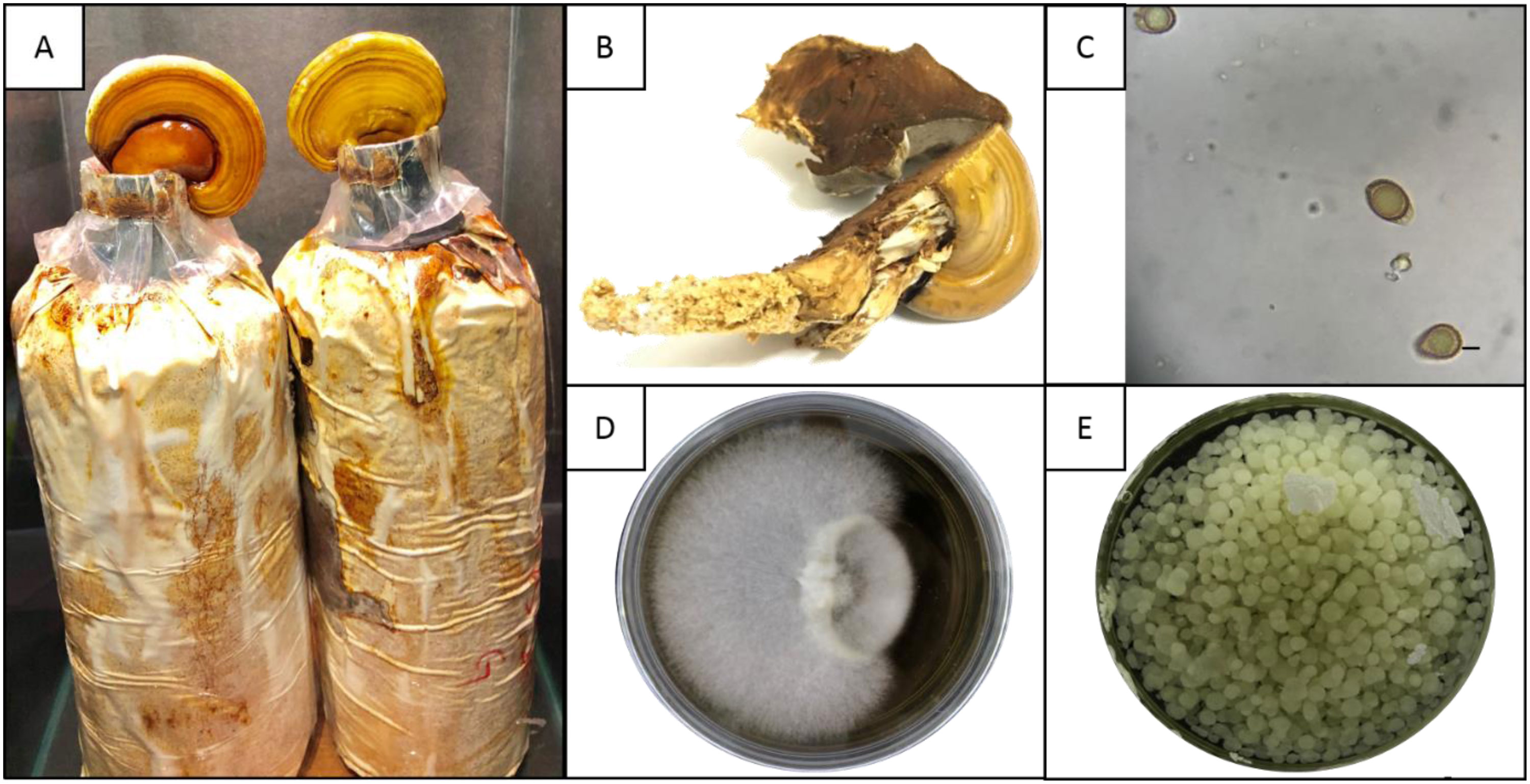
This paper presents an integrated approach combining a sequential neural network (SNN) with model predictive control (MPC) to enhance the performance of a permanent magnet synchronous motor (PMSM). We address the challenges of traditional control methods that struggle with the dynamics and nonlinear nature of PMSMs, offering a solution that leverages the predictive capabilities of MPC and the adaptive learning potential of neural networks. Our SNN-MPC model is contrasted with state-of-the-art genetic algorithm (GA) and ant colony optimization (ACO) methods through a comprehensive simulation analysis. This analysis critically examines the dynamic responses, including current, torque, and speed profiles, of the PMSM under proposed hybrid control strategies. The heart of the work deals with the optimal switching states and subsequent voltage injection to the inverter fed PMSM drive by a predefined minimization principle of a current modulated objective function, where MPC constitutes an integral finite control set (IFCS) mechanism for voltage vector selection and thereby selects the optimized integral gains Kd and Kq for direct and quadrature axes, respectively, with the FCS gain Kfcs obtained from implemented intelligent techniques. Based on the control criteria, the SNN-MPC scheme was established as the preferred benchmark with optimized tuning values of Kd = 0.01, Kq = 0.006, and Kfcs = 0.13, as compared to the gain values tuned from GA and ACO. The experimental setup utilized MATLAB and a Python environment for robust and flexible simulation, ensuring an equitable basis for comparison across all models.
Citation: Shaswat Chirantan, Bibhuti Bhusan Pati. Integration of predictive and computational intelligent techniques: A hybrid optimization mechanism for PMSM dynamics reinforcement[J]. AIMS Electronics and Electrical Engineering, 2024, 8(2): 265-291. doi: 10.3934/electreng.2024012
[1] | Nur Raihan Abdullah, Faez Sharif, Nur Hafizah Azizan, Ismail Fitri Mohd Hafidz, Sugenendran Supramani, Siti Rokhiyah Ahmad Usuldin, Rahayu Ahmad, Wan Abd Al Qadr Imad Wan-Mohtar . Pellet diameter of Ganoderma lucidum in a repeated-batch fermentation for the trio total production of biomass-exopolysaccharide-endopolysaccharide and its anti-oral cancer beta-glucan response. AIMS Microbiology, 2020, 6(4): 379-400. doi: 10.3934/microbiol.2020023 |
[2] | Ahmed Houari, Patrick Di Martino . Polysaccharide-hydrolysing enzymes enhance the in vitro cleaning efficiency of Nanofiltration membranes. AIMS Microbiology, 2019, 5(4): 368-378. doi: 10.3934/microbiol.2019.4.368 |
[3] | Vakhtang V. Dzhavakhiya, Elena V. Glagoleva, Veronika V. Savelyeva, Natalia V. Statsyuk, Maksim I. Kartashov, Tatiana M. Voinova, Alla V. Sergeeva . New bacitracin-resistant nisin-producing strain of Lactococcus lactis and its physiological characterization. AIMS Microbiology, 2018, 4(4): 608-621. doi: 10.3934/microbiol.2018.4.608 |
[4] | Joseph O. Falkinham . Mycobacterium avium complex: Adherence as a way of life. AIMS Microbiology, 2018, 4(3): 428-438. doi: 10.3934/microbiol.2018.3.428 |
[5] | Ananya Mukherjee, Puja Bhattacharjee, Rituparna Das, Arundhati Pal, Amal K. Paul . Endophytic bacteria with plant growth promoting abilities from Ophioglossum reticulatum L.. AIMS Microbiology, 2017, 3(3): 596-612. doi: 10.3934/microbiol.2017.3.596 |
[6] | Thomas Bintsis . Lactic acid bacteria as starter cultures: An update in their metabolism and genetics. AIMS Microbiology, 2018, 4(4): 665-684. doi: 10.3934/microbiol.2018.4.665 |
[7] | Zoya Samoilova, Alexey Tyulenev, Nadezhda Muzyka, Galina Smirnova, Oleg Oktyabrsky . Tannic and gallic acids alter redox-parameters of the medium and modulate biofilm formation. AIMS Microbiology, 2019, 5(4): 379-392. doi: 10.3934/microbiol.2019.4.379 |
[8] | Javier Fernández, Sául Redondo-Blanco, Elisa M. Miguélez, Claudio J. Villar, Alfonso Clemente, Felipe Lombó . Healthy effects of prebiotics and their metabolites against intestinal diseases and colorectal cancer. AIMS Microbiology, 2015, 1(1): 48-71. doi: 10.3934/microbiol.2015.1.48 |
[9] | Yen Thi Ngoc Phan, Minh Thuy Tang, Tu Thi Minh Tran, Van Huu Nguyen, Trang Hien Nguyen, Takeshi Tsuruta, Naoki Nishino . Diversity of lactic acid bacteria in vegetable-based and meat-based fermented foods produced in the central region of Vietnam. AIMS Microbiology, 2017, 3(1): 61-70. doi: 10.3934/microbiol.2017.1.61 |
[10] | Garmasheva I . Isolation and characterization of lactic acid bacteria from Ukrainiantraditional dairy products. AIMS Microbiology, 2016, 2(3): 372-387. doi: 10.3934/microbiol.2016.3.372 |
This paper presents an integrated approach combining a sequential neural network (SNN) with model predictive control (MPC) to enhance the performance of a permanent magnet synchronous motor (PMSM). We address the challenges of traditional control methods that struggle with the dynamics and nonlinear nature of PMSMs, offering a solution that leverages the predictive capabilities of MPC and the adaptive learning potential of neural networks. Our SNN-MPC model is contrasted with state-of-the-art genetic algorithm (GA) and ant colony optimization (ACO) methods through a comprehensive simulation analysis. This analysis critically examines the dynamic responses, including current, torque, and speed profiles, of the PMSM under proposed hybrid control strategies. The heart of the work deals with the optimal switching states and subsequent voltage injection to the inverter fed PMSM drive by a predefined minimization principle of a current modulated objective function, where MPC constitutes an integral finite control set (IFCS) mechanism for voltage vector selection and thereby selects the optimized integral gains Kd and Kq for direct and quadrature axes, respectively, with the FCS gain Kfcs obtained from implemented intelligent techniques. Based on the control criteria, the SNN-MPC scheme was established as the preferred benchmark with optimized tuning values of Kd = 0.01, Kq = 0.006, and Kfcs = 0.13, as compared to the gain values tuned from GA and ACO. The experimental setup utilized MATLAB and a Python environment for robust and flexible simulation, ensuring an equitable basis for comparison across all models.
