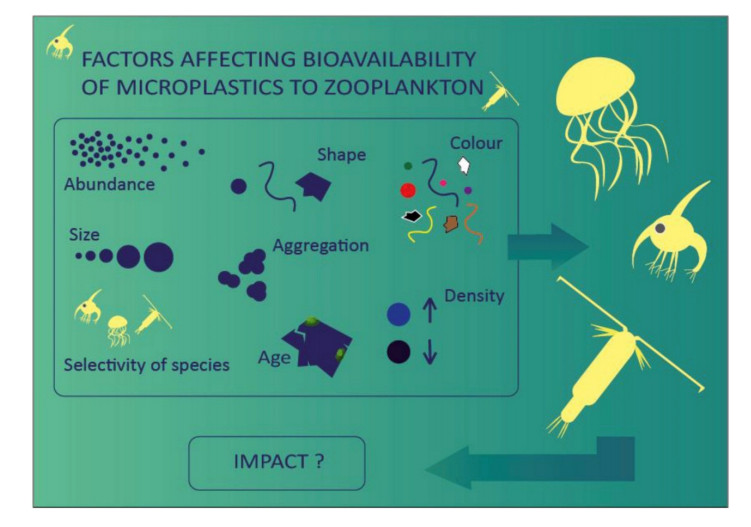
Citation: Judith S. Weis. Improving microplastic research[J]. AIMS Environmental Science, 2019, 6(5): 326-340. doi: 10.3934/environsci.2019.5.326
[1] | Mahidin, Asri Gani, Saiful, Muhammad Irham, Wulan Windari, Erdiwansyah . An overview of the potential risks, sources, and analytical methods for microplastics in soil. AIMS Environmental Science, 2022, 9(2): 185-216. doi: 10.3934/environsci.2022013 |
[2] | Fabiana Carriera, Cristina Di Fiore, Pasquale Avino . Trojan horse effects of microplastics: A mini-review about their role as a vector of organic and inorganic compounds in several matrices. AIMS Environmental Science, 2023, 10(5): 732-742. doi: 10.3934/environsci.2023040 |
[3] | Abigail W. Porter, Sarah J. Wolfson, Lily. Young . Pharmaceutical transforming microbes from wastewater and natural environments can colonize microplastics. AIMS Environmental Science, 2020, 7(1): 99-116. doi: 10.3934/environsci.2020006 |
[4] | Katharina Meixner, Mona Kubiczek, Ines Fritz . Microplastic in soil–current status in Europe with special focus on method tests with Austrian samples. AIMS Environmental Science, 2020, 7(2): 174-191. doi: 10.3934/environsci.2020011 |
[5] | Gina M. Moreno, Keith R. Cooper . Morphometric effects of various weathered and virgin/pure microplastics on sac fry zebrafish (Danio rerio). AIMS Environmental Science, 2021, 8(3): 204-220. doi: 10.3934/environsci.2021014 |
[6] | Andrew Wirnkor Verla, Christian Ebere Enyoh, Isiuku Beniah Obinna, Evelyn Ngozi Verla, Wang Qingyue, Md. Akhter Hossain Chowdhury, Emmanuel Chinedu Enyoh, Tanzin Chowdhury . Effect of macro-and micro-plastics in soil on growth of Juvenile Lime Tree (Citrus aurantium). AIMS Environmental Science, 2020, 7(6): 526-541. doi: 10.3934/environsci.2020033 |
[7] | Nasrul Arahman, Cut Meurah Rosnelly, Sri Mulyati, Wafiq Alni Dzulhijjah, Nur Halimah, Rinal Dia'ul Haikal, Syahril Siddiq, Sharfina Maulidayanti, Muhammad Aziz, Mathias Ulbricht . Investigation of microplastics in community well water in Banda Aceh, Indonesia: a separation technique using polyethersulfone-poloxamer membrane. AIMS Environmental Science, 2025, 12(1): 53-71. doi: 10.3934/environsci.2025003 |
[8] | K. Wayne Forsythe, Chris H. Marvin, Danielle E. Mitchell, Joseph M. Aversa, Stephen J. Swales, Debbie A. Burniston, James P. Watt, Daniel J. Jakubek, Meghan H. McHenry, Richard R. Shaker . Utilization of bathymetry data to examine lead sediment contamination distributions in Lake Ontario. AIMS Environmental Science, 2016, 3(3): 347-361. doi: 10.3934/environsci.2016.3.347 |
[9] | B. Ravit, K. Cooper, G. Moreno, B. Buckley, I. Yang, A. Deshpande, S. Meola, D. Jones, A. Hsieh . Microplastics in urban New Jersey freshwaters: distribution, chemical identification, and biological affects. AIMS Environmental Science, 2017, 4(6): 809-826. doi: 10.3934/environsci.2017.6.809 |
[10] | B. Ravit, K. Cooper, B. Buckley, I. Yang, A. Deshpande . Organic compounds associated with microplastic pollutants in New Jersey, U.S.A. surface waters. AIMS Environmental Science, 2019, 6(6): 445-459. doi: 10.3934/environsci.2019.6.445 |
While plastic items like bottles, bags, and balloons are highly visible litter and well-known as ugly eyesores and hazards to wildlife, there is another form of plastic that is generally not visible but is far more numerous and may be equally or more hazardous in the environment. This is microplastic, small pieces ranging from 5 mm in size down to microscopic. Microplastics are categorized as primary microplastic – that which was always tiny, and secondary microplastic-which results from the fragmentation of larger pieces. As with larger pieces, microplastics represent a variety of different polymers, such as polyethylene, polystyrene, etc. They are found in a variety of shapes, including spheres, fragments, films, and fibers. In the past decade there has been a great amount of study on microplastics – where they come from, where they are found, how organisms interact with them, and what effects they may have on the organisms and the ecosystem. Most of these studies have focused on the marine environment since that is where they were initially detected, but they have subsequently been found to be abundant in freshwater and terrestrial environments as well. This review examines the research, emphasizing aquatic environments, and suggests avenues for improving future research.
Perhaps the most common type of study is one in which researchers count the number of microplastic (MP) particles in their nearby stream, river, estuary, ocean, or lake. Results are consistent: microplastics are found everywhere they are sought, including the deep-sea trenches [1,2], freshwater lakes [3] drinking water [4] outdoor and indoor air [5,6] including remote mountaintops [7] and soils [8]. Thus, it has been adequately demonstrated that MPs are ubiquitous, and it is not necessary to count them in all water bodies. Furthermore, since there are no standardized methods or protocols, it is not possible to compare concentrations in one locality to those in other places or to examine trends over time.
Some researchers count them under a microscope while others use sophisticated chemical analysis such as GC-MS (Gas chromatography-mass spectrometry), Raman Spectroscopy, or Fourier Transform Infrared (FTIR) spectroscopy and can delineate the relative amounts of different plastic polymers. Both FTIR and Raman spectroscopy are used for the quick detection of MPs including microfibers. While counting particles under a microscope is useful and appropriate for citizen science projects and educational programs, perhaps it is no longer appropriate for journal articles, particularly because this method has a very high error rate [9] and can easily confuse plastic microfibers with cotton ones. GESAMP [10] has recently released a report about methods for sampling plastics, including recommendations.
MPs are found in the air, in "fallout" from the air and in the soil; they clearly are present in terrestrial as well as aquatic environments, partly because sewage sludge is often applied on land. There has been little attention paid to their occurrence in the air [11], but in urban air they have been found to be predominantly microfibers from synthetic textiles [12]. It is therefore important to investigate intake via inhalation by terrestrial animals including humans. Since MPs alter the physical properties of soils [8] it is important to investigate uptake by and effects on soil biota. In an early study, it was found [13] that they have transient effects reducing germination in seeds of Lepidium sativum.
