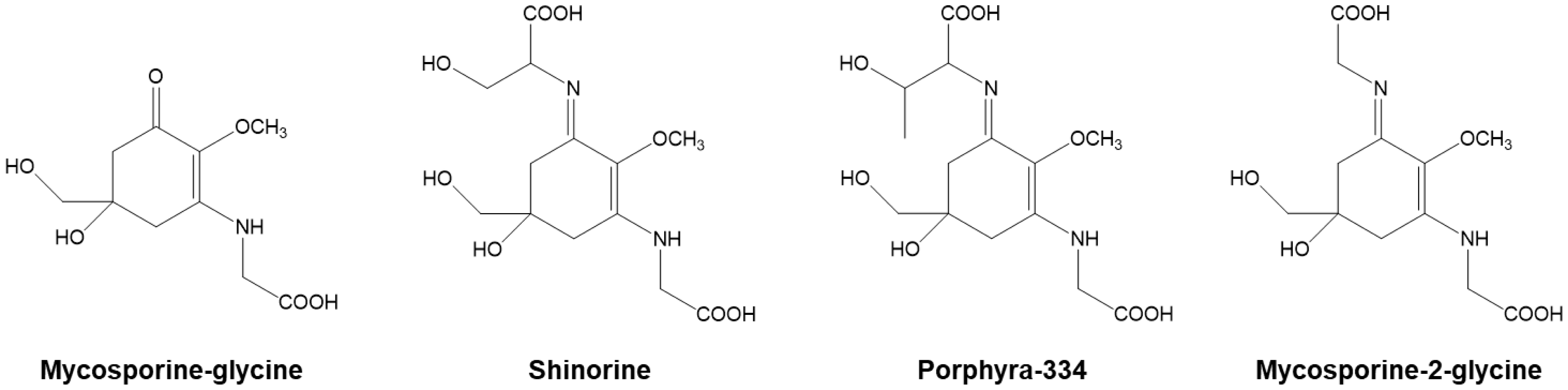
Cyanobacteria are distributed in diverse environments on Earth. They have evolved to synthesize a variety of secondary metabolites to combat and serve as barriers to protect them from distinct environments. Some of these metabolites and compounds exhibit useful physiological activities and are expected to be applied to a wide range of fields, including medicine, agriculture, food, and the cosmetics industry. Due to their photosynthetic ability, cyanobacteria are promising sources for sustainable production of these useful substances. It is expected that future research will lead to the development and efficient production of new compounds. These resources may provide great benefits to our lives and to the environment. This editorial provides an overview of the ultraviolet (UV)-absorbing substances produced by cyanobacteria and discusses future prospects.
Citation: Hakuto Kageyama, Rungaroon Waditee-Sirisattha. Bioactive ultraviolet-absorbing compounds derived from cyanobacteria and microalgae[J]. AIMS Molecular Science, 2025, 12(1): 26-31. doi: 10.3934/molsci.2025002
[1] | Hakuto Kageyama, Rungaroon Waditee-Sirisattha . Distribution, biosynthetic regulation, and bioactivities of mycosporine-2-glycine, a rare UV-protective mycosporine-like amino acid. AIMS Molecular Science, 2023, 10(4): 295-310. doi: 10.3934/molsci.2023017 |
[2] | Yoshie Uchida, Masaki Honda, Rungaroon Waditee-Sirisattha, Hakuto Kageyama . Functional evaluation of saclipins A and B derived from the edible cyanobacterium Aphanothece sacrum: New bioactivities for anti-wrinkle and anti-hypertension. AIMS Molecular Science, 2025, 12(2): 113-121. doi: 10.3934/molsci.2025007 |
[3] | Seyedeh Fahimeh Razavi, Leila Zarandi Miandoab, Elaheh Zadeh Hosseingholi, Nader Chaparzadeh . Radical scavenging capacity of RuBisCO bioactive peptides derived from Dunaliella salina and Spirulina platensis: An in silico and in vitro study. AIMS Molecular Science, 2025, 12(1): 49-66. doi: 10.3934/molsci.2025004 |
[4] | Yuri Pevzner, Daniel N. Santiago, Jacqueline L. von Salm, Rainer S. Metcalf, Kenyon G. Daniel, Laurent Calcul, H. Lee Woodcock, Bill J. Baker, Wayne C. Guida, Wesley H. Brooks . Virtual target screening to rapidly identify potential protein targets of natural products in drug discovery. AIMS Molecular Science, 2014, 1(2): 81-98. doi: 10.3934/molsci.2014.2.81 |
[5] | Hakuto Kageyama, Masaki Honda, Rikio Nakamura, Rungaroon Waditee-Sirisattha, Satoru Kamohara . Processing-induced changes in components that affect the radical scavenging activity of ethanolic extracts from Neopyropia yezoensis. AIMS Molecular Science, 2024, 11(3): 251-261. doi: 10.3934/molsci.2024015 |
[6] | Luiza H. P. Domingues, Matheus S. T. Arantes, Geovana S. Marques, Charles W. I. Haminiuk, Eliton Fontana, Vitor R. da Silva . Extraction of bioactive compounds from yerba mate (Ilex paraguariensis St.-Hil.) leaves by packed-bed extractor using hot water as solvents: Kinetics study and mathematical modeling. AIMS Molecular Science, 2024, 11(1): 42-60. doi: 10.3934/molsci.2024003 |