Ganoderma lucidum is a mushroom traditionally used in Chinese medicine for the prevention and treatment of human disease. Studies on G. lucidum and its products have reported beneficial biological, health-preserving and therapeutic effects [1]–[5]. Fungal polysaccharide has been shown to possess antioxidant, anti-inflammatory, antibacterial, antifungal and antiviral activities [4],[6]–[10], and can be obtained via solid substrate fermentation (SSF) or submerged-liquid fermentation (SLF). However, owing to the inherent nature of the solid substrate in SSF, fungal growth occurs through mycelial colonization of the substrate bed [11]. Furthermore, poor mass transfer and heterogeneity issues within solid matrix render polysaccharide production in SSF a highly time-consuming method. SLF has been shown to be superior to SSF in this respect [11],[12].
In SLF, a suspended biomass grows as a cluster of mycelia that eventually stabilize to form pellets [13] in the form of densely branched hyphae forming a compact ovoid shape. Fungal polysaccharide exists in two forms, exopolysaccharide (EPS) and intracellular polysaccharide (IPS). EPS is secreted outside the mycelium whereas IPS is produced inside the mycelium [10],[14]. Generally, total polysaccharide content produced by the mushroom thus comprises both EPS and IPS. Many factors affect the cultivation of biomass and polysaccharide production in SLF, including pH, agitation speed, oxygen transfer rate (OTR), glucose concentration and temperature [15],[16].
Hence, to enhance the cultivation of biomass and polysaccharide production in SLF, where the key parameters interact with each other in a complex manner, response surface methodology (RSM) represents the most effective solution compared with the one-factor-at-a-time (OFAAT) method [15]. In this study, RSM was used to study the interaction and correlation between the set of experimental variables and obtained results, and to subsequently establish the optimised conditions. The medicinal mushroom G. lucidum was subjected to morphological and molecular analyses prior to liquid fermentation. Next, a preliminary study was conducted using the OFAAT method to obtain baseline data and the working ranges of the selected SLF parameters, prior to the optimisation of biomass, exopolysaccharide (EPS) and intracellular polysaccharide (IPS) production. The selected parameters were initial pH, glucose concentration and agitation rate.
The fruiting body of Ganoderma lucidum was obtained from the Mushroom Unit, Expo Hill, Universiti Putra Malaysia (UPM). The appearance and structure of the fruiting body (Figure 1A) and the basidiospores structure (Figure 1C) was first evaluated to validate the fungus. Next, with some modification of the Stamets [17] method, tissue culture was performed to obtain the mycelium. The fruiting body was washed with 99.9% ethanol (Sigma-Aldrich, Dorset, UK) for 10 s and dried in a laminar flow. Then, it was cracked using a scalpel and the inner part of the fruiting body was twisted and removed using forceps (Figure 1B). The tissue obtained was placed on malt extract agar (MEA) (Sigma-Aldrich, Dorset, UK) and maintained at room temperature until signs of mycelium growth were observed. The mycelium was then sub-cultured onto fresh MEA to obtain pure mycelium (Figure 1D), which was used as an initial culture for preservation in a potato dextrose agar (PDA) (Sigma-Aldrich, Dorset, UK) slant at 4 °C.
The mycelium was separated from agar and placed in pre-cooled pestle and ground to a fine powder under liquid nitrogen. The powder was freeze-dried and stored in an Eppendorf tube (Eppendorf no. 0030120973, Hamburg, Germany) at −20 °C [18],[19].
The fine powdered mycelium (30 mg) was resuspended and lysed in lysis buffer (500 µL) by pipetting multiple times until the suspension became foamy. RNAase A (EN0531, Thermo Scientific, Waltham, MA, USA) was added and the mixture was incubated for 5 min at 37 °C. To remove the cell debris, polysaccharide and protein, NaCl2 solution (165 µL, 5 mol/L) was added and the tube was inverted multiple times before centrifugation (13,000 rpm, 20 min, 4 °C). The resulting supernatant was transferred to a fresh tube and mixed with chloroform (400 µL) and phenol (400 µL) by gentle inversion of the tube multiple times until the solution turned cloudy. The mixture was centrifuged (13,000 rpm, 20 min, 4 °C) and the aqueous phase was removed and extracted using an equal volume of chloroform. DNA was precipitated using 95% ethanol (2 volumes) and purified from polysaccharide by the addition of lysis buffer (500 µL) and mixing by gentle pipetting. NaCl (165 µL, 5 mol/L) was added and mixed by gentle inversion multiple times. To extract the purified DNA, chloroform (2 volumes) was added and the sample was centrifuged (13,000 rpm, 10 min, 4 °C). DNA was precipitated using ethanol (95%) and washed three times in ice-cold ethanol (70%). The washed DNA was dried, dissolved in Tris-EDTA buffer (50 µL) and stored at −20 °C [19].
DNA dissolved in TE buffer (Thermo Fisher no. 12090015, Invitrogen, Waltham, MA, USA) was brought to room temperature slowly from −20 °C. Using universal primers (ITS1 and ITS4), the fungal ITS gene was amplified. First, the solution (500 µL) was added to PCR tubes (Eppendorf no. 0030124332, Hamburg, Germany). Then, 0.5 pmol of ITS1 and ITS4 was added following by deoxynucleotide triphosphates (dNTPs, 200 μM each) (Promega no. U1511, Madison, OH, USA), 0.5 U DNA polymerase (Promega no. D1501, Madison, OH, USA), supplied PCR buffer (ThermoFisher no. 14966123, Platinum II Green PCR Buffer) and water. PCR was performed as follow: 1 cycle (98 °C for 2 min) for initial denaturation; 25 cycles (98 °C for 15 secs; 60 °C for 30 secs; 72 °C for 30 sec) for annealing and extension, and 1 cycle (72 °C for 10 min) for final extension of the amplified DNA (Eppendorf Mastercycler gradient, Hamburg, Germany) [20].
The PCR products were purified and directly sequenced using a 16-capillary 3100 Genetic Analyser (Applied Biosystem, Foster City, CA, USA). A BigDye® Terminator v3.1 Cycle Sequencing Kit (Applied Biosystems, Foster City, CA, USA) was used according to the manufacturer's protocol.
The obtained gDNA sequence was entered into BLAST. The NCBI Nucleotide Collection (nr/nt) database was selected and the query was submitted. Sequences producing significant alignment were identified, and the top 10 hit blast was selected for Multiple Sequencing Alignment (MSA) using Clustal Omega [21].
Using the neighbouring-joining (NJ) in Molecular Evolutionary Genetic Analysis (MEGA-X), the evolutionary distance (Knuc) of identical fungal species was calculated and a phylogenetic tree was generated. The species with closest Knuc were considered the same species [21].
G. lucidum QRS 5120 was subjected to batch fermentation in a 500-mL Erlenmeyer flask using the optimal media compositions and growth parameters (Table 1).
Independent variables | Range and levels |
||
−1 | 0 | 1 | |
Initial pH | 4 | 5 | 6 |
Glucose (g/L) | 10 | 30 | 50 |
Agitation (rpm) | 90 | 100 | 110 |
Based on the preliminary studies, initial pH was shown to have a high significance for the responses (mycelial biomass, EPS production and IPS production) [data not shown]. The media composition of seed culture in the shake flask were constant at (g/L): yeast extract 1 (Oxoid no. LP0021, Dardilly, France), KH2PO4 0.5 (Bendosen Laboratory Chemicals no. C0637, Bendosen, Norway), K2HPO4 0.5 (Bendosen Laboratory Chemicals no. C0680-2296192, Bendosen, Norway), MgSO4 0.5 (Bendosen Laboratory Chemicals no. C0481, Bendosen, Norway), and NH4Cl2 4 (Bendosen Laboratory Chemicals no. C0055, Bendosen, Norway), unless otherwise stated [21]. To optimise the mycelium biomass, EPS and IPS production, CCD was used. The levels and range of the variables for this study are shown in Table 1. The lowest level of variables was initial pH 4; starting glucose concentration = 10 g/L; agitation rate = 90 rpm and the highest level of variables were initial pH 6; starting glucose concentration = 50 g/L; agitation rate = 110 rpm.
To analyse the impact of factors and their interaction, an empirical model was established based on a second-order quadratic model for the responses, as shown in
After the tenth day of fermentation, a 50 mL of sample was filtered using a Buchner funnel filter and the mycelial biomass (Figure 1E) was washed three times with distilled water. The filtered mycelial was dried in a food dehydrator at 35 °C to a constant weight. The mycelial biomass was calculated by subtracting the weight of pre-dried filter paper before filtering from the weight of filter paper with mycelial biomass. To obtain the concentration of mycelial biomass, the value obtained from the subtraction was multiplied by the dilution factor [12].
The supernatant obtained from filtering the mycelial biomass (Section 2.3.1) was mixed with ethanol (95%; 4 volumes), stirred and maintained at 4 °C overnight. The mixture was then transferred to a pre-weighed 50 mL Falcon tube and centrifuged (10,000 rpm, 15 min). The supernatant was discarded upon centrifuging and the pellet was placed in a food dehydrator at the lowest temperature until a constant weight was achieved. Next, the EPS yield was estimated by multiplying the dilution factor by the EPS weight [12].
After weighing, the filtered mycelial biomass (Section 2.3.1) was mixed with distilled water (10 volumes). Then, the mixture was sterilised (121 °C, 30 min, 15 psi) in an autoclave and the mixture was filtered to obtain the supernatant, mixed with ethanol (95%; 4 volumes), stirred and maintained at 4 °C overnight. Next, the mixture was transferred to a pre-weighed 50 mL Falcon tube and centrifuged (10,000 rpm, 15 min). The supernatant was discarded, and the pellet was placed in a food dehydrator at the lowest temperature until a constant weight was achieved. The IPS yield was estimated by multiplying the dilution factor by the IPS weight [14].