Recommendations: Rather than continuing to use microscopes to count numbers in additional water bodies, we should use chemical analysis to learn chemical composition. Standard methods for collection and analysis are greatly needed to enable comparisons. More study of MPs in air and soil is needed.
The numbers of MP found in the counting studies are highly dependent on the method used for collecting the samples. While some researchers use plankton nets (e.g. bongo, manta) to sample the surface layers of the water, those who examine whole water samples obtain very different results. Long thin MPs, namely microfibers, tend to go through holes in nets (as do long thin phytoplankton). Rocha-Santos and Duarte [14] evaluated various sampling methods and approaches. Samples from net collections show microfibers to be much less abundant than in whole water samples. Green et al. [15] reported that manta, bongo and plankton nets may underestimate concentrations of microplastic fibers by three to four orders of magnitude compared to grab (whole water) methods. A meta-analysis [16] found "average sample composition in the water column was 52% fibers, followed by 29% fragments, with other particle morphologies including beads/spherules, films, foams, and others making up only a small proportion" Carr et al. [17] found that over 80% of the MPs in the ocean and freshwater were fibers, and Constant et al. [18] indicated that microfibers were the dominant form of beached MPs.
Browne et al. [19] found that microfibers, an important component of MPs in sediments, were derived from clothing via wastewater from washing machines. Proportions of polyester and acrylic fibers used in synthetic clothing resembled those in habitats receiving sewage effluent. Experiments sampling wastewater from washing machines found that a single garment can produce almost 2000 fibers in a single wash. It is estimated that in a single laundry wash, approximately 121, 465 acrylic, 82, 672 polyester, and 22, 992 poly-cotton microfibers are released into the ocean (https://www.vox.com/the-goods/2018/9/19/17800654/clothes-plastic-pollution-polyester-washing-machine). IUCN [20] reported on the sources of the microfibers and found that two-thirds of the releases were due to the laundering of synthetic textiles, and to the erosion of tires while driving. The third important contribution was city dust. Personal care products accounted for only 2% of the global release of primary microplastics to the ocean.
Recommendations: In order to get representative samples from aquatic environments, grab (whole water) samples are required rather than nets.
Some types of polymers tend to float, and others are denser and tend to sink, so collecting from surface waters does not give a representative sample of the different types of polymers present. Since MPs differ in chemical composition, size, shape, color, density, and effects on biota, they should be regarded as a suite of contaminants, somewhat comparable to metal pollutants, and samples should be characterized that way [21]. Microfibers from clothing, the most abundant type of MP in the water (e.g. polyester and acrylic), tend to sink rather than float [22], as all MPs found in deep-sea organisms were fibers.
Ingestion has been reported in marine mammals, birds, fishes, macroinvertebrates and plankton. Almost as common as papers documenting the numbers of MPs in a body of water are papers demonstrating that some species of aquatic animal consumes them. Most of the plastics found inside animals in the field are microfibers, which may reflect the relative abundance in the environment, or perhaps that they are not egested as readily as other shapes [23].
Some papers go beyond merely reporting that "species X eats microplastics" and investigate why. Ory et al. [24] compared MPs and planktonic organisms in surface waters and as food of Amberstripe scads (Decapterus muroadsi) along the coast of Rapa Nui (Easter Island) to see if fish ingest MPs resembling their natural prey. Eighty percent of the fish ingested mainly blue polyethylene fragments similar in color and size to the blue copepods they normally consume, suggesting that these fish select floating MPs that resemble their natural planktonic food.
Some studies indicate that the bacterial microfilm around the particles is what makes them attractive to consumers [25]. This would at least provide a little nutrition to the animal. Other studies suggest that the attractant for corals is the plastic itself rather than the biofilm [26]. Since the biofilm may concentrate metal pollutants [27] and toxin-producing microbes e.g. Pfiesteria piscicida [28], it may be a source of toxicity as well as nutrition to consumers.
Botterell et al. [29] reviewed the bioavailability and effects of MPs on marine zooplankton. They found that ingestion had been seen in 39 zooplankton species, most of which were studied in the laboratory. They emphasized the importance of physical differences (size, shape, type, age, and abundance) of microplastics in determining their ingestion (Figure 1). They recommend that future research should use MP types more representative of what is in the environment and in comparable concentrations. In another interesting study de Orte et al. [30] exposed both bleached (without algal symbionts) and control (with algal symbionts) sea anemones (Aiptasia pallida) to microfibers of nylon, polyester and polypropylene. The microfibers were introduced either alone or mixed with food (brine shrimp homogenate). When offered without shrimp, more nylon was ingested than other polymers but when offered with shrimp, over 80% of the anemones took up all types of polymers. Retention time differed between symbiotic and bleached anemones with faster egestion in anemones with symbionts. This shows that ingestion of microfibers by anemones depends on the polymer and the presence of chemical cues from prey. The reduced ability of bleached anemones to egest microfibers indicates that susceptibility to plastic is increased by prior exposure to other stressors, in this case elevated temperatures which trigger bleaching. These are all topics of research worth pursuing.
It is important to note that surface-dwelling organisms are more likely to encounter and consume polystyrene, polypropylene, and polyethylene which are less dense than seawater and float, and that animals living deeper are more likely to encounter denser plastics such as polyethylene terephalate and polyvinyl chloride [31].
What is missing in most ingestion studies, however, is investigation of how soon the plastics are egested, and what percent are, in fact, just "passing through" the animal. Most MPs found in animals' guts are fibers and fragments [32], while only a small proportion are beads. Fibers could be most numerous because their ingestion reflects environment composition and/or because fibers may not be egested as efficiently as other particles. There are numerous laboratory studies on ingestion but few on egestion, particularly at concentrations resembling those in the environment. It may be that some shapes (e.g. spheres) are more likely to pass through easily and rapidly, while fragments with sharp edges are more likely to damage tissues, and fibers are more likely to form tangles and clog up the digestive system. However, Grigorakis et al. [33] found rapid gut clearance of both microspheres and microfibers by goldfish. Besseling et al. [34] found that lugworms (Arenicola marina) completely egested all consumed microplastic particles overnight. However, Setä lä et al. [35] found that the clam Scrobicularia plana took over a week to clear all consumed microbeads. Similarly, field-collected crustaceans Nephrops norvegicus retained plastics in their guts for over two weeks [23]. The degree and rate of egestion will depend on the shape, size, etc. of the MP, as well as the length, and complexity/morphology of the digestive tract of the animal. Studies incorporating egestion are of more interest than papers reporting ingestion by one more species.
Mussels and other filter-feeding bivalves reject undesirable particles during or immediately after capture by means of pseudofeces that are egested before being swallowed. In experimental feeding studies, most microfibers (71%) in mussels were found in the pseudofeces at all experimental concentrations [36]. Another 10% were found in feces after passing through digestive system [36]. Li et al. [37] found that in bivalves, PET plastics were taken up much more than other polymers, and that microfiber sizes of 100–250 μm were taken up much more than either smaller or larger sizes.
