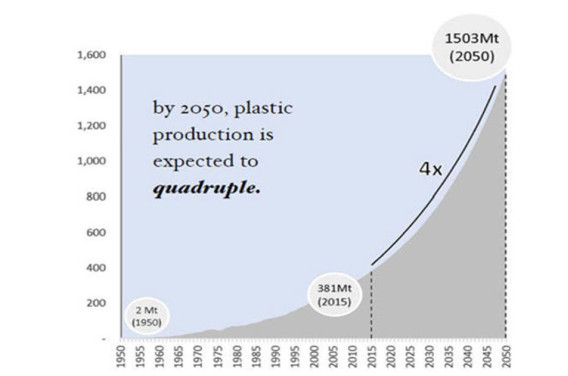
Citation: Serpil Guran. Options to feed plastic waste back into the manufacturing industry to achieve a circular carbon economy[J]. AIMS Environmental Science, 2019, 6(5): 341-355. doi: 10.3934/environsci.2019.5.341
[1] | Goshu Desalegn, Anita Tangl, Maria Fekete-Farkas . Greening through taxation: assessing the potential opportunities and challenges of plastic products in Ethiopia. AIMS Environmental Science, 2022, 9(4): 432-443. doi: 10.3934/environsci.2022026 |
[2] | Ruchira R N Ghormare, Sana Fatima, Priya Grover, Nidhi Phutela, Vinay Kandpal, Ernesto D. R. Santibanez Gonzalez . Exploring the paradigm shift towards sustainability: A systematic literature review on circular economy and eco-innovation. AIMS Environmental Science, 2024, 11(6): 940-959. doi: 10.3934/environsci.2024047 |
[3] | Manfred Kircher . The bioeconomy needs economic, ecological and social sustainability. AIMS Environmental Science, 2022, 9(1): 33-50. doi: 10.3934/environsci.2022003 |
[4] | PA Stapleton . Toxicological considerations of nano-sized plastics. AIMS Environmental Science, 2019, 6(5): 367-378. doi: 10.3934/environsci.2019.5.367 |
[5] | Katharina Meixner, Mona Kubiczek, Ines Fritz . Microplastic in soil–current status in Europe with special focus on method tests with Austrian samples. AIMS Environmental Science, 2020, 7(2): 174-191. doi: 10.3934/environsci.2020011 |
[6] | R. Kajaste, P. Oinas . Plastics value chain - Abatement of greenhouse gas emissions. AIMS Environmental Science, 2021, 8(4): 371-392. doi: 10.3934/environsci.2021024 |
[7] | Atinuke Chineme, Getachew Assefa, Irene M. Herremans, Barry Wylant, Marwa Shumo, Aliceanna Shoo, Mturi James, Frida Ngalesoni, Anthony Ndjovu, Steve Mbuligwe, Mike Yhedgo . Advancing circular economy principles through wild black soldier flies. AIMS Environmental Science, 2023, 10(6): 868-893. doi: 10.3934/environsci.2023047 |
[8] | Chukwuebuka Okafor, Charles Ajaero, Christian Madu, Kingsley Agomuo, Ezekiel Abu . Implementation of circular economy principles in management of end-of-life tyres in a developing country (Nigeria). AIMS Environmental Science, 2020, 7(5): 406-433. doi: 10.3934/environsci.2020027 |
[9] | Lemuel Clark Velasco, Mary Jane Burden, Marie Joy Satiniaman, Rachelle Bea Uy, Luchin Valrian Pueblos, Reynald Gimena . Preliminary assessment of solid waste in Philippine Fabrication Laboratories. AIMS Environmental Science, 2021, 8(3): 255-267. doi: 10.3934/environsci.2021017 |
[10] | Sergey Orlov, Elena Rovenskaya, Julia Puaschunder, Willi Semmler . Green bonds, transition to a low-carbon economy, and intertemporal welfare allocation: Evidence from an extended DICE model. AIMS Environmental Science, 2024, 11(4): 628-648. doi: 10.3934/environsci.2024031 |
It is a known fact that plastics play a significant role in our lives. Medical practices became more efficient with plastic used in a multitude of medical devices, food packaging, storage and delivery are advanced as compared to packaging before plastics, automobiles are lighter and more fuel efficient because of plastic components. The factors that make plastic so convenient in our day-to-day lives is its low production cost and availability. However, plastic also creates one of the planet’s greatest environmental challenges [1]. If we assess our daily activities, we easily can name at least 10–15 or even more items made completely of plastic or with plastic parts and components, including our smart phones or clever appliances in our homes. Currently, the production, consumption and disposal of plastics not only harms the environment, but also create economic problems, since the majority of current approaches do not support closed-loop, low-carbon processes.
Global annual fossil-based plastics production increased from 2 million tons in 1950 to 381million tons in 2015. If this business-as-usual trend continues, plastics production is expected to quadruple (Figure 1) and it is estimated that the total volume of plastics produced will reach 34,000 million tons (Mt) [2,3,4].
Communities generally have dealt with the plastic waste disposal problem by introducing single stream recycling practices. This pathway also included shipping a significant portion of plastic waste to China over the past 25 years. In 2016, 15 million tons of plastic waste was traded globally, with China being the top importer and the US the largest exporter. However, China in early 2018 cancelled its global imports, requiring that the plastics they import be completely uncontaminated. This decision was most likely the result of single stream recycling practices, which comingle paper, glass, metals and plastics together to be sorted at the recycling facility. Experts agree that single stream recycling undeniably increased the quantity of recycled materials, but reduced the quality, resulting in a contaminated supply and reduced economic viability of recycling operations [5]. In addition to contamination problems, the projected plastic waste generation rates also confirm that current inefficient recycling can no longer be considered the primary solution to this growing waste stream. It is reported that since 1950, only 9% of discarded plastics have been recycled. Globally, 58% of plastic waste is discarded or landfilled and only 18% was recycled in 2015 [4,6]. The 2016 statistics for EU reports [7] that recycling rate is 31.1% as compared with only 9.1% post-consumer plastics collected for recycling in the US [8]. These numbers do not include the leakage into the natural environment, i.e. waterways and oceans (Table 1) [8].
