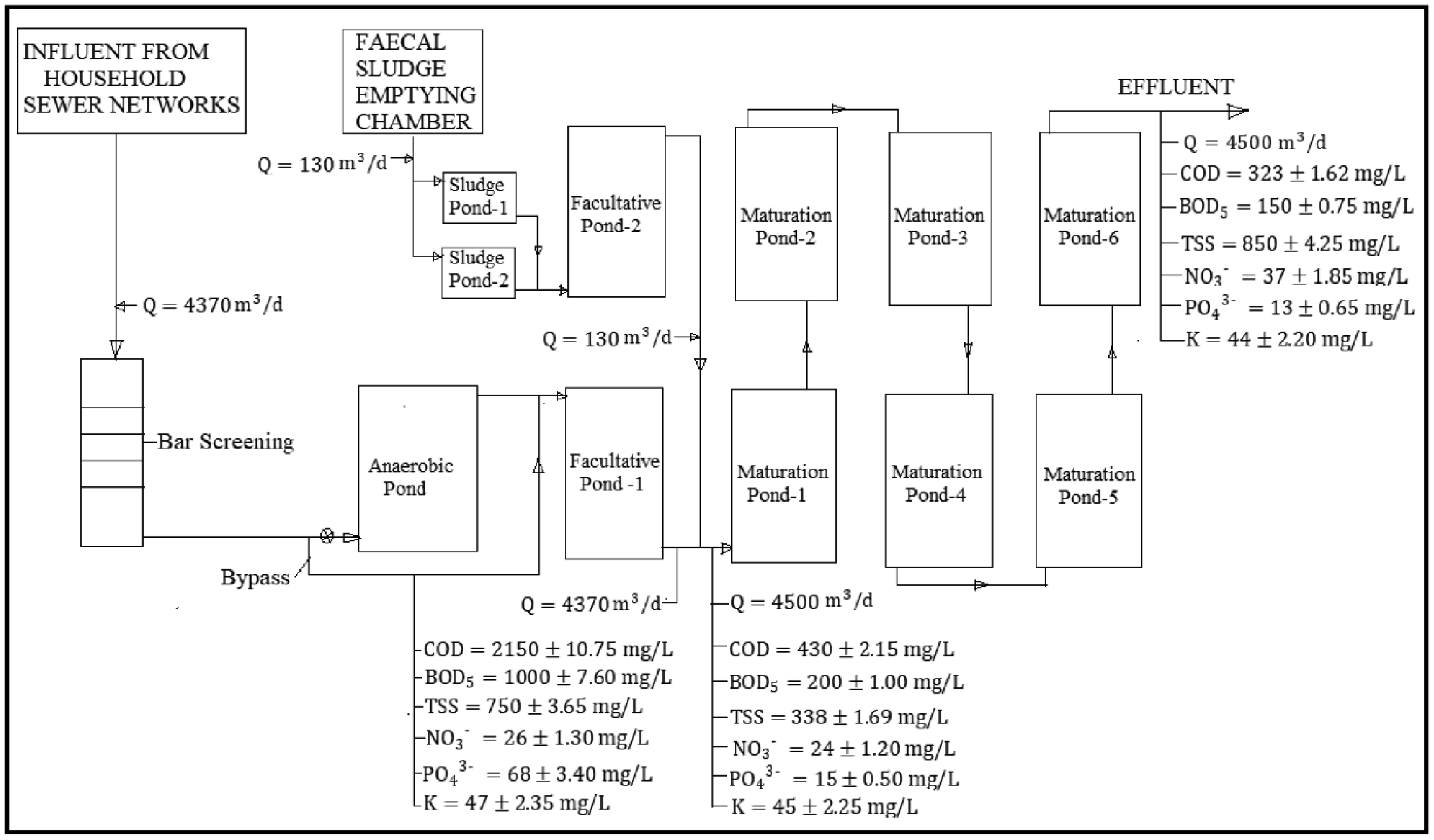
The extremophile microorganism Thermus scotoductus primarily exhibits aerobic metabolism, though some strains are capable of anaerobic growth, utilizing diverse electron acceptors. We focused on the T. scotoductus K1 strain, exploring its aerobic growth and metabolism, responses to various carbon sources, and characterization of its bioenergetic and physiological properties. The strain grew on different carbon sources, depending on their concentration and the medium's pH, demonstrating adaptability to acidic environments (pH 6.0). It was shown that 4 g L−1 glucose inhibited the specific growth rate by approximately 4.8-fold and 5.6-fold compared to 1 g L−1 glucose at pH 8.5 and pH 6.0, respectively. However, this inhibition was not observed in the presence of fructose, galactose, lactose, and starch. Extracellular and intracellular pH variations were mainly alkalifying during growth. At pH 6.0, the membrane potential (ΔΨ) was lower for all carbon sources compared to pH 8.5. The proton motive force (Δp) was lower only during growth on lactose due to the difference in the transmembrane proton gradient (ΔpH). Moreover, at pH 6.0 during growth on lactose, a positive Δp was detected, indicating the cells' ability to employ a unique energy-conserving strategy. Taken together, these findings concluded that Thermus scotoductus K1 exhibits different growth and bioenergetic properties depending on the carbon source, which can be useful for biotechnological applications. These findings offer valuable insights into how bacterial cells function under high-temperature conditions, which is essential for applying bioenergetics knowledge in future biotechnological advancements.
Citation: Hripsime Petrosyan, Karen Trchounian. Growth characteristics, redox potential changes and proton motive force generation in Thermus scotoductus K1 during growth on various carbon sources[J]. AIMS Microbiology, 2024, 10(4): 1052-1067. doi: 10.3934/microbiol.2024045
[1] | Ronald C. Sims, Sean K. Bedingfield, Reese Thompson, Judith L. Sims . Bioenergy from wastewater-based biomass. AIMS Bioengineering, 2016, 3(1): 103-124. doi: 10.3934/bioeng.2016.1.103 |
[2] | Phuong Tran, Linh Nguyen, Huong Nguyen, Bong Nguyen, Linh Nong , Linh Mai, Huyen Tran, Thuy Nguyen, Hai Pham . Effects of inoculation sources on the enrichment and performance of anode bacterial consortia in sensor typed microbial fuel cells. AIMS Bioengineering, 2016, 3(1): 60-74. doi: 10.3934/bioeng.2016.1.60 |
[3] | Joe A. Lemire, Marc A. Demeter, Iain George, Howard Ceri, Raymond J. Turner . A novel approach for harnessing biofilm communities in moving bed biofilm reactors for industrial wastewater treatment. AIMS Bioengineering, 2015, 2(4): 387-403. doi: 10.3934/bioeng.2015.4.387 |
[4] | Yingyu Law, Leo Wein . Reversing the nutrient drain through urban insect farming—opportunities and challenges. AIMS Bioengineering, 2018, 5(4): 226-237. doi: 10.3934/bioeng.2018.4.226 |
[5] | Sara R Robertson, Robert JC McLean . Beneficial biofilms. AIMS Bioengineering, 2015, 2(4): 437-448. doi: 10.3934/bioeng.2015.4.437 |
[6] | Xiu Zhang, Nhuan P. Nghiem . Pretreatment and Fractionation of Wheat Straw for Production of Fuel Ethanol and Value-added Co-products in a Biorefinery. AIMS Bioengineering, 2014, 1(1): 40-52. doi: 10.3934/bioeng.2014.1.40 |
[7] | Daniel Calle, Duygu Yilmaz, Sebastian Cerdan, Armagan Kocer . Drug delivery from engineered organisms and nanocarriers as monitored by multimodal imaging technologies. AIMS Bioengineering, 2017, 4(2): 198-222. doi: 10.3934/bioeng.2017.2.198 |
[8] | Dinesh Adhikari, Ima Yudha Perwira, Kiwako S. Araki, Motoki Kubo . Stimulation of soil microorganisms in pesticide-contaminated soil using organic materials. AIMS Bioengineering, 2016, 3(3): 379-388. doi: 10.3934/bioeng.2016.3.379 |
[9] | Mykhailo A. Anishchenko, Ellina G. Pozdniakova-Kyrbiatieva, Yurii Volodymyrovych Mosaiev, Oleksandr V. Krasnokutskyi, Volodymyr V. Glazunov . Use of “natural therapy” technology in the framework of social and psychological rehabilitation of the elderly. AIMS Bioengineering, 2022, 9(3): 309-318. doi: 10.3934/bioeng.2022022 |
[10] | Ana Meireles, Ana L. Gonçalves, Inês B. Gomes, Lúcia Chaves Simões, Manuel Simões . Methods to study microbial adhesion on abiotic surfaces. AIMS Bioengineering, 2015, 2(4): 297-309. doi: 10.3934/bioeng.2015.4.297 |
The extremophile microorganism Thermus scotoductus primarily exhibits aerobic metabolism, though some strains are capable of anaerobic growth, utilizing diverse electron acceptors. We focused on the T. scotoductus K1 strain, exploring its aerobic growth and metabolism, responses to various carbon sources, and characterization of its bioenergetic and physiological properties. The strain grew on different carbon sources, depending on their concentration and the medium's pH, demonstrating adaptability to acidic environments (pH 6.0). It was shown that 4 g L−1 glucose inhibited the specific growth rate by approximately 4.8-fold and 5.6-fold compared to 1 g L−1 glucose at pH 8.5 and pH 6.0, respectively. However, this inhibition was not observed in the presence of fructose, galactose, lactose, and starch. Extracellular and intracellular pH variations were mainly alkalifying during growth. At pH 6.0, the membrane potential (ΔΨ) was lower for all carbon sources compared to pH 8.5. The proton motive force (Δp) was lower only during growth on lactose due to the difference in the transmembrane proton gradient (ΔpH). Moreover, at pH 6.0 during growth on lactose, a positive Δp was detected, indicating the cells' ability to employ a unique energy-conserving strategy. Taken together, these findings concluded that Thermus scotoductus K1 exhibits different growth and bioenergetic properties depending on the carbon source, which can be useful for biotechnological applications. These findings offer valuable insights into how bacterial cells function under high-temperature conditions, which is essential for applying bioenergetics knowledge in future biotechnological advancements.
The evolution of wastewater treatment technologies over the decades has been observed with the goal of safeguarding human and environmental health [1]–[3]. The technologies that have emerged can be categorized as conventional and modern wastewater treatment technologies, which are used for both industrial and municipal effluents [4]. Despite differences in design approach, each conventional and modern wastewater treatment technology has its own effectiveness in removing pollutants [5]. This is because each technology has its own design approach that distinctly enables it to be more robust in pollutant removal than other technologies [6],[7]. The advancement of wastewater treatment technologies has been driven by the dual purposes of protecting and promoting human health and safeguarding the environment from the harmful effects of untreated effluents [8]–[10]. Regulatory requirements have also encouraged innovations to ensure the protection of human health and the environment [11]. However, to deploy most appropriate technology for wastewater treatment to protect human and environmental health, the consideration of factors such as land requirements, capital costs, operation and maintenance costs, and technology sustainability was found to be very crucial [12],[13].
