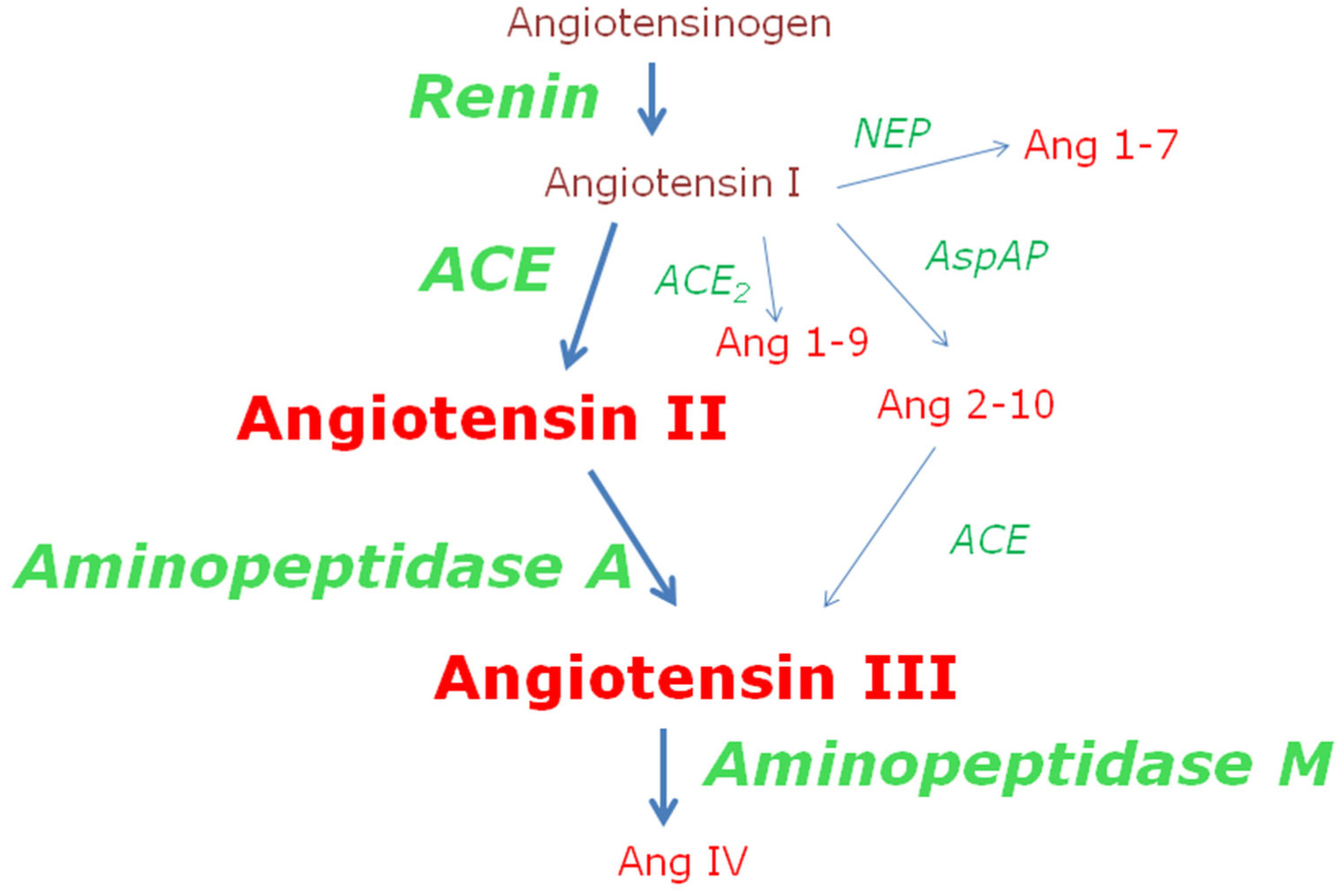
Citation: I. Banegas, I. Prieto, A.B. Segarra, M. Martínez-Cañamero, M. de Gasparo, M. Ramírez-Sánchez. Angiotensin II, dopamine and nitric oxide. An asymmetrical neurovisceral interaction between brain and plasma to regulate blood pressure[J]. AIMS Neuroscience, 2019, 6(3): 116-127. doi: 10.3934/Neuroscience.2019.3.116
[1] | AB Segarra, I Prieto, M Martínez-Cañamero, Manuel Ramírez-Sánchez . Is there a link between depression, neurochemical asymmetry and cardiovascular function?. AIMS Neuroscience, 2020, 7(4): 360-372. doi: 10.3934/Neuroscience.2020022 |
[2] | I. Banegas, I. Prieto, A.B. Segarra, M. de Gasparo, M. Ramírez-Sánchez . Study of the Neuropeptide Function in Parkinson’s Disease Using the 6-Hydroxydopamine Model of Experimental Hemiparkinsonism. AIMS Neuroscience, 2017, 4(4): 223-237. doi: 10.3934/Neuroscience.2017.4.223 |
[3] | Amber Woodard, Brandon Barbery, Reid Wilkinson, Jonathan Strozyk, Mathew Milner, Patrick Doucette, Jarred Doran, Kendra Appleby, Henry Atwill, Wade E. Bell, James E. Turner . The role of neuronal nitric oxide and its pathways in the protection and recovery from neurotoxin-induced de novo hypokinetic motor behaviors in the embryonic zebrafish (Danio rerio). AIMS Neuroscience, 2019, 6(1): 25-42. doi: 10.3934/Neuroscience.2019.1.25 |
[4] | Nicholas J. D. Wright . A review of the actions of Nitric Oxide in development and neuronal function in major invertebrate model systems. AIMS Neuroscience, 2019, 6(3): 146-174. doi: 10.3934/Neuroscience.2019.3.146 |
[5] | Valentina Bashkatova, Athineos Philippu . Role of nitric oxide in psychostimulant-induced neurotoxicity. AIMS Neuroscience, 2019, 6(3): 191-203. doi: 10.3934/Neuroscience.2019.3.191 |
[6] | Gigi Tevzadze, Tamar Barbakadze, Elisabed Kvergelidze, Elene Zhuravliova, Lali Shanshiashvili, David Mikeladze . Gut neurotoxin p-cresol induces brain-derived neurotrophic factor secretion and increases the expression of neurofilament subunits in PC-12 cells. AIMS Neuroscience, 2022, 9(1): 12-23. doi: 10.3934/Neuroscience.2022002 |
[7] | Madhura Kulkarni-Chitnis, Leah Mitchell-Bush, Remmington Belford, Jenaye Robinson, Catherine A. Opere, Sunny E. Ohia, Ya Fatou N. Mbye . Interaction between hydrogen sulfide, nitric oxide, and carbon monoxide pathways in the bovine isolated retina. AIMS Neuroscience, 2019, 6(3): 104-115. doi: 10.3934/Neuroscience.2019.3.104 |
