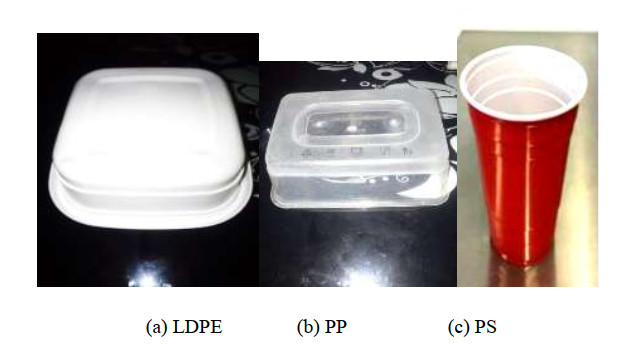
Citation: Andrew Wirnkor Verla, Christian Ebere Enyoh, Isiuku Beniah Obinna, Evelyn Ngozi Verla, Wang Qingyue, Md. Akhter Hossain Chowdhury, Emmanuel Chinedu Enyoh, Tanzin Chowdhury. Effect of macro-and micro-plastics in soil on growth of Juvenile Lime Tree (Citrus aurantium)[J]. AIMS Environmental Science, 2020, 7(6): 526-541. doi: 10.3934/environsci.2020033
[1] | Katharina Meixner, Mona Kubiczek, Ines Fritz . Microplastic in soil–current status in Europe with special focus on method tests with Austrian samples. AIMS Environmental Science, 2020, 7(2): 174-191. doi: 10.3934/environsci.2020011 |
[2] | B. Ravit, K. Cooper, G. Moreno, B. Buckley, I. Yang, A. Deshpande, S. Meola, D. Jones, A. Hsieh . Microplastics in urban New Jersey freshwaters: distribution, chemical identification, and biological affects. AIMS Environmental Science, 2017, 4(6): 809-826. doi: 10.3934/environsci.2017.6.809 |
[3] | B. Ravit, K. Cooper, B. Buckley, I. Yang, A. Deshpande . Organic compounds associated with microplastic pollutants in New Jersey, U.S.A. surface waters. AIMS Environmental Science, 2019, 6(6): 445-459. doi: 10.3934/environsci.2019.6.445 |
[4] | Gina M. Moreno, Keith R. Cooper . Morphometric effects of various weathered and virgin/pure microplastics on sac fry zebrafish (Danio rerio). AIMS Environmental Science, 2021, 8(3): 204-220. doi: 10.3934/environsci.2021014 |
[5] | Mahidin, Asri Gani, Saiful, Muhammad Irham, Wulan Windari, Erdiwansyah . An overview of the potential risks, sources, and analytical methods for microplastics in soil. AIMS Environmental Science, 2022, 9(2): 185-216. doi: 10.3934/environsci.2022013 |
[6] | Ndakara Ofudjaye Emmanuel, Ofuoko Ukaro Albert . Characterizing plant biomass and soil parameters under exotic trees within rainforest environment in southern Nigeria. AIMS Environmental Science, 2020, 7(6): 611-626. doi: 10.3934/environsci.2020039 |
[7] | Rozanna Dewi, Novi Sylvia, Zulnazri, Medyan Riza, Januar Parlaungan Siregar, Tezara Cionita, Budhi Santri Kusuma . Characterization of sago starch-based degradable plastic with agricultural waste cellulose fiber as filler. AIMS Environmental Science, 2024, 11(2): 304-323. doi: 10.3934/environsci.2024014 |
[8] | Judith S. Weis . Improving microplastic research. AIMS Environmental Science, 2019, 6(5): 326-340. doi: 10.3934/environsci.2019.5.326 |
[9] | Akekawat Vitheepradit, Taeng-On Prommi . Microplastics in surface water and tissue of white leg shrimp, Litopenaeus vannamei, in a cultured pond in Nakhon Pathom Province, Central Thailand. AIMS Environmental Science, 2023, 10(4): 478-503. doi: 10.3934/environsci.2023027 |
[10] | Abigail W. Porter, Sarah J. Wolfson, Lily. Young . Pharmaceutical transforming microbes from wastewater and natural environments can colonize microplastics. AIMS Environmental Science, 2020, 7(1): 99-116. doi: 10.3934/environsci.2020006 |
In the last one and half decades, the pollution and impact of plastics in the environment has been the major focus of study for many scientists globally. Studies have demonstrated that presence of macro- and micro- plastics in aquatic ecosystems and atmospheric compartments posing serious threat to aquatic organism, marine ecosystem and human health [1,2,3]. Over the years, soil has become a major sink for microplastics [4,5] and are also part of microplastics contamination cycle involving both atmosphere and aquatic ecosystems [6]. According to Horton AA et al. [7] the soil contains more plastic waste than the ocean. In 2017, an estimated 34.8 million metric tons of plastics ended up as waste in landfills globally [8].
More than half of previous research regarding the occurrence and fate of microplastics in the environment has been focused on aquatic ecosystem [9,10] while soil ecosystems especially agricultural soil has been less studied [1,3,5]. In agriculture, it is a common practice to use shredded vegetable matter and/or plastics (e.g. polyethylene) as mulch material to improve soil quality by retaining the moisture, discouraging weed growth and insulating the soil thus making it more beneficial to desired plant growth [11]. These practices are currently on the rise globally [12,13,14]. According to Brodhagen M et al. [13], the application of plastic mulch, improved plants and economic growth. However, the down side of this practice is that the plastic is left in the soil after harvest due to the difficulties in removing them and coupled with high cost of recycling [13,15]. Over the years the residual plastics accumulates resulting in large quantity of plastics in the soil [15,16]. Unfortunately, the rate of disintegration of these plastic residues in soil is currently unknown [17], therefore the accumulation is undesirable and could affect quality of soil, crop production [16,18] and potentially affect human health [1,3,6,8]. Due to UV radiation, mechanical abrasion, and interaction with fauna, macro plastics (>5 mm) in the soil become brittle and then fragments into smaller particles, then microplastics (MPs, 0.1μm to 5 mm) [19]. Apart from the application of mulch film, microplastics could enter agricultural soil from application of sludge and organic fertilizer on land, waste water irrigation, littering and surface runoff as well as deposition of airborne microplastics [1,4,5,20]. The long-term presence of plastics in agricultural soils could cause stunted plants growth due to the ability to uptake them and negatively affect biodiversity. Previous reports have shown that plants can accumulates micro and nano size plastics, through their cell wall and membrane [5,21,22] and could uptake toxic pollutants as well [1]. Microplastics have the ability to convey toxic pollutants with endocrine disrupting ability within soil-plant system, whether by adsorption or from additives contained in the plastic [1,3]. This has aroused the interest of environmental analytical scientists regarding the soil-plant system.