[7] | Ahmed A. Zaky, Muhammad Usman Akram, Katarzyna Rybak, Dorota Witrowa-Rajchert, Malgorzata Nowacka . Bioactive compounds from plants and by-products: Novel extraction methods, applications, and limitations. AIMS Molecular Science, 2024, 11(2): 150-188. doi: 10.3934/molsci.2024010 |
[8] | Harkirat S. Sethi, Jessica L. Osier, Geordan L. Burks, Jennifer F. Lamar, Hana McFeeters, Robert L. McFeeters . Expedited isolation of natural product peptidyl-tRNA hydrolase inhibitors from a Pth1 affinity column. AIMS Molecular Science, 2017, 4(2): 175-184. doi: 10.3934/molsci.2017.2.175 |
[9] | Abdulrahman Mahmoud Dogara, Ateeq Ahmed Al-Zahrani, Sarwan W. Bradosty, Saber W. Hamad, Aisha Abdullahi Mahmud, Hussain D. Almalki, Mustapha Abdullahi, Abubakar Abdullahi Lema, Hasan Nudin Nur Fatihah . In-vitro biological activity and in-silico studies of some volatile phytochemicals from the ethanol extract of Eugenia uniflora. AIMS Molecular Science, 2024, 11(3): 303-321. doi: 10.3934/molsci.2024018 |
[10] | Navid J. Ayon . Features, roles and chiral analyses of proteinogenic amino acids. AIMS Molecular Science, 2020, 7(3): 229-268. doi: 10.3934/molsci.2020011 |
Cyanobacteria are distributed in diverse environments on Earth. They have evolved to synthesize a variety of secondary metabolites to combat and serve as barriers to protect them from distinct environments. Some of these metabolites and compounds exhibit useful physiological activities and are expected to be applied to a wide range of fields, including medicine, agriculture, food, and the cosmetics industry. Due to their photosynthetic ability, cyanobacteria are promising sources for sustainable production of these useful substances. It is expected that future research will lead to the development and efficient production of new compounds. These resources may provide great benefits to our lives and to the environment. This editorial provides an overview of the ultraviolet (UV)-absorbing substances produced by cyanobacteria and discusses future prospects.
Cyanobacteria have developed mechanisms for coping with high UV radiation. One of the mechanisms is the production of natural UV-absorbing compounds (sunscreen compounds) to overcome the detrimental effects of UV radiation. These UV-absorbing compounds have attracted great interest as new biotechnological resources and ingredients with a high potential for applications in natural sunscreens and skin protection agents. Additionally, natural UV-absorbing compounds are generally considered to be less toxic than chemically synthesized sunscreen ingredients. Therefore, they are expected to be used as ingredients for skin care products. Moreover, natural UV-absorbing compounds have less impact on the environment and minimize the adverse effects on the marine ecosystem. Currently, the known UV-absorbing substances biosynthesized by cyanobacteria include mycosporine-like amino acids (MAAs), scytonemin, and saclipins.