[8] | Md. Mamun Al-Amin, Robert K. P. Sullivan, Suzy Alexander, David A. Carter, DanaKai Bradford, Thomas H. J. Burne . Impaired spatial memory in adult vitamin D deficient BALB/c mice is associated with reductions in spine density, nitric oxide, and neural nitric oxide synthase in the hippocampus. AIMS Neuroscience, 2022, 9(1): 31-56. doi: 10.3934/Neuroscience.2022004 |
[9] | Milad Khodavysi, Nejat Kheiripour, Hassan Ghasemi, Sara Soleimani-Asl, Ali Fathi Jouzdani, Mohammadmahdi Sabahi, Zahra Ganji, Zahra Azizi, Akram Ranjbar . How can nanomicelle-curcumin modulate aluminum phosphide-induced neurotoxicity?: Role of SIRT1/FOXO3 signaling pathway. AIMS Neuroscience, 2023, 10(1): 56-74. doi: 10.3934/Neuroscience.2023005 |
[10] | Zinia Pervin, Julia M Stephen . Effect of alcohol on the central nervous system to develop neurological disorder: pathophysiological and lifestyle modulation can be potential therapeutic options for alcohol-induced neurotoxication. AIMS Neuroscience, 2021, 8(3): 390-413. doi: 10.3934/Neuroscience.2021021 |
It is clearly accepted that the regulation of vital functions such as blood pressure is carried out through the equilibrium between various factors, sometimes with opposite functions, such as those that increase or decrease cardiac output, blood volume or peripheral resistance [1]. In the same way, for the control of blood pressure, a correct interaction between central and peripheral mechanisms is involved [2]–[4]. Furthermore, complex interactions between environmental extrinsic factors and behavioral ones and intrinsic mechanisms of cardiovascular regulation are implicated [5]. In addition, the recent interest in the relation of the brain and peripheral asymmetric organization of the nervous system for the control of blood pressure is increasing [3],[6]–[10]. Among the factors that interact at all these levels, the role of the renin-angiotensin aldosterone system, dopamine and nitric oxide is highlighted [7]. The present article analyzes the most significant reports indicating how these factors interact from a bilateral point of view and provides new relevant data for the understanding of this complex system.