Molecular identification of a wild fungal sample is important to determine the species of the sample [22]. Thus, molecular identification was performed on wild G. lucidum. The base pairs of wild G. lucidum were estimated using agarose gel electrophoresis under UV light (Figure 2). The marker (Lane 1) represented the standard curve, and the base pairs of QRS 5120 were estimated to be 637 bp.
Upon sequencing of the product, it was aligned with the top-10 related species as retrieved from NCBI BLAST. Based on the BLAST reference databases, QRS 5120 was found to be 99% similar to Ganoderma sp. Detailed phylogenetic analyses (Figure 3) showed the evolutionary distance (Knuc) values. Clade A showed that G. lucidum QRS 5120 was closely related to G. lucidum isolate 39s compared with G. lucidum isolate 49s.
Using RSM, the effect of initial pH, starting glucose concentration and agitation rate on the biomass, EPS and IPS production was investigated. CCD design, the level of each variable and the responses are shown in Table 2. In total, twenty experiments were designated by CCD, where the coefficients were evaluated using non-linear regression analysis. To estimate the significance of the model coefficient, analysis of variance (ANOVA) was used. The significance of each coefficient was indicated by p < 0.05.
Run No. | Variables |
Responses |
||||||||
Initial pH | Glucose (g/L) | Agitation (rpm) | Biomass (DCW g/L) |
EPS (g/L) |
IPS (g/L) |
|||||
Actual | Predicted | Actual | Predicted | Actual | Predicted | |||||
1 | 4 | 10 | 90 | 4.6 | 4.52 | 2.2 | 2.31 | 1.2 | 1.28 | |
2 | 6 | 50 | 110 | 3.8 | 3.92 | 1.2 | 1.13 | 0.2 | 0.14 | |
3 | 6 | 50 | 90 | 3.1 | 2.97 | 0.9 | 0.91 | 0.2 | 0.25 | |
4 | 5 | 30 | 100 | 4.1 | 4.12 | 1.1 | 1.31 | 0.8 | 0.85 | |
5 | 5 | 30 | 90 | 3.9 | 3.6 | 1.3 | 1.15 | 0.7 | 0.73 | |
6 | 5 | 50 | 100 | 3.8 | 3.96 | 1 | 0.91 | 0.9 | 0.83 | |
7 | 5 | 30 | 100 | 4.2 | 4.12 | 1.4 | 1.31 | 0.9 | 0.85 | |
8 | 4 | 50 | 110 | 5.1 | 4.79 | 2.1 | 2.18 | 1.4 | 1.53 | |
9 | 5 | 30 | 100 | 3.8 | 4.12 | 1 | 1.31 | 0.9 | 0.85 | |
10 | 4 | 50 | 90 | 4.9 | 5.04 | 1.9 | 1.96 | 1.5 | 1.44 | |
11 | 5 | 30 | 100 | 3.9 | 4.12 | 1.3 | 1.31 | 0.7 | 0.85 | |
12 | 5 | 30 | 110 | 4.2 | 4.3 | 1.4 | 1.37 | 0.9 | 0.77 | |
13 | 5 | 30 | 100 | 4.2 | 4.12 | 1.5 | 1.31 | 0.8 | 0.85 | |
14 | 5 | 30 | 100 | 4.1 | 4.12 | 1.2 | 1.31 | 0.8 | 0.85 | |
15 | 4 | 30 | 100 | 5.2 | 5.26 | 2.9 | 2.61 | 1.7 | 1.57 | |
16 | 6 | 10 | 110 | 4.1 | 4 | 1.2 | 1.18 | 0.1 | 0.18 | |
17 | 6 | 30 | 100 | 4 | 3.74 | 1.3 | 1.41 | 0.3 | 0.33 | |
18 | 4 | 10 | 110 | 4.8 | 4.97 | 2.5 | 2.53 | 1.5 | 1.47 | |
19 | 5 | 10 | 100 | 4.1 | 3.74 | 1.2 | 1.11 | 0.8 | 0.77 | |
20 | 6 | 10 | 90 | 2 | 2.35 | 1 | 0.96 | 0.3 | 0.19 |
The ANOVA for mycelium biomass production is shown in
Source | Sum of Squares | DF | Mean Square | F Value | Prob > F | |
Model | 8.588136364 | 9 | 0.954237374 | 11.90767001 | 0.0003* | significant |
A: pH | 5.776 | 1 | 5.776 | 72.07714124 | <0.0001* | significant |
B: Glucose | 0.121 | 1 | 0.121 | 1.509926262 | 0.2473 | |
C: Agitation | 1.225 | 1 | 1.225 | 15.28644356 | 0.0029* | significant |
A2 | 0.410511364 | 1 | 0.410511364 | 5.122660238 | 0.0471* | significant |
B2 | 0.191136364 | 1 | 0.191136364 | 2.385138968 | 0.1535 | |
C2 | 0.073636364 | 1 | 0.073636364 | 0.918888259 | 0.3604 | |
AB | 0.005 | 1 | 0.005 | 0.062393647 | 0.8078 | |
AC | 0.72 | 1 | 0.72 | 8.984685196 | 0.0134* | significant |
BC | 0.245 | 1 | 0.245 | 3.057288712 | 0.1109 | |
Residual | 0.801363636 | 10 | 0.080136364 | |||
Lack of Fit | 0.666363636 | 5 | 0.133272727 | 4.936026936 | 0.0522 | not significant |
Pure Error | 0.135 | 5 | 0.027 | |||
Cor Total | 9.3895 | 19 | ||||
Std. Dev. = 0.28308366896796 | R2 = 0.91465321514845 | Adeq Precision = 14.52399050576 | ||||
Mean = 4.095 | Adjusted R2 = 0.83784110878205 |
* Significant value.
From the model, initial pH (A) shows the strongest effect (p < 0.0001) on biomass, while agitation (C) shows a significant effect at p < 0.05. Both quadratic terms of initial pH (AA) and initial pH and agitation (AC) show significance effect at p < 0.05 on the yield of mycelium biomass. However, negative effects are shown by glucose (B) and quadratic terms (B2, C2, AB, and BC). Figure 4 shows the combined effect of initial pH, glucose concentration and agitation in three-dimensional (3D) plots. One factor is at the optimum level and the other two factors are within experimental range. Figure 4a shows the effect of initial pH (A) and starting glucose concentration (B), Figure 4b shows the effect of A and agitation rate (C), and Figure 4c shows the effect of B and C on biomass production. From Figure 4a,b, it is clear that increasing the initial pH leads to a decrease in the mycelium biomass, while agitation at all rates shows that high mycelium biomass production and starting glucose concentration is normally distributed. The maximum mycelium biomass obtained was at initial pH 4, glucose concentration 26.5 g/L and 100 rpm. From Figure 4c, no significance effect of B and C on mycelium biomass production was observed.
The ANOVA of EPS production is shown in
Source | Sum of Squares | DF | Mean Square | F Value | Prob > F | |
Model | 5.364181818 | 9 | 0.596020202 | 16.20420717 | <0.0001* | significant |
A: pH | 3.6 | 1 | 3.6 | 97.8744439 | <0.0001* | significant |
B: Glucose | 0.1 | 1 | 0.1 | 2.718734553 | 0.1302 | |
C: Agitation | 0.121 | 1 | 0.121 | 3.289668809 | 0.0998 | |
A2 | 1.330056818 | 1 | 1.330056818 | 36.16071429 | 0.0001* | significant |
B2 | 0.255056818 | 1 | 0.255056818 | 6.934317845 | 0.0250* | significant |
C2 | 0.008181818 | 1 | 0.008181818 | 0.222441918 | 0.6473 | |
AB | 0.045 | 1 | 0.045 | 1.223430549 | 0.2946 | |
AC | 0 | 1 | 0 | 0 | 1.0000 | |
BC | 0 | 1 | 0 | 0 | 1.0000 | |
Residual | 0.367818182 | 10 | 0.036781818 | |||
Lack of Fit | 0.192818182 | 5 | 0.038563636 | 1.101818182 | 0.4589 | not significant |
Pure Error | 0.175 | 5 | 0.035 | |||
Cor Total | 5.732 | 19 | ||||
Std. Dev. = 0.19178586543804 | R2 = 0.93583074287889 | Adeq Precision = 12.53566341056 | ||||
Mean = 1.48 | Adjusted R2 = 0.8780784114699 |
* Significant value.
From the model, initial pH (A) shows the strongest effect (p < 0.0001) on EPS concentration while both quadratic terms of initial pH (AA) and initial pH and glucose (BB) show a significance effect at p < 0.005 and p < 0.05, respectively, on EPS production. However, negative effects are shown by glucose (B), agitation (C) and quadratic terms (C2, AB, AC and BC). Figure 5 shows the combined effect of initial pH, glucose concentration and agitation in 3D plots. One factor is at the optimum level and the other two factors are within experimental range. Figure 5a shows the effect of initial pH (A) and starting glucose concentration (B), Figure 5b shows the effect of A and agitation rate (C) and Figure 5c shows the effect of B and C on biomass production. From Figure 5a,b, increasing initial pH leads decreased EPS production, agitation at all rates shows high EPS production and starting glucose concentration is normally distributed. The maximum EPS obtained was at initial pH 4, glucose concentration 26.5 g/L and 100 rpm. From Figure 5c, no significance effect of B and C on mycelium biomass production was observed.
The ANOVA of IPS production is shown in
Source | Sum of Squares | DF | Mean Square | F Value | Prob > F | |
Model | 3.935181818 | 9 | 0.437242424 | 34.47789725 | <0.0001* | significant |
A: pH | 3.844 | 1 | 3.844 | 303.1111111 | <0.0001* | significant |
B: Glucose | 0.009 | 1 | 0.009 | 0.709677419 | 0.4192 | |
C: Agitation | 0.004 | 1 | 0.004 | 0.315412186 | 0.5867 | |
A2 | 0.030056818 | 1 | 0.030056818 | 2.370071685 | 0.1547 | |
B2 | 0.005681818 | 1 | 0.005681818 | 0.448028674 | 0.5184 | |
C2 | 0.025056818 | 1 | 0.025056818 | 1.975806452 | 0.1901 | |
AB | 0.005 | 1 | 0.005 | 0.394265233 | 0.5441 | |
AC | 0.02 | 1 | 0.02 | 1.577060932 | 0.2377 | |
BC | 0.005 | 1 | 0.005 | 0.394265233 | 0.5441 | |
Residual | 0.126818182 | 10 | 0.012681818 | |||
Lack of Fit | 0.098484848 | 5 | 0.01969697 | 3.475935829 | 0.0989 | not significant |
Pure Error | 0.028333333 | 5 | 0.005666667 | |||
Cor Total | 4.062 | 19 | ||||
Std. Dev. = 0.11261357902943 | R2 = 0.96877937424466 | Adeq Precision = 17.969511850411 | ||||
Mean = 0.83 | Adjusted R2 = 0.94068081106486 |
* Significant value.