MAAs are the most well-characterized UV-absorbing substances from cyanobacteria and are already distributed on the market as cosmetic ingredients [1]. MAAs are found in a variety of organisms including cyanobacteria, algae, fungi, and lichens. The regulation of MAA biosynthesis has been thoroughly investigated and the genes in this pathway were first reported in cyanobacteria in 2010 [2]. In specific strains of cyanobacteria, it has been reported that MAAs are induced by UVB radiation stress or salt stress. Therefore, they are thought to contribute to UV stress defense and osmoregulation [3]. Currently, more than 60 chemical structures of MAAs have been identified from various organisms, including cyanobacteria, and most are water soluble. The basic structures of MAAs include monosubstituted types in which one amino acid is bound to a cyclohexenone ring and disubstituted types in which two amino acids are bound to a cyclohexenimine ring (Figure 1). Although the absorption maximum varies depending on the structure of the substituent, many MAAs can efficiently absorb UVB (280–320 nm) and UVAII (320–340 nm). The molar extinction coefficients of MAAs are large, ranging from 20,900 to 58,800 M−1 cm−1. MAAs not only absorb UV rays but also exhibit various useful physiological activities [4]. It is well documented that many MAAs have antioxidant activity. In particular, mycosporine-glycine has been reported to have stronger antioxidant activity than ascorbic acid [5]. Substances with antioxidant properties can eliminate reactive oxygen species that promote skin aging, so products containing MAAs also exhibit anti-aging activity. In addition to antioxidant activity, MAAs exhibit useful effects, such as anti-inflammatory activity, anti-glycation activity, and collagenase inhibitory activity, making them suitable as ingredients in skin care cosmetics. A robust MAA production strategy is crucial for the wider use of MAAs in industrial applications. Currently, the chemical synthesis of MAAs is notoriously difficult and the development of production process technologies using microorganisms is promising. To date, the industrial production of MAAs using cyanobacteria remains a challenge. However, some progress has been reported in establishing large production systems using other microorganisms. For example, Jin et al. in [6] used the yeast Yarrowia lipolytica which harbored MAA biosynthetic genes derived from cyanobacteria to synthesize MAAs (shinorine and porphyra-334) and achieved a yield of 249.0 mg/L. Moreover, Park et al. created a shinorine-producing Saccharomyces cerevisiae yeast strain by introduction of the MAA biosynthetic genes from the Gram-positive bacteria Actinosynnema mirum and Pseudonocardia pini and obtained a yield of 267.9 mg/L of shinorine [7]. They also reported that the yield was increased to 1.7 g/L of shinorine using a corn steep liquor medium with a mixture of glucose and xylose [7]. Since it is known that the degree of MAA bioactivity varies depending on its chemical structure [8], the selection of the MAA to be produced is also important. For instance, Kim et al. developed an engineered S. cerevisiae yeast strain to produce various specific MAAs, including shinorine, porphyra-334, and mycosporine-2-glycine. This was achieved by the introduction of specific cyanobacterial genes for attaching the second amino acid residues to mycosporine-glycine for the bioproduction of these disubstituted MAAs [9]. Interestingly, it has been reported recently that compounds synthesized based on the chemical structure of MAAs exhibited UV absorption ability, as well as antioxidant and anti-tumor activity [10].
Scytonemin constitutes another important class of UV-absorbing compounds. Scytonemin is a hydrophobic pigment present in the extracellular polysaccharide sheaths (EPSs) of specific cyanobacterial strains [11]. In scytonemin-producer strains, the content reached 5% of the dry weight of the cyanobacterial cells [12]. Scytonemin is formed by two polycyclic alkaloid monomers consisting of an indolic ring and a phenolic ring and has a molecular weight of 544 Da (Figure 2) [13]. The biosynthetic pathway of scytonemin has been elucidated in previous reports [3],[14]. The color of scytonemin can change depending on its redox state, exhibiting a yellow-brown oxidized form or a red reduced form. Scytonemin has an absorption maximum in the UVA range of approximately 370 nm in vivo with additional absorption in the wavelength range of UVB and UVC [13]. Its large molecular extinction coefficient of 136,000 M−1 cm−1 at 384 nm indicates that scytonemin can protect cyanobacterial cells by effectively absorbing UVA [15]. Although scytonemin has not yet been applied in the industrial field, it has been reported to exhibit useful properties, such as antioxidant and anti-inflammatory effects [3]. Since scytonemin is a hydrophobic compound, it may be possible to incorporate it into products that are distinct from the products that contain water-soluble MAAs.