The role of the renin-angiotensin aldosterone system (RAAS) is essential in the control of blood pressure. In fact, most of the anti-hypertensive treatment's protocols include drugs that inhibit or block the RAAS [11] at various levels such as the peripheral inhibition of renin or angiotensin-converting enzyme (ACE), the central inhibition of aminopeptidase A or the blockade of the angiotensin (Ang) II receptor [12]. In the RAAS, the function of the active peptides of the cascade depends on the activity of the enzymes controlling the formation or inactivation of these peptides and not on a RNA-dependent mechanism. Only angiotensinogen, an inactive peptide precursor of the cascade, requires such RNA-dependent mechanism. Therefore, the complex regulation of the formation and inactivation of the various angiotensins, some of them with opposite functions, is conditioned by a subtle coordination between the activities of the enzymes that make up the system (Figure 1) [12]–[15].
The function of brain angiotensin-hydrolyzing aminopeptidases in hypertension has been studied by various authors. Wright et al. [16] showed that after the intracerebroventricular (icv) injection of Ang II and Ang III in spontaneously hypertensive rats (SHR), more sensitivity and a more prolonged increase in blood pressure were observed than in normotensive Wistar-Kyoto rats (WKY). Further, pretreatment with bestatin (an aminopeptidase M inhibitor) preventing the inactivation Ang III potentiated and prolonged the increase of blood pressure after the injection of Ang II and Ang III in SHR. Wright et al. [17] also demonstrated that the icv injection of aminopeptidase M reduced significantly blood pressure in both WKY and SHR due to the central inactivation of Ang III. Llorens-Cortes and her research team also analyzed the role of cerebral aminopeptidase A and M in the control of blood pressure and hydro-electrolytic balance. They demonstrated that the action of hypothalamic Ang II on the release of vasopressin depends on the previous biotransformation of Ang II to Ang III by the action of aminopeptidase A [18]. The subsequent inhibition of aminopeptidase M induces an increase in the release of vasopressin because it enhances and prolongs the action of Ang III. A promising therapeutic strategy in the treatment of hypertension may consist in the development of new drugs aimed at reducing the formation of Ang III through the inhibition of aminopeptidase A [13]–[15].
Therefore, a key role in the central and peripheral function of the RAAS is linked to aminopeptidase A (EC 3.4.11.7) and aminopeptidase M (EC 3.4.11.2) respectively responsible for inactivating angiotensin II and promoting the formation of angiotensin III, and for inactivating angiotensin III [12].
The neuronal bodies, from which all the cerebral dopamine originates, are located in the brainstem, mainly in the pars compact of the substantia nigra and in the ventrotegmental area. Its brain distribution is unilateral without bilateral crossing [19] (Figure 2).
The relationship between dopamine and the control of blood pressure has been known for quite a while: whereas its central administration decreased blood pressure, the intravenous injection increased it [20]. The hypertensive effect of peripheral dopamine administration was abolished by treatment with the alpha-adrenergic receptor blocker phentolamine. In contrast, the decrease in blood pressure induced by the central administration of dopamine was not affected by pre-treatment with phentolamine but it was blocked by metoclopramide, an antagonist of the central D2 receptors. Further, central administration of metoclopramide decreases blood pressure suggesting that central D2 receptors, more than peripheral alpha adrenergic receptors, are involved in the control of blood pressure. The same authors proposed that the regulatory role of the brain dopaminergic system on blood pressure is linked to changes in the RAAS [20]. More recent studies suggest that, in basal conditions, dopamine and its receptors acting through a natriuretic effect counteract the hypertensive effect of the stimulation of alpha-adrenergic receptors and the action of the hypertensive components of the RAAS at the peripheral level. An abnormal interaction of such factors could be involved in the pathogenesis of hypertension [21].