Literature reveals that there has been limited experimental research focusing on macroplastics and microplastics in soil-plant system. Enyoh et al. [1] in their review identified a knowledge gap and called for deliberate studies focusing on the effects of microplastics concentrations and type on plant. Qi et al. [14] already took the first steps towards filling the knowledge gaps by studying the effects of macro- and micro- plastics in presence and absence of earthworm in soil on wheat plant in a climate chamber. Following the study of Qi Y et al. [14], the current research is aimed to further fill knowledge gaps by testing the effects of macro- and micro- plastics of three types, low density polyethylene (LDPE), polypropylene (PP) and polystyrene (PS) in soil on the growth of juvenile lime tree (Citrus aurantium) under ambient field conditions.
Citrus fruits are highly cultivated in Nigeria. Currently, Nigeria is the 9th major global producer and largest in Africa [23]. As at 2008, Food and Agriculture Organization placed annual local production to be about 3.4 million metric tonnes from an estimated 3 million hectares of land [24]. Production has grown ever since at an average rate 3.21 % reaching 4.09 million metric tonnes in 2017 [25] due to increasing demand, mainly from the composition and many usefulness of its different parts. The pulp and peel of citrus fruits are being used as livestock feeds, produce citric acid and feed yeast among others. The peels also contain essential oils, a valuable material in the international market, the bark and leaves are well known to have phytochemicals that are of pharmacological importance. While the cultivation is seemingly rampant, to the best of our knowledge studies focusing on the impact of macro- and micro- plastics in terrestrial ecosystem and particularly agricultural soils in Nigeria is non-existent [26,27]. In Nigeria, current estimate revealed that 114.8 kt of plastics is in the environment as waste and it is predicted to reach 147.8 kt in 2023 [27]. Therefore, the study also provides first assessment of macro- and micro- plastics in agricultural soils on plant in Nigeria. Furthermore, the present study will encourage similar researches that will lead to a proper understanding of the plastic-soil-plant system.
A pot experiment was adopted to evaluate the effects of different types and sizes of plastic fragment on lime tree (Citrus aurantium) in a field condition. The soils used for the study were collected from a farmland in Orji, Owerri Imo State Nigeria (Lat. 5°30′56.778″ N, Lon. 7°2′59.574″ N and above sea level 123.40 m). A simple random sampling technique was adopted for sample collection, to spread sampling point objectively. Top soils (0–5 cm) were collected using a soil auger and transferred into black plastic bags. The sampling materials used were previously soaked and rinsed in 10% HNO3 overnight. The bags were tied tightly and transported to the laboratory for analysis. In the lab, soil samples were air-dried and debris removed. The soil was grounded and sieved with 2 mm mesh-sieve and kept for further use and analysis. The farmlands were used to grow the Citrus aurantium (nurseries) prior to the study. The nurseries collected from the farmland had an average height of 7 ± 2 cm and have been growing for more than a year. The soils collected were analyzed in triplicate for physicochemical characteristics as described previously [28,29]. Temperature was determined in situ using the soil gardeners thermometer while pH using Jenway 3510 pH meter. HANNA HI 8733 EC Meter was used in determining soil electrical conductivity in μS/cm. Moisture contents and soil organic matter were determined based on weight loss on ignition at 105 ℃ and 440 ℃ respectively. Particle size distribution (% sand, % silt and % clay) was determined using hydrometer. The soil colour was dark brown, which consisted of 51 ± 0.02 % clay, 13.6 ± 0.49 % sand and 4.6 ± 0.51% silt with an organic matter and moisture content of 4.21 ± 1.12 % and 18.23 ± 2.14 % respectively. The pH, temperature and electrical conductivity were 6.62 ± 0.04, 28.2 ± 1.34 ℃ and 371.62 ± 3.42 µS/cm respectively. The soil properties are consistent with tropical soils of this region [28,29]. The soil samples were then air-dried under room temperature, removed debris, crushed and sieved through a 2 mm steel sieve for homogeneity before use.
Three types of plastic were evaluated in this experiment: (1) low-density polyethylene (LDPE), (2) polypropylene (PP) and (3) polystyrene (PS) (the sources of these plastic types were from household and containers for personal care products, presented in Figure 1). These plastic types were identified based on their SPI (Society of Plastic Industry) codes [30]. LDPE had code number 4, with density of 0.92 g/cm3, tensile strength of 600–2300 psi, melting temperature of 98–115 ℃ and resistant to solvent below 60 ℃; PP had code number 5, with density: 0.90 g/cm3, tensile strength of 4500–5500 psi, melting temperature of 175 ℃ and resistant to solvent below 80 ℃ while PS had code number 6, with density of 1.05 g/cm3, tensile strength of 5000–7200 psi and melting temperature of 100 ℃.
The macroplastics (Ma) and microplastics (Mi) fragments used were obtained manually as described previously [14]. For Ma, the pieces of plastics were cut on a hard-wooden board using sharp blades and scissors and size of about 5cm were used. After cutting, some pieces of plastic were randomly collected from each sort. To obtain Mi (<5 mm), the remaining unused macroplastics were further cut into pieces and then was frozen and grounded into a powder using a high speed blender. After grinding, the resulting powder was sieved through 1 mm sieves, to obtain microplastics of homogenous sizes.
Three factors were taken into consideration while planning the experiment: single (LDPE/PP/PS), mixture (LDPE+PP/ LDPE+PS/ PP+PS/ LDPE+PP+PS) and sizes of plastic residues (Ma/ Mi) (Table 1). Furthermore, eleven control treatments without any plastic residues were also examined. Overall, the experiment consisted of eight treatments with 165 pots x 3 replicates making a total of 495 pots.