From the model, initial pH (A) shows the strongest effect (p < 0.0001) on IPS concentration. However, negative effects are shown by glucose (B), agitation (C) and quadratic terms (A2, B2, C2, AB, AC and BC). Figure 6 shows the combined effect of initial pH, glucose concentration and agitation in 3D plots. One factor is at the optimum level and the other two factors are within experimental range. Figure 6a shows the effect of initial pH (A) and starting glucose concentration (B), Figure 6b shows the effect of A and agitation rate (C) and Figure 6c shows the effect of B and C on biomass production. From Figure 6a,b, it is clear that increasing initial pH leads to decreased IPS production, agitation at all rates shows high IPS production and all concentrations of starting glucose give high IPS concentration. By this, it was concluded that there was no interaction between the factors. The maximum IPS obtained was at initial pH 4, glucose concentration 40.45 g/L and 103 rpm. From Figure 6c, no significance effect of B and C on IPS production was observed.
Table 6 shows the optimised conditions applied to verify the mycelium biomass, EPS and IPS production statistical model. To verify the strength and precision of the model under Eq 2, Eq 3 and Eq 4, various experiments were performed. The mycelium biomass obtained was 5.12 g/L, EPS production under optimized conditions was 2.49 g/L, and IPS production under optimized conditions was 1.52 g/L, which aligns with the predicted values (5.25 g/L, 2.69 g/L and 1.59 g/L, respectively). This shows that the model (Eq 2, Eq 3 and Eq 4) is valid for mycelium biomass, EPS and IPS production.
Run | Variables |
Response |
||||
pH | Glucose | Agitation | Biomass (DCW g/L) | EPS (g/L) | IPS (g/L) | |
Biomass | 4.01 | 32.09 | 102.45 | 5.12 ± 0.5 | − | − |
EPS | 4 | 24.25 | 110 | − | 2.49 ± 0.8 | − |
IPS | 4 | 40.43 | 103 | − | − | 1.52 ± 0.4 |
Biomass + EPS | 4 | 24.75 | 107.58 | 5.11 ± 0.4 | 2.57 ± 0.7 | − |
Biomass + IPS | 4 | 40.45 | 102.95 | 5.13 ± 0.5 | − | 1.57 ± 0.3 |
EPS + IPS | 4 | 26.5 | 105.92 | − | 2.62 ± 0.4 | 1.52 ± 0.6 |
Biomass + EPS + IPS | 4 | 26.5 | 100 | 5.19 ± 0.6 | 2.64 ± 0.6 | 1.52 ± 0.4 |
The current statistical optimization approach to determine the preeminent parameters for obtaining efficient biomass, EPS and IPS production using G. lucidum in controlled shake-flask fermentation is shown in Table 7. As reported, only two studies utilizing G. lucidum for this purpose have previously been reported, utilizing only EPS and biomass production. ChangTsai and Houng [23] stated that mycelium formation and polysaccharide production were markedly improved by cultivation in optimal medium under optimal operating conditions. YuanChi and Zhang [24] also supported this observation by stating that optimal media markedly increases EPS production and mycelium formation. The process described in the current study was more efficient in producing biomass and EPS concentration compared with the results of YuanChi and Zhang [24] and ChangTsai and Houng [23], with the addition of IPS. The optimized key parameters reported in this study are the most up-to-date for G. lucidum using RSM on initial pH, glucose concentration and agitation for enhancing the production of biomass, EPS and IPS. The current optimized method can therefore be applied to achieve a combination of lower biomass with higher EPS and IPS in specialized bioreactors.
Optimization method | Cultivation mode | Initial pH | Glucose concentration (g/L) | Agitation (rpm) | EPS (g/L) | IPS (g/L) | Biomass (g/L) | Reference |
Response surface methodology | Shake Flask | 4 | 26.5 | 100 | 2.64 | 1.52 | 5.19 | Current study |
Taguchi's orthogonal array | Shake Flask | 6.5 | 12.1 | 160 | 0.420 | NA | 18.70 | [23] |
Orthogonal matrix | Shake Flask | − | 50 | 150 | 1.723 | NA | 7.235 | [24] |
*NA = Not available, EPS = Exopolysaccharide, IPS = Intracellular polysaccharide.
The wild-cultivated Malaysian medicinal mushroom G. lucidum strain QRS 5120 was successfully identified using morphological and biomolecular methods. Using response surface methodology in a submerged-liquid fermentation, maximum mycelial biomass (5.19 ± 0.6 g/L) and EPS (2.64 ± 0.6 g/L) were obtained under optimized conditions of initial pH 4, 26.52 g/L glucose concentration and 103 rpm. On the other hand, maximum IPS production (1.57 ± 0.3 g/L) was obtained under optimized conditions of initial pH 4, glucose concentration 40.45 g/L and agitation 103 rpm. In addition, in order to maximise all three responses together, i.e. biomass, EPS and IPS (5.19 g/L, 2.64 g/L and 1.52 g/L respectively), the optimised conditions were initial pH 4, glucose concentration at 26.5 g/L and 100 rpm. It was found that by using the optimised conditions, the biomass, EPS and IPS production could be maximized. The initial pH could significantly affect biomass, EPS and IPS production in comparison to glucose concentration and agitation rate. The current work is suitable to be applied for other type of fungal fermentation for efficient EPS, IPS and biomass production.
[1] | Wang L, Chai S, Yoo D, Gan L, Ng K (2015) PID and Predictive Control of Electrical Drives and Power Converters using MATLAB/Simulink, John Wiley & Sons. https://doi.org/10.1002/9781118339459 |
[2] |
Zhang X, Zhao Z (2021) Multi-stage Series Model Predictive Control for PMSM Drives. IEEE T Veh Technol 70: 6591-6600. https://doi.org/10.1109/TVT.2021.3086532 doi: 10.1109/TVT.2021.3086532
![]() |
[3] |
Ren B, Chen H, Zhao H, Xu W (2017) MPC-based torque control of permanent magnet synchronous motor for electric vehicles via switching optimization. Control Theory Technology 15: 138–149. https://doi.org/10.1007/s11768-017-6193-z doi: 10.1007/s11768-017-6193-z
![]() |
[4] |
Liu M, Chan KW, Hu J, Xu W, Rodriguez J (2019) Model predictive direct speed control with torque oscillation reduction for PMSM drives. IEEE T Ind Inform 15: 4944-4956. https://doi.org/10.1109/TⅡ.2019.2898004 doi: 10.1109/TⅡ.2019.2898004
![]() |
[5] |
Tan LN, Pham TC (2021) Optimal Tracking Control for PMSM With Partially Unknown Dynamics, Saturation Voltages, Torque, and Voltage Disturbances. IEEE T Ind Electron 69: 3481-3491. https://doi.org/10.1109/TIE.2021.3075892 doi: 10.1109/TIE.2021.3075892
![]() |
[6] |
Liu Z, Han Y, Feng G, Kar NC (2022) Efficient Nonlinear Multi-Parameter Decoupled Estimation of PMSM Drives Based on Multi-State Voltage and Torque Measurements. IEEE T Energy Conver 38: 321-331. https://doi.org/10.1109/TEC.2022.3187130 doi: 10.1109/TEC.2022.3187130
![]() |
[7] | Marulasiddappa HB, Pushparajesh V, Begum A (2023) Direct Torque Control Based Jelly Fish Algorithm For Torque Ripple Reduction in Permanent Magnet Synchronous Motor. 2023 International Conference on Network, Multimedia and Information Technology (NMITCON), 1-6. |
[8] | Kakodia SK, Giribabu D, Ravula RK (2022) Torque Ripple Minimization using an Artificial Neural Network based Speed Sensor less control of SVM-DTC fed PMSM Drive. 2022 IEEE Texas Power and Energy Conference (TPEC), 1-6. https://doi.org/10.1109/TPEC54980.2022.9750850 |
[9] |
Wang F, Zuo K, Tao P, Rodriguez J (2020) High performance model predictive control for PMSM by using stator current mathematical model self-regulation technique. IEEE T Power Electron 35: 13652-13662. https://doi.org/10.1109/TPEL.2020.2994948 doi: 10.1109/TPEL.2020.2994948
![]() |
[10] |
Zhang Y, Jiang H, Yang H (2020) Model Predictive Control of PMSM Drives Based on General Discrete Space Vector Modulation. IEEE T Energy Conver 36: 1300-1307. https://doi.org/10.1109/TEC.2020.3036082 doi: 10.1109/TEC.2020.3036082
![]() |
[11] |