The first engineered wastewater treatment system used for many decades was a septic tank combined with a soak-away pit [14]. It has been estimated that 26% of households in Europe, 25% in the US, and 20% in Australia rely on septic tank systems for onsite sanitation [15],[16]. The septic tank system can settle suspended solids and stabilize wastewater to about 50% of the organic load of sewage, but it is less effective in removing pathogens [17]. Properly planned, designed, installed, operated, and maintained, septic tank systems can achieve a removal efficiency of pollutants from 70% to >90% [16]. However, for nitrogen removal, it can achieve efficiency between 20% to 80%, and for phosphate removal, it can achieve a small efficiency between 10% to 30%, which can pose pollution risks to groundwater [18]. To improve the performance of the septic tank, it was determined that a soak-away pit needed to be incorporated subsequently to further remove pollutants through physical, chemical, and biological processes as a result of percolation in soil strata [19]. Nevertheless, modifications to the septic tank have resulted in improved treatment performance. For instance, the removal of total coliforms has been reported to reach 99.99%, total suspended solids removal at 99.57%, ammonia nitrogen at 46.83%, nitrate nitrogen at 31.08%, total Kjeldahl nitrogen at 48.39%, BOD5 at 94.4%, and phosphates at 71.74% [20]–[22].
Due to the risks of contaminating groundwater, strict environmental legislation has been imposed in most developed countries to prohibit using percolation systems for partially treated sewage disposal into soils [18],[23]. However, septic tanks are used at the household level but are inadequate community settings [24],[25]. To address communal wastewater treatment, technologies have been developed and categorized as conventional and advanced technologies [6],[7]. Conventional wastewater treatment technologies include aerobic, anaerobic, and biofilm treatment technologies [26]. Aerobic treatment technologies include activated sludge process, aerobic lagoon, aerobic oxidation pond and trickling filters [27]. Anaerobic treatment technologies include anaerobic lagoons, anaerobic oxidation ponds, waste stabilization ponds, upflow anaerobic sludge blankets, anaerobic baffled reactors, and expanded granular sludge blankets [28],[29]. Biofilm technologies include membrane bioreactors, rotating biological contactors, moving bed biological reactors, fixed film bioreactors, and fluidized bed bioreactors [30]–[32]. Advanced technologies comprise sequencing batch reactors, aerobic granular sludge, advanced oxidation processes, and advanced reduction/oxidation processes [33],[34].
The selection of conventional and advanced technologies must consider factors such as implementation costs (including capital costs, operation and maintenance costs, and land requirements) [35],[36], regulatory requirements (to comply with allowable discharge limits to protect human health and the environment) [37], technology level (advanced technology), and energy consumption (related to electrical power for mechanical equipment) [38]. Therefore, the selection of wastewater treatment technology involves complex and multidisciplinary considerations, including environmental, social, technical, and economic aspects [35],[39],[40].
In developing countries, especially in Africa, waste stabilization ponds (WSPs) are commonly used for municipal wastewater treatment WSPs [41]. Activated sludge (ASP), trickling filters (TF), and other biofilms are rarely used due to insufficient energy and operating costs [42]. WSPs are preferred because they can handle high organic loads, uncontrolled input, power outages, and increasing wastewater flow rates [43]. However, WSPs face challenges such as poor operation and maintenance due to lack of funds, requiring large areas, and lack of reinvestment [44]. This can lead to poor effluent quality, which can negatively impact human health and the acceptance of treated water discharge into the environment and for reuse [45],[46].
In order to address the challenges faced by WSPs, an advanced facultative pond (AFP) system was deployed. This system consists of a series of interconnected ponding systems with mechanical aeration for wastewater treatment [47]. The AFP was capable of producing high-quality effluent by removing 60–80% of the influent BOD5, but it resulted in high levels of NO3− and PO43− due to aeration systems, sludge accumulation and loss of biogas [48]. Due to energy consumption, the modification to pond in pond (PIP) system which has interior pond of depth of 3–4 m submerged within the outer pond without mechanical aeration system [47],[49]. The PIP system offers the advantages of increased solid retention time and biogas capture by covering the inner pond, in contrast to the AFP [48]. However, challenges arose in ensuring complete coverage of the inner pond and desludging of the slurry due to the connectivity of the outer pond [48]. Activated sludge pond (ASP) is used to treat both municipal and industrial wastewater by supplying oxygen to bacteria, fungi, and protozoa to aerobically degrade organic matter to meet effluent discharge standards [50]–[52]. Microbial consortia degrade colloidal and dissolved carbonaceous compounds for energy generation and ammonium and phosphorus for new cellular tissue production [53]. Although ASP requires less space and semi-skilled personnel, it can be expensive due to energy requirements for the aeration system and pumps for sludge recycling to maintain high bacterial concentrations for organic matter degradation [54].
In Tanzania, the technologies commonly used for wastewater treatment include waste stabilization ponds and the activated sludge process, as well as the upflow anaerobic sludge blanket (UASB), which is used for domestic and food industry effluent treatment [55],[56]. The UASB is an anaerobic reactor that reduces high biomass concentration in the absence of oxygen through auto-flocculation, generating granular biomass that forms a blanket [57],[58]. The process in UASB degrades organic matter to produce methane-rich biogas, offering operational flexibility and not requiring complex or expensive mechanical equipment or skilled labor to operate [59],[60]. The UASB is seen as a potential sustainable technology for decentralized wastewater treatment, particularly for urban sewage and food industry effluents [61]. However, when the UASB is used solely for anaerobic wastewater treatment, it discharges partially treated effluent in terms of organic matter and nutrients, which may be harmful to human health and the environment [62],[63]. This necessitates UASB to require subsequent treatment systems to reduce organic matter and nutrients to acceptable discharge limits [62],[64].
Constructed wetlands in Tanzania found to be effective in polishing effluents from UASB and WSPs by reducing organic matter, pathogens, and heavy metals and regulating nutrients [65],[66]. Therefore, our purpose was to evaluate the functional and investment benefits of WSPs and advanced facultative pond integrated constructed wetlands (AFP-CW) with the aim of extracting resources for agricultural use. The selection process was based on mathematical modeling that incorporated economic, environmental, and social indicators. A reconnaissance survey was conducted in Kilimanjaro to evaluate implemented WSPs, which were found to be among the best functioning systems based on their maintenance and operational status in Tanzania. Samples were collected, and the efficiency of the WSPs was assessed in the laboratory by analyzing various environmental parameters. The mathematical model for sizing the AFP-CW and WSPs for treating organic-rich wastewater was also examined. The ultimate aim was to identify the most suitable organic-rich wastewater treatment technology for resource recovery based on technical, economic, environmental, and social aspects.
The study was conducted at Moshi urban water supply and sanitation authority (MUWSA), located in Moshi municipality at 3.35° south latitude, 37.33° east longitude and at elevation of 888 m above the sea level.
The WSPs implemented were anaerobic pond, facultative ponds, and maturation ponds as shown in Figure 1. The WSPs were designed to treat 4500 m3/d flow rate (Q) of Municipal wastewater in Moshi Municipality. The WSPs receive municipal wastewater from established household sewer networks at a flow rate of 4370 m3/d, while 130 m3/d of fecal sludge is discharged by sludge empty trucks from non-networked household sewers.
The layout of WSPs (Figure 1) include one anaerobic pond, two fecal sludge ponds, two facultative ponds in parallel, and six maturation ponds connected in a series. The anaerobic pond is 55 m wide, 115 m long, and 4.50 m deep. Each of the two facultative ponds in parallel is 67 m wide, 147 m long, and 2.50 m deep. Each of the six maturation ponds connected in series is 70 m wide, 138 m long, and 1.50 m deep. The two fecal sludge ponds constructed in parallel are 20 m wide, 20 m long, and 2.00 m deep. The influent to the facultative ponds contains chemical oxygen demand (COD) concentrations of 2150 ± 10.75 mg/L, nitrate (NO3−) of 26 ± 1.30 mg/L, phosphate (PO43−) of 68 ± 3.40 mg/L, and potassium (K) of 47 ± 3.25 mg/L. The effluent from the facultative ponds contains COD concentrations of 430 ± 2.15 mg/L, biological oxygen demand (BOD5) of 200 ± 1.00 mg/L, total suspended solids (TSS) of 338 ± 1.69 mg/L, NO3− of 24 ± 1.20 mg/L, PO43− of 15 ± 0.50 mg/L, and K of 45 ± 2.25 mg/L. The effluent from maturation pond-6 to the pad farm for irrigation contains COD concentrations of 323 ± 1.62 mg/L, BOD5 of 150 ± 0.75 mg/L, TSS of 850 ± 4.25 mg/L, NO3− of 37 ± 1.85 mg/L, PO43− of 13 ± 0.65 mg/L, and K of 44 ± 2.20 mg/L.
The study utilized both secondary and experimental data. The secondary data consisted water quality monitoring records of WSPs conducted by MUWSA in 2014 and 2016. The experimental data included laboratory results of samples analyzed at Nelson Mandela Institution of Science and Technology (NM-AIST) laboratory in 2017 over a period of three-months spent to assess the performance of WSPs. Both secondary and experimental data were used to evaluate the performance trend of WSPs since its implementation in 2008. Unfortunately, some of the years of WSPs performance monitoring were not recorded.
The major parameters monitored were BOD5, COD, TSS, NO3−, PO43−, K, dissolve oxygen (DO), electrical conductivity (EC), TDS, temperature (T), pH, fecal coliforms (FC), and escherichia coli (E-coli). The COD was analyzed calorimetrically using Multi-parameter Bench Photometer (Model HI 83099 HANNA), the BOD5 was analyzed at 20 °C using Oxitop IS 12 BOD5 incubator and TSS was analyzed gravimetrically at temperature ranging from 103 to 105 °C. The NO3−, was determined using Spectrophotometer (Model HACH–DR 2800) with the cadmium reduction method at 355 nm, and PO43− by Ascorbic acid method at 510 nm. The physical parameters such as, pH, EC, TDS, and T °C were measured using multi-parameters (Model HI 9024 HANNA) while the FC and E-coli were counted using Agar membrane filtration method.
Anaerobic ponds (AnPs) are primary treatment ponds used in WSPs, constructed either in series (single pond) or in parallel (multiple ponds), with a high capacity for degrading high organic loads (BOD5) in the absence of oxygen [58]. The design of AnP depends on the ambient temperature and hydraulic retention time [67],[68] for it determines the volumetric loading that used to provide a volume of anaerobic pond as expressed in Eqs 1 and 2. To ensure that the AnP operates in an oxygen-free environment, its depth is typically maintained between 2.50 and 5.00 m, which is used to calculate the area of the AnP and establish its dimensions (length and width), as expressed in Eqs 3 to 6. For industrial and agricultural processes with strong organic loads, an HRT of 20 to 50 days is recommended [69], however, for municipal wastewater, the HRT is determined by Eq 7.
where Va = volume of anaerobic pond (m3), λv = volumetric loading (g/m3·d), Li = influent BOD5 (mg/L), Q = flow rate (m3/d), T = ambient temperature (°C).
where Aa = area of anaerobic pond (m2), h = effective depth of anaerobic pond (m), L = length of anaerobic pond (m), and W = width of anaerobic pond (m), α = angle of inclination (30–60°).
where τa = hydraulic retention time in anaerobic pond (d).