Days | Control | Treatment | |||||||||||||
LDPE | PP | PS | LDPE+PP | LDPE+PS | PP+PS | LDPE+PP+PS | |||||||||
0 | Control 1 | Ma | Mi | Ma | Mi | Ma | Mi | Ma | Mi | Ma | Mi | Ma | Mi | Ma | Mi |
10 | Control 2 | Ma | Mi | Ma | Mi | Ma | Mi | Ma | Mi | Ma | Mi | Ma | Mi | Ma | Mi |
30 | Control 3 | Ma | Mi | Ma | Mi | Ma | Mi | Ma | Mi | Ma | Mi | Ma | Mi | Ma | Mi |
60 | Control 4 | Ma | Mi | Ma | Mi | Ma | Mi | Ma | Mi | Ma | Mi | Ma | Mi | Ma | Mi |
90 | Control 5 | Ma | Mi | Ma | Mi | Ma | Mi | Ma | Mi | Ma | Mi | Ma | Mi | Ma | Mi |
120 | Control 6 | Ma | Mi | Ma | Mi | Ma | Mi | Ma | Mi | Ma | Mi | Ma | Mi | Ma | Mi |
150 | Control 7 | Ma | Mi | Ma | Mi | Ma | Mi | Ma | Mi | Ma | Mi | Ma | Mi | Ma | Mi |
180 | Control 8 | Ma | Mi | Ma | Mi | Ma | Mi | Ma | Mi | Ma | Mi | Ma | Mi | Ma | Mi |
210 | Control 9 | Ma | Mi | Ma | Mi | Ma | Mi | Ma | Mi | Ma | Mi | Ma | Mi | Ma | Mi |
240 | Control 10 | Ma | Mi | Ma | Mi | Ma | Mi | Ma | Mi | Ma | Mi | Ma | Mi | Ma | Mi |
270 | Control 11 | Ma | Mi | Ma | Mi | Ma | Mi | Ma | Mi | Ma | Mi | Ma | Mi | Ma | Mi |
Notes: Ma- Macroplastics; Mi- Microplastics. |
For the experimental set up, with slight modifications, we followed a procedure described previously [14]. For each pot (height = 7 cm, top diameter = 6 cm and bottom diameter = 4 cm), we added the sieved soil of weight 3000 g. Then accurately weighed plastic material (30 g) was mixed manually with water and the mixture poured in the pot containing the soil (except for the control which was free from plastic contamination) to make a treatment of 1 % w/w. The plastic mixture treatment also followed the same procedure except that the plastics (either Ma or Mi) added were 15 g + 15 g for two mixtures (also 1 % w/w for LDPE+PP, LDPE+PS and PP+PS) and 10 g + 10 g + 10 g for three mixtures treatment (1 % w/w for LDPE+PP+PS). Similar treatments have been applied in other studies [14,31,32]. After the set-up, moisture contents of the pots were unified to 18 % similar to the soil field capacity. All the pots were allowed to settle down for a period of one week before lime juvenile plants were transplanted from the field into pots. The cultivation of the plant was done under ambient field conditions (relative humidity: 72.70 ± 10.37 % and temperature: 29 ± 4.82 ℃. Both parameters were measured with CO2 gas analyzer AZ77535 as described by [33]. The pots were placed randomly (and position shifted once a month) and watered weekly with tap water and the soil moisture was kept at around 12% to 18% with respect to weight [14].
Plant heights were measured from the root to the tip of the plant using a tape rule. The number of branches and leaves were counted manually and recorded. Plants dry biomasses were recorded after drying at 70 ℃ (using DHG-9023A Oven by B. Bran Scientific and Instrument Company, England) to a constant weight. Leaf areas were measured using a plastic ruler as shown in Figure 2. The length (L cm) of the leaf was measured at the apex and width measured at the center (W cm). Leaf areas were then calculated as the product of the length and the width (L* W) in cm2. The growth parameters were measured for all treatments at 30 days interval for 270 days (between January 2019 and October 2019, covering both the dry and rainy period).
Statistical data analyses were performed using IBM SPSS Statistics 20. Mean values from replicate observations were recorded for each plant and were expressed as mean ± standard deviation. Line plots were made for 60,120,180,240 and 270 days for clearer view of trend while box-plot was created to summarize data obtained over 270 days. One-way analysis of variance (ANOVA) was carried out to compare the different treatment and differences were considered significant at P < 0.05. Chemometric models such as relative growth rate (RGR), tolerance index (TI) and % inhibition were employed to assess the effects of treatment on vegetative growth of the juvenile lime tree. RGR value is an important index for estimating growth trends of plants exposed to the different treatment groups. It was proposed by Fisher in 1921 [34,35] and computed using Eq 1, where: ln(m1): logarithm of the final dry mass (g); ln(m0): logarithm of the initial dry mass (g); t0: initial time (d); t1: final time (d).
RGR=(In(m1)−In(m0)╱t1−t0)∗1000 | (1) |
The tolerance index (TI) was proposed by [36]. In the context of this study, it provides information regarding the tolerance of the plant to plastic contamination of the soil. It was calculated using Eq 2 as the ratio of growth rate of the plant in the plastic treated soil to growth rate of the plant in the control soil [34]. In the equation, RGR is the growth rate of the plant in the solution contaminated while RGRc is the growth rate of the plant in the control soil, without treatment. The % inhibition (Eq 3) provides information on the extent by which the contaminations of plastic in the soil inhibit/reduce growth of the plant with respect to the control. The variables for % inhibition is same as the ones in Eq 2.
TI=(RGR╱RGRc)∗100 | (2) |
%Inhibition=1−(RGR╱RGRc) | (3) |
The effects of different treatment for both macroplastics and microplastics in comparison to control are presented in Figure 3. Over 270 days, the effect trend for macroplastics on plant height was; LDPE+PP+PS (10.21 ± 5.36 cm) > LDPE+PS (8.58 ± 5.40 cm) > Control (8.42 ± 5.19 cm) > PS+PP (8.36 ± 5.64 cm) > PP (7.61 ± 4.26 cm) > PS (7.29 ± 4.33 cm) > LDPE+PP (7.20 ± 5.15 cm) > LDPE (7.17 ± 4.44 cm) while for microplastics the effect trend was; LDPE+PP+PS (10.45 ± 6.00 cm) > Control (8.42 ± 5.19 cm) > PS+PP (8.20 ± 5.33 cm) > PP (7.70 ± 4.44 cm) > LDPE+PP (6.94 ± 4.44 cm) > LDPE (6.64 ± 5.71 cm) > PS (6.59 ± 3.46 cm) > LDPE+PS (6.18 ± 4.22 cm) respectively. This therefore showed that only Ma-LDPE+PP+PS, Ma-LDPE+PS and Mi-LDPE+PP+PS increased the height of the plant than the control over 270 days. However, all treatments assessed by One-way ANOVA showed significant differences (P < 0.05) from the control (Figure 4). Between the two plastic sizes, there was also a significant difference (P < 0.05), with addition of microplastics having greater negative effects (reducing) on plant height than the addition of macroplastics (Figure 4).