Favato A, Carlet PG, Toso F, Torchio R, Bolognani S (2021) Integral Model Predictive Current Control for Synchronous Motor Drives. IEEE T Power Electron 36: 13293-13303. https://doi.org/10.1109/TPEL.2021.3081827 doi: 10.1109/TPEL.2021.3081827
![]() |
[12] | Li Y, Xiao H, Jin N, Yan G (2022) Model predictive control of NPC three-level grid-tied converter based on reconstructed current. Archives of Electrical Engineering 71. https://doi.org/10.1109/ACCESS.2021.3119566 |
[13] |
Agoro S, Husain I (2021) Robust Deadbeat Finite-Set Predictive Current Control With Torque Oscillation and Noise Reduction for PMSM Drives. IEEE T Ind Appl 58: 365-374. https://doi.org/10.1109/TIA.2021.3130022 doi: 10.1109/TIA.2021.3130022
![]() |
[14] |
Cui J, Tao T, Zhao W (2023) Optimized Control Set Model Predictive Control for Dual Three-Phase PMSM With Minimum Error Duty Cycle Regulation. IEEE T Power Electr 39: 1319-1332. https://doi.org/10.1109/TPEL.2023.3324209 doi: 10.1109/TPEL.2023.3324209
![]() |
[15] |
Hassan M, Ge X, Atif R, Woldegiorgis AT, Mastoi MS, Shahid MB (2022) Computational efficient model predictive current control for interior permanent magnet synchronous motor drives. IET Power Electronics 15: 1111-1133. https://doi.org/10.1049/pel2.12294 doi: 10.1049/pel2.12294
![]() |
[16] |
Hassan M, Ge X, Woldegiorgis AT, Mastoi MS, Shahid MB, Atif R, et al. (2023) A look-up table-based model predictive torque control of IPMSM drives with duty cycle optimization. ISA T 138: 670-686. https://doi.org/10.1016/j.isatra.2023.02.007 doi: 10.1016/j.isatra.2023.02.007
![]() |
[17] |
Yao C, Sun Z, Xu S, Zhang H, Ren G, Ma G (2022) ANN Optimization of Weighting Factors Using Genetic Algorithm for Model Predictive Control of PMSM Drives. IEEE T Ind Appl 58: 7346-7362. https://doi.org/10.1109/TIA.2022.3190812 doi: 10.1109/TIA.2022.3190812
![]() |
[18] | Li Y, Guo W, Liu D, Zhang X, Deng Y (2023) Model Predictive Current Control for Permanent Magnet Synchronous Motor based on Neural Network. 2023 IEEE International Conference on Predictive Control of Electrical Drives and Power Electronics (PRECEDE), 1-6. https://doi.org/10.1109/PRECEDE57319.2023.10174503 |
[19] |
Mao H, Tang X, Tang H (2022) Speed control of PMSM based on neural network model predictive control. Tran Inst Meas Control 44: 2781–2794. https://doi.org/10.1177/01423312221086267 doi: 10.1177/01423312221086267
![]() |
[20] | Valdez F, Vázquez JC, Melin P (2021) A New Hybrid Method Based on ACO and PSO with Fuzzy Dynamic Parameter Adaptation for Modular Neural Networks Optimization. Fuzzy Logic Hybrid Extensions of Neural and Optimization Algorithms: Theory and Applications, 337-361. https://doi.org/10.1007/978-3-030-68776-2_20 |
[21] |
Saeed IK, Sheikhyounis K (2022) Power quality improvement of distribution systems asymmetry caused by power disturbances based on particle swarm optimization-artificial neural network. Int J Electr Comput Eng Syst 25: 666–679. https://doi.org/10.11591/ijeecs.v25.i2.pp666-679 doi: 10.11591/ijeecs.v25.i2.pp666-679
![]() |
[22] | Chafi ZS, Afrakhte H (2021) Short-Term Load Forecasting Using Neural Network and Particle Swarm Optimization (PSO) Algorithm. Math Probl Eng 2021: Article ID 5598267. https://doi.org/10.1155/2021/5598267 |
[23] | Alhmoud L (2019) Short-term load forecasting for Jordan's Power System Using Neural Network based Different Optimization Techniques. 2019 IEEE International Conference on Environment and Electrical Engineering and 2019 IEEE Industrial and Commercial Power Systems Europe (EEEIC/I & CPS Europe), 1-6. |
[24] | Abdolrasol MGM, Hussain S, Ustun T, Sarker M, Hannan M, Mohamed R, et al. (2021) Artificial Neural Networks Based Optimization Techniques: A Review. Electronics 10: 2689. https://doi.org/10.3390/electronics10212689 |
[25] |
Moayedi H, Bui D, Gör M, Pradhan B, Jaafari A (2019) The Feasibility of Three Prediction Techniques of the Artificial Neural Network, Adaptive Neuro-Fuzzy Inference System, and Hybrid Particle Swarm Optimization for Assessing the Safety Factor of Cohesive Slopes. ISPRS Int J Geo-Inf 8: 391. https://doi.org/10.3390/ijgi8090391 doi: 10.3390/ijgi8090391
![]() |
[26] |
Mahadeva R, Kumar M, Manik G, Patole S (2021) Modeling, simulation, and optimization of the membrane performance of seawater reverse osmosis desalination plant using neural network and fuzzy based soft computing techniques. Desalin Water Treat 229: 17-30. https://doi.org/10.5004/dwt.2021.27386 doi: 10.5004/dwt.2021.27386
![]() |
[27] |
Jayalakshmi M, Rao S (2020) Discrete Wavelet Transmission and Modified PSO with ACO Based Feed Forward Neural Network Model for Brain Tumour Detection. CMC-Comput Mater Continua 65: 1081-1096. https://doi.org/10.32604/cmc.2020.011710 doi: 10.32604/cmc.2020.011710
![]() |
[28] |
de Jesus KLD, Senoro D, dela Cruz JD, Chan E (2021) A Hybrid Neural Network–Particle Swarm Optimization Informed Spatial Interpolation Technique for Groundwater Quality Mapping in a Small Island Province of the Philippines. Toxics 9: 273. https://doi.org/10.3390/toxics9110273 doi: 10.3390/toxics9110273
![]() |
[29] |
Krishna K, Thirumuru R (2023) Enhanced QOS energy-efficient routing algorithm using deep belief neural network in hybrid falcon-improved ACO nature-inspired optimization in wireless sensor networks. Neural Netw World 33: 113-141. https://doi.org/10.14311/nnw.2023.33.008 doi: 10.14311/nnw.2023.33.008
![]() |
[30] |
Chirantan S, Pati BB (2024) Torque and dq axis current dynamics of an inverter fed induction motor drive that leverages computational intelligent techniques. AIMS Electronics and Electrical Engineering 8: 28-52. https://doi.org/10.3934/electreng.2024002 doi: 10.3934/electreng.2024002
![]() |
[31] | Dat NT, Kien CV, Anh HPH (2021) Hybrid Super-Twisting Sliding Mode and FOC Scheme for Advanced PMSM Driving Control. International Conference on Advanced Mechanical Engineering, Automation, and Sustainable Development, 776-780. https://doi.org/10.1007/978-3-030-99666-6_112 |
[32] | Sahu S, Nayak B, Dash RN (2022) Analysis of Five-Phase Surface PMSM for Application in Electric Vehicles. Sustainable Energy and Technological Advancements: Proceedings of ISSETA 2021, 587-595. https://doi.org/10.1007/978-981-16-9033-4_43 |
1. | Zarimah Mohd Hanafiah, Wan Hanna Melini Wan Mohtar, Hassimi Abu Hasan, Henriette Stokbro Jensen, Anita Klaus, Wan Abd Al Qadr Imad Wan-Mohtar, Performance of wild-Serbian Ganoderma lucidum mycelium in treating synthetic sewage loading using batch bioreactor, 2019, 9, 2045-2322, 10.1038/s41598-019-52493-y | |
2. | Norhidayah Mohd Taufek, Hanis H. Harith, Muhamad Hafiz Abd Rahim, Zul Ilham, Neil Rowan, Wan Abd Al Qadr Imad Wan-Mohtar, Performance of mycelial biomass and exopolysaccharide from Malaysian Ganoderma lucidum for the fungivore red hybrid Tilapia (Oreochromis sp.) in Zebrafish embryo, 2020, 17, 23525134, 100322, 10.1016/j.aqrep.2020.100322 | |
3. | Mohamad Nor Azzimi Sohedein, Wan Abd Al Qadr Imad Wan-Mohtar, Yeong Hui-Yin, Zul Ilham, Jo-Shu Chang, Sugenendran Supramani, Phang Siew-Moi, Optimisation of biomass and lipid production of a tropical thraustochytrid Aurantiochytrium sp. UMACC-T023 in submerged-liquid fermentation for large-scale biodiesel production, 2020, 23, 18788181, 101496, 10.1016/j.bcab.2020.101496 | |
4. | Siti Rokhiyah Ahmad Usuldin, Norfaizah Mahmud, Zul Ilham, Nur Kusaira Khairul Ikram, Rahayu Ahmad, Wan Abd Al Qadr Imad Wan-Mohtar, In-depth spectral characterization of antioxidative (1,3)-β-D-glucan from the mycelium of an identified tiger milk mushroom Lignosus rhinocerus strain ABI in a stirred-tank bioreactor, 2020, 23, 18788181, 101455, 10.1016/j.bcab.2019.101455 | |