Nitric oxide is a gas, highly diffusible through cell membranes, which produces vasodilatation and therefore is of hypotensive nature. It also acts as a neurotransmitter with important functions such as synaptic plasticity or memory processes. It is therefore an important central and peripheral regulator of the blood pressure [22]. It is produced by the action of various nitric oxide synthases (NOS) (EC 1.14.13.39): endothelial (eNOS), inducible (iNOS) and neuronal (nNOS). Due to the impossibility of limiting its diffusion between compartments, the nitric oxide produced by the action of eNOS or iNOS will be able to diffuse towards the nervous system: alterations in its plasma levels may produce not only alterations of its peripheral functions related to the vascular tone [23], but also contribute to alter the normal interaction exerted by the central nitric oxide with other blood pressure regulating factors, changing the central vascular function [24] and/or participating in the pathogenesis of various neurodegenerative diseases [25], schizophrenia and other psychiatric disorders such as bipolar disorders or depression [26]. For example, the decrease in the availability of nitric oxide has been related to its cortical and peripheral reduction that occurs during aging and with the severity of Alzheimer's disease [24]. Reduced levels of nitric oxide in plasma have also been associated with the pathophysiology of negative symptoms in schizophrenia [27].
Although nNOS has been studied primarily in brain, it is also found peripherally in perivascular nerves, heart or skeletal muscle. Therefore, although the importance of eNOS in cardiovascular regulation is clearly established, the participation of nNOS in the physiological regulation of systemic vascular resistance and blood pressure in healthy humans by modulating sympathetic activity, renal release of renin, hydro-electrolytic balance as well as cardiac function is also proposed [28].
Ischemic or hemorrhagic stroke is a serious acute cerebrovascular process in which nitric oxide could play an important role due to its essential role in the maintenance of vascular tone. It has been suggested that nitric oxide derived from the action of iNOS and nNOS have neurotoxic character while nitric oxide derived from the action of eNOS have a neuroprotective role in acute ischemic stroke. In relation to cerebral hemorrhage, there is no clear differentiation in the role that nitric oxide derived from the different NOS isoenzymes can exert, suggesting that an increase in nitric oxide levels after cerebral hemorrhage would improve the prognosis of these patients [29]. This has been hypothesized on the basis of plasma studies in animal models with subarachnoid hemorrhage [30] and in patients after acute stroke [31]. More recently, data supporting a relationship of eNOS with the cognitive alteration that occurs after an acute ischemic stroke have been provided [32].
The concept of “Neurovisceral Integration” in the control of blood pressure is more than 100 years old [33],[34]. However, diverse data are recently accumulating which extend the idea that the bidirectional interaction between brain and peripheral tissues for the control of blood pressure is asymmetrically organized [3].
We have contributed to studies that suggest a bidirectional connection between the brain and various peripheral tissues, such as heart and kidney, for the control of blood pressure and hydro-electrolytic balance: these involve several enzymes of the RAAS cascade. Such neuro-visceral interaction is modified by changes in blood pressure induced by the use of vasoactive hypertensive or hypotensive drugs and suggests to be mediated by the autonomic nervous system [2].
On the other hand, changes in blood pressure induce variations in the bilateral brain distribution of aminopeptidase activities as well as in the intra-hemispheric and inter-hemispheric interactions established between several of these enzymes [10]. While in normotensive animals, and in those animals that were treated with the hypotensive drug captopril, appeared a predominance of intra-hemispheric correlations into the left hemisphere, the hypertensive animals and those treated with the hypertensive drug L-NAME exhibited it into the right one. An asymmetry was also observed in the inter-hemispheric correlations that were established between aminopeptidase activities of the left and right hemisphere: there were more inter-hemispheric correlations in normotensive animals and in those treated with antihypertensive drugs than in hypertensive patients or those treated with hypertensive drugs [10].
Finally, other data suggest that this neurovisceral interaction between aminopeptidases that hydrolyze angiotensin peptides in the control of blood pressure is asymmetrically organized. An asymmetry has been observed in the correlation established between the frontal cortex and the plasma in hypertensive animals: while in hypertensive animals a correlation between plasma and the right frontal cortex is observed, such correlation is established with the left frontal cortex after the treatment with captopril and the related reduction of blood pressure [35]. The relationship established between the frontal cortex and the cardiac tissue, specifically the left ventricle, is also asymmetric being the opposite in comparison with the correlation observed with plasma: the most significant correlation between frontal cortex and ventricle was observed with the right frontal cortex in the animals treated with captopril compared with the control group of hypertensive animals [36].
All these previously reviewed factors, renin-angiotensin aldosterone system, dopamine, nitric oxide and asymmetry in neurovisceral integration for the control of blood pressure could be inter-connected.