The effects of the different treatment and plastic sizes on number of branches are presented in Figure 5. Similar trend was generally observed for both size group of the plastic. At day 60 and 120 all treatment showed equal number of branches with control ( = 2 and 3 respectively). Over the entire study period, Ma-LDPE+PP+PS (3.72 ± 2.33) and Mi-LDPE+PP+PS (3.63 ± 2.15) had the highest number of branches while Ma/Mi-LDPE (3.00 ± 1.79) had lowest respectively. All treatment showed no significant differences (P > 0.05, Figure 6) from the control (3.55 ± 2.11) over the study period.
The effects of the different treatment and plastic sizes on number of leaves are presented in Figure 7. Over the entire study period (270 days), the highest and lowest number of leaves was observed for LDPE+PP+PS treatment (Ma: 28.73 ± 25.06 > Mi: 28.18 ± 25.06) and PS treatment (Ma: 22.18 ± 18.72 < Mi: 22.73 ± 19.48) (Figure 8). The former only had higher number of leaves than the control (28.27 ± 24.23), but not significantly different (P > 0.05, Figure 8). However, comparing plastic size effects (Figure 7), microplastics treatment appeared to have stronger inhibition on the number of leaves, but they also showed no significant differences (P > 0.05) (Figure 8).
The leaf areas of the different treatment groups are presented in Figure 9. In size Ma, except for treatment LDPE+PP+PS (P < 0.05) and LDPE+PP (P < 0.05), all other treatment had no significant difference from the control (P > 0.05). Furthermore, only Ma-PS (19.82 cm2) and PP+PS (19.24 cm2) had lower leaf areas than the control (20.15 cm2). In size group Mi, all treatment (except for PP, PS, LDPE+PS and PP+PS) significantly reduced the leaf areas compared to macro sizes. The effect of microplastics showed significant differences from macroplastics, for LDPE, LDPE+PP and LDPE+PP+PS treatments. The effect trend over the entire period of study was LDPE+PP > LDPE+PS > PP+PS > PP > PS > LDPE > LDPE+PP+PS (Figure 9).
The trend for total plant biomass is presented in Figure 10. The TPB of the plant increased with time from 1.40 g from the initial day to as high as 9.22 g at the 270th day. Overall, for macroplastics, the control (5.00 ± 3.10 g) had higher biomass than all treatments except for LDPE+PP+PS (5.43 ± 2.39 g) while for microplastics, all treatments had lower biomass than the control. However, all treatments for both macroplastics and microplastics showed significant differences from the control (P < 0.05) also between the size categories (P < 0.05).
The relative growth rate of C. aurantium to the different treatment is presented in Table 2. The control had a better growth rate (10.29 ± 2.65) for all treatments except for Ma-LDPE+PP+PS. The lowest growth rate of the plant was observed for LDPE treatment. RGR of LDPE, PP+PS and LDPE+PS treatments for both plastic sizes were significantly different from the control (P < 0.05), while other treatment groups showed no significant differences from the control (P > 0.05).
Treatments | |||||||
LDPE | PP | PS | LDPE+PP | LDPE+PS | PP+PS | LDPE+PP+PS | |
Relative growth rate (RGR) | |||||||
Control | 10.29 ± 2.65a | 10.29 ± 2.65a | 10.29 ± 2.65a | 10.29 ± 2.65a | 10.29 ± 2.65a | 10.29 ± 2.65a | 10.29 ± 2.65a |
Macroplastics | 7.62 ± 2.22b | 9.02 ± 2.29aa | 9.23 ± 2.34a | 9.78 ± 2.75a | 9.68 ± 2.95bc | 9.41 ± 1.54de | 10.97 ± 2.39a |
Microplastics | 7.60 ± 2.19b | 8.91 ± 2.26ab | 9.14 ± 2.32a | 9.73 ± 2.76a | 9.61 ± 2.94bc | 9.23 ± 1.71ef | 10.13 ± 2.25a |
% Inhibition | |||||||
Macroplastics | 0.26a | 0.12a | 0.10a | 0.05a | 0.06a | 0.09a | -0.07a |
Microplastics | 0.26a | 0.13a | 0.11a | 0.05a | 0.07a | 0.10a | 0.02b |
Tolerance index (%) | |||||||
Macroplastics | 74.05a | 87.66a | 89.70a | 95.04a | 94.07a | 91.45a | 106.61a |
Microplastics | 73.85a | 86.59a | 88.82a | 94.56a | 93.39a | 89.69a | 98.45b |
*Values in column with the same alphabet are not significantly different at 5% level of probability |
Similar to relative growth rate, the highest inhibition (Table 2) was observed for the LDPE treatment (0.26) while other treatments were in the order of PP > PS > LDPE+PS > LDPE+PP > PP+PS > LDPE+PP+PS. Ma-LDPE+PP+PS had a negative % inhibition due its RGR being higher than that of control and was significantly different from other treatment (P < 0.05).
C. aurantium generally showed high tolerance to the different treatment groups (Table 2). For macroplastics, the tolerance index ranged from 74.05 % for LDPE to 106.6 % for LDPE+PP+PS while for microplastics group, the tolerance index ranged from 73.85 % for LDPE to 98.45 % for LDPE+PP+PS. Overall, high tolerance to macroplastics than microplastics contamination was shown by the plant.
The growth of the lime tree was not severely affected by macroplastic and microplastic exposure in this study when in comparison with the control. Despite the observed toxicity symptoms as the decrease in total plant biomass, there was no unfavorable impact on the relative growth rate (RGR). In fact, there was better growth stimulation in one of the treatments (Ma-LDPE+PP+PS) than the control. This growth could also be due to the possibility of the plastic treatments improving soil biophysical properties such as bulk density, soil agammaegation and water holding capacity [32,37] and other factors such as soil animals such as earthworms. Recent studies have suggested that earthworm presence in soil could reduce the deterioration made by plastic residues on growing plants [3,14]. It was explained further that the particles in the soil are eaten by earthworm [37], although unfavorable for the earthworm, but the endeavor will reduce plastic quantity and in turn may improve soil condition. The result from the study also showed that LDPE have more negative effects on soil-plant system than PP and PS. Similar studies elsewhere have made similar observation for LDPE having negative impact on growth of plant under differing treatment conditions [14,39,40]. Qi et al. [14] mixed 1% concentration of LDPE residues (macro- and micro- plastics) in sandy soil and assessed the impact on wheat growth in a pot study. They reported that both above and below ground parts of the plant were affected during both vegetative and reproductive growth [14]. Dong et al. [40] used six film (made of LDPE) residue gradient density (0,250,500, 1000, 1500, 2000 kg hm-2) to find the reason for decline in cotton yield in northern Xinjiang, China. They found that cumulative LDPE residue negatively affected cotton yield after 121 years and 157 years of mulching with decrease in yield reaching 60.3 % [40]. However, in contrast, an improvement of soil microenvironment and fertility was observed due to accumulated LDPE by Tao Z et al. [41] using horse bean mixed with high molecular weights (>2000) of LDPE powder and using corn [12]. These studies however were conducted using treatments that are different from the study, thereby suggesting that the effect could be based on quantity and plant type. For example, microfibers added at concentrations of 0.05% to 0.40% in soil had more effects on soil properties compared to beads added at concentrations from 0.25% to 2.00% [31]. However, between PP and PS, PP generally showed stronger effects on the vegetative growth of C. aurantium (Table 2). The effects of these plastic types are varied based on exposure concentrations, plastic types, shape, and size. PP particles at 7 % and 28 % concentration added to soil was reported to show positive effects on soil microbial community [42] while at smaller concentrations (0.05–0.4 %) PS was observed to show negative effects [3,31,43]. Both macroplastics and microplastics treatment for LDPE had the highest % inhibition and lowest growth rate while plastic mixture of LDPE, PP and PS had higher growth rate than the control (with no plastics) (Table 2). Thus, in order to have a comprehensive understanding of why LDPE had this effect, further studies need to examine the chemical composition and release from LDPE compared to other studied plastics.