5. | Wan Abd Al Qadr Imad Wan-Mohtar, Norhidayah Mohd Taufek, Jaganath Puvanen Thiran, Janathu Ferdaus Pajaru Rahman, Grema Yerima, Kumeera Subramaniam, Neil Rowan, Investigations on the use of exopolysaccharide derived from mycelial extract of Ganoderma lucidum as functional feed ingredient for aquaculture-farmed red hybrid Tilapia (Oreochromis sp.), 2021, 3, 26668335, 100018, 10.1016/j.fufo.2021.100018 | |
6. | Wan Abd Al Qadr Imad Wan-Mohtar, Norhidayah Mohd Taufek, Grema Yerima, Janathu Rahman, Jaganath P. Thiran, Kumeera Subramaniam, Vikineswary Sabaratnam, Effect of bioreactor-grown biomass from Ganoderma lucidum mycelium on growth performance and physiological response of red hybrid tilapia (Oreochromis sp.) for sustainable aquaculture, 2020, 1879-4238, 10.1007/s13165-020-00303-5 | |
7. | Saeideh Ahmadifar, syed Mohsen Hosseini, Ebrahim Mohammadi Goltapeh, Akbar Jahedi, Optimal method for production of mycelia biomass of Ganoderma lucidum in sugarcane molasses, 2020, 9, 2251-9270, 1, 10.29252/pps.9.1.1 | |
8. | Nurul Amirah Hassan, Sugenendran Supramani, Mohamad Nor Azzimi Sohedein, Siti Rokhiyah Ahmad Usuldin, Anita Klaus, Zul Ilham, Wei-Hsin Chen, Wan Abd Al Qadr Imad Wan-Mohtar, Efficient biomass-exopolysaccharide production from an identified wild-Serbian Ganoderma lucidum strain BGF4A1 mycelium in a controlled submerged fermentation, 2019, 21, 18788181, 101305, 10.1016/j.bcab.2019.101305 | |
9. | Anita Klaus, Wan Abd Al Qadr Imad Wan-Mohtar, Biljana Nikolić, Stefana Cvetković, Jovana Vunduk, Pink oyster mushroom Pleurotus flabellatus mycelium produced by an airlift bioreactor—the evidence of potent in vitro biological activities, 2021, 37, 0959-3993, 10.1007/s11274-020-02980-6 | |
10. | Wan Abd Al Qadr Imad Wan-Mohtar, Zul Ilham, Adi Ainurzaman Jamaludin, Neil Rowan, Use of Zebrafish Embryo Assay to Evaluate Toxicity and Safety of Bioreactor-Grown Exopolysaccharides and Endopolysaccharides from European Ganoderma applanatum Mycelium for Future Aquaculture Applications, 2021, 22, 1422-0067, 1675, 10.3390/ijms22041675 | |
11. | Silambarasi Mooralitharan, Zarimah Mohd Hanafiah, Teh Sabariah Binti Abd Manan, Hassimi Abu Hasan, Henriette S. Jensen, Wan Abd Al Qadr Imad Wan-Mohtar, Wan Hanna Melini Wan Mohtar, Optimization of mycoremediation treatment for the chemical oxygen demand (COD) and ammonia nitrogen (AN) removal from domestic effluent using wild-Serbian Ganoderma lucidum (WSGL), 2021, 0944-1344, 10.1007/s11356-021-12686-3 | |
12. | Jovana Vunduk, Wan Abd Al Qadr Imad Wan-Mohtar, Shaiful Azuar Mohamad, Nur Hafizati Abd Halim, Ahmad Zainuri Mohd Dzomir, Željko Žižak, Anita Klaus, Polysaccharides of Pleurotus flabellatus strain Mynuk produced by submerged fermentation as a promising novel tool against adhesion and biofilm formation of foodborne pathogens, 2019, 112, 00236438, 108221, 10.1016/j.lwt.2019.05.119 | |
13. | Z Ilham, M R A Mansor, Optimized Conversion of Nyamplung Seeds Oil to Biodiesel Using Box-Behnken Response Surface Methodology (RSM), 2020, 877, 1757-899X, 012029, 10.1088/1757-899X/877/1/012029 | |
14. | Fátima R. Viceconte, Marina L. Diaz, Daniela S. Soresi, Ileana B. Lencinas, Alicia Carrera, María Inés Prat, María Soledad Vela Gurovic, Ganoderma sessile is a fast polysaccharide producer among Ganoderma species, 2021, 0027-5514, 1, 10.1080/00275514.2020.1870255 | |
15. | Liping Liu, Jie Feng, Kun Gao, Shuai Zhou, Mengqiu Yan, Chuanhong Tang, Jing Zhou, Yanfang Liu, Jingsong Zhang, Influence of carbon and nitrogen sources on structural features and immunomodulatory activity of exopolysaccharides from Ganoderma lucidum, 2022, 119, 13595113, 96, 10.1016/j.procbio.2022.05.016 | |
16. | Arman Hamza, Shreya Ghanekar, Devarai Santhosh Kumar, Current trends in health-promoting potential and biomaterial applications of edible mushrooms for human wellness, 2023, 51, 22124292, 102290, 10.1016/j.fbio.2022.102290 | |
17. | Besek Mariam Mohamad Jahis, Zul Ilham, Sugenendran Supramani, Mohamad Nor Azzimi Sohedein, Mohamad Faizal Ibrahim, Suraini Abd-Aziz, Neil Rowan, Wan Abd Al Qadr Imad Wan-Mohtar, Ganodiesel: A New Biodiesel Feedstock from Biomass of the Mushroom Ganoderma lucidum, 2022, 14, 2071-1050, 10764, 10.3390/su141710764 | |
18. | Siti Rokhiyah Ahmad Usuldin, Wan Abd Al Qadr Imad Wan-Mohtar, Zul Ilham, Adi Ainurzaman Jamaludin, Nur Raihan Abdullah, Neil Rowan, In vivo toxicity of bioreactor-grown biomass and exopolysaccharides from Malaysian tiger milk mushroom mycelium for potential future health applications, 2021, 11, 2045-2322, 10.1038/s41598-021-02486-7 | |
19. | Rahayu Ahmad, Srivani Sellathoroe, Ehwan Ngadi, Tengku Shafazila Tengku Saharuddin, Iffah Izzati Zakaria, Suguna Selvakumaran, Wan Abd Al Qadr Imad Wan-Mohtar, Isolation, identification, cultivation and determination of antimicrobial β-glucan from a wild-termite mushroom Termitomyces heimii RFES 230662, 2021, 37, 18788181, 102187, 10.1016/j.bcab.2021.102187 | |
20. | Z. M. Hanafiah, W. H. M. Wan Mohtar, H. A. Hasan, H. S. Jensen, A. Klaus, S. Sharil, W. A. A. Q. I. Wan-Mohtar, Ability of Ganoderma lucidum mycelial pellets to remove ammonia and organic matter from domestic wastewater, 2022, 19, 1735-1472, 7307, 10.1007/s13762-021-03633-3 | |
21. | Siti Rokhiyah Ahmad Usuldin, Zul Ilham, Adi Ainurzaman Jamaludin, Rahayu Ahmad, Wan Abd Al Qadr Imad Wan-Mohtar, Enhancing Biomass-Exopolysaccharides Production of Lignosus rhinocerus in a High-Scale Stirred-Tank Bioreactor and Its Potential Lipid as Bioenergy, 2023, 16, 1996-1073, 2330, 10.3390/en16052330 | |
22. | Sugenendran Supramani, Nur Ardiyana Rejab, Zul Ilham, Rahayu Ahmad, Pau-Loke Show, Mohamad Faizal Ibrahim, Wan Abd Al Qadr Imad Wan-Mohtar, Performance of Biomass and Exopolysaccharide Production from the Medicinal Mushroom Ganoderma lucidum in a New Fabricated Air-L-Shaped Bioreactor (ALSB), 2023, 11, 2227-9717, 670, 10.3390/pr11030670 | |
23. | Joshini Pillai Balamurugan, Sugenendran Supramani, Siti Rokhiyah Ahmad Usuldin, Zul Ilham, Anita Klaus, Nur Kusaira Khairul Ikram, Rahayu Ahmad, Wan Abd Al Qadr Imad Wan-Mohtar, Efficient biomass-endopolysaccharide production from an identified wild-Serbian Ganoderma applanatum strain BGS6Ap mycelium in a controlled submerged fermentation, 2021, 37, 18788181, 102166, 10.1016/j.bcab.2021.102166 | |
24. | Alireza Tajik, Hamid Reza Samadlouie, Amir Salek Farrokhi, Amir Ghasemi, Optimization of chemical conditions for metabolites production by Ganoderma lucidum using response surface methodology and investigation of antimicrobial as well as anticancer activities, 2024, 14, 1664-302X, 10.3389/fmicb.2023.1280405 | |
25. | Jianying Huang, Tao Liu, Yimin Zhang, Pengcheng Hu, Comparative study of microwave-assisted and hydrothermal hydroxyapatite synthesis from neutralization slag: Optimization, energy, and adsorption, 2024, 50, 02728842, 42157, 10.1016/j.ceramint.2024.08.059 | |
26. | Arman Hamza, Ankit Mylarapu, K. Vijay Krishna, Devarai Santhosh Kumar, An insight into the nutritional and medicinal value of edible mushrooms: A natural treasury for human health, 2024, 381, 01681656, 86, 10.1016/j.jbiotec.2023.12.014 | |
27. | Lina Dávila Giraldo, Paula Villanueva Baez, Cristian Zambrano Forero, Walter Murillo Arango, Production, Extraction, and Solubilization of Exopolysaccharides Using Submerged Cultures of Agaricomycetes, 2023, 13, 2331-8325, 10.21769/BioProtoc.4841 | |
28. | Arman Hamza, Abdul Khalad, Devarai Santhosh Kumar, Enhanced production of mycelium biomass and exopolysaccharides of Pleurotus ostreatus by integrating response surface methodology and artificial neural network, 2024, 399, 09608524, 130577, 10.1016/j.biortech.2024.130577 | |