The facultative ponds (FaP) are secondary parallel constructed ponds associated with WSPs that operate under dual conditions, which are stratified at a recommended depth of 1.2 to 2.5 m [69]. Aerobic conditions prevail at the top due to photosynthesis and atmospheric re-aeration, while anaerobic conditions dominate at the bottom of the FaP [45],[70]. The oxygen at the surface of the FaP is utilized by aerobic bacteria to stabilize the organic load, whereas anaerobic bacteria at the bottom digest the organic matter that has accumulated in the sludge zone. The design of surface area of FaP is based on empirical methods, as expressed in Eqs 8 and 9, as well as rational formulas outlined in Eqs 10 to 13. The volume of facultative ponds is determined by their depth, and the hydraulic retention times are calculated using Eqs 14 and 15 respectively.
where Af = area of facultative ponds (m2), Le = effluent BOD5 (mg/L), kT = reaction rate coefficient at ambient temperature (d−1), k20 = the reaction rate coefficient at 20 °C temperature (d−1), θ = temperature correction factor , T = ambient temperature (°C), Vf= volume of facultative ponds (m2), h = effective depth of pond (m), L = length of facultative pond (m), and W = width of facultative pond (m), and n = number of facultative ponds. Other parameters were defined previously.
where τf = hydraulic retention time in facultative ponds (d), e = net evaporation rate (mm/d).
The maturation ponds (MaPs) in WSPs are polishing ponds with shallow depths ranging from 0.9 to 1.2 meters and are characterized by the presence of oxygen [69]. MaPs are considered low-cost polishing ponds that operate under aerobic conditions and are connected in series, as described by Eq 16. The design for nitrate removal in the maturation pond is outlined in Eqs 17 to 20, while the removal of fecal bacteria is addressed in Eqs 21 and 22. The design for helminth egg removal is specified in Eq 23, BOD5 removal in Eq 24, and the pond's volume is determined by Eq 25.
where Am = area of maturation ponds (m2), τm = hydraulic retention time for maturation pond (d), e = net evaporation rate (mm/d).
The formula for nitrate removal in facultative and maturation ponds arranged in series at temperatures above 20 °C is provided by Eqs 18 and 19, respectively, while pH is determined by Eq 20.
but,
where Ni = influent nitrate (mg/L), Ne, AnP = effluent nitrate from anaerobic pond (mg/L), Ne = effluent from maturation ponds (mg/L), pH = measure of the concentration of H+, A = influent alkalinity (mg CaCO3/L).
The reduction of fecal bacteria in WSPs was found to depend greatly on temperature, as indicated by Eqs 21 and 22.
but,
where fci = influent number of fecal coliforms (Counts/100 mL), fce = effluent influent number of fecal coliforms (Counts/100 mL), Kb = temperature dependent first order rate constant (d−1).
Helminth eggs were reported to be removed through the sedimentation process in anaerobic and primary facultative ponds of wastewater stabilization ponds (WSPs [67],[68]. For restricted effluent water usage, it is recommended that the water contains zero helminth eggs [45],[70]. The percentage removal (R) of helminth eggs can be determined by Eq 23.
where Vm = volume of maturation ponds (m3).
The AFP has been modified to enhance the advanced facultative pond for improved organic degradation, biogas capture, nitrification, and effluent polishing. The inner pit of the AFP was replaced by an upflow anaerobic sludge blanket, while the outer pond was replaced by an aeration tank. Additionally, a constructed wetland was integrated for effluent polishing, which involves the removal of nutrients, organics, pathogens, heavy metals, and other emerging chemicals [72],[73]. The enhanced advanced facultative pond is abbreviated as AFP-CW, and the design of its components is represented by Eqs 26–34.
where Vn = nominal/effective liquid volume of reactor (m3), Q = influent flow rate (m3/d), So = influent COD (mg/L), Lorg = organic loading rate (kg/m3·d) and τU = hydraulic retention time in UASB (days).
where VL = total liquid volume of the reactor (m3), E = efficiency of bioreactor (%).
but,
hence,
Where y = biomass yield M of cell formed per M of substrate consumed = 0.08 gVSS/g COD, kd = endogenous decay coefficient = 0.03 gVSS/VSS.d, fd = fraction of cell mass remaining as cell debris = 0.15 g VSS cell debris/g VSS biomass decay, nbVSS = non-biodegradable volatile suspended solids, mg/L, So = Initial substrate concentration (COD) at time t = 0, mg/L, S = substrate concentration (COD) at time t, mg/L, SRT = sludge retention time, d, µm = maximum growth rate = 0.25 gVSS/g VSS.d, Xe = the particulate COD (mg/L), and ϵ = efficiency of bioreactor to degrade COD.
The methane gas (CH4) is considered to be produced when 0.67 g COD is removed when sulfate reducing bacteria consume 1g SO4 (0.67 g CODdegraded/1g SO4, reduced) as expressed in Eq 33. Moreover, CH4 production rate depends on temperature normally 35 °C at a ratio of 0.4 L CH4/g COD [75]–[77] as per Eq 34.
where CODSR = COD removed when sulfate is reduced (mg/L), SO4, in = influent sulfate (mg/L), CODdegr. = degraded COD (mg/L), and SO4, red. = reduced sulfate (mg/L).
Aeration is referred to as a mass transfer process, in which oxygen molecules are transferred from the gaseous phase to the liquid phase [78]. The cascade aeration tank (CAT) allows water to flow over a series of steps, creating turbulence to facilitate oxygen diffusion. The wastewater flows through the system for an extended residence time of 1 to 2 hours, during which vigorous mixing leads to significant air bubble entrainment [79]. This process reduces odors, ion concentrations, and chemical oxygen demand (COD) [80],[81]. The CAT was implemented to avoid the use of mechanical aeration systems, which require electrical energy [82]. The design of the CAT is detailed in Eqs 35–47.
where VL, CAT = total liquid volume of cascade aeration tank (m3), Q = influent flow rate (m3/d), and τCAT = hydraulic retention time in cascade aeration tank (hr).
where AS, CAT = surface area of cascade aeration tank (m2), h = depth of cascade aeration tank (m).
but,
where LCAT = length of cascade aeration tank (m), WCAT = width of cascade aeration tank (m).
where AAS = area of cascade aeration step (m2), g = acceleration due to gravity (m/s2) and hc = critical depth of liquid flow (m).
where LAS = length of aerator step (m).
where VAP = approach velocity (m/s).
where NS = number of aerator steps.
where Hf = height of fall of aeration step (m), hSf = height of fall of cascade aeration step (m), Sl = slope (%).
where VAS = capacity/volume of cascade aerator step (m3).
but,
where Nce = number of cells of cascade aeration tank and Lco = typical length of cascade aeration step (0.5–1.0 m) [81].
The settling tank (ST) was used to settle the biomass produced in the CAT. Additionally, it was anticipated that further nitrogen transformation processes would occur in the ST, which is open to the atmosphere. This design allows for air stratification, facilitating the transition between aerobic and anoxic reactions carried out by indigenous microbial cultures [84]. In the ST, biomass is produced and settles at the bottom as slurry, where nitrate is reduced to molecular nitrogen under anaerobic conditions [85]. The design of the ST was developed considering various parameters, as expressed in Eqs 48–71.
where Px, TSS = sludge production, rate (kg/d), SRT = sludge retention time (d), Q = flow rate (m3/hr), kinetic coefficients: y = biomass yield M of cell formed per M of substrate consumed, 0.4 gVSS/gb COD, yn = biomass yield of cell formed per substrate consumed, for nitrification 0.12 gVSS/gNOx, kd, 12 °C = endogenous decay coefficient, 0.12 g/g.d, kdn, 12 °C = endogenous decay coefficient for nitrifying organisms, 0.08 g/g.d, fd = fraction of cell mass remaining as cell debris, 0.15 g/g, So = initial substrate concentration at time t = 0, mg/L, S = substrate concentration at time t, mg/L, nbVSS = non-biodegradable volatile suspended solids, mg/L, iTSS = inert total suspended solids, mg/L, and Nox = Nitrogen oxidized, mg/L.
but,
where pCOD = the particulate COD, mg/L, TKN = Total Kjeldahl Nitrogen, mg/L.
but,
where VAE = volume of aeration tank (m3), XMLSS = Mixed liquor suspended solid (mg/L), and τS = hydraulic retention time in settling tank (hr).
Consider that efficiency (ϵ) 50% of influent VSS is degraded [75]–[77].
where iTSS = Inert total suspended solids, mg/L, TSSe = effluent total suspended solids, mg/L, VSSe = effluent Volatile Suspended Solids, mg/L, ηR = total suspended solids removal, efficiency (%) and a, b are empirical constants, (a = 0.0075 and b = 0.014) [75]–[77].
where VSL = volume of sludge produced in settling tank (m3).
where VST = total volume of settling tank (m3).
where XMLVSS = mixed liquor volatile suspended solids (mg/L).
where Ne = Effluent Nitrogen (mg/L), NoX = Ammonium (mg/L), and PX,bio = biodegradable organic nitrogen (kg/d).
but,
where bCOD = biodegradable chemical oxygen demand (mg/L), BOD5 = 5 days biochemical oxygen demand (mg/L), and fC = Conversion factor for municipal wastewater.
but,
where AST = area settling tank (m2), h = effective depth of settling tank (m), L = length of settling tank (m), and W = width of settling tank (m).
The design of horizontal subsurface horizontal flow constructed wetland (HSSF-CW) was based on the plug flow reactor (PFR) modeling which considers: plug flow, steady state, constant density (valid for most liquids), and single reaction [86]–[89]. The surface area design of the CW, and its removal mechanisms of organic matter, nitrates, and phosphates was represented by Eqs 72–83.
but,
where AS = Surface area of constructed wetland, (m2), Q = flow rate (m3/d), kT = Reaction rate constant (d−1), y = Effective depth of the liquid, (m), ϵ = Porosity of the media, (%), Co = Inflow BOD5, (mg/L), Ce = Effluent BOD5, (mg/L), and φ = Temperature correction coefficient (1.1) [75]–[77].
where OLR = Organic loading rate, (kg/ha.d) (limited to 180 kg/ha.d)
where HLR = hydraulic loading rate, (cm/d) (limited to 20 cm/d)
Consider a ratio of length to width, L: W equal to 3: 1,
where L = length (m), W = width (m).
where tCW = detention time in constructed wetland (d), Sl = slope (%) or water head difference and kC = hydraulic conductivity (m/s) (range 0.001–0.1 m/s).
but,
where
but,
where CFCe = coliform in effluent (Counts/100 mL), CFCo = coliform in influent (Counts/100 mL), KFC = temperature rate constant (d−1), and n = number of constructed wetland cells.
where Pi = Inflow phosphate, (mg/L), Pe = Effluent phosphate, (mg/L), Kp = phosphate removal rate constant (2.73 cm/day).