According to this study, macro- or micro- sizes of plastic residues showed slightly differing effects on lime tree growth and microplastics showed more negative effects than macroplastics. Similar observation was also made in previous study [14]. The tolerance of the plant to the different treatments was evaluated using the tolerance index (TI). Generally, the plant showed high tolerance (>70 %) to the different treatments. However, the lowest and highest tolerance was observed for LDPE (Ma- 74.05 % > Mi- 73.85 %) and LDPE, PP and PS mixtures (Ma- 106.6 % > Mi- 98.45 %). For the doubts at present, the underlying reason why the lime tree had high tolerance to the treatments has not been traced. It is hypothesized that the presence of micro- and macro- plastics in the soil altered the soil properties and structure, to conditions favoring the lime tree growth.
Based on the findings of this study, it can be concluded that macro- and micro- plastic residues of low density polyethylene, polypropylene and polystyrene in soils could have positive and negative effects on both vegetative growth of juvenile lime tree. However, plastic mixture of LDPE, PP and PS in soil showed some positive effects on vegetative growth of juvenile lime tree while LDPE had the strongest negative effect on the growth. C. aurantium generally showed high tolerance to plastic contamination. Without doubt, more research is urgently needed in order to fully understand the effects of macroplastics and microplastics in soil-plant system, given that macro- and micro- plastics as emerging pollutants in our environments.
The authors are grateful to farm owners who allowed the collection of soil and juvenile lime tree from their respective farms.
The fund for this project was partly provided by Group Research in Analytical Chemistry, Environment and Climate Change, Imo State University, Owerri, Nigeria with no. GRACE & CC/MP/2019/05.
The authors declare no conflict of interest.
[1] | Enyoh CE, Verla AW, Verla EN (2019) Uptake of microplastics by plant: a reason to worry or to be happy. World Sci News 131: 256-267. |
[2] | Enyoh CE, Shafea L, Verla AW, et al. (2020) Microplastics Exposure Routes and Toxicity Studies to Ecosystems: An Overview. Environ Anal Health Tox 35: 1–10. |
[3] | Verla AW, Enyoh CE, Verla EN, et al. (2019) Microplastic-Toxic Chemical Interaction: a Review Study on Quantified Levels, Mechanism and Implications. Springer Nature App Sci 1: 1400. |
[4] | Bläsing M, Amelung W (2018) Plastics in soil: analytical methods and possible sources. Sci Total Environ 612,422–435 |
[5] | Zhu F, Changyin Z, Chao W, et al. (2019) Occurrence and Ecological Impacts of Microplastics in Soil Systems: A Review. Bull Environ Contamin Tox |
[6] | Enyoh CE, Verla AW, Verla EN, et al. (2019) Airborne Microplastics: a Review Study on Method for Analysis, Occurrence, Movement and Risks. Environ Mon Assess191: 668. |
[7] | Horton AA, Walton A, Spurgeon DJ, et al. (2017) Microplastics in freshwater and terrestrial environments: evaluating the current understanding to identify the knowledge gaps and future research priorities. Sci Total Environ 586: 127–141 |
[8] | Verla AW, Enyoh CE, Verla EN (2019) Microplastics, an emerging concern: a review of analytical techniques for detecting and quantifying microplatics. Anal Method Environ Chem J 2 15–32. |
[9] | Cole M, Lindeque P, Halsband C, et al. (2011) Microplastics as contaminants in the marine environment: a review. Mar Pollut Bull 62: 2588–2597. |
[10] | Eerkes-Medrano D, Thompson RC, Aldridge DC (2015) Microplastics in freshwater systems: a review of the emerging threats, identification of knowledge gaps and prioritisation of research needs. Water Res 75: 63–82. |
[11] | Ekebafe L, Ogbeifun D, Okieimen F (2011) Polymer applications in agriculture. Biokemistri 23: 1–9. |
[12] | Zhang Z, Luo X, Fan Y et al. (2015) Cumulative effects of powders of degraded PE mulching-films on chemical properties of soil. Environ Sci Technol 38: 115–119. |
[13] | Brodhagen M, Goldberger JR, Hayes DG et al. (2017) Policy considerations for limiting unintended residual plastic in agricultural soils. Environ Sci Pol 69: 81–84. |
[14] | Qi Y, Yang X, Pelaez AM, et al. (2018) Macro-and micro-plastics in soil-plant system: effects of plastic mulch film residues on wheat (Triticum aestivum) growth. Sci Total Environ 645: 1048–1056 |
[15] | Steinmetz Z, Wollmann C, Schaefer M, et al. (2016) Plastic mulching in agriculture. Trading short-term agronomic benefits for long term soil degradation? Sci. Total Environ. 550,690–705. |
[16] | Liu E, He W, Yan C (2014) 'White revolution' to 'white pollution'—agricultural plastic film mulch in China. Environ Res Lett 9: 091001. |
[17] | Rillig MC (2012) Microplastic in terrestrial ecosystems and the soil? Environ Sci Technol 46: 6453–6454 |