The asymmetric behavior of the relationship between nitric oxide, dopamine and RAAS in blood pressure control was not apparent until the work of Banegas et al. [7]. The unilateral distribution of dopamine, without inter-hemispheric crossing, offers us a valuable tool to selectively analyze the response of one or the other side depending on whether we eliminate the dopamine from the left or the right hemisphere [19]. The results demonstrated that blood pressure levels as well as aminopeptidase activities in brain and plasma and nitric oxide in plasma varied depending on whether the animals were normotensive or hypertensive and whether the injured hemisphere (to deplete dopamine) was the left or the right one. The interaction between factors also varied depending on whether the lesion was performed on one side of the brain or the other [7]–[9] (Figure 3).
The asymmetric character of Parkinson's disease produced by a lateralized distribution of brain dopamine is not only manifested by lateralized consequences of motor behavior but also by the rise of cognitive and autonomic changes including cardiovascular alterations [37] in which the nitric oxide may have a relevant role [38].
Banegas et al. [8] showed a marked increase in blood pressure in animals in which dopamine had been depleted from the left hemisphere following left unilateral injection of the neurotoxic 6-hydroxydopamine in comparison with the right side lesions that did not induce variations in blood pressure or these were much less pronounced. Because heart rate in these animals did not change after lesion, the authors suggested that the marked increase of blood pressure in the animals with left lesions was due to an increase in the peripheral resistances rather than an increase in cardiac output, a mechanism linked to an asymmetry in the vascular autonomic control. Alternatively, since an asymmetrical baroreceptor activity was reported, possibly due in part to a lateralization of tyroxine hydroxylase activity in the median eminence [39], the left or right depletion of dopamine could influence differentially on blood pressure blunting asymmetrically the baroreceptor activity.
The asymmetric behavior after the left or right unilateral lesions of the nigrostriatal system is not only reflected in changes in blood pressure but also extends to differential changes in the levels of plasma factors which are depending on whether the lesion occurred on the left side or in the right one. Among others, nitric oxide and aminopeptidase A are modified in plasma. While in normotensive animals (WKY) the left lesion increases plasma nitric oxide and reduces aminopeptidase A, the right lesion does not modify either of the two factors. In contrast, in hypertensive animals (SHR) while the left lesion does not modify nitric oxide and decreases aminopepidase A, the right lesion decreases nitric oxide and increases aminopeptidase A. This is just the inverse behavior to what happened in normotensive animals with left lesion (Figure 3). If we take into account the ratio “high enzymatic activity/low level of hydrolyzed substrate” and vice versa (Figure 1), we can suggest that the left lesion maintains elevated plasma levels of angiotensin II in WKY and SHR whereas the right lesion does not modify (in WKY) or decreases them (in SHR).
The correlation study between nitric oxide and plasma aminopeptidase A in the various groups studied showed significant negative correlations (the higher nitric oxide level, the lower aminopeptidase A and vice versa) between aminopeptidase A and nitric oxide in the WKY group with right lesion and in the group of SHR with left lesion.
Banegas et al. [9] described the bilateral behavior of aminopeptidase M activity and its role as enkephalinase in rats with left or right lesions of the nigrostriatal system [40]. However, this enzyme also hydrolyzes angiotensin III [41] (Figure 1). It is therefore interesting to analyze these results considering this other enzymatic target. It has been demonstrated that angiotensin III is the most active peptide of the RAAS in the brain and its cerebral function is clearly hypertensive [41]. In comparison with control animals, left side-lesioned WKY animals showed a decrease in aminopeptidase M in the left hemisphere and an increase in the right one. In SHR, the left lesion produces exactly the opposite: an increase in the left hemisphere and a decrease in the right one. The right lesion in WKY did not modify the activity of aminopeptidase M in comparison with the control animals. In contrast, in the SHR this activity decreased in the left side and increased in the right one (Figure 4).
If we consider the relationship “high enzymatic activity/low concentration of the substrate” and vice versa, we would obtain angiotensin III levels that would correspond to the inverse behavior of the previously described one for aminopeptidase M i.e. in the left lesion WKY animals, the left hemisphere would have elevated levels of angiotensin III and the right one decreased values compared with control animals. In comparison with the WKY, the left lesion in the SHR would result in an opposite response: low levels of Angiotensin III in the left side and high levels in the right one. Also, the right lesion in SHR increases Ang III in the left side but decreases it in the right one (Figure 4). This is in contrast with WKY where the right lesion does not modify the aminopeptidase M (and consequently Ang III) in any of the hemispheres compared to controls.