The cost-benefit analysis was conducted to estimate the total costs and benefits of recovering resources from treated wastewater. The treatment costs include the expenses related to land acquisition for implementing wastewater treatment technologies, which ultimately lead to the economic benefits derived from the effluents and resources obtained after treatment [90]. The cost-benefit analysis was calculated using Eqs 84–93.
where CETP = costs for effluent treatment facility (TZS), CCo = costs for construction of effluent treatment facility (TZS), and CLa = costs for land acquisition for construction of effluent (TZS).
where BWWT = benefits from received wastewater treatment (TZS), τan = annum period (d), δ = wastewater treatment tariff (TZS), and Qi = inflow wastewater flow rate (m3/d).
but,
further,
Where BRG = benefits from resources gained (TZS), BRR = benefits from resource recycling (TZS) BEMP = benefits from employment (TZS), BENP = benefits from environmental protection (TZS), BTW = benefits from treated water gained (TZS), τan = annum period (d), α = water tariff for irrigation purposes (TZS), Qe = treated water flow rate (m3/d), BNu = benefits from nutrient like nitrogen, phosphorous and potassium (NPK) gained, NuTW = nutrient (NPK) gained from treated water (TZS), NuSl = nutrient (NPK) gained from sludge (TZS), CoNef = concentration of nitrogen in effluent/treated water (mg/L), CoPef = concentration of phosphorus in effluent/treated water (mg/L), CoKef = concentration of potassium in effluent/treated water (mg/L), CoNsl = concentration of nitrogen in sludge (mg/L), CoPsl = concentration of phosphorus in sludge (mg/L), CoKsl = concentration of potassium in sludge (mg/L), Vsl = Volumetric rate of sludge (m3/d), MpNPK–F = Market price of NPK fertilizer (TZS), BBG = benefits from biogas generation (TZS), ψ =electricity unit price (TZS/KWh), VBG = volume of biogas (m3), ϵ = fraction of methane in biogas (%), Nan = paid number of days per annum, n = number of pollutants, i, βi = number of laborers employed, Ci = average unit cost paid to one laborers in a day (TZS), ηi = unit discharge fee per pollution equivalent of pollutant, i (TZS), ei = concentration of pollutant, i, λi = standard concentration of pollutant, i, γi = cost for reduction weight of pollutant, i, (TZS), and φi = discharge concentration of pollutant i.
The net benefit value (NBV) is a model indicator as computed to justify the economically most advantageous effluent/wastewater treatment technology [91] as expressed by Eq 94.
where NBV = net value of benefit (TZS).
The operational costs may refer to all costs incurred to maintain and operate the wastewater treatment plant and include costs for personnel, maintenance costs, operational costs, chemicals, utilities, laboratory supplies, office supplies, aeration costs (electricity), and sludge disposal costs, discharge costs for treated water into water bodies and costs for equivalent reduction of air pollutant [92] as presented in Eq 95.
where COM = operation and maintenance costs (TZS), Coi = component i, that uses electricity (kW), Etar = unit electricity tariffs (TZS/kWh), Pai = parameter i, for water quality monitoring, AnCi = analysis costs for parameter i, (TZS), Cmo = costs for water quality monitoring (TZS), Pocw = costs for discharge treated water into water bodies (TZS/kgPO43− p. e/yr), and Poca = costs for equivalent reduction of air pollutant (TZS/kgCO2 p. e/yr) and p. e = pollution equivalent.
Sustainability indicators are the specific measurements of value assigned to reflect assessment criteria and the overall sustainability of technologies. In case of wastewater treatment technologies, the sustainability indicators applied are environmental, technical, social-cultural and economic dimensions [93]. The sustainability indicators are measured as individual sustainability indicators [94] as expressed in Eqs 96–99 and composite sustainability indicators [97] as expressed in Eq 100.
This is a normalization procedure of weights for criteria related to the removal efficiency of wastewater treatment system [94].
where W = normalized weight, Vwi = weights for parameter removal efficiency in ith wastewater treatment units, Vwj = sum weights of jth parameters removal efficiency, n = number of treatment units in wastewater treatment plant, and m = maximum value of parameters reduced by treatment plant.
where CECSi = economic sustainability of the ith wastewater treatment technology; CBENSi is the environmental sustainability of ith wastewater treatment technology; BSSi is the social sustainability of the ith wastewater treatment technology; i = 1, 2,..., n = number of wastewater treatment technology; p = 1, 2, ..., P = the number of economic indicators; q = 1, 2, ..., Q = number of environmental indicators; r = 1, 2, ..., R = number of social indicators; Wp = weight of the indicator p; Wq = weight of the indicator q; Wr = weight of the indicator r; NViq = normalized value of the ith wastewater treatment technology in the pth indicator; NVip = normalized value of the ith wastewater treatment technology in the qth indicator; and NVir is the normalized value of the ith wastewater treatment technology in the rth indicator.
The CSI is the overall sustainability of wastewater treatment technology based, environmental, economic and social indicators [97] as expressed in Eq 100.
where GSi = the global sustainability indicator of the ith wastewater treatment technology; WECSWENS and WSS = sustainability weights of economic, environmental, and social dimensions, respectively.
The scenarios were established in four main groups: R, S, T, and U [97]. Scenario R involved calculated dimensional weights of economic, environmental, and social indicators.
Scenario S considered all dimensional indicators to be of equal importance (33.33% for all dimensions). Scenario T was broken down into T1, T2, and T3, with one dimension considered to be more relevant at a time (weighing 50%) than the other dimensions, which were given the same importance (25% each). Scenario U was divided into U1, U2, and U3, with one dimension considered the most extreme, with a weight of 80% attributed to that dimension, compared to 10% for the other two dimensions. Ultimately, a total of eight scenarios were analyzed, each with predetermined weights for each dimension and established groups (S1, S2, S3), (T1, T2, T3), and (U1, U2, U3) in order to select a sustainable wastewater treatment technology.
The statistical analysis of data was performed using Origin Pro version 15.0-software and excel 2013 for data fitting and analysis of variance (ANOVA) was performed to compare variances across the average (mean) of different groups.
The WSPs at MUWSA were designed to treat 4500 m3/d of municipal wastewater from sewer connected and unconnected households. The performance of the WSPs in removing COD, BOD5, and TSS is shown in Figure 2, and the removal of NO3−, PO43−, and K is shown in Figure 3. The monitored performance of the WSPs (Figure 2) in removing COD, BOD5, and TSS in the years 2014 and 2016 met the allowable discharge limits set by Tanzania's effluent standards (TSE). However, in 2017, the WSPs were found to release effluent with higher concentrations of COD, BOD5, and TSS compared to TSE.
The effluent from WSPs in 2014 and 2016 (Figure 3), was found to contain lower NO3−, PO43−, and K compared to TSE, in contrast to the higher concentration of nutrients observed to be discharged in 2017.
Furthermore, the reduction of fecal coliform (FC) in WSPs (Figure 4) in the year 2014 and 2016 was below the discharge limits acceptable for effluent release into environment (TSE), while in 2017 levels were observed to be higher than TSE.
The monitored performance of WSPs in removing contaminants as presented in Figures 2–4 was found to be influenced by various conditions revealed within WSPs' as shown in Table 1 during performance monitoring. The levels of pH, T, EC and TDS in WSPs were found to be within the recommended conditions TSE for the records of 2014, 2016, and 2017. However, DO was not measured in 2014 and 2016 for unknown reasons.
When it was measured in 2017, it was observed to have a lower concentration than the recommended level according to Tanzania's standards for effluent discharge from municipal and industrial wastewater treatment technologies. It was noted that, the DO level in 2017 had increased in the facultative pond from 0.55 mg/L to 0.95 mg/L in the effluent released from Maturation 6. However, this was lower than the recommended DO level of 3.50 mg/L as per Tanzania's standards for effluent.
During the monitoring of WSPs at MUWSA, heavy metals were also analyzed to assess the safety of the treated effluent discharged for irrigation of pad farm at Mabogini area, as shown in Table 2. It was observed in Table 2, that all measured heavy metals such as Zn, Cr, S, Pb, Cd, Fe, and Cu were within the acceptable limits set as per Tanzania standards for effluent discharge (TSE).
WSPs | 2014 |
2016 |
2017 |
||||||||||
pH | T °C | EC (µS/cm) | TDS (mg/L) | pH | T °C | EC (µS/cm) | TDS (mg/L) | pH | T °C | EC (µS/cm) | TDS (mg/L) | DO (mg/L) | |
Inlet anaerobic pond | 7.33 | 26.80 | 891 | 438 | 7.98 | 26.70 | 704 | 350 | 6.86 | 28.90 | 952 | 626 | 0.15 |
Inlet facultative pond | 7.45 | 27.00 | 845 | 423 | 7.47 | 25.10 | 667 | 321 | 7.60 | 28.60 | 920 | 609 | 0.23 |
Outlet facultative pond | 7.57 | 26.90 | 815 | 410 | 7.69 | 25.60 | 600 | 297 | 7.66 | 30.03 | 890 | 582 | 0.55 |
Outlet maturation pond 6 | 7.87 | 26.50 | 795 | 391 | 7.85 | 26.10 | 601 | 299 | 8.11 | 31.10 | 860 | 560 | 0.95 |
TSE (mg/L) | 6.50–8.50 | 25 | 1500 | 3000 | 6.50–8.50 | 25 | 1500 | 3000 | 6.50–8.50 | 25 | 1500 | 3000 | 3.50 |
WSPs | 2014 |
2016 |
2017 |
||||||||||
Zn | Cr | S | Zn | Cr | S | Zn | Cr | S | Pb | Cd | Fe | Cu | |
Inlet anaerobic pond | 0.53 | 0.33 | 0.22 | 0.97 | 0.43 | 1.31 | 0.85 | 0.95 | 1.25 | 1.75 | 0.75 | 10.00 | 9.50 |
Inlet facultative pond | 0.38 | 0.27 | 0.18 | 0.60 | 0.39 | 0.71 | 0.65 | 0.75 | 0.75 | 0.55 | 0.35 | 5.25 | 6.55 |
Outlet facultative pond | 0.32 | 0.24 | 0.15 | 0.44 | 0.18 | 0.35 | 0.35 | 0.57 | 0.45 | 0.14 | 0.11 | 3.15 | 4.75 |
Outlet maturation pond 6 | 0.24 | 0.11 | 0.10 | 0.17 | 0.13 | 0.14 | 0.25 | 0.45 | 0.35 | 0.07 | 0.05 | 2.55 | 1.75 |
TSE (mg/L) | 1.00 | 1.00 | 0.50 | 1.00 | 1.00 | 0.50 | 1.00 | 1.00 | 0.50 | 0.10 | 0.10 | 5.00 | 2.00 |
WSPs and enhanced AFP-CW were evaluated as technologies to determine the best option for treating organic-rich wastewater. A comparative study was conducted to select the most suitable organic-rich wastewater treatment technology using sustainability indicators such as economic, environmental, and social factors [95],[96]. The design flow rate of 4500 m3/d and wastewater characteristics analyzed in 2017 (refer Figures 2–4) were used as inputs to mathematical model to design WSPs and AFP-CW technologies.