[18] | Jiang XJ, Liu W, Wang E, et al. (2017) Residual plastic mulch fragments effects on soil physical properties and water flow behavior in the Minqin oasis, northwestern China. Soil Tillage Res 166: 100–107. |
[19] | Wang Q, Enyoh CE, Chowdhury T, et al. (2020) Analytical Techniques, Occurrence and Health Effects of Micro and Nano Plastics Deposited in Street Dust. Int J Environ Anal Chem |
[20] | Enyoh CE, Verla AW (2019) We are breathing plastic; don't just look down, look up. Presented at the 3rd IMSU world environment day international conference. https://doi.org/10.13140/RG.2.2.21027.91680 |
[21] | Bandmann V, Müller JD, Köhler T, et al. (2012) Uptake of fluorescent nano beads into BY2-cells involves clathrin dependent and clathrin-independent endocytosis. FEBS Lett 586: 3626–3632. |
[22] | Li L, Zhou Q, Yin N et al. (2019) Uptake and accumulation of microplastics in an edible plant. Chinese Sci Bull 64: 928–934. |
[23] | Choji F (2019) How to Start Orange and Lemon Farming in Nigeria. https://www.wealthresult.com/agriculture/orange-lemon-farming-nigeria. Assessed 20/11/19 |
[24] | FAO (2017) Citrus Fruit - Fresh and Processed Statistical Bulletin 2016. Market and Policy Analysis of Raw Materials, Horticulture and Tropical (RAMHOT) Products. Team Trade and Markets Division I8092EN/1/11.17. http://www.fao.org/3/a-i8092e.pdf Assessed 20/11/2019. |
[25] | Konema (2018) Nigeria - Citrus fruit production quantity. Assessed 21/11/2019. https://knoema.com/atlas/Nigeria/topics/Agriculture/Crops-Production-Quantity-tonnes/Citrus-fruit-production. |
[26] | Enyoh CE (2019) Microplastic pollution in Nigeria: a call for concern. 26th meeting of the Imo State chapter of the Chemical Society of Nigeria (CSN) held at Imo State University, Nigeria. https://doi.org/10.13140/RG.2.2.21027.9190 |
[27] | Verla AW, Enyoh CE, Verla EN (2019) The importance of microplastics pollution studies in water and soil of Nigeria ecosystems. Anal Meth Environ Chem J 2: 89–96. |
[28] | Enyoh CE, Ihionu EA, Verla AW, et al. (2017) Physicochemical Properties of Palm Oil and Soil from Ihube Community, Okigwe, Imo State, Nigeria. Intl Lett Nat Sci 62: 35–49. |
[29] | Verla EN, Verla AW, Enyoh CE (2017) Pollution Assessment Models of Soils In Portharcourt City, Rivers State, Nigeria. World News Nat Sci 12: 1–23. |
[30] | Ryedale (2016) Different types of plastics and their classification. Available: http://www.ryedale.gov.uk/attachments/article/690/Different_plastic_polymer_types.pdf |
[31] | Machado AAS, Lau CW, Till J, et al. (2018) Impacts of microplastics on the soil biophysical environment. Environ Sci Techn 52: 9656–9665. |
[32] | Enyoh CE, Verla AW, Verla EN, et al. (2020) Effect of Macro- and Micro- Plastics in Soil on Quantitative Phytochemicals in Different Part of Juvenile Lime Tree (Citrus aurantium). Int J Environ Res 2020: 705–726. |
[33] | Verla AW, Enyoh CE, Ngozi VE (2018) Evaluation of Anthropogenic Carbon Dioxide (CO2) Concentrations along River Nworie, Imo State, Nigeria. Environ Pollut Cli Chan 2: 159. |
[34] | Isiuku BO, Enyoh CE (2019) Water pollution by heavy metal and organic pollutants: Brief review of sources, effects and progress on remediation with aquatic plants. Anal Meth Environ Chem J 2: 5–38. |
[35] | Fisher RA (1921) Some remarks on the methods formulated in a recent article on 'The quantitative analysis of plant growth. Ann Appl Biol 7: 367–372. |
[36] | Wilkins DA (1978) The measurement of tolerance to edaphic factors by means of root growth. New Phytol 80: 623–633. |
[37] | Wan Y, Wu C, Xue Q, et al. (2019) Effects of plastic contamination on water evaporation and desiccation cracking in soil. Sci Total Environ 654: 576–582 |
[38] | Derek B (2019) Microplastics in the soil point to another potential ecosystem collapse. https://bigthink.com/surprising-science/microplastics-soil. Assessed 19/11/2019 |
[39] | Parvathy PC, Jyothi AN, John KS, et al. (2014) Cassava starch based superabsorbent polymer as soil conditioner: impact on soil physico-chemical and biological properties and plant growth. Clean Soil Air Water 42: 1610–1617. |
[40] | Dong HD, Liu T, Han ZQ, et al. (2015) Determining time limits of continuous film mulching and examining residual effects on cotton yield and soil properties. J Environ Biol 36: 677–684. |
[41] | Tao Z, Cao X, Luo X, (2012) Responses of three enzyme activities to lower molecular weight polyethylene added in pot-cultured horse bean soil. Chinese J Soil Sci 43: 1104–1110 |
[42] | Liu M, Lu S, Song Y, et al. (2018) Microplastic and mesoplastic pollution in farmland soils in suburbs of Shanghai, China. Environ Pollut 242: 855–862. |
[43] | Artham T, Sudhakar M, Venkatesan R, et al. (2009) Biofouling and stability of synthetic polymers in sea water. Int Biodeterior Biodegr 63: 884–890. |