What is also missing from most exposure studies is the examination of another route of uptake, namely respiration. There are very few papers examining gill uptake. Watts et al. [38] found that exposure to polystyrene microspheres (8 μm) with different surface coatings had transient effects on branchial function of shore crabs, Carcinus maenas. Microspheres inhaled into the gill chamber had a small but significant effect on oxygen consumption after 1 h of exposure which returned to normal levels after 16 h. Ion exchange was also affected, with a small but significant decrease in hemolymph sodium ions and increase in calcium ions after 24 h. To examine effects on osmoregulation, they subjected crabs to reduced salinity but the response to osmotic stress was unaffected by plastic concentration. Lu et al. [39] examined uptake and tissue accumulation of polystyrene microplastics (PS-MPs) in zebrafish and found that after 7 days of exposure, 5 μm diameter MPs accumulated in gills, liver, and gut, while 20 μm diameter MPs accumulated only in gills and gut. Histopathological analysis showed that both 5 μm and 70 nm (nanometers) PS-MPs caused inflammation and lipid accumulation in the liver. PS-MPs also increased the activities of the enzymes superoxide dismutase and catalase, indicating oxidative stress was induced. Since uptake by gills is passive, it may be as common as ingestion, and is worth considerably more study in more organisms, using microfibers, and not just spheres. In addition, the findings of MPs in air should prompt inhalation studies in terrestrial animals.
Recommendations: Rather than reporting that another species consumes MPs, researchers should examine what attracts them to the MP, which chemical type and shape of MPs are preferred by different organisms, and rates of egestion. Researchers should not continue to focus on microspheres, which are rare in the environment, but use representative shapes, primarily microfibers. Research into respiration as a mode of intake is greatly needed in both aquatic and terrestrial environments.
It is important to study to what degree MPs can move out of the digestive system into other tissues rather than being egested. Field-collected anchovy, Engraulis encrasicolus, were found to have MPs, primarily polyethylene, in their livers [40]. There are relatively few laboratory observations of MPs moving out from the digestive tract into tissues. D. magna exposed to fluorescent polystyrene spheres appeared to show translocation across the gut epithelial barrier [41]. However, when this study was repeated, Schür et al. [42] demonstrated that the fluorescence in D. magna resulted from leaching of the fluorescent dye rather than the transport of MPs through the gut wall. Crabs exposed to 0.5 mm spheres showed translocation to the hemolymph, gills, and ovary [43]. However, it seems likely that some of the MPs in the crab gills got there directly from the water rather than translocating from the digestive tract. Both of these laboratory studies used spheres.
Recommendations: Most of the attention was originally on microspheres, but now that it is understood that they comprise only a small percentage of MPs in the environment future studies should use environmental mixtures (microfibers primarily) that reflect environmental concentrations of the different shapes. More definitive studies on translocation of MPs from the gut into tissues in a variety of organisms are needed.
In laboratory studies, copepods (Eurytemora affinis) and polychaete larvae (Marenzellaria sp) ingested fluorescent polystyrene microspheres and subsequently passed them on to mysids which consumed them [36]. The blue mussel, Mytilus edulis took up green fluorescent polystyrene microspheres, before being fed to shore crabs (Carcinus maenas). Particles were subsequently detected in the hemolymph, stomach, hepatopancreas, ovary and gills of the crabs [43]. The microspheres in the crab hemolymph were 0.04% of the amount to which the mussels were exposed. Microspheres were also found in the stomach, hepatopancreas, ovary and gills of the crabs, decreasing over the trial period. Brown mussels (Perna perna) were fed polyvinylchloride (PVC) microspheres and then fed to the fish Spheroides greelyi, and to crabs, Callinectes ornatus [44]. The plastics were transferred to the predators. Thus, transfer up the food web has been demonstrated in the laboratory, but in these studies, prey organisms were fed only MPs and then fed to predators prior to egestion; then MPs were measured in the predators prior to any egestion. This sort of experimental design is not realistic.
Recommendations: Studies are needed to quantify to what degree MPs of different types, sizes, and shapes can be transferred from gut to tissues of animals and then through the food web. In such laboratory studies, MPs should be combined with "real" food and time should be provided for egestion.
The degree and type of effects that MPs can have in an organism may depend on polymer type, size, shape, concentration, and adsorbed chemicals. Because of physical and biological processes, such as agammaegation and biofouling, plastic particles on the bottom may greatly exceed the number in the water column and pose a greater risk to benthic fauna. However, most of the studies of effects have focused on pelagic organisms so there is need for more study of benthic organisms [45]. In their review [45], Haegerbaeumer et al. recommended more studies on freshwater organisms, on meiobenthic organisms in both marine and freshwater environments, and studies on MPs whose polymer composition, shape, surface properties, and exposure routes reflect those in the natural environment. They also recommended long-term studies of multiple species (model ecosystems) for greater ecological relevance.
Studies on soil organisms are also ongoing, which will be mentioned only briefly. The most abundant type of MP found in soils was microfibers, which were transported to deeper layers [46] and eaten by earthworms Lumbricus terrestris [47]. L terrestris in high concentrations of MPs showed lower cast production and altered gene expression [48], lower growth rate and higher mortality [49].
Some MPs have been found to adversely affect organisms at concentrations above environmental levels. Green et al. [50] exposed mussels (Mytilus edulis) to high concentrations of polyethylene fragments and noted that the number of byssus threads produced was decreased as well as their attachment strength. In many studies, clean spherical particles are used, rather than fibers or weathered fragments. In amphipods, ingested MPs can block the digestive tract and thereby reduce the amount of food they can eat [51]. Microfibers were more damaging than other types of microplastics possibly because they remained in the gut longer [51]. Jovanovic et al. [52] found that microfiber ingestion by small fish can block the digestive system, interfere with feeding, and change the animal's behavior. While Jovanovic et al. [52] used microfibers, many other studies use sizes, morphology, and/or concentration of microplastics very different from those in the environment. In a study that used environmentally relevant levels of polyethylene MPs and two bivalve species, Bour et al. [53] found changes in energy reserves in the clam Ennucula tenuis, in which total energy decreased in those exposed to the largest particles (125–500 μm). While changes in energy were not seen in the clam Abra nitida, protein content decreased in individuals exposed to the largest particles, at all concentrations. When microbeads and microfibers were compared for toxicity to Daphnids, fibers caused greater adverse effects than beads [54]. Scanning electron microscopy showed deformities in the carapace and antenna after exposure to fibers but not beads. This study showed that fibers pose a greater risk to Ceriodaphnia. dubia [54]. Foley et al. [55] performed a meta-analysis of impacts of exposure to MPs on feeding, growth, reproduction, and survival of fish and aquatic invertebrates. They observed some negative effects for all four categories of responses, but many of the effects were neutral, indicating that effects of exposure are highly variable across taxa. The most consistent effect was a reduction in consumption of natural prey when MPs were present. This effect would be expected if animals preferentially consume MPs. For some taxa, negative effects on growth, reproduction and even survival were seen. Organisms that are prey to larger predators, e.g. zooplankton, were thought to be particularly susceptible to impacts of MP pollution.
Recommendations: Rather than continuing to test clean microbeads, researchers should ideally test fibers, fragments, and beads of appropriate size ranges simultaneously in the appropriate proportions, and at concentrations near what occur in the environment. Furthermore, rather than feeding plastics with no food future studies should add MPs to food. When animals are not given food, observed effects might be attributed to microplastic intake but may in fact be due to lack of food, i.e. starvation.
There are two types of chemicals of concern in MP toxicity studies: (1) the chemicals in the plastic itself, including the PAH (polyaromatic hydrocarbon) polymer plus additives such as phthalates, flame retardants, pigments, stabilizers or BPA (bisphenol A), and (2) the environmental chemicals that adsorb onto the plastic, such as PCBs (polychlorinated biphenyls), metals, and PBDEs (polybrominated diphenyl ethers). The high surface/volume ratio and small size of MPs allow substantial amounts of many environmental chemicals to adsorb onto them, but polymer type determines the degree of adsorption [56,57,58]. Many of these chemicals can be toxic, carcinogenic, mutagenic or endocrine disruptors.