Recently, two novel cyanobacterial oxylipin compounds, referred to as saclipin A and saclipin B, were isolated from the cyanobacterium Aphanothece sacrum [16]. Oxylipins are a group of oxygenated natural products formed from fatty acids through pathways involving oxidation [17]. The chemical structures of saclipins A and B were elucidated, and it was found that they were all-E and 12Z in the triene structure, respectively, and had an isomeric relationship (Figure 3). The preferred International Union of Pure and Applied Chemistry (IUPAC) names for saclipins A and B are (10E,12E,14E)-9,16-dioxoocta-deca-10,12,14-trienoic acid and (10E,12Z,14E)-9,16-dioxoocta-deca-10,12,14-trienoic acid, respectively. The characteristic chemical properties of saclipins have been documented. The trans-form saclipin A can be isomerized to the cis-form saclipin B by photoirradiation. Due to a five conjugated double bond system in both structures, saclipins A and B exhibit a UV absorption maximum at 316 and 319 nm, respectively. The molar extinction coefficients of saclipins A and B were found to be 26,454 and 30,555 M−1 cm−1, respectively, at each absorption maxima. Thus, saclipins are novel UV-absorbing compounds in cyanobacteria. Interestingly, it was revealed that saclipins could be induced by desiccation stress in A. sacrum [16]. Currently, the biosynthetic pathway of saclipins is completely unknown. Importantly, saclipins possess several biological activities that may benefit skin care. Saclipins exhibited relatively weak free radical scavenging activity but potently inhibited the glycation of collagen and elastin [16]. In addition, it has recently been revealed that saclipins inhibit both elastase activity and tyrosinase activity. They also promote collagen and hyaluronic acid production, and inhibit melanogenesis [18]. Since saclipins exhibit biocompatibility with human skin cells, they are promising natural compounds for incorporation into skin care products.
Photosynthetic microorganisms, such as cyanobacteria and microalgae, are thought to play a very important role in the future of biotechnology. In addition to the UV-absorbing substances outlined in this paper, useful biologically active compounds derived from these organisms may lead to the development of compounding agents for pharmaceuticals, cosmetics, and functional foods. These compounds may even lead to breakthroughs that could greatly change human life, such as the development of carbon-neutral biofuels.
The authors declare they have not used Artificial Intelligence (AI) tools in the creation of this article.
[1] |
Kageyama H, Waditee-Sirisattha R (2018) Mycosporine-like amino acids as multifunctional secondary metabolites in cyanobacteria: From biochemical to application aspects. Studies in natural products chemistry. Amsterdam, Netherlands: Elsevier 153-194. https://doi.org/10.1016/B978-0-444-64179-3.00005-0 ![]() |
[2] |
Balskus EP, Walsh CT (2010) The genetic and molecular basis for sunscreen biosynthesis in cyanobacteria. Science 329: 1653-1656. https://doi.org/10.1126/science.1193637 ![]() |
[3] |
Kageyama H, Waditee-Sirisattha R (2022) Mycosporine-like amino acids and scytonemin: Regulations, roles for adaptation of cyanobacteria to the environment, and potential applications. Cyanobacterial physiology: From fundamentals to biotechnology. SanDiego, CA, USA: Academic Press (An imprint of Elsevier) 101-111. https://doi.org/10.1016/B978-0-323-96106-6.00016-2 ![]() |
[4] |
Kageyama H, Waditee-Sirisattha R (2019) Antioxidative, anti-inflammatory, and anti-aging properties of mycosporine-like amino acids: molecular and cellular mechanisms in the protection of skin-aging. Mar Drugs 17: 222. https://doi.org/10.3390/md17040222 ![]() |