Waste material | Weight generated Mt | Weight recycled Mt | Weight incinerated for energy Mt | Weight landfilled Mt | Recycled % |
Incinerated % |
Landfilled % |
Plastics | 34.50 | 3.14 | 5.35 | 26.01 | 9.1 | 15.5 | 75.4 |
Why are recycling rates so low? Because people voluntarily recycle, decide on what to put into their recycling bins because of single stream recycling and there are no incentives for plastics recycling [3]. In addition, recycling lighter weight plastics that contain additives is unattractive and economically unfeasible. Because fossil feedstocks are abundant and available at low cost, fossil-based virgin plastic prices are also low and outcompete recycled plastics. In addition, there are no mechanisms in place to ensure manufacturers use recycled plastic content in their products. So, what happens if the plastic waste is not recycled? They leave the economy – plastic is manufactured, used, and thrown away (Figure 2a, b).
The current throwaway culture treats plastics as disposable materials rather than a valuable resource to be valorized. The linear economy pathway of material movement is rooted in exponentially increasing resource consumption, excessive energy use, erosion of ecosystems, and massive amount of waste generation [9,10,11]. As urbanization increases, the global solid waste problem, and consequently the plastic waste problem, is expected to grow if current waste generation is not minimized and current linear waste disposal practices and inefficient recycling pathways do not change.
Non-recycled plastics end up in landfills, dumpsites, incinerators, or leak into waterways and oceans where they will remain for hundreds or even thousands of years. The United Nations Environment Program reports that more than 8 million tons of plastics leak into lakes and oceans each year - equal to dumping a garbage truck of plastic every minute [1]. If the current trend continues, the rate of dumping is expected to increase to two trucks per minute by 2030.
Beginning with the industrial revolution till now, overreliance on fossil fuels, industrial forms of agriculture and overall human activities have reached to a level of environmental conditions that could result irreversible results on planetary boundaries [12]. Literature identified nine processes that define the planetary boundaries: climate change; rate of biodiversity loss (terrestrial and marine); interference with nitrogen and phosphorus cycles; stratospheric ozone depletion; ocean acidification; global fresh water use; change in land use; chemical pollution; and atmospheric aerosol loading [13]. The planetary boundaries define the safe operating space for humanity and global societal development [12]. Research stated that three of the earth-system processes – climate change, rate of biodiversity loss and interference with the nitrogen cycle – have already transgressed their boundaries. In addition, these boundaries are tightly intertwined and we should recognize that improving one will positively impact others. However, if one boundary is transgressed, other boundaries are also under serious risk. It is also proven that overreliance on fossil fuels, linear consumption of resources, unsustainable land and water management and industrial form of agriculture all lead to damaging the ecosystem and overall planetary boundaries [12]. In air, land and water addition pollution and waste accumulation are part of the problem and urgent and paradigm shift is needed.
Currently, the plastic industry consumes 6% of world total oil production. If current production practices do not change, oil consumption for manufacture of plastics is estimated to reach 20% of oil production by 2050. This rate would contribute not only 15% of the global annual carbon budget but also negatively impact other planetary boundaries. In the US alone, 264 new-plastics related facilities and expansions are currently planned and/or built to use fracked natural gas [2,3,14,15].
The global economy produced 407Mt of plastics in 2015. Conventional (fossil-based) plastics production emitted 1.8GtCO2e, which is 3.8% of the 47GtCO2e emitted that year. If the current demand trend of 4% annual growth rate for plastics consumption continues, annual plastics production is expected to reach 1606Mt by 2050 with life-cycle GHG emissions of 6.5GtCO2e in 2050 [6]. In order to reverse this trend, we need to find options within the circular carbon economy concept to feed plastic waste into plastics production.
The emerging Circular Carbon Economy Concept (CCEC) refers to an “economic system based on reuse of products and raw materials and the restorative capacity of natural resources.” CCEC also attempts to minimize value destruction in the overall system and maximize value creation. The goal is to counteract depletion of natural resources, reduce GHG emissions and use of hazardous substances, eliminate waste, and make a complete transition to renewable and sustainable energy supplies” [16]. Therefore, promoting combined understanding of circularity and a lower-carbon economy as “circular carbon economy” and transforming the linear make-it /use-it/dispose-it pathway to a circular resource recovery pathway can be an effective pathway for mitigating climate change within a lower-carbon economy. The circularity approach redefines waste as a “resource” and feeds the resource back into the economy efficiently (Figure 3).
CCEC promotes transforming waste practices into circular pathways to achieve natural resource conservation and economic growth, while avoiding environmental degradation. Currently, worldwide waste is generally managed by landfilling, recovery through recycling and by incineration where waste energy is recovered at the waste-to-energy facilities. Some developing and undeveloped countries also still practice waste dumping and open burning. Municipal solid waste is a complex heterogeneous mix of materials and a single technology treatment of landfilling or incineration approaches are considered inefficient when it comes to climate change mitigation and a circular carbon economy approach. In order to transform these practices for environmentally better, economically feasible and socially acceptable new pathways and efficiently valorize waste materials to feed them back into the economy depends on the quality of the waste and the degree of the cross contamination.