Some of the additive chemicals can leach out from MPs. The leachate from the raw resin pellets used in manufacture of plastic products was found to affect anti-predator behaviors of periwinkle snails [59] but the snails responded differently to virgin vs beached pellets. It is likely that the virgin plastic leached out plastic additives, while the beached older plastic leached out adsorbed chemicals such as persistent organic pollutants, PAHs and metals, a more complicated mixture of contaminants. In contrast, no toxicity was observed for extracts of virgin MPs on embryos of the medaka (Oryzias latipes) regardless of the endpoint [60] while extracts of virgin MPs coated with 250 µg g-1 of B[a]P (benzo [a] pyrene) induced lethal effects with high embryo mortality and low hatching rate, altered swimming behavior, increase of EROD (Ethoxyresorufin-O-deethylase) activity, and DNA damage.
Since they attract environmental chemicals, it has been assumed that MPs act as a vector to transfer these toxic environmental pollutants to animals. Wardrop et al. [61] indicated that ingested microbeads can transfer adsorbed PBDEs (polybrominated diphenyl ethers) to the rainbow fish (Melanotaenia fluviatilis). Lower brominated congeners had the highest assimilation while higher brominated congeners did not transfer, indicating they may be too tightly adsorbed onto the plastic or unable to be assimilated for other reasons. Beckingham and Ghosh [62] demonstrated uptake of PCBs from microplastic in benthic worms, but the PCB uptake by worms was much greater from sediments than from MPs. PCB uptake was 76% lower when the PCBs were associated with polypropylene MPs, compared to sediment. The presence of MPs in the sediments had an overall effect of reducing the bioavailability and transfer of the chemicals from MPs to sediment-ingesting organisms.
In the terrestrial environment, Hodson et al. [63] found that while very little Zn desorbed from MPs in synthetic soil solution, there was greater Zn desorption from MPs in earthworm digestive systems. Tourinho et al. [64] assessed the distribution of two PCBs and phenanthrene in the soil in the presence of MPs using a mass balance model. The results showed a high variation among chemicals and microplastic types. Overall, higher partitioning of chemical contaminants to MPs in soil were predicted in comparison to aquatic environments. They reviewed many studies indicating that the presence of MPs could either increase or decrease or not affect the toxicity of environmental chemicals to soil organisms.
Batel et al. [65] found that in the laboratory benzo [a] pyrene was transferred from microplastics to Artemia nauplii and from them to zebrafish, via trophic transfer. Most of these studies did not use microfibers, and research is needed to ascertain which environmental chemicals may be more tightly bound to MPs of certain shape and chemistry, and how quickly they could be desorbed in the gut. Some laboratory studies report complete egestion in 24–48 hours, so it is important to learn whether the plastic remains in the gut long enough for significant desorption of various chemicals. Perhaps species with longer, more convoluted digestive systems retain plastics longer and are more likely to desorb more. It could also depend on the chemistry within the digestive tract. For example, Bakir et al. [66] found that acidic gut conditions facilitate desorption, but Bakir et al. [67] modelled the transfer of adsorbed organic contaminants to a benthic invertebrate, a fish and a seabird, and concluded that intake from food and water was the main route of exposure for phenanthrene, di(2-ethylhexyl) phthalate (DEHP), and dichlorodiphenyltrichloroethane (DDT), with negligible input from the plastic, and that contaminated MPs had a negligible impact on transfer to biota under both relevant and worst case scenarios. It was recently found that the presence of polyethylene MPs can reduce the acute toxicity of the synthetic pyrethroid bifenthrin to larvae of the midge Chironomus tepperi [68]. The addition of MPs significantly reduced toxicity, probably because sorption of bifenthrin to the MPs reduced its availability to the larvae [68]. Similar observations were made by Wang et al. [69] with earthworms, Eisenia fetida, in soil, in which the presence of MPs decreased the uptake of PCBs and PAHs into the worms.
Therefore, available evidence that microplastics act as a vector of organic pollutants into organisms is thus far inconclusive and probably depends on the pollutant, the MP type, and the organism.
Recommendations: Treating animals with extracts is not a realistic scenario. It appears likely that bioavailability of these chemical contaminants is greater from their natural food than from ingested MPs which bind the chemicals tightly; these two sources of contaminants should be compared. Studies should explore the possibility that MP types that clog up the gut and stay there longer (e.g. fibers) are likely to have more time for chemicals to desorb. Research is needed to examine the bioavailability of toxic chemicals from MPs lodged in respiratory organs, where plastics may remain for longer time periods than in the gut. These are important questions to study. There is also a need to use more microfibers instead of microspheres in such studies, reflecting the shape that is most prevalent in the environment.
While environmental scientists focus on understanding in greater depth the environmental fate and effects of MPs, scientists from other disciplines are needed to work to reduce the inputs of MPs to the environment. Since it is best to focus such studies in fields that will make the most difference and microfibers are the most abundant MPs, work should be concentrated on reducing inputs of microfibers from washing machines. Filters on washing machines may be a simple solution to reduce the release of microfibers into the environment. A filter has been developed for washing machines that traps most of the microfibers coming off clothing. A "Cora Ball" can be put into the washing machine to capture fibers during the wash; however, it captures less than 30% of the microfibers. The Lint LUV-R filter, however, captured an average of 87% of microfibers in the wash, and significantly reduced the total weight and average length of fibers in effluent [70]. The Seabin Project is trying to decrease marine pollution from MPs. They have designed a bag that can capture MPs including microfibers. However, while it traps MPs of other shapes, the bag captures only 18% of microfibers (https://seabinproject.com/seabin-project-tackling-microfibers-head-on/). This is reminiscent of the poor success of plankton nets in catching microfibers from the water, as discussed earlier.
Going back to the original source of the problem – synthetic textiles: not all synthetics release the same amount of fibers, and it may be possible to re-engineer the way they are made so that they won't shed (as many) fibers when washed [71]. Almroth et al. [72] tested different types of fabrics and found that all textiles shed, but polyester fleece fabrics shed the greatest amounts, averaging 7360 fibers/m-2/L-1 in one wash, compared with polyester fabrics which shed 87 fibers/m-2/L-1. They found that loose textile constructions shed more, as did worn fabrics, so that high twist yarns are to be preferred for reduced shedding. Zambrano et al. [73] found that cotton and rayon fabrics also release microfibers when laundered but that those microfibers degrade in natural aquatic environments, while plastic microfibers do not. Belzagui et al. [74] recognizing that there is no consensus on the methodologies to quantify and report the results of microfiber detachment from textile garments, evaluated microfiber detachment rates of various garments to provide a set of comparable units to report the results. They found two different sources in the fabric: some are microfibers that were already loosely entangled with the fibers' grid of the garments, the others are microfibers that were ripped-off by the mechanical stress in the washing machine. They found that the microfiber length decreased from the first to the third washing cycle and noted a strong relationship between microfiber detachment and the textile's density, as noted by Almroth et al. [72]. As one example of research to re-engineer fabrics, Pena-Francesch and Demirel [75] have found a protein in squid suckers that enables tears in fabrics to heal, and thus reduces fiber shedding.
Recommendations: There is a need for textile scientists, polymer chemists, and materials scientists to develop new ways of making fabrics so they will not shed (as many) fibers when laundered. This will be an important way to reduce MP pollution in the future.