29. | Zarimah Mohd Hanafiah, Ammar Radzi Azmi, Wan Abd Al Qadr Imad Wan-Mohtar, Fabrizio Olivito, Giovanni Golemme, Zul Ilham, Adi Ainurzaman Jamaludin, Nadzmin Razali, Sarina Abdul Halim-Lim, Wan Hanna Melini Wan Mohtar, Water Quality Assessment and Decolourisation of Contaminated Ex-Mining Lake Water Using Bioreactor Dye-Eating Fungus (BioDeF) System: A Real Case Study, 2024, 12, 2305-6304, 60, 10.3390/toxics12010060 | |
30. | Khalid Sayed, Wan Hanna Melini Wan Mohtar, Zarimah Mohd Hanafiah, Wan Abd Al Qadr Imad Wan-Mohtar, Teh Sabariah Binti Abd Manan, Sarah Athirah Binti Mohamad Sharif, Simultaneous enhanced removal of pharmaceuticals and hormone from wastewaters using series combinations of ultra-violet irradiation, bioremediation, and adsorption technologies, 2024, 57, 22147144, 104589, 10.1016/j.jwpe.2023.104589 | |
31. | Teik Chee Lim, Zul Ilham, Wan Abd Al Qadr Imad Wan-Mohtar, Production of Ganodiesel from the biomass of Ganoderma lucidum in air-L-shaped bioreactor (ALSB), 2024, 10, 24058440, e35170, 10.1016/j.heliyon.2024.e35170 | |
32. | Niketan Deshmukh, Lakshmi Bhaskaran, Optimization of cultural and nutritional conditions to enhance mycelial biomass of Cordyceps militaris using statistical approach, 2024, 55, 1517-8382, 235, 10.1007/s42770-023-01222-9 | |
33. | Zarimah Mohd Hanafiah, Wan Hanna Melini Wan Mohtar, Wan Abd Al Qadr Imad Wan-Mohtar, Aziza Sultana Bithi, Rosiah Rohani, Antonius Indarto, Zaher Mundher Yaseen, Suraya Sharil, Teh Sabariah Binti Abdul Manan, Removal of pharmaceutical compounds and toxicology study in wastewater using Malaysian fungal Ganoderma lucidum, 2024, 358, 00456535, 142209, 10.1016/j.chemosphere.2024.142209 | |
34. | Zarimah Mohd Hanafiah, Anggita Rahmi Hafsari, Malini Elango, Zul Ilham, Febri Doni, Wan Hanna Melini Wan Mohtar, Yusufjon Gafforov, Yong Jie Wong, Khairul Nizam Abdul Maulud, Nor Hidayah Ismail, Mohd Yusmiaidil Putera Mohd Yusof, Wan Abd Al Qadr Imad Wan-Mohtar, Removal of cyanobacterial harmful algal blooms (HABs) from contaminated local park lake using Ganoderma lucidum mycelial pellets, 2025, 11, 24058440, e41205, 10.1016/j.heliyon.2024.e41205 |
Independent variables | Range and levels |
||
−1 | 0 | 1 | |
Initial pH | 4 | 5 | 6 |
Glucose (g/L) | 10 | 30 | 50 |
Agitation (rpm) | 90 | 100 | 110 |
Run No. | Variables |
Responses |
||||||||
Initial pH | Glucose (g/L) | Agitation (rpm) | Biomass (DCW g/L) |
EPS (g/L) |
IPS (g/L) |
|||||
Actual | Predicted | Actual | Predicted | Actual | Predicted | |||||
1 | 4 | 10 | 90 | 4.6 | 4.52 | 2.2 | 2.31 | 1.2 | 1.28 | |
2 | 6 | 50 | 110 | 3.8 | 3.92 | 1.2 | 1.13 | 0.2 | 0.14 | |
3 | 6 | 50 | 90 | 3.1 | 2.97 | 0.9 | 0.91 | 0.2 | 0.25 | |
4 | 5 | 30 | 100 | 4.1 | 4.12 | 1.1 | 1.31 | 0.8 | 0.85 | |
5 | 5 | 30 | 90 | 3.9 | 3.6 | 1.3 | 1.15 | 0.7 | 0.73 | |
6 | 5 | 50 | 100 | 3.8 | 3.96 | 1 | 0.91 | 0.9 | 0.83 | |
7 | 5 | 30 | 100 | 4.2 | 4.12 | 1.4 | 1.31 | 0.9 | 0.85 | |
8 | 4 | 50 | 110 | 5.1 | 4.79 | 2.1 | 2.18 | 1.4 | 1.53 | |
9 | 5 | 30 | 100 | 3.8 | 4.12 | 1 | 1.31 | 0.9 | 0.85 | |
10 | 4 | 50 | 90 | 4.9 | 5.04 | 1.9 | 1.96 | 1.5 | 1.44 | |
11 | 5 | 30 | 100 | 3.9 | 4.12 | 1.3 | 1.31 | 0.7 | 0.85 | |
12 | 5 | 30 | 110 | 4.2 | 4.3 | 1.4 | 1.37 | 0.9 | 0.77 | |
13 | 5 | 30 | 100 | 4.2 | 4.12 | 1.5 | 1.31 | 0.8 | 0.85 | |
14 | 5 | 30 | 100 | 4.1 | 4.12 | 1.2 | 1.31 | 0.8 | 0.85 | |
15 | 4 | 30 | 100 | 5.2 | 5.26 | 2.9 | 2.61 | 1.7 | 1.57 | |
16 | 6 | 10 | 110 | 4.1 | 4 | 1.2 | 1.18 | 0.1 | 0.18 | |
17 | 6 | 30 | 100 | 4 | 3.74 | 1.3 | 1.41 | 0.3 | 0.33 | |
18 | 4 | 10 | 110 | 4.8 | 4.97 | 2.5 | 2.53 | 1.5 | 1.47 | |
19 | 5 | 10 | 100 | 4.1 | 3.74 | 1.2 | 1.11 | 0.8 | 0.77 | |
20 | 6 | 10 | 90 | 2 | 2.35 | 1 | 0.96 | 0.3 | 0.19 |
Source | Sum of Squares | DF | Mean Square | F Value | Prob > F | |
Model | 8.588136364 | 9 | 0.954237374 | 11.90767001 | 0.0003* | significant |
A: pH | 5.776 | 1 | 5.776 | 72.07714124 | <0.0001* | significant |
B: Glucose | 0.121 | 1 | 0.121 | 1.509926262 | 0.2473 | |
C: Agitation | 1.225 | 1 | 1.225 | 15.28644356 | 0.0029* | significant |
A2 | 0.410511364 | 1 | 0.410511364 | 5.122660238 | 0.0471* | significant |
B2 | 0.191136364 | 1 | 0.191136364 | 2.385138968 | 0.1535 | |
C2 | 0.073636364 | 1 | 0.073636364 | 0.918888259 | 0.3604 | |
AB | 0.005 | 1 | 0.005 | 0.062393647 | 0.8078 | |
AC | 0.72 | 1 | 0.72 | 8.984685196 | 0.0134* | significant |
BC | 0.245 | 1 | 0.245 | 3.057288712 | 0.1109 | |
Residual | 0.801363636 | 10 | 0.080136364 | |||
Lack of Fit | 0.666363636 | 5 | 0.133272727 | 4.936026936 | 0.0522 | not significant |
Pure Error | 0.135 | 5 | 0.027 | |||
Cor Total | 9.3895 | 19 | ||||
Std. Dev. = 0.28308366896796 | R2 = 0.91465321514845 | Adeq Precision = 14.52399050576 | ||||
Mean = 4.095 | Adjusted R2 = 0.83784110878205 |
* Significant value.
Source | Sum of Squares | DF | Mean Square | F Value | Prob > F | |
Model | 5.364181818 | 9 | 0.596020202 | 16.20420717 | <0.0001* | significant |
A: pH | 3.6 | 1 | 3.6 | 97.8744439 | <0.0001* | significant |
B: Glucose | 0.1 | 1 | 0.1 | 2.718734553 | 0.1302 | |
C: Agitation | 0.121 | 1 | 0.121 | 3.289668809 | 0.0998 | |
A2 | 1.330056818 | 1 | 1.330056818 | 36.16071429 | 0.0001* | significant |
B2 | 0.255056818 | 1 | 0.255056818 | 6.934317845 | 0.0250* | significant |
C2 | 0.008181818 | 1 | 0.008181818 | 0.222441918 | 0.6473 | |
AB | 0.045 | 1 | 0.045 | 1.223430549 | 0.2946 | |
AC | 0 | 1 | 0 | 0 | 1.0000 | |
BC | 0 | 1 | 0 | 0 | 1.0000 | |
Residual | 0.367818182 | 10 | 0.036781818 | |||
Lack of Fit | 0.192818182 | 5 | 0.038563636 | 1.101818182 | 0.4589 | not significant |
Pure Error | 0.175 | 5 | 0.035 | |||
Cor Total | 5.732 | 19 | ||||
Std. Dev. = 0.19178586543804 | R2 = 0.93583074287889 | Adeq Precision = 12.53566341056 | ||||
Mean = 1.48 | Adjusted R2 = 0.8780784114699 |
* Significant value.
Source | Sum of Squares | DF | Mean Square | F Value | Prob > F | |
Model | 3.935181818 | 9 | 0.437242424 | 34.47789725 | <0.0001* | significant |
A: pH | 3.844 | 1 | 3.844 | 303.1111111 | <0.0001* | significant |
B: Glucose | 0.009 | 1 | 0.009 | 0.709677419 | 0.4192 | |
C: Agitation | 0.004 | 1 | 0.004 | 0.315412186 | 0.5867 | |
A2 | 0.030056818 | 1 | 0.030056818 | 2.370071685 | 0.1547 | |
B2 | 0.005681818 | 1 | 0.005681818 | 0.448028674 | 0.5184 | |
C2 | 0.025056818 | 1 | 0.025056818 | 1.975806452 | 0.1901 | |
AB | 0.005 | 1 | 0.005 | 0.394265233 | 0.5441 | |
AC | 0.02 | 1 | 0.02 | 1.577060932 | 0.2377 | |
BC | 0.005 | 1 | 0.005 | 0.394265233 | 0.5441 | |
Residual | 0.126818182 | 10 | 0.012681818 | |||
Lack of Fit | 0.098484848 | 5 | 0.01969697 | 3.475935829 | 0.0989 | not significant |
Pure Error | 0.028333333 | 5 | 0.005666667 | |||
Cor Total | 4.062 | 19 | ||||
Std. Dev. = 0.11261357902943 | R2 = 0.96877937424466 | Adeq Precision = 17.969511850411 | ||||
Mean = 0.83 | Adjusted R2 = 0.94068081106486 |
* Significant value.
Run | Variables |
Response |
||||
pH | Glucose | Agitation | Biomass (DCW g/L) | EPS (g/L) | IPS (g/L) | |
Biomass | 4.01 | 32.09 | 102.45 | 5.12 ± 0.5 | − | − |
EPS | 4 | 24.25 | 110 | − | 2.49 ± 0.8 | − |
IPS | 4 | 40.43 | 103 | − | − | 1.52 ± 0.4 |
Biomass + EPS | 4 | 24.75 | 107.58 | 5.11 ± 0.4 | 2.57 ± 0.7 | − |
Biomass + IPS | 4 | 40.45 | 102.95 | 5.13 ± 0.5 | − | 1.57 ± 0.3 |
EPS + IPS | 4 | 26.5 | 105.92 | − | 2.62 ± 0.4 | 1.52 ± 0.6 |
Biomass + EPS + IPS | 4 | 26.5 | 100 | 5.19 ± 0.6 | 2.64 ± 0.6 | 1.52 ± 0.4 |
Optimization method | Cultivation mode | Initial pH | Glucose concentration (g/L) | Agitation (rpm) | EPS (g/L) | IPS (g/L) | Biomass (g/L) | Reference |
Response surface methodology | Shake Flask | 4 | 26.5 | 100 | 2.64 | 1.52 | 5.19 | Current study |
Taguchi's orthogonal array | Shake Flask | 6.5 | 12.1 | 160 | 0.420 | NA | 18.70 | [23] |
Orthogonal matrix | Shake Flask | − | 50 | 150 | 1.723 | NA | 7.235 | [24] |
*NA = Not available, EPS = Exopolysaccharide, IPS = Intracellular polysaccharide.