Source separation of the waste components is essential to increase the efficiency of valorization processes. In order to feed waste components, plastic waste in this case, as feedstocks into a circular carbon economy model, there is a need for new “circular carbon systems” that employ new innovative technologies. An approach that couples the components of waste with emerging suitable conversion technologies to produce end-products is essential.
Recent assessment of global life-cycle emissions of conventional plastics found that the End-of-Life (EoL) stage accounted for 9% of total life-cycle emissions of plastics (Figure 4) [4]. This study considered incineration and landfilling of plastic waste as the dominant EoL options.
Zheng and Suh (2019) reported that landfilling generated the least GHG emissions despite handling 58% of the global plastics waste generated in 2015. The study also underlined that although recycling practices generated 49MtCO2e, and if the displacement of carbon intensive virgin polymer production by recyclates of carbon intensive virgin polymer production is considered, the GHG emissions of recycling would go down to negative 67 MtCO2e, and the total emissions from the EoL stage would be reduced from 161 MtCO2e to 45 MtCO2e. In this case, the total global life-cycle GHG emissions of plastics become 1.7 GtCO2e, or 3.5% of the global annual GHG emissions [4]. This displacement scenario only considered the recycled part of EoL in the plastics life cycle. If the scenario of displacement had considered all plastic waste components that were landfilled and incinerated, the amount of GHG reduction would have been much larger. In addition, the scenario did not specify whether the EoL stage includes global plastic waste leakage into waterways and oceans. Utilizing plastic waste by returning them to a circular carbon system for new plastics and products instead of landfilling and incinerating would have further positively reduced the GHG carbon footprint of plastics.
Mechanical recycling is a method by which waste materials are recycled into “new” (secondary) raw materials without changing the basic structure of the material. It is also known as material recycling, material recovery or, related to plastics, back-to-plastics recycling. In plastics recycling, the handling of pre-consumer (post-industrial) material and post-consumer material need to be distinguished. In principle, the technology of mechanical recycling is applicable to both bio-based conventional plastic and to most grades of biodegradable plastics [16]. Mechanical recycling is a well-established technology for the material recovery of conventional plastics, such as polyethylene (PE), polyethylene terephthalate (PET), polypropylene (PP) and polystyrene (PS). Its main advantage is that part of the resources consumed for the production of the plastic materials is not wasted, but preserved for a use in the same, similar or different applications. Products made with biobased feedstocks (drop-ins) of conventional polymers can also be recycled with mechanical recycling. Other innovative biopolymers can also be recovered with mechanical recycling especially when sufficient volumes of homogenous waste material streams are available, either through separate collection or through sorting routines [17].
In one study, researchers found that 69.74% of the plastic waste was not suitable for mechanical recycling. However, it is not stated whether this is the result of local waste generation or the recycling methodology, i.e. certain plastics not suitable for further utilization or contamination by food or other materials [18]. In addition, waste streams contain a non-recycled plastic waste portion due to throwaway culture. For understanding the real reason, localized and regional assessments should be based on real time data. One study of products manufactured from recycled plastics shows that recycled plastics can serve as valuable feedstocks (Table 2) [19]. Displacing fossil-based virgin plastics especially polypropylene composites with rPC resulted in higher environmental benefits [19].
Products | Applications |
rPE | Agricultural seeding trays |
rPE | Horticultural applications: water proof materials |
rPP | Turnover boxes, pallets and folding containers |
rPP | Wire reels |
rPC | Suitcase accessories; handles, wheels and pull rods |
rPC | Interior automobile trims: air-condition ducts, doorsills, door panels, engine covers, |
fenders and mud-boards, wheel covers, bumpers | |
rPP | Household tools |
rPE: Recycled Polyethylene; rPP: recycled Polypropylene; rPC: recycled polypropylene composite |
Despite the recycling potential of PE, PET, PP and PS, polyvinyl chloride (PVC) appears to be one of the most difficult to recycle and also environmentally hazardous polymer because of the specific characteristics. It is reported that PVC is one the most commonly used thermoplastic materials compared to the worldwide polymer consumption. PVC is the world’s third widely produced synthetic polymer after PE and PP. It’s low cost, strong performance and application in wide range of products made PVC a universal polymer [20]. It is widely used in industries including architecture and building, electronics, chemical engineering, packaging, transportation [36]. The efficient performance of PVC products have increased the utilization of this polymer in buildings, mainly in exterior applications, such as window profiles, cladding structure and sidings (Table 3) [20,21].
Application rigid PVC applications | Share of the components (weight%) | ||||
PVC polymer | Plasticizer | Stabilizer | Filter | Others | |
Pipes | 98 | - | 1–2 | - | |
Window profiles | 85 | - | 3 | 4 | 8 |
Other profiles | 90 | - | 3 | 6 | 1 |
Rigid films | 95 | - | - | - | 5 |
Flexible PVC applications | |||||
Cable insulation | 42 | 23 | 2 | 33 | - |
Flooring | 42 | 15 | 2 | 41 | 0 |
Flooring (past upper layer) | 65 | 32 | 1 | - | 2 |
Flooring (paste, inside material) | 35 | 25 | 1 | 40 | - |
Synthetic leather | 53 | 40 | 1 | 5 | 1 |
Furniture films | 75 | 10 | 2 | 5 | 8 |
Leisure articles | 60 | 30 | 2 | 5 | 3 |
In plastics recycling, recyclates must comply with a set of technical specifications for them to be used in new products. Technical specifications included composition and contamination of recyclates. Because, PVC is a compound material which does not only consists of polymer PVC alone, but also includes variety of additives such as stabilizers to eliminate degradation of PVC, plasticizers to make some PVC flexible, fillers, pigments, impact modifiers and processing agents, mechanical recycling has been challenging technically and economically [20,21,22,23]. In addition, composition of the PVC compounds may differ depending on the producer or processor. Furthermore, the composition of the PVC compounds for a specific application has changed in time due to technological changes, e.g. today window profiles are produced from different PVC compounds than window profiles 20 years ago. Despite challenges PVC recycling is advancing especially in Europe. There are several conventional and non-conventional PVC recycling programs emerging such as Vinylplus, Vinlyloop, RecoVinyl that PVC through mechanical recycling is utilized in several products from plastic-wood to light concrete [24].