The results of economic, environmental, and social indicators for individual sustainability for AFP-CW and WSPs were presented in Table 3.
Dimensions | Indicators | AFP-CW |
WSPs |
||
Weighting indicator (%) | Weight of dimensions (%) | Weighting indicator (%) | Weight of dimensions (%) | ||
Economic | Investment Costa | 52.16 | 10.18 | 78.43 | 14.55 |
Operation and Maintenance Costa | 0.58 | 0.15 | |||
Environmental | Energy consumptiona | 0.01 | 0.001 | ||
Land area requireda | 0.61 | 3.64 | |||
Pollutant removal efficiency costsa | 44.28 | 14.12 | |||
Water-bodies Eutrophicationa | 1.81 | 51.11 | 0.60 | 48.39 | |
Global Warminga | 0.02 | 2.93 | |||
Durabilityb | 75 | 80 | |||
Flexibilityb | 68 | 75 | |||
Reliabilityb | 75 | 85 | |||
Social | Employmentb | 0.53 | 0.12 | ||
Public acceptanceb | 85 | 55 | |||
Complexityb | 50 | 38.71 | 25 | 37.06 | |
Odour impactb | 25 | 70 | |||
Noise impactb | 20 | 10 | |||
Visual impacta | 20 | 40 | |||
Total | 518.00 | 100.00 | 540.00 | 100.00 |
Note: Where a the cost due to bills of quantities; b adopted secondary data
Referring to Table 3, it was found that the environmental sustainability indicators for both AFP-CW and WSPs were given highest weight, followed by social indicators and the lowest weight was assigned to economic indicators. Specifically, the environmental and social sustainability indicators for AFP-CW had a higher weight than those for WSPs, while the economic sustainability indicator for WSPs had a higher weight than that of AFP-CW.
The CI for sustainability of AFP-CW and WSPs treatment technology was determined by adding together the weights of the economic, environmental, and social indicators listed in Table 3. The global sustainability indicator (GSi) considers all indicators for each sustainability dimension simultaneously, as shown in Table 4.
S/No. | Sustainability | AFP-CW | WSPs | ||
1 | Economic | 1.05 | 0.04 | 2.12 | 0.11 |
2 | Environmental | 26.15 | 16.15 | 23.41 | 13.96 |
3 | Social | 14.94 | 5.35 | 13.74 | 4.81 |
CI (%) | 42.14 | 39.27 | |||
GSi (%) | 21.54 | 18.88 |
Table 3 shows that the CSI and global GSi for AFP-CW were higher than those for WSPs. This suggests that AFP-CW may be a preferable option compared to WSPs in terms of a multidimensional measure of sustainability. The environmental aspects of AFP-CW were given more importance than WSPs, followed by social indicators. However, the economic aspect, which had the lowest weight among all indicators, was found to be higher for WSPs than for AFP-CW as shown in Figure 5.
To determine the best organic-rich wastewater treatment option, a CSI was used to calculate the percentage of global GSi for AFP-CW and WSPs, as shown in Figure 6. It was found that the AFP-CW technology for municipal wastewater treatment had a higher percentage of CSI and global sustainability indicator compared to WSPs. The results can bring an obvious conclusion that the AFP-CW is the best option for treating organic-rich wastewater. However, the results need the validate from dimension indicators used to calculate the CSI and GSi [95].
It is sensitivity analysis that bring insights on variations between dimensions as influential factors for the selection of the appropriate organic-rich wastewater treatment technologies. Scenario analysis was performed in order to validate the dimension indicators used to calculate the composite and global sustainability indicators as key factors for selection of appropriate technology in question. Therefore, eight scenarios were analyzed for sensitivity analysis, as shown in Table 5.
The scenario R had original dimensional weight of sustainability indicators (Table 3) for AFP-CW and WSPs wastewater treatment technologies. Scenario, S was assigned an equal dimensional weight of 33.33% for economic, environmental, and social indicators. Scenario, T1 was assigned a 50% dimensional weight, while T2 and T3 were assigned 25% each. Scenario, U1, was assigned the highest dimensional weight of 80%, while U2 and U3 were each assigned equal dimensional weights of 10%.
Scenario | AFP-CW Dimensional weight (%) |
WSPs Dimensional weight (%) |
||||
Economic | Environmental | Social | Economic | Environmental | Social | |
R | 10.18 | 51.11 | 38.71 | 14.55 | 48.39 | 37.06 |
S | 33.33 | 33.33 | 33.33 | 33.33 | 33.33 | 33.33 |
T1 | 50 | 25 | 25 | 50 | 25 | 25 |
T2 | 25 | 50 | 25 | 25 | 50 | 25 |
T3 | 25 | 25 | 50 | 25 | 25 | 50 |
U1 | 80 | 10 | 10 | 80 | 10 | 10 |
U2 | 10 | 80 | 10 | 10 | 80 | 10 |
U3 | 10 | 10 | 80 | 10 | 10 | 80 |
Therefore, each scenario in Table 5 has a total of 100% as the sum of dimensional weights of economic, environmental and social indicators. By utilizing the dimensional weights for scenarios in Table 5, the maximum, calculated and minimum global sustainability indicators were determined as shown in Table 6.
Dimensional weight (%) | AFP-CW |
WSPs |
||||
Economic | Environmental | Social | Economic | Environmental | Social | |
Maximum | 80.00 | 80.00 | 80.00 | 80.00 | 80.00 | 80.00 |
Minimum | 10 | 10 | 10 | 10 | 10 | 10 |
Average | 30.44 | 35.56 | 34.01 | 30.99 | 35.22 | 33.80 |
Standard deviation | 24.36 | 23.78 | 23.02 | 23.88 | 23.55 | 22.98 |
Maximum-GSi | 22.34 | 19.54 | ||||
Calculated-GSi | 21.54 | 18.88 | ||||
Minimum-GSi | 7.26 | 6.32 |
The max-GSi, cal-GSi, and min-GSi for AFP-CW technology were obtained from Table 6 and found to have a higher weight than that of WSPs. To validate if the CI were significantly different from AFP-CW and WSPs technologies, the sensitivity analysis was performed as shown in Figures 7 and 8.
Referring to Figure 7, the environmental indicator for global sustainability of AFP-CW was found to be highly sensitive, social was medium indicator and economic indicators was the lowest.
From Figure 8, it was found that the environmental indicator for global sustainability of WSPs is highly sensitive, whereas the social and economic indicators are less sensitive.
The variations in sensitivity shown in Figures 7 and 8 suggest that one of the two technologies may be more suitable for treating organic-rich wastewater for resource recovery. To determine the most suitable technology, the stability of the technology must be considered as the most important factor. Therefore, the variations in the GSi (Table 6), including the minimum GSi value, model GSi value (calculated), and maximum GSi value for AFP-CW and WSPs, were illustrated in the bar graph shown in Figure 9.
The length of the graph intervals (Figure 9) indicates the stability levels of the evaluated technologies for treating organic-rich wastewater for resource recovery. It was observed that the GSi for AFP-CW had a difference of 0.80% between the max-GSi and cal-GSi. For WSPs, the difference between the max-GSi and cal-GSi was 0.66%.
The performance of WSPs operated by MUWSA, as documented in the year 2014 and 2016, complied with Tanzania's standards for effluent discharge from treatment systems [43],[99]. As shown in Figure 2, the effluent from WSPs in 2014 and 2016 contained COD < 60 mg/L, BOD5 < 30 mg/L, and TSS < 100 mg/L. Likewise, the effluent contained NO3− < 20 mg/L, PO43− < 15 mg/L, and K < 30 mg/L (Figure 3). However, in 2017, the quality of the effluent deteriorated, with concentrations of certain parameters exceeding permissible limits, with the exception of fecal coliforms (Figure 4) and heavy metals (Table 2). The fecal coliform counts analyzed in 2014, 2016, and 2017 (Figure 4) were consistently below 1,000 counts /100 mL, which is an acceptable limit for discharging effluent into the environment and for irrigation purposes [100],[101]. Furthermore, the levels of heavy metals were also found to be below the Tanzanian standards for effluent (TSE) [43], as indicated in Table 2. The low concentrations of heavy metals, particularly chromium (Cr) at 0.95 mg/L, lead (Pb) at 1.75 mg/L, and cadmium (Cd) at 0.75 mg/L (Table 2), suggest that the influent primarily originated from households.
Additionally, the concentrations of Cd, Pb, and Cr in the effluent of WSPs were lower than 1.00 mg/L, 0.10 mg/L, and 0.10 mg/L, respectively, indicating that the effluent is safe and has potential for use in irrigation [102]. Findings revealed that the performance of the WSPs (Figures 2–4) in the years 2014, 2016, and 2017 may have been influenced by the availing favorable conditions, as shown in Table 1. The performance driving factors such as T (25 °C), pH (6.5–8.5), EC (1500 µS/cm), and TDS (3000 mg/L) of the WSPs were found to be within the recommended standards, including [43]. However, DO was observed to increase from 0.15 mg/L in the facultative ponds to 0.95 mg/L in Maturation 6. Nevertheless, the effluent DO in Maturation 6 was less than the recommended level of 3.50 mg/L for effluent from treatment technologies (Figure 5) [99].
In general, it was observed that the performance of WSPs in 2014 and 2016 was better than in 2017. The anaerobic pond, which was designed to serve as the primary treatment pond in 2017, was abandoned for treating influent due to being filled with sludge. As a result, the influent was redirected to facultative ponds, which operate as secondary treatment ponds. However, the performance of these ponds was found to decrease. This decline in performance occurred because, without the anaerobic pond, the breakdown of recalcitrant organic matter was impaired, and the retention time to enhance the settling of suspended solids was not sufficient.