Environmental sampling-At this point, more studies counting numbers in shallow aquatic samples, using nets to collect them, and counting them under a microscope are not needed to move the field forward. More analysis of concentrations in mid-water and deep sediments in the ocean, in air, and soils in various regions, including the abundance of different polymers, sizes, and shapes are needed, viewing MPs as a suite of contaminants. There is an urgent need to develop standardized methods so valid comparisons can be made.
Exposure studies-Additional reports documenting that other aquatic animals consume MPs are not necessary. Future studies should examine ingestion and egestion rates, and what attracts different species to eat MPs. Future studies examining soil biota (animals and plants) for rates of uptake from the soil, and studies investigating respiration as a route of intake, both in aquatic and terrestrial animals are recommended. Since microfibers are the most abundant MP in the environment, exposure and effects studies should focus mostly on them and not continue to use spheres.
Transfer out of the digestive tract into tissues-More studies are needed to quantify and document rates of transfer to other tissues, using environmentally representative types and concentrations of MPs.
Trophic transfer studies-There is a need for realistic studies that quantify transfer rates using environmentally relevant concentrations and types of MPs, and which give both prey and predator "real food" and adequate time for egestion.
Effects-Future studies should use different polymers and shapes and should give animals "real" food in addition to MPs, so that symptoms of starvation are not interpreted as effects of the plastic. Future studies should have measures that can be compared with one another (e.g. using dry weight). Studies are needed on uptake and effects of MPs acquired through respiration.
Toxicology-Research is needed to learn what fraction of adsorbed chemical contaminants of different types can be pulled off MPs during the time that they are traveling through digestive tracts of different organisms. Future studies should examine the availability of environmental chemicals desorbed from microplastics in respiratory organs.
Other types of research that are needed to solve the problems-While environmental scientists can analyze the problems caused by MPs, other kinds of scientists are needed to develop ways of reducing their release into the environment. Inexpensive attachments to washing machines that can trap MPs can be improved, and research into polymer chemistry and materials/textile science is needed to develop textiles that do not shed (as many) microfibers.
I am grateful to Beth Ravit and Keith Cooper for inviting me to give the final talk "Next Steps" at the Impacts of Microplastics in the Urban Environment Conference at Rutgers in March 2019, and to Peddrick Weis and two anonymous reviewers for their suggestions for improving this manuscript.
I have no conflict of interest or source of funds for this article.
[1] |
Courtene-Jones W, Quinn B, Gary S, et al. (2017) Microplastic pollution identified in deep-sea water and ingested by benthic invertebrates in the Rockasll Trough, North Atlantic Ocean. Environ Poll 231: 271–280. doi: 10.1016/j.envpol.2017.08.026
![]() |
[2] | Sanchez-Vidal A, Thompson R, Canala M, et al. (2018) The imprint of microfibres in Southern European deep seas. PLoS ONE 13: e0207033 |
[3] |
Mason S, Kammin L, Eriksen M, et al. (2016) Pelagic plastic pollution within the surface waters of Lake Michigan, USA. J Great Lakes Res 42: 753–759. doi: 10.1016/j.jglr.2016.05.009
![]() |
[4] |
Schymanski D, Goldbeck C, Humpf HU, et al. (2018) Analysis of microplastics in water by micro-Raman spectroscopy: Release of plastic particles from different packaging into mineral water. Water Res 129: 154–162. doi: 10.1016/j.watres.2017.11.011
![]() |
[5] |
Dris RJ, Gaspari J, Saad M, et al. (2016) Synthetic fibers in atmospheric fallout: a source of microplastics in the environment? Mar Poll Bull 104: 290–293. doi: 10.1016/j.marpolbul.2016.01.006
![]() |
[6] |
Dris RJ, Gaspari J, Mirande C, et al. (2017) A first overview of textile fibers, including microplastics, in indoor and outdoor environments. Environ Pol 221: 453–458. doi: 10.1016/j.envpol.2016.12.013
![]() |
[7] |
Allen S, Allen D, Phoenix V, et al. (2019) Atmospheric transport and deposition of microplastics in a remote mountain catchment. Nature Geosci 12: 339–344. doi: 10.1038/s41561-019-0335-5
![]() |
[8] |
Zhang G, Zhang F, Li X (2019) Effects of polyester microfibers on soil physical properties: Perception from a field and pot experiment. Sci Total Envir 670: 1–7. doi: 10.1016/j.scitotenv.2019.03.149
![]() |
[9] | Hidalgo-Ruz V, Gutow L, Thompson R, et al. (2013) Microplastics in the marine environment: a review of the methods used for identification and quantification. Envir Sci Tech46: 3060–3075. |
[10] | GESAMP (2019) Guidelines or the monitoring and assessment of plastic litter and microplastics in the ocean (Kershaw P.J, Turra A. and Galgani F. editors), (IMO/FAO/UNESCO-IOC/UNIDO/WMO/IAEA/UN/UNEP/UNDP/ISA Joint Group of Experts on the Scientific Aspects of Marine Environmental Protection). Rep. Stud. GESAMP No. 99, 130p |
[11] |
Horton A, Walton A, Spurgeon D, et al. (2017) Microplastics in freshwater and terrestrial environments: Evaluating the current understanding to identify the knowledge gaps and future research priorities. Sci Total Environ 586: 127–141. doi: 10.1016/j.scitotenv.2017.01.190
![]() |
[12] |
Liu D, Wang X, Fang T, et al. (2019) Source and potential risk assessment of suspended atmospheric microplastics in Shanghai. Sci Total Environ 675: 462–471. doi: 10.1016/j.scitotenv.2019.04.110
![]() |
[13] | Bosker T, Bouwman L, Brun N, et al. (2019) Microplastics accumulate on pores in seed capsule and delay germination and root growth of the terrestrial vascular plant Lepidium sativum Chemosphere 226: 774–781. |
[14] |
Rocha-Santos T, Duarte A (2015) A critical overview of the analytical approaches to the occurrence, the fate and the behavior of microplastics in the environment. Trends Analyt Chem 65: 47–53. doi: 10.1016/j.trac.2014.10.011
![]() |
[15] |
Green D, Kregting L, Boots B, et al. (2018) A comparison of sampling methods for seawater microplastics and a first report of the microplastic litter in coastal waters of Ascension and Falkland Islands. Mar Poll Bull 137: 695–701. doi: 10.1016/j.marpolbul.2018.11.004