Another plastic group that pose challenges are called black plastics. Because, efficient recovery and recycling of plastics rely on sorting into monopolymeric fractions that can be performed economically, reliably, safely and automatically. Currently available technology is based on spectral signatures derived from near infra-red (NIR) reflectance spectroscopy where plastics are identified according to stretching vibration modes of CH, CH2 and CH3 groups. Plastics colored with carbon black and other black pigments, however, exhibit very low reflectance of light in the NIR spectral region and the signal-to-noise ratio of present sensors is insufficient to allow classification according to polymer type identification. If inefficiently to carry restricted and hazardous substances into the recyclate, including brominated flame retardants (BFRs), Sb, a flame retardant synergist, and the heavy metals, Cd, Cr, Hg and Pb. If reusing these materials in food packaging occurs serious health related issues can arise. Therefore tracing, collection, sorting and reusing these polymers should be strictly regulated through close monitoring and reporting [25,26].
In addition to mechanical plastics recycling approach, waste plastics can be integrated into the economy through thermochemical conversion technologies, such as pyrolysis and gasification, to produce recycled chemical intermediates and building blocks that can displace virgin fossil feedstocks [27]. A life-cycle assessment of waste treatment technologies study found that when mechanical recycling of plastics cannot fully substitute for virgin plastics, thermochemical recycling is a better option [28].
Pyrolysis- Pyrolysis is a thermochemical conversion process in the absence of added oxygen or other oxidizers at a relatively lower temperature compared to incineration temperatures. This process can be used to recover materials and energy in the form of chemicals and fuels. Pyrolysis technology at higher temperatures enables the recovery of monomer from polymers [29]. Mixed plastics pyrolysis with or without catalysts can utilize waste plastics as a feedstock for BTX (Benzene, Toluene and Xylene) aromatics, which are fundamental petrochemicals and serve as building blocks of many chemicals and products. The technology needs to be optimized, scaled up, and commercially tested [30,31,32,33]. Although pyrolysis is reported to be one of the efficient recycling methods, the presence of PVC by itself or as mixed with other plastics is undesirable in the pyrolysis conversion since PVC contains chlorine compound as high as 56.7% that will result chlorine presence in the pyrolysis products will reduce the market potential and value of gaseous, liquid and solid end products [34].
Gasification- Gasification is a partial oxidation technology to convert feedstocks into syngas, which is a mixture of H2 and CO. Syngas can further be processed into chemicals and fuels. It is a key interface technology to convert any carbon containing feed material into syngas required for any product synthesis. Through synthesis, platform chemicals such as methanol (MeOH) can be produced from syngas. Subsequent derivatives, such as olefins that form the basic raw material for production of a wide range of chemical products, can be synthesized via the methanol-to-olefins process [35,36]. This approach ensures that with a gasification pathway, waste plastics can be converted into new chemical products, enabling the transformation to a circular carbon economy [37].
Similar to pyrolysis, gasification technology applications are not widespread because of the cheap fossil fuel prices and availability of landfilling and incineration options. However, because both landfilling and incineration pathways are proven that they do not support CCEC, thermochemical conversion options should be further improved, tested and commercialized in order to integrate plastic waste back into the economy.
In addition to recovering recycled intermediates from plastic waste, an important reduction of GHG emissions can be obtained by recovering the large amounts of gasification produced excess heat. This heat source can produce steam, which can be exported to chemical plants by appropriate placement of the gasification processes adjacent to, or part of, chemical complex sites. This steam is about 70% of the steam currently produced in natural gas boilers. The reduction of natural gas consumption in steam boiler contributes to about 20% to 30% of the total GHG emission reduction potential, which highlights the suitability of the process [38,39,40].
To date, the inefficient integration of pre- and post-consumer plastic waste into the economy is mainly due to economic reasons. Because fossil-based virgin plastics are cheap and abundant, the plastics industry has chosen to utilize virgin feedstocks instead of recycled ones. Further, because the plastics have dyes and additives, and/or other contamination caused by either inefficient collection or packaging waste, reutilizing of plastics was not preferred by the industry.
In addition, GHG emissions inventories generally report that emissions related to waste management globally may not be as high as other GHG emitting sources, i.e. electricity generation from fossil fuel combustion or transportation fuels. This creates the impression that innovative approaches in the waste management sector may not contribute substantially to climate change mitigation efforts. European Greenhouse emission statistics report that the waste sector contributed approximately 3% of the total global GHG emissions in 2016. Similarly, US waste related GHG emissions reported by USEPA are around 2% of total emissions. These figures grossly underestimate the potential contribution of improved waste and resource management to GHG mitigation [41]. The underestimation of potential contribution of improvement is also similar to plastic waste disposal. As mentioned, researchers estimated plastics EoL GHG emission contribution 9%, as compared to other stages of plastics production. [5]. However, with improvement in the EoL recycling they determined the potential for further substantial reductions in GHG emissions. In addition, if landfilling and incinerating portions of plastics were innovatively reintegrated back into the economy, benefits would be even higher. Especially, while recovering chemical intermediates from plastic waste via gasification or pyrolysis, recovering energy from the system would reduce the GHG emissions even further. Therefore, current waste management policies which are still not driven by climate concerns, should be reevaluated. Research indicates that the potential contribution of waste prevention and management to GHG abatement could be far greater than the total reported emissions from the waste parts of the inventories [42,43]. The Global Waste Management Outlook (GWMO) stated that the potential impact of improved waste and resource management on reducing GHG emissions could be as high as 15–20% [41,44].