Additionally, channeling the influent to facultative ponds decreased DO level to 0.15 mg/L, resulting in anaerobic conditions (Table 1). This observation suggests that the facultative ponds have begun to accumulate sludge, which could lead to significant poor performance of WSPs over time [43]. Consequently, the WSPs release high concentrations of suspended solids exceeding 100 mg/L in the effluent, along with other parameters into the maturation ponds due to reduced retention time and digestion capacity. The results obtained can be compared to findings by Letshwenyo et al. [103] as reported that maturation ponds release 150 mg/L of suspended solids as result of partial degradation of organic matter, which in turn leads to production of new cells in maturation ponds. Furthermore, a decline in the performance of WSPs of MUWSA at Mabogini was observed. This decline was attributed to fluctuations in driving factors such as retention time, temperature, dissolved oxygen, and redox potential, which were caused by the malfunctioning of the anaerobic pond [104],[105]. In order to mitigate the negative effects of reduced performance in WSPs, it is recommended to use two anaerobic ponds in series. One pond should be dedicated to sludge settling, while the other serves as a standby during the desludging process of the first pond [99]. Additionally, incorporating a constructed wetland to improve the effluent from WSPs has the potential to reduce suspended solids, nutrients, algae, BOD5, total suspended solids (TSS), heavy metals, and pathogens, making the effluent suitable for agricultural applications [43],[100],[102],[106].
The effectiveness of WSPs in treating organic-rich wastewater was further assessed using sustainability indicators, which included economic, environmental, and social factors [32],[80]. The evaluation compared WSPs with advanced facultative ponds with AFP-CW to identify the most sustainable technology for municipal wastewater treatment. This analysis aimed to address concerns regarding WSPs, such as the significant area required for implementation, challenges in recovering nutrients from sludge and biogas, and their contributions to global warming, while also considering the benefits of nutrient and water recycling for irrigation purposes [45],[99],[105].
Referring to Table 3, it was noted that both AFP-CW and WSP technologies had a higher percentage of environmental sustainability indicators compared to social and economic sustainability indicators. Specifically, for AFP-CW, the weights of the environmental, social, and economic indicators were 51.11%, 38.71%, and 10.18%, respectively. For WSPs, the weights of these indicators were 48.39%, 37.06%, and 14.55%, respectively. However, the economic indicator for AFP-CW was rated lower than that for WSPs [96]. To expand the decision-making framework for selecting the appropriate technology for treating organic-rich wastewater, the dimensional CI and GSi were calculated (see Table 3). The GSi was determined to provide a multidimensional measure of sustainability for technology selection [98].
Referring to Figure 5, the dimensional environmental, social, and economic indicators for AFP-CW were 26.12%, 14.99%, and 1.04%, respectively. For WSPs, the corresponding indicators were 23.41%, 13.73%, and 2.12%. The observed dimensional environmental and social indicators for AFP-CW were higher than those for WSPs, while the economic indicator was lower than that of WSPs. The Composite Index (CI) was calculated to be 42.14% for AFP-CW and 39.27% for WSPs. The global indicator, GSi, was 21.54% for AFP-CW and 18.88% for WSPs, as shown in Figure 6. The global sustainability composite indicators were evaluated using eight scenarios, which are presented in Figure 7a,b. The composite sustainability environmental indicator for AFP-CW and WSPs was found to be highly sensitive, followed by the social and economic indicators.
This suggests that AFP-CW performs better on all sustainability indicators compared to WSPs. However, it is insufficient to rely solely on the legitimacy of the dimensional indicators when selecting appropriate technology. Therefore, a sensitivity scenario analysis was conducted to assess variations in the best and worst sensitivity of the composite sustainability indicator [39].
The selection of the appropriate organic-rich wastewater treatment technology was based on the differences observed between the best (max-GSi) and worst (min-GSi) case scenarios. According to Table 6, max-GSi for AFP-CW was 22.34%, compared to 21.54% as shown in Table 4. For WSPs, the max-GSi was 19.54%, compared to 18.88% as indicated in Table 4. The min-GSi for AFP-CW and WSPs were 7.26% and 6.32%, respectively. The interval length between the calculated global sustainability indicator (Cal-GSi) and the max-GSi reflects the stability level of the evaluated technologies. Results showing the different intervals are presented in Figure 8. The variability of GSi was 14.28% for AFP-CW and 12.56% for WSPs. The percentage variation was 63.92 for AFP-CW, while for WSPs it was 64.28. Given the percentage variation in global sustainability indicators, AFP-CW is deemed an appropriate technology option for treating organic-rich municipal wastewater. This is primarily due to its sustainability related to environmental factors, followed by social considerations, as well as lower implementation costs compared to WSPs.
Additionally, AFP-CW has the advantage of recovering nutrients and biogas during the municipal wastewater treatment process. When the AFP-CW system is applied to treat municipal wastewater at a flow rate of 4500 m³/day, it has the potential to recover 213 kg/ha of nitrogen, 30 kg/ha of phosphorus, and 120 kg/ha of potassium in the effluent for irrigation purposes annually. Furthermore, from the 182.60 tons/year of sludge generated as dry matter (biofertilizer) in the treatment of 4500 m³/day of municipal wastewater, around 704 kg N/ha, 337.50 kg P/ha, and 313 kg K/ha can be recovered for land application. This results in a supplementary potential of approximately 169.31 kg NPK/ha/year for agricultural use. However, a major concern regarding sludge application is the content of heavy metals. Therefore, the application of sludge to agricultural land must adhere to annual recommended maximum concentrations of heavy metals per hectare, which should not exceed 0.75 g of cadmium, 300 g of copper, 1.5 g of mercury, 25 g of lead, and 600 g of zinc per kg of dry matter [107]. Another advantage of AFP-CW is energy recovery; it can produce 1071 m³/day of biogas from a wastewater flow rate of 4500 m³/day.
The performance assessment of WSPs at MUWSA in 2014 and 2016 was revealed that, the effluent released into environment complied with Tanzania standards. The effluent discharged was found to have concentrations of COD < 60 mg/L, BOD5 < 30 mg/L, TSS < 100 mg/L, NO3− < 20 mg/L, PO43− < 15 mg/L, and K < 30 mg/L as well as acceptable levels for pathogens, and heavy metals. However, in 2017, it was observed that effluent from WSPs was discharged with levels higher than the aforementioned standards. In the case of fecal coliforms, the effluent from the WSPs had counts < 1000 counts/mL which is recommended for irrigation purposes. In case of heavy metals, the released effluent contained concentrations below acceptable limits such as Zn < 1.0 mg/L, Cr < 1.0 mg/L, Pb < 0.1 mg/L, Cd < 0.1 mg/L, Fe < 5.0 mg/L, and Cu < 2.0 mg/L. During the analysis of economic, environmental, and social (EES) indicators for selecting appropriated technology for municipal wastewater treatment and resource recovery, AFP-CW was found to be a more sustainable treatment option compared to WSPs. This conclusion was influenced by environmental factors such as recovery of biofertilizer, biogas, and reduction of global warming potential. Additionally, AFP-CW demonstrated greater effectiveness in reducing environmental pollutants, recovery and recycling nutrients, and release safer treated water compared to WSPs. From an economic perspective, AFP-CW proved to be a more sustainable choice for treating organic-rich wastewater, as it incurs lower costs compared to WSPs. Considering the treatment of municipal wastewater with a flow rate of 4,500 m3/d using AFP-CW technology, significant nutrients can be derived. The process can generate 182.60 tons of sludge annually, which can be used as biofertilizer. The NPK content from the effluent amounts to 545 kg/ha per year, resulting in savings of 9,147,600 TZS, along with 169.31 kg/ha per year for biofertilizer, leading to an additional saving of 2,844,450 TZS. Furthermore, AFP-CW technology can enhance energy recovery from biogas, producing 1,071 m3/d. This can generate approximately 215,003.25 kWh of electrical energy per year, translating to savings of about 76,650,808.66 TZS in electricity costs.
Given the inherent benefits of using AFP-CW technology for municipal wastewater treatment, it is essential to engage various stakeholders to discuss additional criteria for selecting the most appropriate technology. This process should consider the diverse opinions and preferences of key stakeholders, including decision-makers, experts, planners, and analysts, as well as interest groups such as political parties, civic organizations, and local residents. The goal is to develop a policy for the implementation of appropriate technology for municipal wastewater treatment and resource recovery.
The authors declare they have not used Artificial Intelligence (AI) tools in the creation of this article.