![]() |
[16] |
Burns E, Boxall A (2018) Microplastics in the aquatic environment: Evidence for or against adverse impacts and major knowledge gaps. Envir Toxicol Chem 37: 2776–2796. doi: 10.1002/etc.4268
![]() |
[17] |
Carr SA (2017) Sources and dispersive modes of microfibers in the environment. Integr Environ Assess Mgmt 13: 466–469. doi: 10.1002/ieam.1916
![]() |
[18] |
Constant M, Kerherve P, Mino-Vercello-Verollet M, et al. (2019) Beached microplastics in the northwestern Mediterranean Sea. Mar Poll Bull 142: 263–273. doi: 10.1016/j.marpolbul.2019.03.032
![]() |
[19] |
Browne MA, Crump P, Nivenet SJ, et al. (2011) Accumulation of Microplastic on Shorelines Worldwide: Sources and Sinks. Environ Sci Technol 45: 9175–9179. doi: 10.1021/es201811s
![]() |
[20] | IUCN: Boucher J, Friot D (2017) Primary Microplastics in the Oceans: A Global Evaluation of Sources. Gland, Switzerland: IUCN. 43pp. |
[21] |
Rochman C, Brookman C, Bikker J, et al. (2019) Rethinking microplastics as a diverse contaminant suite. Envir Toxicol Chem 38: 703–711. doi: 10.1002/etc.4371
![]() |
[22] | Taylor M.L, Gwinnett C, Robinson L, et al. (2016) Plastic microfibre ingestion by deep-sea organisms. Sci Rept 6 article 33997. |
[23] |
Murray F, Cowie P (2011) Plastic contamination in the decapod crustacean Nephrops norvegicus (Linnaeus 1758). Mar Poll Bull 62: 1207–1217. doi: 10.1016/j.marpolbul.2011.03.032
![]() |
[24] |
Ory N, Sobral P, Ferreira M, et al. (2017) Amberstripe scad Decapterus muroadsi (Carangidae) fish ingest blue microplastics resembling their copepod prey along the coast of Rapa Nui (Easter Island) in the South Pacific subtropical gyre. Sci Total Envir 586: 430–437. doi: 10.1016/j.scitotenv.2017.01.175
![]() |
[25] |
Savoca MS, Tyson CW, McGill M, et al. (2017) Odours from marine plastic debris induce food search behaviours in a forage fish. Proc. R. Soc. B 284: 20171000. http://dx.doi.org/10.1098/rspb.2017.1000. doi: 10.1098/rspb.2017.1000
![]() |
[26] |
Allen AS, Seymour A, Rittschof D (2017) Chemoreception drives plastic consumption in a hard coral. Mar Poll Bull 124: 198–205. doi: 10.1016/j.marpolbul.2017.07.030
![]() |
[27] |
Richard H, Carpenter E, Komada T, et al. (2019) Biofilm facilitates metal accumulation onto microplastics in estuarine waters. Sci Total Environ 683: 600–608. doi: 10.1016/j.scitotenv.2019.04.331
![]() |
[28] |
Kettner MT, Oberbeckmann S, Labrenz M, et al. (2019) The eukaryotic life of microplastics in brackish environments. Front Microbiol 10: 1–13 doi: 10.3389/fmicb.2019.00001
![]() |
[29] |
Botterell Z, Beaumont N, Dorrington T, et al. (2019) Bioavailability and effects of microplastics on marine zooplankton: A review. Environ Poll 245: 98–110. doi: 10.1016/j.envpol.2018.10.065
![]() |
[30] |
de Orte MS, Clowez S, Caldeira K (2019) Response of bleached and symbiotic sea anemones to plastic microfiber exposure. Environ Poll 249: 512–517. doi: 10.1016/j.envpol.2019.02.100
![]() |
[31] |
Cole M, Lindeque P, Fileman E, et al. (2013) Microplastic ingestion by zooplankton. Envir Sci Tech 47: 6646–6655. doi: 10.1021/es400663f
![]() |
[32] |
Mohsen M, Wang Q, Zhang L, et al. (2019) Microplastic ingestion by the farmed sea cucoumber Apostichopus japonicus in China. Environ Pollut 245: 1071–1078. doi: 10.1016/j.envpol.2018.11.083
![]() |
[33] |
Grigorakus S, Mason S, Drouillard K (2017) Determination of the gut retention of plastic microbeads and microfibers in goldfish (Carassius auratus). Chemosphere 169: 233–238. doi: 10.1016/j.chemosphere.2016.11.055
![]() |
[34] |
Besseling E, Wegner A, Foekema EM, et al. (2013) Effects of microplastics on fitness and PCB bioaccumulation by the lugworm Arenicola marina (L.). Environ Sci Tech 47: 593–600. doi: 10.1021/es302763x
![]() |
[35] |
Setälä O, Fleming-Lehtinen V, Lehtiniemi M (2014) Ingestion and transfer of microplastics in the planktonic food web. Environ Poll 185: 77–83. doi: 10.1016/j.envpol.2013.10.013
![]() |
[36] |
Woods M, Stack M, Fields D, et al. (2018) Microplastic fiber uptake, ingestion, and egestion rates in blue mussel (Mytilus edulis). Mar Poll Bull 137: 638–645. doi: 10.1016/j.marpolbul.2018.10.061
![]() |
[37] |
Li L, Cai H, Rochman C, et al. (2019) The uptake of microfibers by freshwater Asian clams (Corbicula fluminea) varies based upon physicochemical properties. Chemosphere 221: 107–114. doi: 10.1016/j.chemosphere.2019.01.024
![]() |
[38] |
Watts AJ, Urbina MA, Goodhead R, et al. (2016) Effect of microplastic on the gills of the shore crab Carcinus maenas. Environ Sci Technol 50: 5364–5369. doi: 10.1021/acs.est.6b01187
![]() |
[39] |
Lu Y, Zhang Y, Deng Y, et al. (2016) Uptake and accumulation of polystyrene microplastics in zebrafish (Danio rerio) and toxic effects in liver. Envir Sci Tech 50: 4054–4060. doi: 10.1021/acs.est.6b00183
![]() |
[40] |
Collard F, Gilbert B, Compere P, et al. (2017) Microplastics in the livers of European anchovies (Engraulis encrasicolus L.). Environ Poll 229: 1000–1005. doi: 10.1016/j.envpol.2017.07.089
![]() |
[41] |
Rosenkrantz P, Chaudhry Q, Stone V, et al. (2009) A comparison of nanoparticle and fine particle uptake by Daphnia magna. Environ Toxicol Chem 28: 2142–2149. doi: 10.1897/08-559.1
![]() |
[42] | Schür C, Rist S, Baun A, et al. (2019) When fluorescence is not a particle: The tissue translocation of microplastics in Daphnia magna seems an artifact. Environ Toxicol Chem |
[43] | Farrell P, Nelson K (2013) Trophic level transfer of microplastic: Mytilus edulis (L.) to Carcinus maenas (L.). Envir Poll 177: 1–3. |
[44] |
Santana M, Moreira F, Turra A (2017) Trophic transference of microplastics under a low exposure scenario: insights on the likelihood of particle cascading along marine food webs. Mar Poll Bull 121: 154–159. doi: 10.1016/j.marpolbul.2017.05.061
![]() |
[45] |
Haegerbaeumer A, Mueller MT, Fueser H, et al. (2019) Impacts of micro-and nano-sized plastic particles on benthic invertebrates: A literature review and gap analysis. Front Envir Sci 7: 17. doi: 10.3389/fenvs.2019.00017
![]() |
[46] | Rillig M, Ziersch L, Hempel S (2017) Microplastic transport in soil by earthworms. Sci Reports 7. Article number 1362. |
[47] |
Zhu F, Zhu C, Wang C, et al. (2019) Occurrence and ecological impacts of microplastics in soil systems: A review. Bull Environ Contam Toxicol 102: 741–749. doi: 10.1007/s00128-019-02623-z
![]() |
[48] |