In order to integrate plastic wastes into the circular carbon economy, identifying barriers and near-, mid-, and long-term planning is essential. Barriers and challenges in achieving a circular approach are summarized in Table 4.
Type of challenge | |
Economic cost (up-front investment, risks) |
Assessing financial benefits of circular economy |
Assessing financial profitability | |
Structural lack of understanding & participation (business, consumer and decision makers |
Achieving exchange of information |
Defining responsibility distribution | |
Operational Complex international production & consumption supply-chains |
Redefining the infrastructure |
Strong supply chain | |
Knowledge & behavior need for knowledge and capacity for implementation need for education |
Perception of sustainability |
Behavior change | |
Technological need for innovative technologies |
New product design to absorb waste resources |
Integration into processing | |
Environmental assessment | Correct LCA of circular approaches |
need for regulations | Lack of standards, monitoring & reporting |
Economic challenges:
Because fossil carbon prices and consequently fossil-based virgin plastic prices are low integration of plastic waste into circular carbon economy has economic barriers. In order to overcome the challenges financial benefits of circular economy and potential profitability should be assessed.
Structural challenges:
Currently, there is lack of understanding the importance of circular carbon economy concept. Especially, consumers, business and regulatory decision makers should be communicated by efficient and transparent information exchange.
Operational challenges:
Currently our economy is part of complex international production & consumption supply-chains. Effective systems for tracking, collection, sorting and recycling of used plastics are needed. New approaches should be adopted internationally by avoiding regional isolated solutions when redefining stronger supply chains that include recycled plastic components.
Knowledge & behavioral challenges;
In order to achieve a paradigm shift, new knowledge and capacity is needed for implementation of efficient circular carbon economy models. Education and information sharing will be essential in schools, business, media, and governments.
Technological challenges:
Current available technologies may not be sufficient efficiently integration plastic wastes into circular carbon economy. Therefore, there is need for innovative circular carbon systems and technologies. Innovations should include new conversion technologies and new product design to absorb waste resources.
Environmental assessment challenges:
Because of complex international supply chain, traceability of plastics sources are essential when assessing the circular carbon economy benefits. Transparent information is needed for reliable Life Cycle Assessment for determining environmental footprint of improved circular carbon systems.
Lack of regulations challenges:
In achieving efficient integration of plastic wastes into circular carbon economy, reliable standards, monitoring & reporting regulations are needed for efficient results.
It is essential to create realistic short-, mid-, and long-term plans when dealing with environmental issues that involve many stakeholders, including the public, business and governmental decision makers. Planning and subsequent decision-making processes should be based on reliable data, science and innovation. Plans, decisions, set targets and results should always be transparently communicated with the stakeholders. In addition, comprehensive outreach and education should be part of the planning. The planning steps are as follows:
● Short-term planning:
– Engage decision & policy makers
– Avoid contamination in the waste streams
– Improved collection and sorting for polymers (i.e. black plastics)
– Improved labelling and traceability systems
– Enable secondary markets
– Facilitate collaboration across value chains
– Innovative thinking to reduce the leakage of plastics into the natural systems
● Mid- & long-term planning:
– Innovative thinking in creation of after-use plastics economy
– Investment in better packaging
– Policies and Intervention for decoupling plastics production from fossil feedstocks
– Funding for innovative research & Development
Transformation of inefficient linear plastic waste disposal practices and into efficient circular carbon systems is required to treat plastic waste as a resource. Integrating waste into circular carbon systems will transform current linear make-it /use-it/dispose-it pathways to circular resource recovery pathways and can also provide climate change mitigation. This transformation, if planned thoroughly, would create sustainable solutions while reducing GHG emissions.
The author claims that this paper has no conflict of interest.