[1] |
Sharp R, Williams R (1995) Thermus Species. New York: Springer. https://doi.org/10.1007/978-1-4615-1831-0 ![]() |
[2] |
Saghatelyan A, Panosyan H, Trchounian A, et al. (2021) Characteristics of DNA polymerase I from an extreme thermophile, Thermus scotoductus strain K1. MicrobiologyOpen 10: e1149. https://doi.org/10.1002/mbo3.1149 ![]() |
[3] |
Kristjánsson JK, Hjörleifsdóttir S, Marteinsson VT, et al. (1994) Thermus scotoductus, sp. nov., a pigment-producing thermophilic bacterium from hot tap water in iceland and including Thermus sp. X-1. Syst Appl Microbiol 17: 44-50. https://doi.org/10.1016/S0723-2020(11)80030-5 ![]() |
[4] |
Babák L, Šupinová P, Burdychová R (2013) Growth models of Thermus aquaticus and Thermus scotoductus. Acta Univ Agric Silvic Mendel Brun 60: 19-26. https://doi.org/10.11118/actaun201260050019 ![]() |
[5] |
Aulitto M, Fusco S, Fiorentino G, et al. (2017) Thermus thermophilus as source of thermozymes for biotechnological applications: Homologous expression and biochemical characterization of an α-galactosidase. Microb Cell Fact 16: 28. https://doi.org/10.1186/s12934-017-0638-4 ![]() |
[6] |
Wheaton S, Hauer M (2011) Recent applications of THERMUS. Phys Part Nuclei Lett 8: 869-873. https://doi.org/10.1134/S1547477111080152 ![]() |
[7] |
Da Costa MS, Rainey FA, Nobre MF (2006) The Genus Thermus and relatives. The Prokaryotes. New York: Springer 797-812. https://doi.org/10.1007/0-387-30747-8_32 ![]() |
[8] |
Elleuche S, Schröder C, Sahm K, et al. (2014) Extremozymes—biocatalysts with unique properties from extremophilic microorganisms. Curr Opin Biotechnol 29: 116-123. https://doi.org/10.1016/j.copbio.2014.04.003 ![]() |
[9] | Chen L, Biosketch S, Sharmili A, et al. (2019) Advances and Trends in Biotechnology and Genetics, Vol. 3. London: Book Publisher International, SCIENCEDOMAIN International Ltd. http://dx.doi.org/10.9734/bpi/atbg/v3 |
[10] |
Albers SV, Vossenberg JL, Driessen AJ, et al. (2001) Bioenergetics and solute uptake under extreme conditions. Extremophiles 5: 285-294. https://doi.org/10.1007/s007920100214 ![]() |
[11] |
Somayaji A, Dhanjal CR, Lingamsetty R, et al. (2022) An insight into the mechanisms of homeostasis in extremophiles. Microbiol Res 263: 127115. https://doi.org/10.1016/j.micres.2022.127115 ![]() |
[12] |
Vieille C, Zeikus GJ (2001) Hyperthermophilic Enzymes: Sources, uses, and molecular mechanisms for thermostability. Microbiol Mol Biol Rev 65: 1-43. https://doi.org/10.1128/MMBR.65.1.1-43.2001 ![]() |
[13] |
Rekadwad BN, Li WJ, Gonzalez JM, et al. (2023) Extremophiles: the species that evolve and survive under hostile conditions. 3 Biotech 13: 316. https://doi.org/10.1007/s13205-023-03733-6 ![]() |
[14] |
Siliakus MF, van der Oost J, Kengen SWM (2017) Adaptations of archaeal and bacterial membranes to variations in temperature, pH and pressure. Extremophiles 21: 651-670. https://doi.org/10.1007/s00792-017-0939-x ![]() |
[15] |
Dick JM, Boyer GM, Canovas PA, et al. (2023) Using thermodynamics to obtain geochemical information from genomes. Geobiology 21: 262-273. https://doi.org/10.1111/gbi.12532 ![]() |
[16] |
Cava F, Hidalgo A, Berenguer J (2009) Thermus thermophilus as biological model. Extremophiles 13: 213-231. https://doi.org/10.1007/s00792-009-0226-6 ![]() |
[17] |
Mefferd CC, Zhou E, Seymour CO, et al. (2022) Incomplete denitrification phenotypes in diverse Thermus species from diverse geothermal spring sediments and adjacent soils in southwest China. Extremophiles 26: 23. https://doi.org/10.1007/s00792-022-01272-1 ![]() |
[18] |
Saghatelyan A, Poghosyan L, Panosyan H, et al. (2015) Draft genome sequence of Thermus scotoductus strain K1, Isolated from a Geothermal Spring in Karvachar, Nagorno Karabakh. Genome Announc 3: 10. https://doi.org/10.1128/genomeA.01346-15 ![]() |
[19] |
Kieft TL, Fredrickson JK, Onstott TC, et al. (1999) Dissimilatory reduction of Fe(III) and other electron acceptors by a Thermus Isolate. Appl Environ Microbiol 65: 1214-1221. https://doi.org/10.1128/AEM.65.3.1214-1221.1999 ![]() |
[20] |
Skirnisdottir S, Hreggvidsson GO, Holst O, et al. (2001) Isolation and characterization of a mixotrophic sulfur-oxidizing Thermus scotoductus. Extremophiles 5: 45-51. https://doi.org/10.1007/s007920000172 ![]() |
[21] |
Cordova LT, Lu J, Cipolla RM, et al. (2016) Co-utilization of glucose and xylose by evolved Thermus thermophilus LC113 strain elucidated by 13 C metabolic flux analysis and whole genome sequencing. Metab Eng 37: 63-71. https://doi.org/10.1016/j.ymben.2016.05.001 ![]() |
[22] |
Boyer GM, Schubotz F, Summons RE, et al. (2020) Carbon oxidation state in microbial polar lipids suggests adaptation to hot spring temperature and redox gradients. Front Microbiol 11: 229. https://doi.org/10.3389/fmicb.2020.00229 ![]() |
[23] |
Straub CT, Zeldes BM, Schut GJ, et al. (2017) Extremely thermophilic energy metabolisms: Biotechnological prospects. Curr Opin Biotechnol 45: 104-112. https://doi.org/10.1016/j.copbio.2017.02.016 ![]() |
[24] |
Gounder K, Brzuszkiewicz E, Liesegang H, et al. (2011) Sequence of the hyperplastic genome of the naturally competent Thermus scotoductus SA-01. BMC Genomics 12: 577. https://doi.org/10.1186/1471-2164-12-577 ![]() |
[25] |
Radax C, Sigurdsson O, Hreggvidsson GO, et al. (1998) F- and V-ATPases in the genus Thermus and related species. Syst Appl Microbiol 21: 12-22. https://doi.org/10.1016/S0723-2020(98)80003-9 ![]() |
[26] |
Ranawat P, Rawat S (2017) Stress response physiology of thermophiles. Arch Microbiol 199: 391-414. https://doi.org/10.1007/s00203-016-1331-4 ![]() |
[27] |
Bruins ME, Janssen AEM, Boom RM (2001) Thermozymes and their applications: A review of recent literature and patents. Appl Biochem Biotechnol 90: 155-186. https://doi.org/10.1385/abab:90:2:155 ![]() |
[28] |
Tadevosyan M, Yeghiazaryan S, Ghevondyan D, et al. (2022) Extremozymes and Their Industrial Applications.Elsevier 177-204. https://doi.org/10.1016/B978-0-323-90274-8.00007-1 ![]() |
[29] |
Pantazaki A, Pritsa A, Kyriakidis D (2002) Biotechnologically relevant enzymes from Thermus thermophilus. Appl Microbiol Biotechnol 58: 1-12. https://doi.org/10.1007/s00253-001-0843-1 ![]() |
[30] |
Mandelli F, Miranda VS, Rodrigues E, et al. (2012) Identification of carotenoids with high antioxidant capacity produced by extremophile microorganisms. World J Microbiol Biotechnol 28: 1781-1790. https://doi.org/10.1007/s11274-011-0993-y ![]() |
[31] |
Krulwich TA, Sachs G, Padan E (2011) Molecular aspects of bacterial pH sensing and homeostasis. Nat Rev Microbiol 9: 330-343. https://doi.org/10.1038/nrmicro2549 ![]() |
[32] |
Kim YJ, Lee HS, Kim ES, et al. (2010) Formate-driven growth coupled with H2 production. Nature 467: 352-355. https://doi.org/10.1038/nature09375 ![]() |
[33] |
Petrosyan H, Vanyan L, Mirzoyan S, et al. (2020) Roasted coffee wastes as a substrate for Escherichia coli to grow and produce hydrogen. FEMS Microbiol Lett 367: fnaa088. https://doi.org/10.1093/femsle/fnaa088 ![]() |
[34] |
Puchkov EO, Bulatov IS, Zinchenko VP (1983) Investigation of intracellular pH in Escherichia coli by 9-aminoacridine fluorescence measurements. FEMS Microbiol Lett 20: 41-45. https://doi.org/10.1111/j.1574-6968.1983.tb00086.x ![]() |
[35] |
Gevorgyan H, Khalatyan S, Vassilian A, et al. (2021) The role of Escherichia coli FhlA transcriptional activator in generation of proton motive force and FoF1-ATPase activity at pH 7.5. IUBMB Life 73: 883-892. https://doi.org/10.1002/iub.2470 ![]() |
[36] |
Gevorgyan H, Khalatyan S, Vassilian A, et al. (2022) Metabolic pathways and ΔpH regulation in Escherichia coli during the fermentation of glucose and glycerol in the presence of formate at pH 6.5: The role of FhlA transcriptional activator. FEMS Microbiol Lett 369: fnac109. https://doi.org/10.1093/femsle/fnac109 ![]() |
[37] |
Katsu T, Nakagawa H, Yasuda K (2002) Interaction between polyamines and bacterial outer membranes as investigated with ion-selective electrodes. Antimicrob Agents Chemother 46: 1073-1079. https://doi.org/10.1128/AAC.46.4.1073-1079.2002 ![]() |
[38] |
Zakharyan E, Trchounian A (2001) K+ influx by Kup in Escherichia coli is accompanied by a decrease in H+ efflux. FEMS Microbiol Lett 204: 61-64. https://doi.org/10.1111/j.1574-6968.2001.tb10863.x ![]() |
[39] | Nicholls DG, Ferguson SJ (2013) Bioenergetics.Elsevier 419. https://doi.org/10.1016/C2010-0-64902-9 |
[40] |
Shirvanyan A, Mirzoyan S, Trchounian K (2023) Relationship between proton/potassium fluxes and central carbon catabolic pathways in different Saccharomyces cerevisiae strains under osmotic stress conditions. Process Biochem 133: 309-318. https://doi.org/10.1016/j.procbio.2023.09.015 ![]() |
[41] |