Independent variables | Range and levels |
||
−1 | 0 | 1 | |
Initial pH | 4 | 5 | 6 |
Glucose (g/L) | 10 | 30 | 50 |
Agitation (rpm) | 90 | 100 | 110 |
Run No. | Variables |
Responses |
||||||||
Initial pH | Glucose (g/L) | Agitation (rpm) | Biomass (DCW g/L) |
EPS (g/L) |
IPS (g/L) |
|||||
Actual | Predicted | Actual | Predicted | Actual | Predicted | |||||
1 | 4 | 10 | 90 | 4.6 | 4.52 | 2.2 | 2.31 | 1.2 | 1.28 | |
2 | 6 | 50 | 110 | 3.8 | 3.92 | 1.2 | 1.13 | 0.2 | 0.14 | |
3 | 6 | 50 | 90 | 3.1 | 2.97 | 0.9 | 0.91 | 0.2 | 0.25 | |
4 | 5 | 30 | 100 | 4.1 | 4.12 | 1.1 | 1.31 | 0.8 | 0.85 | |
5 | 5 | 30 | 90 | 3.9 | 3.6 | 1.3 | 1.15 | 0.7 | 0.73 | |
6 | 5 | 50 | 100 | 3.8 | 3.96 | 1 | 0.91 | 0.9 | 0.83 | |
7 | 5 | 30 | 100 | 4.2 | 4.12 | 1.4 | 1.31 | 0.9 | 0.85 | |
8 | 4 | 50 | 110 | 5.1 | 4.79 | 2.1 | 2.18 | 1.4 | 1.53 | |
9 | 5 | 30 | 100 | 3.8 | 4.12 | 1 | 1.31 | 0.9 | 0.85 | |
10 | 4 | 50 | 90 | 4.9 | 5.04 | 1.9 | 1.96 | 1.5 | 1.44 | |
11 | 5 | 30 | 100 | 3.9 | 4.12 | 1.3 | 1.31 | 0.7 | 0.85 | |
12 | 5 | 30 | 110 | 4.2 | 4.3 | 1.4 | 1.37 | 0.9 | 0.77 | |
13 | 5 | 30 | 100 | 4.2 | 4.12 | 1.5 | 1.31 | 0.8 | 0.85 | |
14 | 5 | 30 | 100 | 4.1 | 4.12 | 1.2 | 1.31 | 0.8 | 0.85 | |
15 | 4 | 30 | 100 | 5.2 | 5.26 | 2.9 | 2.61 | 1.7 | 1.57 | |
16 | 6 | 10 | 110 | 4.1 | 4 | 1.2 | 1.18 | 0.1 | 0.18 | |
17 | 6 | 30 | 100 | 4 | 3.74 | 1.3 | 1.41 | 0.3 | 0.33 | |
18 | 4 | 10 | 110 | 4.8 | 4.97 | 2.5 | 2.53 | 1.5 | 1.47 | |
19 | 5 | 10 | 100 | 4.1 | 3.74 | 1.2 | 1.11 | 0.8 | 0.77 | |
20 | 6 | 10 | 90 | 2 | 2.35 | 1 | 0.96 | 0.3 | 0.19 |
Source | Sum of Squares | DF | Mean Square | F Value | Prob > F | |
Model | 8.588136364 | 9 | 0.954237374 | 11.90767001 | 0.0003* | significant |
A: pH | 5.776 | 1 | 5.776 | 72.07714124 | <0.0001* | significant |
B: Glucose | 0.121 | 1 | 0.121 | 1.509926262 | 0.2473 | |
C: Agitation | 1.225 | 1 | 1.225 | 15.28644356 | 0.0029* | significant |
A2 | 0.410511364 | 1 | 0.410511364 | 5.122660238 | 0.0471* | significant |
B2 | 0.191136364 | 1 | 0.191136364 | 2.385138968 | 0.1535 | |
C2 | 0.073636364 | 1 | 0.073636364 | 0.918888259 | 0.3604 | |
AB | 0.005 | 1 | 0.005 | 0.062393647 | 0.8078 | |
AC | 0.72 | 1 | 0.72 | 8.984685196 | 0.0134* | significant |
BC | 0.245 | 1 | 0.245 | 3.057288712 | 0.1109 | |
Residual | 0.801363636 | 10 | 0.080136364 | |||
Lack of Fit | 0.666363636 | 5 | 0.133272727 | 4.936026936 | 0.0522 | not significant |
Pure Error | 0.135 | 5 | 0.027 | |||
Cor Total | 9.3895 | 19 | ||||
Std. Dev. = 0.28308366896796 | R2 = 0.91465321514845 | Adeq Precision = 14.52399050576 | ||||
Mean = 4.095 | Adjusted R2 = 0.83784110878205 |
Source | Sum of Squares | DF | Mean Square | F Value | Prob > F | |
Model | 5.364181818 | 9 | 0.596020202 | 16.20420717 | <0.0001* | significant |
A: pH | 3.6 | 1 | 3.6 | 97.8744439 | <0.0001* | significant |
B: Glucose | 0.1 | 1 | 0.1 | 2.718734553 | 0.1302 | |
C: Agitation | 0.121 | 1 | 0.121 | 3.289668809 | 0.0998 | |
A2 | 1.330056818 | 1 | 1.330056818 | 36.16071429 | 0.0001* | significant |
B2 | 0.255056818 | 1 | 0.255056818 | 6.934317845 | 0.0250* | significant |
C2 | 0.008181818 | 1 | 0.008181818 | 0.222441918 | 0.6473 | |
AB | 0.045 | 1 | 0.045 | 1.223430549 | 0.2946 | |
AC | 0 | 1 | 0 | 0 | 1.0000 | |
BC | 0 | 1 | 0 | 0 | 1.0000 | |
Residual | 0.367818182 | 10 | 0.036781818 | |||
Lack of Fit | 0.192818182 | 5 | 0.038563636 | 1.101818182 | 0.4589 | not significant |
Pure Error | 0.175 | 5 | 0.035 | |||
Cor Total | 5.732 | 19 | ||||
Std. Dev. = 0.19178586543804 | R2 = 0.93583074287889 | Adeq Precision = 12.53566341056 | ||||
Mean = 1.48 | Adjusted R2 = 0.8780784114699 |
Source | Sum of Squares | DF | Mean Square | F Value | Prob > F | |
Model | 3.935181818 | 9 | 0.437242424 | 34.47789725 | <0.0001* | significant |
A: pH | 3.844 | 1 | 3.844 | 303.1111111 | <0.0001* | significant |
B: Glucose | 0.009 | 1 | 0.009 | 0.709677419 | 0.4192 | |
C: Agitation | 0.004 | 1 | 0.004 | 0.315412186 | 0.5867 | |
A2 | 0.030056818 | 1 | 0.030056818 | 2.370071685 | 0.1547 | |
B2 | 0.005681818 | 1 | 0.005681818 | 0.448028674 | 0.5184 | |
C2 | 0.025056818 | 1 | 0.025056818 | 1.975806452 | 0.1901 | |
AB | 0.005 | 1 | 0.005 | 0.394265233 | 0.5441 | |
AC | 0.02 | 1 | 0.02 | 1.577060932 | 0.2377 | |
BC | 0.005 | 1 | 0.005 | 0.394265233 | 0.5441 | |
Residual | 0.126818182 | 10 | 0.012681818 | |||
Lack of Fit | 0.098484848 | 5 | 0.01969697 | 3.475935829 | 0.0989 | not significant |
Pure Error | 0.028333333 | 5 | 0.005666667 | |||
Cor Total | 4.062 | 19 | ||||
Std. Dev. = 0.11261357902943 | R2 = 0.96877937424466 | Adeq Precision = 17.969511850411 | ||||
Mean = 0.83 | Adjusted R2 = 0.94068081106486 |
Run | Variables |
Response |
||||
pH | Glucose | Agitation | Biomass (DCW g/L) | EPS (g/L) | IPS (g/L) | |
Biomass | 4.01 | 32.09 | 102.45 | 5.12 ± 0.5 | − | − |
EPS | 4 | 24.25 | 110 | − | 2.49 ± 0.8 | − |
IPS | 4 | 40.43 | 103 | − | − | 1.52 ± 0.4 |
Biomass + EPS | 4 | 24.75 | 107.58 | 5.11 ± 0.4 | 2.57 ± 0.7 | − |
Biomass + IPS | 4 | 40.45 | 102.95 | 5.13 ± 0.5 | − | 1.57 ± 0.3 |
EPS + IPS | 4 | 26.5 | 105.92 | − | 2.62 ± 0.4 | 1.52 ± 0.6 |
Biomass + EPS + IPS | 4 | 26.5 | 100 | 5.19 ± 0.6 | 2.64 ± 0.6 | 1.52 ± 0.4 |
Optimization method | Cultivation mode | Initial pH | Glucose concentration (g/L) | Agitation (rpm) | EPS (g/L) | IPS (g/L) | Biomass (g/L) | Reference |
Response surface methodology | Shake Flask | 4 | 26.5 | 100 | 2.64 | 1.52 | 5.19 | Current study |
Taguchi's orthogonal array | Shake Flask | 6.5 | 12.1 | 160 | 0.420 | NA | 18.70 | [23] |
Orthogonal matrix | Shake Flask | − | 50 | 150 | 1.723 | NA | 7.235 | [24] |