[1] | Giacovelli C, UNEP: SINGLE-USE PLASTICS: A Roadmap for Sustainability, 2018. Available from: https://www.academia.edu/37294255/SINGLE-USE_PLASTICS_A_Roadmap_for_Sustainability. |
[2] | Recycling is not enough, GAIA/Zero Waste Europe, 2018. Available from: http://www.no-burn.org/wp-content/uploads/Recycling-is-Not-Enough-online-version.pdf. |
[3] | World Economic Forum, Ellen McArthur Foundation and McKinsey &Company. The New Plastics Economy-Rethinking the future of Plastics, 2016. Available from: http://www3.weforum.org/docs/WEF_The_New_Plastics_Economy.pdf. |
[4] |
Zheng J, Suh S (2019) Strategies to reduce the global carbon footprint of plastics. Nat Clim Change 9: 374–378. doi: 10.1038/s41558-019-0459-z
![]() |
[5] | Parker L, Elliott K. Plastics recycling is broken. Here's how to fix it, National Geographic, 2018. Available from: https://news.nationalgeographic.com/2018/06/china-plastic-recycling-ban-solutions-science-environment/. |
[6] | Geyer R, Jambeck JR, Law KL (2017) Production, use and fate of all plastics ever made. Sci Adv 3: el700782. |
[7] | Plastics-the facts An analysis of European plastic production, demand and waste data, Plastics Europe, (2018), Available from: https://www.plasticseurope.org/application/files/6315/4510/9658/Plastics_the_facts_2018_AF_web.pdf |
[8] | USEPA, (2018). Advancing Sustainable Materials Management: (2015) Fact Sheet-Assessing Trends in Material Generation, Recycling, Composting, Combustion with Energy Recovery and Landfilling in the United States. |
[9] |
Lahti T, Wincent J, Parida N (2018) A definition and theoretical review of circular economy, value creation, and sustainable business models: where are we now and where should research move in the future? Sustain 10: 2799–2817. doi: 10.3390/su10082799
![]() |
[10] | Kok L, Wurpel G, Ten Wolde A. Unleashing the power of the circular economy, IMSA and Circle Economy, Amsterdam, Netherlands, 2013. Available from: https://mvonederland.nl/system/files/media/unleashing_the_power_of_the_circular_economy-circle_economy.pdf. |
[11] |
Michelini G, Moraes RN, Cunha RN, et al. (2017) From linear to circular economy: PSS conducting the transition. Procedia CIRP 64: 2–6. doi: 10.1016/j.procir.2017.03.012
![]() |
[12] | Vinylplus, PVC recycling technologies, 2017. Available from: www.vinylplus.eu. |
[13] |
Steffen W, Richardson K, Rockström J, et al. (2015) Planetary boundaries: Guiding human development on a changing planet. Science 347: 1259855. doi: 10.1126/science.1259855
![]() |
[14] | How Fracked Gas, Cheap Oil and Unburnable Coal are Driving the Plastics Boom. by The Center for International Environmental Law is licensed under a Creative Commons Attribution 4.0 International License, Center for International Environmental Law, 2017. Available from: https://www.ciel.org/wp-content/uploads/2017/09/Fueling-Plastics-How-Fracked-Gas-Cheap-Oil-and-Unburnable-Coal-are-Driving-the-Plastics-Boom.pdf. |
[15] | Olivier JGJ, Schure KM, Peters JAHW (2017) Trends in Global CO2 and Total Greenhouse Gas Emissions: Summary of the 2017 Report, PBL. Netherlands Environ Assess Agen 2017: 5. |
[16] | Cramer J (2017) The raw materials transition in the Amsterdam Metropolitan are: Added value for the economy, well-being and the environment. Enviro 59:15–21. |
[17] | Mechanical Recycling Fact Sheet (2015) European Bioplastics, https://docs.european-bioplastics.org/publications/bp/EUBP_BP_Mechanical_recycling.pdf. |
[18] |
Horwart B, Mallinguh E, Fogarassy C (2018) Designing business solutions for plastic waste management to enhance circular transitions in Kenya. Sustain, 10:1664. doi: 10.3390/su10051664
![]() |
[19] | Gu F, Guo J, Zhang W, et al. (2017) From waste plastics to industrial raw materials: A life cycle assessment of mechanical plastic recycling practice based on a real-world case study. Sci Total Environ 601–602: 1192–1207 |
[20] |
Yousif E, Hasan A (2015) Photostabilization of poly(vinyl chloride) – Still on the run. J Taibah Univ Sci 9: 421–448. doi: 10.1016/j.jtusci.2014.09.007
![]() |
[21] | Cao Q, Yuan G, Yin L, et.al. (2016) Morphological characteristics of polyvinyl chloride (PVC) dechlorination during pyrolysis process: Influence of PVC content and heating rate. Waste Manage 58: 241–249. |
[22] |
Sadat-Shojai M, Bakhshandeh G (2011) Recycling of PVC wastes. Polym Degrad Stabil 96: 404–415 doi: 10.1016/j.polymdegradstab.2010.12.001
![]() |
[23] | Plinke E, Wenk N, Wolff G, et al. (2000) Mechanical recycling of PVC wastes, Study for DG XI of the European Commission (B4-3040/98/000821/MAR/E3). |
[24] | Vinylplus, PVC recycling technologies, www.vinylplus.eu. |
[25] |
Turner A (2018) Black plastics: Linear and circular economies, hazardous additives and marine pollution. Environ Int 117: 308–318. doi: 10.1016/j.envint.2018.04.036
![]() |
[26] | Samsonek J, Puype F (2013) Occurrence of brominated flame retardants in black thermo cups and selected kitchen utensils purchased on the European market. Food Ad & Contaminants. 30: 1976–1986. |
[27] | Morandin M, Heyne S, Jilvero H, et al. Thermochemical recycling of plastics for production of chemical intermediates at a Swedish chemical complex site, Proceedings The 29th International Conference on Efficiency, Cost, Optimization, Simulation and Environmental Impact of Energy Systems, 2016. Available from: http://publications.lib.chalmers.se/records/fulltext/251393/local_251393.pdf. |