Prendergast-Miller M, Katsiamides A, Abbass, M, et al. (2019) Polyester-derived microfibre impacts on the soil dwelling earthworm, Lumbricus terrestris. Environ Pollut 251: 453–459. doi: 10.1016/j.envpol.2019.05.037
![]() |
[49] | Lwanga E, Gertsen H, Gooren H (2016) Microplastics in the terrestrial ecosystem: Implications for Lumbricus terrestris (Oligochaeta, Lumbricidae). Environ Sci Tech 505: 2685–2691. |
[50] |
Green D, Colgan T, Thompson R, et al. (2019) Exposure to microplastics reduces attainment strength and alters the haemolymph proteome of blue mussels (Mytilus edulis). Envir Poll 246: 423–434. doi: 10.1016/j.envpol.2018.12.017
![]() |
[51] |
Au S, Bruce T, Bridges W (2015) Responses of Hyalella azteca to acute and chronic microplastic exposures. Environ Toxicol Chem 34: 2564–2572. doi: 10.1002/etc.3093
![]() |
[52] |
Jovanovic B (2017) Ingestion of microplastic by fish and its potential consequences from a physical perspective. Integr Environ Assess Managmt 13: 510–515. doi: 10.1002/ieam.1913
![]() |
[53] |
Bour A, Haarr A, Keiter S, et al. (2018) Environmentally relevant microplastic exposure affects sediment-dwelling bivalves. Envir Poll 236: 652–660. doi: 10.1016/j.envpol.2018.02.006
![]() |
[54] |
Ziajahromi S, Kumar A, Neale P, et al. (2017) Impact of microplastic beads and fibers on waterflea (Ceriodaphnia dubia) survival, growth, and reproduction: Implications of single and mixture exposures. Environ Sci Technol 51: 13397–13406. doi: 10.1021/acs.est.7b03574
![]() |
[55] | Foley CJ, Feiner ZS, Malinich TD, et al. (2018) A meta-analysis of the effects of exposure to microplastics on fish and aquatic invertebrates. Sci Total Envir 631–632: 550–559. |
[56] | Rochman C, Hoh E, Hentschel B, et al. (2013) Long-term field measurement of sorption of organic contaminants to five types of plastic pellets: implications for plastic marine debris. Environ Sci Tech 47: 1646–1654. |
[57] |
Rochman C, Hoh E, Kurobe T, et al. (2013) Ingested plastic transfers hazardous chemicals to fish and induces hepatic stress. Sci Rep 3: 3263. doi: 10.1038/srep03263
![]() |
[58] |
Rochman C, Hentschel B, Teh S (2014) Long-term sorption of metals is similar among plastic types: implications for plastic debris in aquatic environments. PloS One 9: e85433. doi: 10.1371/journal.pone.0085433
![]() |
[59] |
Seuront L (2018) Microplastic leachates impair behavioural vigilance and predator avoidance in a temperate intertidal gastropod. Biol Lett 14: 20180453. doi: 10.1098/rsbl.2018.0453
![]() |
[60] |
Pannetier P, Morin B, Clérandeau C, et al. (2019) Toxicity assessment of pollutants sorbed on environmental microplastics collected on beaches: Part II – adverse effects on Japanese medaka early life stages. Environ Poll 248: 1098–1107. doi: 10.1016/j.envpol.2018.10.129
![]() |
[61] |
Wardrop P, Shimeta J, Nugegoda D, et al. (2016) Chemical pollutants sorbed to ingested microbeads from personal care products accumulate in fish. Environ Sci Technol 50: 4037–4044. doi: 10.1021/acs.est.5b06280
![]() |
[62] |
Beckingham B, Ghosh U (2017) Differential bioavailability of polychlorinated biphenyls associated with environmental particles: Microplastic in comparison to wood, coal and biochar. Environ Poll 220: 150–158. doi: 10.1016/j.envpol.2016.09.033
![]() |
[63] | Hodson M, Duffus-Hodson C, Clark A (2017) Plastic bag derived microplastics as a vector for metal exposure in terrestrial invertebrates. Environ Sci Tech 518: 4714–4721. |
[64] |
Tourinho P, Koci V, Loureiro S, et al. (2019) Partitioning of chemical contaminants to microplastics: Sorption mechanisms, environmental distribution and effects on toxicity and bioaccumulation. Envir Pollut 252: 1246–1256. doi: 10.1016/j.envpol.2019.06.030
![]() |
[65] |
Batel A, Linti F, Scherer M, et al. (2016) Transfer of benzo [a] pyrene from microplastics to Artemia nauplii and further to zebrafish via a trophic food web experiment: CYP1A induction and visual tracking of persistent organic pollutants. Environ Toxicol Chem 35: 1656–1666. doi: 10.1002/etc.3361
![]() |
[66] |
Bakir A, Rowland S, Thompson R (2014) Enhanced desorption of persistent organic pollutants from microplastics under simulated physiological conditions. Environ Poll 185: 16–23. doi: 10.1016/j.envpol.2013.10.007
![]() |
[67] |
Bakir A, O'Connor I, Rowland S, et al. (2016) Relative importance of microplastics as a pathway for the transfer of hydrophobic organic chemicals to marine life. Environ Poll 219: 56–65. doi: 10.1016/j.envpol.2016.09.046
![]() |
[68] |
Ziajahromi S, Kumar A, Neale P (2019) Effects of polyethylene microplastics on the acute toxicity of a synthetic pyrethroid to midge larvae (Chironomus tepperi) in synthetic and river water. Sci Total Envir 671: 971–975. doi: 10.1016/j.scitotenv.2019.03.425
![]() |
[69] |
Wang J, Coffin S, Sun C, et al. (2019) Negligible effects of microplastics on animal fitness and HOC bioaccumulation in earthworm Eisenia fetida in soil. Environ Pollut 249: 776–784. doi: 10.1016/j.envpol.2019.03.102
![]() |
[70] |
McIlwraitha H, Lina J, Erdleb L, et al. (2019) Capturing microfibers – marketed technologies reduce microfiber emissions from washing machines. Mar Poll Bull 139: 40–45. doi: 10.1016/j.marpolbul.2018.12.012
![]() |
[71] | Weis JS (2018) Cooperative work is needed between textile scientists and environmental scientists to tackle the problems of pollution by microfibers. J Textile Apparel Tech Mgmt 10: 1–3. |
[72] |
Almroth B, Åström L, Roslund S, et al. (2018) Quantifying shedding of synthetic fibers from textiles; a source of microplastics released into the environment. Envi Sci Poll Res 25: 1191–1199. doi: 10.1007/s11356-017-0528-7
![]() |
[73] |
Zambrano M, Pawlak J, Daystar J, et al. (2019) Microfibers generated from the laundering of cotton, nylon, and polyester based fabrics and their aquatic degradation. Mar Poll Bull 142: 394–407. doi: 10.1016/j.marpolbul.2019.02.062
![]() |
[74] |
Belzagui F, Crespi M, Álvarez A, et al. (2019) Microplastics' emission: microfibers' detachment from textile garments. Envir Poll 248: 1028–1035. doi: 10.1016/j.envpol.2019.02.059
![]() |
[75] |
Pena-Francesch A, Demirel M (2019) Squid-inspired tandem repeat proteins: Functional fibers and films. Front Chem 7: 69. doi: 10.3389/fchem.2019.00069
![]() |
1. | Nicola A. Forster, Matthew K. Tighe, Susan C. Wilson, Microplastics in soils of wilderness areas: What is the significance of outdoor clothing and footwear?, 2020, 378, 00167061, 114612, 10.1016/j.geoderma.2020.114612 | |