[5] |
de la Coba F, Aguilera J, Figueroa FL, et al. (2009) Antioxidant activity of mycosporine-like amino acids isolated from three red macroalgae and one marine lichen. J Appl Phycol 21: 161-169. https://doi.org/10.1007/s10811-008-9345-1 ![]() |
[6] | Jin H, Kim S, Lee D, et al. (2023) Efficient production of mycosporine-like amino acids, natural sunscreens, in Yarrowia lipolytica. Biotechnol Biof Biop 16: 162. https://doi.org/10.1186/s13068-023-02415-y |
[7] | Park BR, Jeong CR, Cha M, et al. (2024) Sustainable production of shinorine from agricultural wastes using engineered Saccharomyces cerevisiae expressing novel d-alanine-d-alanine ligase from Pseudonocardia pini. J Agric Food Chem 72: 22710-22721. https://doi.org/10.1021/acs.jafc.4c05664 |
[8] |
Kageyama H, Waditee-Sirisattha R (2023) Distribution, biosynthetic regulation, and bioactivities of mycosporine-2-glycine, a rare UV-protective mycosporine-like amino acid. AIMS Mol Sci 10: 295-310. https://doi.org/10.3934/molsci.2023017 ![]() |
[9] |
Kim S, Park BG, Jin H, et al. (2023) Efficient production of natural sunscreens shinorine, porphyra-334, and mycosporine-2-glycine in Saccharomyces cerevisiae. Metab Eng 78: 137-147. https://doi.org/10.1016/j.ymben.2023.05.009 ![]() |
[10] |
Figueroa FL, Castro-Varela P, Vega J, et al. (2024) Novel synthetic UV screen compounds inspired in mycosporine-like amino acids (MAAs): Antioxidant capacity, photoprotective properties and toxicity. J Photoch Photobiol B 261: 113050. https://doi.org/10.1016/j.jphotobiol.2024.113050 ![]() |
[11] |
Garcia-Pichel F, Castenholz RW (1991) Characterization and biological implications of scytonemin, a cyanobacterial sheath pigment. J Phycol 27: 395-409. https://doi.org/10.1111/j.0022-3646.1991.00395.x ![]() |
[12] |
Sinha RP, Klisch M, Gröniger A, et al. (2001) Responses of aquatic algae and cyanobacteria to solar UV-B. Plant Ecology 154: 219-236. https://doi.org/10.1023/A:1012986500543 ![]() |
[13] |
Proteau PJ, Gerwick WH, Garcia-Pichel F, et al. (1993) The structure of scytonemin, an ultraviolet sunscreen pigment from the sheaths of cyanobacteria. Experientia 49: 825-829. https://doi.org/10.1007/BF01923559 ![]() |
[14] | Kageyama H, Waditee-Sirisattha R (2018) Cyanobacterial UV sunscreen: Biosynthesis, regulation, and application. Sunscreens: source, formulations, efficacy and recommendations.NOVA Science Publishers 1-28. |
[15] |
Wada N, Sakamoto T, Matsugo S (2013) Multiple roles of photosynthetic and sunscreen pigments in cyanobacteria focusing on the oxidative stress. Metabolites 3: 463-483. https://doi.org/10.3390/metabo3020463 ![]() |
[16] |
Uchida Y, Maoka T, Palaga T, et al. (2023) Identification of desiccation stress-inducible antioxidative and antiglycative ultraviolet-absorbing oxylipins, saclipin A and saclipin B, in an edible cyanobacterium Aphanothece sacrum. J Agric Food Chem 71: 16137-16147. https://doi.org/10.1021/acs.jafc.3c05152 ![]() |
[17] |
Gerwick WH, Moghaddam M, Hamberg M (1991) Oxylipin metabolism in the red alga Gracilariopsis lemaneiformis: Mechanism of formation of vicinal dihydroxy fatty acids. Arch Biochem Biophys 290: 436-444. https://doi.org/10.1016/0003-9861(91)90563-x ![]() |
[18] |
Uchida Y, Honda M, Waditee-Sirisattha R, et al. (2024) Photo- and thermo-chemical properties and biological activities of saclipins, UV-absorbing compounds derived from the cyanobacterium Aphanothece sacrum. ACS Agric Sci Technol 4: 1260-1270. https://doi.org/10.1021/acsagscitech.4c00571 ![]() |