[28] |
Al-Salem SM, Evangelisti S, Lettieri P (2014) Life-cycle-assessment of alternative technologies for municipal solid waste and plastic solid waste management in the Greatr London area. Chem Eng J 244: 391–402. doi: 10.1016/j.cej.2014.01.066
![]() |
[29] | Achilias DS, Antonakou EV (2015) Chemical and thermochemical recycling of polymers from waste electrical and electronic equipment, 37–61. Available from: https://www.intechopen.com/books/recycling-materials-based-on-environmentally-friendly-techniques/chemical-and-thermochemical-recycling-of-polymers-from-waste-electrical-and-electronic-equipment. |
[30] | Kaminsky W, Kim JS (1998) Pyrolysis of mixed plastics into aromatics. J Anal Appl Prol 51: 127–134. |
[31] |
Lee HW, Park YK (2018) Catalytic pyrolysis of polyethylene and polypropylene over desilicated beta and AI-MSU-F. Catal 8: 501. doi: 10.3390/catal8110501
![]() |
[32] |
Sophonrat N, Sandstrom L, Zaini IN, et al. (2018) Stepwise pyrolysis of mixed plastics and paper for separation of oxygenate and hydrocarbon condensates. Appl Energy 229: 314–325. doi: 10.1016/j.apenergy.2018.08.006
![]() |
[33] | Gaurh P, Pramanik H (2018) Thermal and catalytic pyrolysis of plastic waste propylene for recovery of petroleum range hydrocarbon. Inter J Resear Sci & Engin CHEMCON Special Issue: March 2018: 228–233. |
[34] |
Cao Q, Yuan G, Yin L, et.al. (2016) Morphological characteristics of polyvinyl chloride (PVC) dechlorination during pyrolysis process: Influence of PVC content and heating rate. Waste Manage 58: 241–249. doi: 10.1016/j.wasman.2016.08.031
![]() |
[35] | Bertau M, Offersmanns H, Plass L, et al. Methanol: The Basic Chemical and Energy Feedstock of the Future. Berlin, Germany: Springer, 2014. |
[36] |
Lee RP, Keller F, Meyer B (2017) A concept to support the transformation from a linear to circular carbon economy: net zero emissions, resource efficiency and conservation through a coupling of the energy, chemical and waste management sectors. Clean Energy 1: 102–113. doi: 10.1093/ce/zkx004
![]() |
[37] |
Lopez G, Artetxe M, Amutio M, et.al. (2018) Recent advances in the gasification of waste plastics. A critical overview. Renew Sust Energy Rev 82: 576–596. doi: 10.1016/j.rser.2017.09.032
![]() |
[38] |
Saad JM, Nahil MA, Williams PT (2015) Influence of process conditions on syngas production from the thermal processing of waste high density polyethylene. J Anal Appl Pyrolysis 113: 35–40. doi: 10.1016/j.jaap.2014.09.027
![]() |
[39] |
Lee U, Chung JN, Ingley HA (2014) High-temperature steam gasification of municipal solid waste, rubber, plastic and wood. Energy Fuels 28: 4573–87. doi: 10.1021/ef500713j
![]() |
[40] |
Wu C, Williams PT (2010) Pyrolysis-gasification of post-consumer municipal solid plastic waste for hydrogen production. Int J of Hydrogen Energy 35: 949–957. doi: 10.1016/j.ijhydene.2009.11.045
![]() |
[41] |
Wilson D, Velis C (2015) Waste management-still a global challenge in the 21st century: an evidence based call for an action. Waste Manage Res 33: 1049–1051. doi: 10.1177/0734242X15616055
![]() |
[42] | Inventory of US Greenhouse Gas Emissions and Sinks (1990–2016). EPA 430-R-18-003 https://www.epa.gov/ghgemissions/inventory-us-greenhouse-gas-emissions-and-sinks. |
[43] | The Potential Contribution of Waste Management to a Low Carbon Economy (2015) EUNOMIA https://zerowasteeurope.eu/downloads/the-potential-contribution-of-waste-management-to-a-low-carbon-economy/. |
[44] | United Nations Environment Programme (UNEP) and International Solid Waste Association (ISWA) (2015). Global Waste Management Outlook. Wilson DC (Ed) Authors: Wilson, D.C., Rodic, L, Modak P, et.al. UNEP International Environment Technology Centre: Osaka, September 2015. Available at: http://web.unep.org/ourplanet/september-2015/unep-publications (Accessed January 21, 2019) |
[45] |
Ritzen F, Sandstrom GO (2017) Barriers to the circular economy-integration of perspectives and domains. Procedia CIRP 64: 7–12. doi: 10.1016/j.procir.2017.03.005
![]() |
1. | Ning Wang, Yubing Bai, Zhanqiang Guo, Yu Fan, Fanxin Meng, Synergies between the circular economy and carbon emission reduction, 2024, 951, 00489697, 175603, 10.1016/j.scitotenv.2024.175603 | |
2. | Emre Hatipoglu, Jitendra Roychoudhury, 2024, Chapter 19, 978-3-031-26603-4, 3, 10.1007/978-3-031-26604-1_19 | |
3. | Xiang Zhao, Fengqi You, From sustainable macro debris chemical recycling to microplastic reclamation: Overview, research challenges, and outlook, 2024, 454, 09596526, 142281, 10.1016/j.jclepro.2024.142281 | |
4. | Lukas Zeilerbauer, Valerie Rodin, Stefan Puschnigg, Hannah Aster, Stella Danner, Argjenta Veseli, Christian Paulik, Jörg Fischer, Circular, avoided, or captured carbon (dioxide)? - A taxonomy approach for life cycle assessment and CO 2 accounting , 2024, 15, 1758-3004, 10.1080/17583004.2024.2408285 | |
5. | Elias W. Gabisa, Chavalit Ratanatamskul, Shabbir H. Gheewala, Recycling of Plastics as a Strategy to Reduce Life Cycle GHG Emission, Microplastics and Resource Depletion, 2023, 15, 2071-1050, 11529, 10.3390/su151511529 | |