2. | Judith S. Weis, Aquatic Microplastic Research—A Critique and Suggestions for the Future, 2020, 12, 2073-4441, 1475, 10.3390/w12051475 | |
3. | Stephen D. Burrows, Simona Frustaci, Kevin V. Thomas, Tamara Galloway, Expanding exploration of dynamic microplastic surface characteristics and interactions, 2020, 130, 01659936, 115993, 10.1016/j.trac.2020.115993 | |
4. | Thilakshani Atugoda, Hansika Piyumali, Sureka Liyanage, Kushani Mahatantila, Meththika Vithanage, 2020, Chapter 42-1, 978-3-030-10618-8, 1, 10.1007/978-3-030-10618-8_42-1 | |
5. | Benjamin O. Asamoah, Emilia Uurasjärvi, Jukka Räty, Arto Koistinen, Matthieu Roussey, Kai-Erik Peiponen, Towards the Development of Portable and In Situ Optical Devices for Detection of Micro-and Nanoplastics in Water: A Review on the Current Status, 2021, 13, 2073-4360, 730, 10.3390/polym13050730 | |
6. | Arianna Bellasi, Gilberto Binda, Andrea Pozzi, Silvia Galafassi, Pietro Volta, Roberta Bettinetti, Microplastic Contamination in Freshwater Environments: A Review, Focusing on Interactions with Sediments and Benthic Organisms, 2020, 7, 2076-3298, 30, 10.3390/environments7040030 | |
7. | Agathe Bour, Monica Hamann Sandgaard, Kristian Syberg, Annemette Palmqvist, Bethanie Carney Almroth, Comprehending the complexity of microplastic organismal exposures and effects, to improve testing frameworks, 2021, 415, 03043894, 125652, 10.1016/j.jhazmat.2021.125652 | |
8. | Theresa Stoll, Peter Stoett, Joanna Vince, Britta Denise Hardesty, 2020, Chapter 26-1, 978-3-030-10618-8, 1, 10.1007/978-3-030-10618-8_26-1 | |
9. | Adrián López-Rosales, Jose M. Andrade, Purificación López-Mahía, Soledad Muniategui-Lorenzo, Development of an analytical procedure to analyze microplastics in edible macroalgae using an enzymatic-oxidative digestion, 2022, 183, 0025326X, 114061, 10.1016/j.marpolbul.2022.114061 | |
10. | Thilakshani Atugoda, Hansika Piyumali, Sureka Liyanage, Kushani Mahatantila, Meththika Vithanage, 2022, Chapter 42, 978-3-030-39040-2, 781, 10.1007/978-3-030-39041-9_42 | |
11. | Arianna Bellasi, Gilberto Binda, Ginevra Boldrocchi, Andrea Pozzi, Roberta Bettinetti, What Are Lake Beaches Made of? An Assessment of Plastic Beach Litter on the Shores of Como Bay (Italy), 2022, 12, 2076-3417, 5388, 10.3390/app12115388 | |
12. | Cecilia Askham, Valentina H. Pauna, Anne-Marie Boulay, Peter Fantke, Olivier Jolliet, Jérôme Lavoie, Andy M. Booth, Claire Coutris, Francesca Verones, Miriam Weber, Martina G. Vijver, Amy Lusher, Carla Hajjar, Generating environmental sampling and testing data for micro- and nanoplastics for use in life cycle impact assessment, 2023, 859, 00489697, 160038, 10.1016/j.scitotenv.2022.160038 | |
13. | Renjith VishnuRadhan, Divya David Thresyamma, T I Eldho, Jacky Bhagat, Atmospheric plastics- a potential airborne fomite with an emerging climate signature, 2021, 3, 26672782, 100037, 10.1016/j.joclim.2021.100037 | |
14. | Dalia Saad, 2023, 0, 2754-6713, 10.5772/intechopen.109173 | |
15. | Widya Ningsih, Khusnul Yaqin, Sri Wahyuni Rahim, Microplastic contamination in coastal waters of South Larompong, Luwu, South Sulawesi, Indonesia, 2022, 6, 2598-8298, 101, 10.29239/j.akuatikisle.6.2.101-108 | |
16. | Xue Zhang, Naifan Hu, Zhenfan Yu, Yurun Du, Yu Liu, Huihui Wang, Lining Pu, Xiaoxue He, Degong Pan, Shulan He, Hongyan Qiu, Jiangping Li, Research tendency of microplastics and nanoplastics based on bibliometric analysis and perspective of the influence of human health, 2022, 4, 2515-7620, 095004, 10.1088/2515-7620/ac8bc8 | |
17. | Mahdieh Samavi, Niravkumar Mahendrasinh Kosamia, Ellen Caroline Silverio Vieira, Zannat Mahal, Sudip Kumar Rakshit, 2023, 9780323999083, 125, 10.1016/B978-0-323-99908-3.00011-7 | |
18. | Aadhityaa Mohanavelu, Shivansh Shrivastava, Sujay Raghavendra Naganna, Streambed pollution: A comprehensive review of its sources, eco-hydro-geo-chemical impacts, assessment, and mitigation strategies, 2022, 300, 00456535, 134589, 10.1016/j.chemosphere.2022.134589 | |
19. | Theresa Stoll, Peter Stoett, Joanna Vince, Britta Denise Hardesty, 2022, Chapter 26, 978-3-030-39040-2, 1129, 10.1007/978-3-030-39041-9_26 | |
20. | Etty Riani, Muhammad Reza Cordova, Assessment of Sediment Grain Size and Its Correlation with Microplastic Accumulation and Characteristics in the Kahayan River, Indonesia, 2024, 1527-5922, 1, 10.1080/15275922.2023.2297434 | |
21. | Shreya Roy, Dhruba Jyoti Sarkar, Nabanita Chakraborty, Kausik Mondal, Basanta Kumar Das, Bioaccumulation of polystyrene microplastics and changes in antioxidant and AChE pattern in a freshwater snail (Filopaludina bengalensis) from river Ganga, 2023, 263, 0166445X, 106697, 10.1016/j.aquatox.2023.106697 | |
22. | Sandra E. Shumway, Kayla Mladinich, Noreen Blaschik, Bridget A. Holohan, J. Evan Ward, A Critical Assessment of Microplastics in Molluscan Shellfish with Recommendations for Experimental Protocols, Animal Husbandry, Publication, and Future Research, 2023, 2330-8249, 1, 10.1080/23308249.2023.2216301 | |
23. | Todd Gouin, Robert Ellis-Hutchings, Mark Pemberton, Bianca Wilhelmus, Addressing the relevance of polystyrene nano- and microplastic particles used to support exposure, toxicity and risk assessment: implications and recommendations, 2024, 21, 1743-8977, 10.1186/s12989-024-00599-1 | |
24. | Maura Gabriela da Silva Brochado, Iara Barbosa Magalhães, Júlia Martins Soares, Alessandro da Costa Lima, Laura Bordignon, Allana Grecco Guedes, Mariana Ramírez Castillo, Kassio Ferreira Mendes, Does Microplastic Contamination in Agricultural Soils Decrease the Efficiency of Herbicides for Weed Control?, 2024, 3, 2673-8929, 771, 10.3390/microplastics3040048 | |
25. | Xin Li Ching, Syamsyahidah Samsol, Mohd Uzair Rusli, Mohamad Aqmal-Naser, Joseph Anak Bidai, Christian Sonne, Xin Wu, Nyuk Ling Ma, Blood and cloacal microbiome profile of captive green turtles (Chelonia mydas) and hawksbill turtles (Eretmochelys imbricata): Water quality and conservation implications, 2025, 375, 00456535, 144223, 10.1016/j.chemosphere.2025.144223 | |
26. | Toheeb Lekan Jolaosho, Mariam Folashade Rasaq, Eniola Victoria Omotoye, Oluwadamilola Victoria Araomo, Opeyemi Shakirat Adekoya, Opeyemi Yusuf Abolaji, Jesuyon Joseph Hungbo, Microplastics in freshwater and marine ecosystems: Occurrence, characterization, sources, distribution dynamics, fate, transport processes, potential mitigation strategies, and policy interventions, 2025, 294, 01476513, 118036, 10.1016/j.ecoenv.2025.118036 |