Physiology is an integrating discipline, in which it is clearly shown that there are no independent compartments in the functioning of the organism but it works as an integrated whole. The control of cardiovascular function is an example of this integration. In this review we have discussed how factors with very diverse functions and from several locations can interact with each other for the control of blood pressure. From our results, it is also deduced that the neurovisceral integration for the control of blood pressure is organized asymmetrically and the response is not the same if the alteration occur on the left or right side of the brain. In relation to cardiovascular function, we could hypothesize from these results a greater severity in the processes that affect the left hemisphere than in those involving the right one. This situation would have to be taken into account in processes such as for example Parkinson's disease or schizophrenia in which an asymmetric development and evolution of the disease is clearly demonstrated. The vascular and/or autonomic consequences will vary depending on the alteration of the nitric oxide if the process involves one side or the other of the brain. Indeed, in brain strokes involving the left or right hemisphere, we have been able to confirm that the response in the plasma levels of nitric oxide varies depending on the injured side. A possible beneficial treatment aimed to restore nitric oxide levels will therefore depend on the affected brain side. The understanding of the bilateral behavior of the interaction of multiple factors with functions apparently opposite in some cases constitutes a complex challenge. Thus further studies are necessary to structure the bilateral behavior of the nervous system and the peripheral response in the control of blood pressure.
[1] |
Oparil S, Acelajado MC, Bakris GL, et al. (2018) Hypertension. Nat Rev Dis Primers 4: 18014. doi: 10.1038/nrdp.2018.14
![]() |
[2] |
Prieto I, Villarejo AB, Segarra AB, et al. (2014) Brain, heart and kidney correlate for the control of blood pressure and water balance: role of angiotensinases. Neuroendocrinology 100: 198–208. doi: 10.1159/000368835
![]() |
[3] |
Prieto I, Segarra AB, Martinez-Canamero M, et al. (2017) Bidirectional asymmetry in the neurovisceral communication for the cardiovascular control: New insights. Endocr Regul 51: 157–167. doi: 10.1515/enr-2017-0017
![]() |
[4] | Prieto I, Segarra AB, de Gasparo M, et al. (2018) Divergent profile between hypothalamic and plasmatic aminopeptidase activities in WKY and SHR. Influence of beta-adrenergic blockade. Life Sci 192: 9–17. |
[5] |
Parati G, Ochoa JE, Lombardi C, et al. (2013) Assessment and management of blood-pressure variability. Nat Rev Cardiol 10: 143–155. doi: 10.1038/nrcardio.2013.1
![]() |
[6] |
Banegas I, Prieto I, Vives F, et al. (2006) Brain aminopeptidases and hypertension. J Renin Angiotensin Aldosterone Syst 7: 129–134. doi: 10.3317/jraas.2006.021
![]() |
[7] |
Banegas I, Prieto I, Vives F, et al. (2009) Asymmetrical response of aminopeptidase A and nitric oxide in plasma of normotensive and hypertensive rats with experimental hemiparkinsonism. Neuropharmacology 56: 573–579. doi: 10.1016/j.neuropharm.2008.10.011
![]() |
[8] |
Banegas I, Prieto I, Segarra AB, et al. (2011) Blood pressure increased dramatically in hypertensive rats after left hemisphere lesions with 6-hydroxydopamine. Neurosci Lett 500: 148–150. doi: 10.1016/j.neulet.2011.06.025
![]() |
[9] |
Banegas I, Prieto I, Segarra AB, et al. (2017) Bilateral distribution of enkephalinase activity in the medial prefrontal cortex differs between WKY and SHR rats unilaterally lesioned with 6-hydroxydopamine. Prog Neuropsychopharmacol Biol Psychiatry 75: 213–218. doi: 10.1016/j.pnpbp.2017.02.015
![]() |
[10] |
