters of European eel (Anguilla anguilla) in an intensive farming system. Cen Eur J Immunol 40: 5–10. https://doi.org/10.5114/ceji.2015.50826 doi: 10.5114/ceji.2015.50826
![]() |
[29] |
Febriyanti AE, Sari CN (2016) Effectiveness of growth media commercial yeast (Saccharomyces cerevisiae) for bioethanol fermentation from water Hyacinth (Eicchornia crassipes). Bioma 12: 43–48. https://doi.org/10.21009/Bioma12(2).6 doi: 10.21009/Bioma12(2).6
![]() |
[30] |
Zeković DB, Kwiatkowski S, Vrvić MM, et al. (2005) Natural and modified (1→3)-β-D glucans in health promotion and disease alleviation. Crit Rev Biotechnol 25: 205–230. https://doi.org/10.1080/07388550500376166 doi: 10.1080/07388550500376166
![]() |
[31] |
Kapteyn JC, Van Den Ende H, Klis FM (1999) The contribution of the O-glycosylated protein Pir2p/Hsp150 to the construction of the yeast cell wall in wild-type cells and β1, 6-glucan-deficient mutants. Mol Microbiol 31: 1835–1844. https://doi.org/10.1046/j.1365-2958.1999.01320.x doi: 10.1046/j.1365-2958.1999.01320.x
![]() |
[32] |
Appeldoorn NG, Sergeeva L, Vreugdenhil D, et al. (2002) In situ analysis of enzymes involved in sucrose to hexose-phosphate conversion during stolon-to-tuber transition of potato. Physiol Plantarum 115: 303–310. https://doi.org/10.1034/j.1399-3054.2002.1150218.x doi: 10.1034/j.1399-3054.2002.1150218.x
![]() |
[33] | Bacic A, Fincher GB, Stone BA (2009) Chemistry, biochemistry, and biology of 1-3 beta glucans and related polysaccharides, Burlington: Academic Press. |
[34] | Andriani Y (2007) Uji aktivitas antioksidan ekstrak β-glukan dari Saccharomyces cerevisiae. J Gradien 3: 226–230 |
[35] |
Nicolasi R (1999) Plasmalipid changes after supplementation with β-glucan fiber from yeast. Am J Clin Nutr 70: 208–212. https://doi.org/10.1093/ajcn.70.2.208 doi: 10.1093/ajcn.70.2.208
![]() |
[36] |
Ferdiansyah MK (2018) Lipid effect of dietary fiber of barley grains on lipid metabolism. J Ilmu Pangan dan Hasil Pertanian 2: 72–81. https://doi.org/10.26877/jiphp.v2i1.2441 doi: 10.26877/jiphp.v2i1.2441
![]() |
[37] |
Banobe CO, Kusumawati IGAW, Wiradnyani NK (2019) Value of macro nutrients and antioxidant activities soybean (glycine max l.) combination of winged bean (Psophocarpus Tetragonolobus). Pro Food 5: 486–495. https://doi.org/10.29303/profood.v5i2.111 doi: 10.29303/profood.v5i2.111
![]() |
[38] |
Widoyo S, Handajani S, Nandariyah (2015) The effect of fermentation time to crude fiber contents and antioxidant activities in several soybean varieties of tempeh. Biofarmasi 13: 59–65. https://doi.org/10.13057/biofar/f130203 doi: 10.13057/biofar/f130203
![]() |
[39] |
Ningsih TE, Siswanto, Winarsa R (2018) Activity of antoxidants edamame soybeans fermented result of mixed culture by Rhizopus oligosporus and Bacillus subtilis. Berkala Sainstek 6: 17–21. https://doi.org/10.19184/bst.v6i1.7556 doi: 10.19184/bst.v6i1.7556
![]() |
[40] |
Maryati Y, Susilowati A, Artanti N, et al. (2020) Effect of fermentation on antioxidant activities and betacyanin content of functional beverages from dragon fruit and beetroot. JBBI 7: 48–58. https://doi.org/10.29122/jbbi.v7i1.3732 doi: 10.29122/jbbi.v7i1.3732
![]() |
[41] |
Mojsov K (2010) Effects of different carbon sources on the synthesis of pectinase by Aspergillus niger in submerged and solid state fermentations. Appl Microbiol Biotechnol 39: 36–41. DOI:10.1007/BF00166845 doi: 10.1007/BF00166845
![]() |
1. | Samsul Rizal, Maria Erna Kustyawati, Theresia Santika Kusuma Putri, Teguh Endaryanto, Effect of substrate type and incubation time on the microbial viability of instant starter for premium tempeh, 2023, 8, 2471-2086, 461, 10.3934/agrfood.2023024 | |
2. | Samsul Rizal, Maria Erna Kustyawati, Defina Zulfa, Firda Rosida, Fairuzsita Naura Amalia Syifani, Ayu Dian Pratiwi Permatahati, Chemical properties, sensory characteristics, and antibacterial activity to Staphylococcus aureus of tempeh gembus fermented with Mosaccha inocula, 2024, 95, 14668564, 103742, 10.1016/j.ifset.2024.103742 | |
3. | Samsul Rizal, Maria Erna Kustyawati, Dewi Sartika, Rachma Sasriany, Rahmat Hidayat, Atris Suyantohadi, Innovation of tempeh from jack bean (Canavalia ensiformis) fermented with Mosaccha inoculum, 2024, 62, 22124292, 105564, 10.1016/j.fbio.2024.105564 |