1. | Christian Ebere Enyoh, Andrew Wirnkor Verla, Franklyn Okechukwu Ohiagu, Emmanuel Chinedu Enyoh, Progress and future perspectives of microplastic research in Nigeria, 2021, 0306-7319, 1, 10.1080/03067319.2021.1887161 | |
2. | Gustavo Führ Hartmann, Felipe Klein Ricachenevsky, Neidiquele Maria Silveira, Alice Pita-Barbosa, Phytotoxic effects of plastic pollution in crops: what is the size of the problem?, 2022, 292, 02697491, 118420, 10.1016/j.envpol.2021.118420 | |
3. | Periyasamy Dhevagi, Ramesh Poornima, Raveendra Gnana Keerthi Sahasa, Ambikapathi Ramya, Sangilidurai Karthika, Karuppusamy Sivasubramanian, The crux of microplastics in soil - a review, 2022, 0306-7319, 1, 10.1080/03067319.2022.2148528 | |
4. | Leila Shafea, Julia Yap, Nicolas Beriot, Vincent J. M. N. L. Felde, Elvis D. Okoffo, Christian Ebere Enyoh, Stephan Peth, Microplastics in agroecosystems: A review of effects on soil biota and key soil functions, 2023, 186, 1436-8730, 5, 10.1002/jpln.202200136 | |
5. | Reza Pashaei, Steven Arthur Loiselle, Gemma Leone, Gabriella Tamasi, Reda Dzingelevičienė, Tomasz Kowalkowski, Mortaza Gholizadeh, Marco Consumi, Sajjad Abbasi, Viktorija Sabaliauskaitė, Boguslaw Buszewski, Determination of nano and microplastic particles in hypersaline lakes by multiple methods, 2021, 193, 0167-6369, 10.1007/s10661-021-09470-8 | |
6. | Mandeep Kaur, Ming Xu, Lin Wang, Cyto–Genotoxic Effect Causing Potential of Polystyrene Micro-Plastics in Terrestrial Plants, 2022, 12, 2079-4991, 2024, 10.3390/nano12122024 | |
7. | Aziz-Ur-Rahim Bacha, Iqra Nabi, Muhammad Zaheer, Wenbiao Jin, Lei Yang, Biodegradation of macro- and micro-plastics in environment: A review on mechanism, toxicity, and future perspectives, 2023, 858, 00489697, 160108, 10.1016/j.scitotenv.2022.160108 | |
8. | Claudia Campanale, Silvia Galafassi, Ilaria Savino, Carmine Massarelli, Valeria Ancona, Pietro Volta, Vito Felice Uricchio, Microplastics pollution in the terrestrial environments: Poorly known diffuse sources and implications for plants, 2022, 805, 00489697, 150431, 10.1016/j.scitotenv.2021.150431 | |
9. | Christian Ebere Enyoh, Qingyue Wang, Adsorption of ciprofloxacin from aqueous solution by plastic-based adsorbents: a review, 2022, 0306-7319, 1, 10.1080/03067319.2022.2106432 | |
10. | Tapati Roy, Thuhin K. Dey, Mamun Jamal, Microplastic/nanoplastic toxicity in plants: an imminent concern, 2023, 195, 0167-6369, 10.1007/s10661-022-10654-z | |
11. | Kauê Pelegrini, Talita Carneiro Brandão Pereira, Thuany Garcia Maraschin, Lilian De Souza Teodoro, Nara Regina De Souza Basso, Griselda Ligia Barrera De Galland, Rosane Angelica Ligabue, Mauricio Reis Bogo, Micro- and nanoplastic toxicity: A review on size, type, source, and test-organism implications, 2023, 878, 00489697, 162954, 10.1016/j.scitotenv.2023.162954 | |
12. | John Bethanis, Evangelia E. Golia, Micro- and nano-plastics in agricultural soils: A critical meta-analysis of their impact on plant growth, nutrition, metal accumulation in plant tissues and crop yield, 2024, 194, 09291393, 105202, 10.1016/j.apsoil.2023.105202 | |
13. | Christian Ebere Enyoh, Qingyue Wang, Adsorption and toxicity characteristics of ciprofloxacin on differently prepared polyethylene terephthalate microplastics from both experimental and theoretical perspectives, 2023, 53, 22147144, 103909, 10.1016/j.jwpe.2023.103909 | |
14. | Krishan K. Verma, Xiu-Peng Song, Lin Xu, Hai-Rong Huang, Qiang Liang, Chandra Shekhar Seth, Yang-Rui Li, Nano-microplastic and agro-ecosystems: a mini-review, 2023, 14, 1664-462X, 10.3389/fpls.2023.1283852 | |
15. | Yanyan Wang, Qingyue Wang, Weiqian Wang, Fenwu Liu, Shangrong Wu, Migration of fluoranthene, phenanthrene, and pyrene in soil environment during the growth of Brassica rapa subsp. chinensis, 2024, 110, 13826689, 104535, 10.1016/j.etap.2024.104535 | |
16. | Jacqueline Zanin Lima, Raul Cassaro, Allan Pretti Ogura, Marilda Mendonça Guazzelli Ramos Vianna, A systematic review of the effects of microplastics and nanoplastics on the soil-plant system, 2023, 38, 23525509, 266, 10.1016/j.spc.2023.04.010 | |
17. | Rogers Wainkwa Chia, Jin-Yong Lee, Minwook Lee, Gyu-Sang Lee, Chan-Duck Jeong, Role of soil microplastic pollution in climate change, 2023, 887, 00489697, 164112, 10.1016/j.scitotenv.2023.164112 | |
18. | Lei Mai, Lian-Jun Bao, Charles S. Wong, Eddy Y. Zeng, 2024, 9780443153327, 229, 10.1016/B978-0-443-15332-7.00012-0 | |
19. | Zahra Dehghanian, Behnam Asgari Lajayer, Zahra Biglari Quchan Atigh, Shahnoush Nayeri, Mohammad Ahmadabadi, Leila Taghipour, Venkatramanan Senapathi, Tess Astatkie, G.W. Price, Micro (nano) plastics uptake, toxicity and detoxification in plants: Challenges and prospects, 2023, 268, 01476513, 115676, 10.1016/j.ecoenv.2023.115676 | |