6. | Benett Siyabonga Madonsela, Machete Machete, Karabo Shale, Indigenous Knowledge Systems of Solid Waste Management in Bushbuckridge Rural Communities, South Africa, 2024, 2, 2813-0391, 293, 10.3390/waste2030017 | |
7. | Peter Dauvergne, The necessity of justice for a fair, legitimate, and effective treaty on plastic pollution, 2023, 155, 0308597X, 105785, 10.1016/j.marpol.2023.105785 | |
8. | Emre Hatipoglu, Jitendra Roychoudhury, 2023, Chapter 19-1, 978-3-030-74380-2, 1, 10.1007/978-3-030-74380-2_19-1 | |
9. | Benett Siyabonga Madonsela, Khomotso Semenya, Karabo Shale, Lusiwe Maduna, Sustainability of Indigenous Solid Waste Management Practices in Rural Communities of South Africa, 2024, 9, 2313-4321, 113, 10.3390/recycling9060113 |
Waste material | Weight generated Mt | Weight recycled Mt | Weight incinerated for energy Mt | Weight landfilled Mt | Recycled % |
Incinerated % |
Landfilled % |
Plastics | 34.50 | 3.14 | 5.35 | 26.01 | 9.1 | 15.5 | 75.4 |
Products | Applications |
rPE | Agricultural seeding trays |
rPE | Horticultural applications: water proof materials |
rPP | Turnover boxes, pallets and folding containers |
rPP | Wire reels |
rPC | Suitcase accessories; handles, wheels and pull rods |
rPC | Interior automobile trims: air-condition ducts, doorsills, door panels, engine covers, |
fenders and mud-boards, wheel covers, bumpers | |
rPP | Household tools |
rPE: Recycled Polyethylene; rPP: recycled Polypropylene; rPC: recycled polypropylene composite |
Application rigid PVC applications | Share of the components (weight%) | ||||
PVC polymer | Plasticizer | Stabilizer | Filter | Others | |
Pipes | 98 | - | 1–2 | - | |
Window profiles | 85 | - | 3 | 4 | 8 |
Other profiles | 90 | - | 3 | 6 | 1 |
Rigid films | 95 | - | - | - | 5 |
Flexible PVC applications | |||||
Cable insulation | 42 | 23 | 2 | 33 | - |
Flooring | 42 | 15 | 2 | 41 | 0 |
Flooring (past upper layer) | 65 | 32 | 1 | - | 2 |
Flooring (paste, inside material) | 35 | 25 | 1 | 40 | - |
Synthetic leather | 53 | 40 | 1 | 5 | 1 |
Furniture films | 75 | 10 | 2 | 5 | 8 |
Leisure articles | 60 | 30 | 2 | 5 | 3 |
Type of challenge | |
Economic cost (up-front investment, risks) |
Assessing financial benefits of circular economy |
Assessing financial profitability | |
Structural lack of understanding & participation (business, consumer and decision makers |
Achieving exchange of information |
Defining responsibility distribution | |
Operational Complex international production & consumption supply-chains |
Redefining the infrastructure |
Strong supply chain | |
Knowledge & behavior need for knowledge and capacity for implementation need for education |
Perception of sustainability |
Behavior change | |
Technological need for innovative technologies |
New product design to absorb waste resources |
Integration into processing | |
Environmental assessment | Correct LCA of circular approaches |
need for regulations | Lack of standards, monitoring & reporting |
Waste material | Weight generated Mt | Weight recycled Mt | Weight incinerated for energy Mt | Weight landfilled Mt | Recycled % |
Incinerated % |
Landfilled % |
Plastics | 34.50 | 3.14 | 5.35 | 26.01 | 9.1 | 15.5 | 75.4 |
Products | Applications |
rPE | Agricultural seeding trays |
rPE | Horticultural applications: water proof materials |
rPP | Turnover boxes, pallets and folding containers |
rPP | Wire reels |
rPC | Suitcase accessories; handles, wheels and pull rods |
rPC | Interior automobile trims: air-condition ducts, doorsills, door panels, engine covers, |
fenders and mud-boards, wheel covers, bumpers | |
rPP | Household tools |
rPE: Recycled Polyethylene; rPP: recycled Polypropylene; rPC: recycled polypropylene composite |
Application rigid PVC applications | Share of the components (weight%) | ||||
PVC polymer | Plasticizer | Stabilizer | Filter | Others | |
Pipes | 98 | - | 1–2 | - | |
Window profiles | 85 | - | 3 | 4 | 8 |
Other profiles | 90 | - | 3 | 6 | 1 |
Rigid films | 95 | - | - | - | 5 |
Flexible PVC applications | |||||
Cable insulation | 42 | 23 | 2 | 33 | - |
Flooring | 42 | 15 | 2 | 41 | 0 |
Flooring (past upper layer) | 65 | 32 | 1 | - | 2 |
Flooring (paste, inside material) | 35 | 25 | 1 | 40 | - |
Synthetic leather | 53 | 40 | 1 | 5 | 1 |
Furniture films | 75 | 10 | 2 | 5 | 8 |
Leisure articles | 60 | 30 | 2 | 5 | 3 |
Type of challenge | |
Economic cost (up-front investment, risks) |
Assessing financial benefits of circular economy |
Assessing financial profitability | |
Structural lack of understanding & participation (business, consumer and decision makers |
Achieving exchange of information |
Defining responsibility distribution | |
Operational Complex international production & consumption supply-chains |
Redefining the infrastructure |
Strong supply chain | |
Knowledge & behavior need for knowledge and capacity for implementation need for education |
Perception of sustainability |
Behavior change | |
Technological need for innovative technologies |
New product design to absorb waste resources |
Integration into processing | |
Environmental assessment | Correct LCA of circular approaches |
need for regulations | Lack of standards, monitoring & reporting |