Prieto I, Segarra AB, Villarejo AB, et al. (2019) Neuropeptidase activity in the frontal cortex of Wistar-Kyoto and spontaneously hypertensive rats treated with vasoactive drugs: a bilateral study. J Hypertens 37: 612–628. doi: 10.1097/HJH.0000000000001884
![]() |
[11] | Cuspidi C, Tadic M, Grassi G, et al. (2018) Mancia G. Treatment of hypertension: The ESH/ESC guidelines recommendations. Pharmacol Res 128: 315–321. |
[12] |
Ramírez-Sánchez M, Prieto I, Wangensteen R, et al. (2013) The renin-angiotensin system: new insight into old therapies. Curr Med Chem 20: 1313–1322. doi: 10.2174/0929867311320100008
![]() |
[13] |
Ferdinand KC, Balavoine F, Besse B, et al. (2019) Efficacy and safety of Firibastat, a first-in-class brain aminopeptidase A inhibitor, in hypertensive overweight patients of multiple ethnic origins: A Phase 2, open-label, multicenter, dose-titrating study. Circulation 140: 138–146. doi: 10.1161/CIRCULATIONAHA.119.040070
![]() |
[14] |
Gao J, Marc Y, Iturrioz X, et al. (2014) A new strategy for treating hypertension by blocking the activity of the brain renin-angiotensin system with aminopeptidase A inhibitors. Clin Sci (Lond) 127: 135–148. doi: 10.1042/CS20130396
![]() |
[15] |
Keck M, De Almeida H, Compère D, et al. (2019) NI956/QGC006, a potent orally active, brain-penetrating aminopeptidase a inhibitor for treating hypertension. Hypertension 73: 1300–1307. doi: 10.1161/HYPERTENSIONAHA.118.12499
![]() |
[16] |
Wright JW, Mizutani S, Murray CE, et al. (1990) Aminopeptidase-induced elevations and reductions in blood pressure in the spontaneously hypertensive rat. J Hypertens 8: 969–974. doi: 10.1097/00004872-199010000-00013
![]() |
[17] |
Wright JW, Jensen LL, Cushing LL, et al. (1989) Leucine aminopeptidase M-induced reductions in blood pressure in spontaneously hypertensive rats. Hypertension 13: 910–915. doi: 10.1161/01.HYP.13.6.910
![]() |
[18] |
Zini S, Masdehors P, Lenkei Z, et al. (1997) Aminopeptidase A: distribution in rat brain nuclei and increased activity in spontaneously hypertensive rats. Neuroscience 78: 1187–1193. doi: 10.1016/S0306-4522(96)00660-4
![]() |
[19] |
Banegas I, Prieto I, Segarra AB, et al. (2017) Study of the neuropeptide function in Parkinson's disease using the 6-Hydroxydopamine model of experimental Hemiparkinsonism. AIMS Neuroscience 4: 223–237. doi: 10.3934/Neuroscience.2017.4.223
![]() |
[20] |
Kondo K, Ebihara A, Suzuki H, et al. (1981) Role of dopamine in the regulation of blood pressure and the renin--angiotensin-aldosterone system in conscious rats. Clin Sci (Lond) 61: 235s–237s. doi: 10.1042/cs061235s
![]() |
[21] |
Zeng C, Jose PA (2011) Dopamine receptors: important antihypertensive counterbalance against hypertensive factors. Hypertension 57: 11–17. doi: 10.1161/HYPERTENSIONAHA.110.157727
![]() |
[22] |
Förstermann U, Sessa WC (2012) Nitric oxide synthases: regulation and function. Eur Heart J 33: 829–837. doi: 10.1093/eurheartj/ehr304
![]() |
[23] | Calver A, Collier J, Vallance P (1993) Nitric oxide and the control of human vascular tone in health and disease. Eur J Med 2: 48–53. |
[24] |
Venturelli M, Pedrinolla A, Boscolo Galazzo I, et al. (2018) Impact of nitric oxide bioavailability on the progressive cerebral and peripheral circulatory impairments during aging and Alzheimer's disease. Front Physiol 9: 169. doi: 10.3389/fphys.2018.00169
![]() |
[25] |
Steinert JR, Chernova T, Forsythe ID (2010) Nitric oxide signaling in brain function, dysfunction, and dementia. Neuroscientist 16: 435–452. doi: 10.1177/1073858410366481
![]() |
[26] | Maia-de-Oliveira JP, Trzesniak C, Oliveira IR, et al. (2012) Nitric oxide plasma/serum levels in patients with schizophrenia: a systematic review and meta-analysis. Braz J Psychiatry 34: S149–155. |
[27] |