Swarup A, Lu J, DeWoody KC, et al. (2014) Metabolic network reconstruction, growth characterization and 13C-metabolic flux analysis of the extremophile Thermus thermophilus HB8. Metab Eng 24: 173-180. https://doi.org/10.1016/j.ymben.2014.05.013 ![]() |
[42] | Saghatelyan A, Panosyan H, Birkeland NK (2021) The genus Thermus: A brief history of cosmopolitan extreme thermophiles: Diversity, distribution, biotechnological potential and applications. Microbial Communities and their Interactions in the Extreme Environment. Microorganisms for Sustainability 32: 141-175. https://doi.org/10.1007/978-981-16-3731-5_8 |
[43] |
Slonczewski JL, Fujisawa M, Dopson M, et al. (2009) Cytoplasmic pH measurement and homeostasis in bacteria and archaea. Adv Microb Physiol 55: 1-317. https://doi.org/10.1016/S0065-2911(09)05501-5 ![]() |
[44] |
Cook GM (2000) The intracellular pH of the thermophilic bacterium Thermoanaerobacter wiegelii during growth and production of fermentation acids. Extremophiles 4: 279-284. https://doi.org/10.1007/s007920070014 ![]() |
[45] |
Cook GM, Russell JB, Reichert A, et al. (1996) The intracellular pH of Clostridium paradoxum, an anaerobic, alkaliphilic, and thermophilic bacterium. Appl Environ Microbiol 62: 4576-4579. https://doi.org/10.1128/aem.62.12.4576-4579.1996 ![]() |
[46] |
Gezgin Y, Tanyolac B, Eltem R (2013) Some characteristics and isolation of novel thermostable β-galactosidase from Thermus oshimai DSM 12092. Food Sci Biotechnol 22: 63-70. https://doi.org/10.1007/s10068-013-0009-9 ![]() |
[47] |
Pantazaki AA, Papaneophytou CP, Pritsa AG, et al. (2009) Production of polyhydroxyalkanoates from whey by Thermus thermophilus HB8. Process Biochem 44: 847-853. https://doi.org/10.1016/j.procbio.2009.04.002 ![]() |
[48] |
Kang SK, Cho KK, Ahn JK, et al. (2005) Three forms of thermostable lactose-hydrolase from Thermus sp. IB-21: Cloning, expression, and enzyme characterization. J Biotechnol 116: 337-346. https://doi.org/10.1016/j.jbiotec.2004.07.019 ![]() |
WSPs | 2014 |
2016 |
2017 |
||||||||||
pH | T °C | EC (µS/cm) | TDS (mg/L) | pH | T °C | EC (µS/cm) | TDS (mg/L) | pH | T °C | EC (µS/cm) | TDS (mg/L) | DO (mg/L) | |
Inlet anaerobic pond | 7.33 | 26.80 | 891 | 438 | 7.98 | 26.70 | 704 | 350 | 6.86 | 28.90 | 952 | 626 | 0.15 |
Inlet facultative pond | 7.45 | 27.00 | 845 | 423 | 7.47 | 25.10 | 667 | 321 | 7.60 | 28.60 | 920 | 609 | 0.23 |
Outlet facultative pond | 7.57 | 26.90 | 815 | 410 | 7.69 | 25.60 | 600 | 297 | 7.66 | 30.03 | 890 | 582 | 0.55 |
Outlet maturation pond 6 | 7.87 | 26.50 | 795 | 391 | 7.85 | 26.10 | 601 | 299 | 8.11 | 31.10 | 860 | 560 | 0.95 |
TSE (mg/L) | 6.50–8.50 | 25 | 1500 | 3000 | 6.50–8.50 | 25 | 1500 | 3000 | 6.50–8.50 | 25 | 1500 | 3000 | 3.50 |
WSPs | 2014 |
2016 |
2017 |
||||||||||
Zn | Cr | S | Zn | Cr | S | Zn | Cr | S | Pb | Cd | Fe | Cu | |
Inlet anaerobic pond | 0.53 | 0.33 | 0.22 | 0.97 | 0.43 | 1.31 | 0.85 | 0.95 | 1.25 | 1.75 | 0.75 | 10.00 | 9.50 |
Inlet facultative pond | 0.38 | 0.27 | 0.18 | 0.60 | 0.39 | 0.71 | 0.65 | 0.75 | 0.75 | 0.55 | 0.35 | 5.25 | 6.55 |
Outlet facultative pond | 0.32 | 0.24 | 0.15 | 0.44 | 0.18 | 0.35 | 0.35 | 0.57 | 0.45 | 0.14 | 0.11 | 3.15 | 4.75 |
Outlet maturation pond 6 | 0.24 | 0.11 | 0.10 | 0.17 | 0.13 | 0.14 | 0.25 | 0.45 | 0.35 | 0.07 | 0.05 | 2.55 | 1.75 |
TSE (mg/L) | 1.00 | 1.00 | 0.50 | 1.00 | 1.00 | 0.50 | 1.00 | 1.00 | 0.50 | 0.10 | 0.10 | 5.00 | 2.00 |
Dimensions | Indicators | AFP-CW |
WSPs |
||
Weighting indicator (%) | Weight of dimensions (%) | Weighting indicator (%) | Weight of dimensions (%) | ||
Economic | Investment Costa | 52.16 | 10.18 | 78.43 | 14.55 |
Operation and Maintenance Costa | 0.58 | 0.15 | |||
Environmental | Energy consumptiona | 0.01 | 0.001 | ||
Land area requireda | 0.61 | 3.64 | |||
Pollutant removal efficiency costsa | 44.28 | 14.12 | |||
Water-bodies Eutrophicationa | 1.81 | 51.11 | 0.60 | 48.39 | |
Global Warminga | 0.02 | 2.93 | |||
Durabilityb | 75 | 80 | |||
Flexibilityb | 68 | 75 | |||
Reliabilityb | 75 | 85 | |||
Social | Employmentb | 0.53 | 0.12 | ||
Public acceptanceb | 85 | 55 | |||
Complexityb | 50 | 38.71 | 25 | 37.06 | |
Odour impactb | 25 | 70 | |||
Noise impactb | 20 | 10 | |||
Visual impacta | 20 | 40 | |||
Total | 518.00 | 100.00 | 540.00 | 100.00 |
Note: Where a the cost due to bills of quantities; b adopted secondary data
S/No. | Sustainability | AFP-CW | WSPs | ||
1 | Economic | 1.05 | 0.04 | 2.12 | 0.11 |
2 | Environmental | 26.15 | 16.15 | 23.41 | 13.96 |
3 | Social | 14.94 | 5.35 | 13.74 | 4.81 |
CI (%) | 42.14 | 39.27 | |||
GSi (%) | 21.54 | 18.88 |
Scenario | AFP-CW Dimensional weight (%) |
WSPs Dimensional weight (%) |
||||
Economic | Environmental | Social | Economic | Environmental | Social | |
R | 10.18 | 51.11 | 38.71 | 14.55 | 48.39 | 37.06 |
S | 33.33 | 33.33 | 33.33 | 33.33 | 33.33 | 33.33 |
T1 | 50 | 25 | 25 | 50 | 25 | 25 |
T2 | 25 | 50 | 25 | 25 | 50 | 25 |
T3 | 25 | 25 | 50 | 25 | 25 | 50 |
U1 | 80 | 10 | 10 | 80 | 10 | 10 |
U2 | 10 | 80 | 10 | 10 | 80 | 10 |
U3 | 10 | 10 | 80 | 10 | 10 | 80 |
Dimensional weight (%) | AFP-CW |
WSPs |
||||
Economic | Environmental | Social | Economic | Environmental | Social | |
Maximum | 80.00 | 80.00 | 80.00 | 80.00 | 80.00 | 80.00 |
Minimum | 10 | 10 | 10 | 10 | 10 | 10 |
Average | 30.44 | 35.56 | 34.01 | 30.99 | 35.22 | 33.80 |
Standard deviation | 24.36 | 23.78 | 23.02 | 23.88 | 23.55 | 22.98 |
Maximum-GSi | 22.34 | 19.54 | ||||
Calculated-GSi | 21.54 | 18.88 | ||||
Minimum-GSi | 7.26 | 6.32 |
WSPs | 2014 |
2016 |
2017 |
||||||||||
pH | T °C | EC (µS/cm) | TDS (mg/L) | pH | T °C | EC (µS/cm) | TDS (mg/L) | pH | T °C | EC (µS/cm) | TDS (mg/L) | DO (mg/L) | |
Inlet anaerobic pond | 7.33 | 26.80 | 891 | 438 | 7.98 | 26.70 | 704 | 350 | 6.86 | 28.90 | 952 | 626 | 0.15 |
Inlet facultative pond | 7.45 | 27.00 | 845 | 423 | 7.47 | 25.10 | 667 | 321 | 7.60 | 28.60 | 920 | 609 | 0.23 |
Outlet facultative pond | 7.57 | 26.90 | 815 | 410 | 7.69 | 25.60 | 600 | 297 | 7.66 | 30.03 | 890 | 582 | 0.55 |
Outlet maturation pond 6 | 7.87 | 26.50 | 795 | 391 | 7.85 | 26.10 | 601 | 299 | 8.11 | 31.10 | 860 | 560 | 0.95 |
TSE (mg/L) | 6.50–8.50 | 25 | 1500 | 3000 | 6.50–8.50 | 25 | 1500 | 3000 | 6.50–8.50 | 25 | 1500 | 3000 | 3.50 |
WSPs | 2014 |
2016 |
2017 |
||||||||||
Zn | Cr | S | Zn | Cr | S | Zn | Cr | S | Pb | Cd | Fe | Cu | |
Inlet anaerobic pond | 0.53 | 0.33 | 0.22 | 0.97 | 0.43 | 1.31 | 0.85 | 0.95 | 1.25 | 1.75 | 0.75 | 10.00 | 9.50 |
Inlet facultative pond | 0.38 | 0.27 | 0.18 | 0.60 | 0.39 | 0.71 | 0.65 | 0.75 | 0.75 | 0.55 | 0.35 | 5.25 | 6.55 |
Outlet facultative pond | 0.32 | 0.24 | 0.15 | 0.44 | 0.18 | 0.35 | 0.35 | 0.57 | 0.45 | 0.14 | 0.11 | 3.15 | 4.75 |
Outlet maturation pond 6 | 0.24 | 0.11 | 0.10 | 0.17 | 0.13 | 0.14 | 0.25 | 0.45 | 0.35 | 0.07 | 0.05 | 2.55 | 1.75 |
TSE (mg/L) | 1.00 | 1.00 | 0.50 | 1.00 | 1.00 | 0.50 | 1.00 | 1.00 | 0.50 | 0.10 | 0.10 | 5.00 | 2.00 |
Dimensions | Indicators | AFP-CW |
WSPs |
||
Weighting indicator (%) | Weight of dimensions (%) | Weighting indicator (%) | Weight of dimensions (%) | ||
Economic | Investment Costa | 52.16 | 10.18 | 78.43 | 14.55 |
Operation and Maintenance Costa | 0.58 | 0.15 | |||
Environmental | Energy consumptiona | 0.01 | 0.001 | ||
Land area requireda | 0.61 | 3.64 | |||
Pollutant removal efficiency costsa | 44.28 | 14.12 | |||
Water-bodies Eutrophicationa | 1.81 | 51.11 | 0.60 | 48.39 | |
Global Warminga | 0.02 | 2.93 | |||
Durabilityb | 75 | 80 | |||
Flexibilityb | 68 | 75 | |||
Reliabilityb | 75 | 85 | |||
Social | Employmentb | 0.53 | 0.12 | ||
Public acceptanceb | 85 | 55 | |||
Complexityb | 50 | 38.71 | 25 | 37.06 | |
Odour impactb | 25 | 70 | |||
Noise impactb | 20 | 10 | |||
Visual impacta | 20 | 40 | |||
Total | 518.00 | 100.00 | 540.00 | 100.00 |
S/No. | Sustainability | AFP-CW | WSPs | ||
1 | Economic | 1.05 | 0.04 | 2.12 | 0.11 |
2 | Environmental | 26.15 | 16.15 | 23.41 | 13.96 |
3 | Social | 14.94 | 5.35 | 13.74 | 4.81 |
CI (%) | 42.14 | 39.27 | |||
GSi (%) | 21.54 | 18.88 |
Scenario | AFP-CW Dimensional weight (%) |
WSPs Dimensional weight (%) |
||||
Economic | Environmental | Social | Economic | Environmental | Social | |
R | 10.18 | 51.11 | 38.71 | 14.55 | 48.39 | 37.06 |
S | 33.33 | 33.33 | 33.33 | 33.33 | 33.33 | 33.33 |
T1 | 50 | 25 | 25 | 50 | 25 | 25 |
T2 | 25 | 50 | 25 | 25 | 50 | 25 |
T3 | 25 | 25 | 50 | 25 | 25 | 50 |
U1 | 80 | 10 | 10 | 80 | 10 | 10 |
U2 | 10 | 80 | 10 | 10 | 80 | 10 |
U3 | 10 | 10 | 80 | 10 | 10 | 80 |
Dimensional weight (%) | AFP-CW |
WSPs |
||||
Economic | Environmental | Social | Economic | Environmental | Social | |
Maximum | 80.00 | 80.00 | 80.00 | 80.00 | 80.00 | 80.00 |
Minimum | 10 | 10 | 10 | 10 | 10 | 10 |
Average | 30.44 | 35.56 | 34.01 | 30.99 | 35.22 | 33.80 |
Standard deviation | 24.36 | 23.78 | 23.02 | 23.88 | 23.55 | 22.98 |
Maximum-GSi | 22.34 | 19.54 | ||||
Calculated-GSi | 21.54 | 18.88 | ||||
Minimum-GSi | 7.26 | 6.32 |