20. | Pawan Kumar Rose, Sangita Yadav, Navish Kataria, Kuan Shiong Khoo, Microplastics and nanoplastics in the terrestrial food chain: Uptake, translocation, trophic transfer, ecotoxicology, and human health risk, 2023, 167, 01659936, 117249, 10.1016/j.trac.2023.117249 | |
21. | Hira Azhar, Noreen Khalid, Zonaira Qaiser, Muhammad Aqeel, Wajiha Sarfraz, Ujala Ejaz, Zarrin Fatima Rizvi, Shujaul Mulk Khan, Ali Noman, 2025, 9780443298042, 129, 10.1016/B978-0-443-29804-2.00005-6 | |
22. | Riti Thapar Kapoor, Mohd Rafatullah, Leveraging biotechnological approaches for the degradation of plastics: A sustainable tool for plastic waste management and environment protection, 2025, 69, 22147144, 106736, 10.1016/j.jwpe.2024.106736 |
Days | Control | Treatment | |||||||||||||
LDPE | PP | PS | LDPE+PP | LDPE+PS | PP+PS | LDPE+PP+PS | |||||||||
0 | Control 1 | Ma | Mi | Ma | Mi | Ma | Mi | Ma | Mi | Ma | Mi | Ma | Mi | Ma | Mi |
10 | Control 2 | Ma | Mi | Ma | Mi | Ma | Mi | Ma | Mi | Ma | Mi | Ma | Mi | Ma | Mi |
30 | Control 3 | Ma | Mi | Ma | Mi | Ma | Mi | Ma | Mi | Ma | Mi | Ma | Mi | Ma | Mi |
60 | Control 4 | Ma | Mi | Ma | Mi | Ma | Mi | Ma | Mi | Ma | Mi | Ma | Mi | Ma | Mi |
90 | Control 5 | Ma | Mi | Ma | Mi | Ma | Mi | Ma | Mi | Ma | Mi | Ma | Mi | Ma | Mi |
120 | Control 6 | Ma | Mi | Ma | Mi | Ma | Mi | Ma | Mi | Ma | Mi | Ma | Mi | Ma | Mi |
150 | Control 7 | Ma | Mi | Ma | Mi | Ma | Mi | Ma | Mi | Ma | Mi | Ma | Mi | Ma | Mi |
180 | Control 8 | Ma | Mi | Ma | Mi | Ma | Mi | Ma | Mi | Ma | Mi | Ma | Mi | Ma | Mi |
210 | Control 9 | Ma | Mi | Ma | Mi | Ma | Mi | Ma | Mi | Ma | Mi | Ma | Mi | Ma | Mi |
240 | Control 10 | Ma | Mi | Ma | Mi | Ma | Mi | Ma | Mi | Ma | Mi | Ma | Mi | Ma | Mi |
270 | Control 11 | Ma | Mi | Ma | Mi | Ma | Mi | Ma | Mi | Ma | Mi | Ma | Mi | Ma | Mi |
Notes: Ma- Macroplastics; Mi- Microplastics. |
Treatments | |||||||
LDPE | PP | PS | LDPE+PP | LDPE+PS | PP+PS | LDPE+PP+PS | |
Relative growth rate (RGR) | |||||||
Control | 10.29 ± 2.65a | 10.29 ± 2.65a | 10.29 ± 2.65a | 10.29 ± 2.65a | 10.29 ± 2.65a | 10.29 ± 2.65a | 10.29 ± 2.65a |
Macroplastics | 7.62 ± 2.22b | 9.02 ± 2.29aa | 9.23 ± 2.34a | 9.78 ± 2.75a | 9.68 ± 2.95bc | 9.41 ± 1.54de | 10.97 ± 2.39a |
Microplastics | 7.60 ± 2.19b | 8.91 ± 2.26ab | 9.14 ± 2.32a | 9.73 ± 2.76a | 9.61 ± 2.94bc | 9.23 ± 1.71ef | 10.13 ± 2.25a |
% Inhibition | |||||||
Macroplastics | 0.26a | 0.12a | 0.10a | 0.05a | 0.06a | 0.09a | -0.07a |
Microplastics | 0.26a | 0.13a | 0.11a | 0.05a | 0.07a | 0.10a | 0.02b |
Tolerance index (%) | |||||||
Macroplastics | 74.05a | 87.66a | 89.70a | 95.04a | 94.07a | 91.45a | 106.61a |
Microplastics | 73.85a | 86.59a | 88.82a | 94.56a | 93.39a | 89.69a | 98.45b |
*Values in column with the same alphabet are not significantly different at 5% level of probability |
Days | Control | Treatment | |||||||||||||
LDPE | PP | PS | LDPE+PP | LDPE+PS | PP+PS | LDPE+PP+PS | |||||||||
0 | Control 1 | Ma | Mi | Ma | Mi | Ma | Mi | Ma | Mi | Ma | Mi | Ma | Mi | Ma | Mi |
10 | Control 2 | Ma | Mi | Ma | Mi | Ma | Mi | Ma | Mi | Ma | Mi | Ma | Mi | Ma | Mi |
30 | Control 3 | Ma | Mi | Ma | Mi | Ma | Mi | Ma | Mi | Ma | Mi | Ma | Mi | Ma | Mi |
60 | Control 4 | Ma | Mi | Ma | Mi | Ma | Mi | Ma | Mi | Ma | Mi | Ma | Mi | Ma | Mi |
90 | Control 5 | Ma | Mi | Ma | Mi | Ma | Mi | Ma | Mi | Ma | Mi | Ma | Mi | Ma | Mi |
120 | Control 6 | Ma | Mi | Ma | Mi | Ma | Mi | Ma | Mi | Ma | Mi | Ma | Mi | Ma | Mi |
150 | Control 7 | Ma | Mi | Ma | Mi | Ma | Mi | Ma | Mi | Ma | Mi | Ma | Mi | Ma | Mi |
180 | Control 8 | Ma | Mi | Ma | Mi | Ma | Mi | Ma | Mi | Ma | Mi | Ma | Mi | Ma | Mi |
210 | Control 9 | Ma | Mi | Ma | Mi | Ma | Mi | Ma | Mi | Ma | Mi | Ma | Mi | Ma | Mi |
240 | Control 10 | Ma | Mi | Ma | Mi | Ma | Mi | Ma | Mi | Ma | Mi | Ma | Mi | Ma | Mi |
270 | Control 11 | Ma | Mi | Ma | Mi | Ma | Mi | Ma | Mi | Ma | Mi | Ma | Mi | Ma | Mi |
Notes: Ma- Macroplastics; Mi- Microplastics. |
Treatments | |||||||
LDPE | PP | PS | LDPE+PP | LDPE+PS | PP+PS | LDPE+PP+PS | |
Relative growth rate (RGR) | |||||||
Control | 10.29 ± 2.65a | 10.29 ± 2.65a | 10.29 ± 2.65a | 10.29 ± 2.65a | 10.29 ± 2.65a | 10.29 ± 2.65a | 10.29 ± 2.65a |
Macroplastics | 7.62 ± 2.22b | 9.02 ± 2.29aa | 9.23 ± 2.34a | 9.78 ± 2.75a | 9.68 ± 2.95bc | 9.41 ± 1.54de | 10.97 ± 2.39a |
Microplastics | 7.60 ± 2.19b | 8.91 ± 2.26ab | 9.14 ± 2.32a | 9.73 ± 2.76a | 9.61 ± 2.94bc | 9.23 ± 1.71ef | 10.13 ± 2.25a |
% Inhibition | |||||||
Macroplastics | 0.26a | 0.12a | 0.10a | 0.05a | 0.06a | 0.09a | -0.07a |
Microplastics | 0.26a | 0.13a | 0.11a | 0.05a | 0.07a | 0.10a | 0.02b |
Tolerance index (%) | |||||||
Macroplastics | 74.05a | 87.66a | 89.70a | 95.04a | 94.07a | 91.45a | 106.61a |
Microplastics | 73.85a | 86.59a | 88.82a | 94.56a | 93.39a | 89.69a | 98.45b |
*Values in column with the same alphabet are not significantly different at 5% level of probability |