Nakano Y, Yoshimura R, Nakano H, et al. (2010) Association between plasma nitric oxide metabolites levels and negative symptoms of schizophrenia: a pilot study. Hum Psychopharmacol 25: 139–144. doi: 10.1002/hup.1102
![]() |
[28] |
Shabeeh H, Khan S, Jiang B, et al. (2017) Blood pressure in healthy humans is regulated by neuronal no synthase. Hypertension 69: 970–976. doi: 10.1161/HYPERTENSIONAHA.116.08792
![]() |
[29] |
Chen ZQ, Mou RT, Feng DX, et al. (2017) The role of nitric oxide in stroke. Med Gas Res 7: 194–203. doi: 10.4103/2045-9912.215750
![]() |
[30] |
Zheng R, Qin L, Li S, et al. (2014) CT perfusion-derived mean transit time of cortical brain has a negative correlation with the plasma level of nitric oxide after subarachnoid hemorrhage. Acta Neurochir (Wien) 156: 527–533. doi: 10.1007/s00701-013-1968-6
![]() |
[31] |
Taffi R, Nanetti L, Mazzanti L, et al. (2008) Plasma levels of nitric oxide and stroke outcome. J Neurol 255: 94–98. doi: 10.1007/s00415-007-0700-y
![]() |
[32] |
Li S, Wang Y, Jiang Z, et al. (2018) Impaired cognitive performance in endothelial nitric oxide synthase knockout mice after ischemic stroke: A pilot study. Am J Phys Med Rehabil 97: 492–499. doi: 10.1097/PHM.0000000000000904
![]() |
[33] | Bernard C (1878) Etude sur la physiologie du coeur. La science experimentale: 316–366. Available from: https://gallica.bnf.fr/ark:/12148/bpt6k69992b/f316.image. |
[34] |
Thayer JF, Lane RD (2009) Claude Bernard and the heart-brain connection: further elaboration of a model of neurovisceral integration. Neurosci Biobehav Rev 33: 81–88. doi: 10.1016/j.neubiorev.2008.08.004
![]() |
[35] |
Segarra AB, Prieto I, Banegas I, et al. (2012) Asymmetrical effect of captopril on the angiotensinase activity in frontal cortex and plasma of the spontaneously hypertensive rats: expanding the model of neuroendocrine integration. Behav Brain Res 230: 423–427. doi: 10.1016/j.bbr.2012.02.039
![]() |
[36] | Segarra AB, Prieto I, Banegas I, et al. (2013) The brain-heart connection: frontal cortex and left ventricle angiotensinase activities in control and captopril-treated hypertensive rats-a bilateral study. Int J Hypertens 2013: 156179. |
[37] | Segarra AB, Banegas I, Prieto I, et al. (2016) Brain asymmetry and dopamine: beyond motor implications in Parkinson's disease and experimental hemiparkinsonism. Rev Neurol 63: 415–421. |
[38] |
de Jager L, Amorim EDT, Lucchetti BFC, et al. (2018) Nitric oxide alterations in cardiovascular system of rats with Parkinsonism induced by 6-OHDA and submitted to previous exercise. Life Sci 204: 78–86. doi: 10.1016/j.lfs.2018.05.017
![]() |
[39] |
Alexander N, Kaneda N, Ishii A, et al. (1990) Right-left asymmetry of tyrosine hydroxylase in rat median eminence: influence of arterial baroreflex nerves. Brain Res 523: 195–198. doi: 10.1016/0006-8993(90)91487-2
![]() |
[40] |
Hersh LB (1985) Characterization of membrane-bound aminopeptidases from rat brain:identification of the enkephalin-degrading aminopeptidase. J Neurochem 44: 1427–1435. doi: 10.1111/j.1471-4159.1985.tb08779.x
![]() |
[41] |
Wright JW, Harding JW (1997) Important roles for angiotensin III and IV in the brain renin-angiotensin system. Brain Res Rev 25: 96–124. doi: 10.1016/S0165-0173(97)00019-2
![]() |
1. | Ganiyu Oboh, Opeyemi Oluwafemi Ojueromi, Ayokunle Olubode Ademosun, Tosan Peter Omayone, Ademola Adetokunbo Oyagbemi, Temitayo Olabisi Ajibade, Adeolu Alex Adedapo, Effects of caffeine and caffeic acid on selected biochemical parameters in L‐NAME‐induced hypertensive rats, 2021, 45, 0145-8884, 10.1111/jfbc.13384 | |
2. | Ana Belén Segarra, Isabel Prieto, Inmaculada Banegas, Magdalena Martínez-Cañamero, Marc de Gasparo, Manuel Ramírez-Sánchez, Blood Pressure Correlates Asymmetrically with Neuropeptidase Activities of the Left and Right Frontal Cortices, 2021, 13, 2073-8994, 105, 10.3390/sym13010105 |