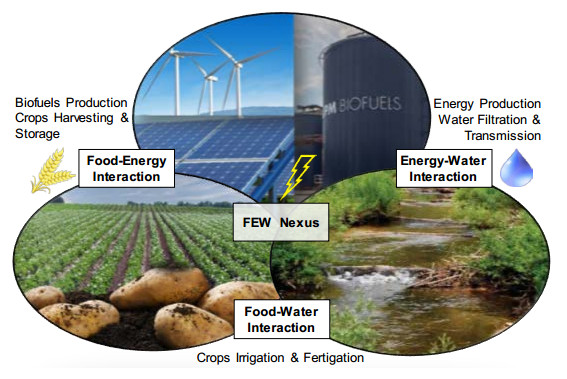
Citation: Benjamin Hersh, Amin Mirkouei, John Sessions, Behnaz Rezaie, Yaqi You. A review and future directions on enhancing sustainability benefits across food-energy-water systems: the potential role of biochar-derived products[J]. AIMS Environmental Science, 2019, 6(5): 379-416. doi: 10.3934/environsci.2019.5.379
[1] | Laura Romero, Modesto Pérez-Sánchez, P. Amparo López-Jiménez . Improvement of sustainability indicators when traditional water management changes: a case study in Alicante (Spain). AIMS Environmental Science, 2017, 4(3): 502-522. doi: 10.3934/environsci.2017.3.502 |
[2] | Pablo Andrés–Meza, Noé Aguilar–Rivera, Isaac Meneses–Márquez, José Luis Del Rosario–Arellano, Gloria Ivette Bolio–López, Otto Raúl Leyva–Ovalle . Cassava cultivation; current and potential use of agroindustrial co–products. AIMS Environmental Science, 2024, 11(2): 248-278. doi: 10.3934/environsci.2024012 |
[3] | Adam O'Keeffe, Erin Brooks, Chad Dunkel, Dev S. Shrestha . Soil moisture routing modeling of targeted biochar amendment in undulating topographies: an analysis of biochar's effects on streamflow. AIMS Environmental Science, 2023, 10(4): 529-546. doi: 10.3934/environsci.2023030 |
[4] | Bedassa D. Kitessa, Semu M. Ayalew, Geremew S. Gebrie, Solomon T. Teferi . Quantifying water-energy nexus for urban water systems: A case study of Addis Ababa city. AIMS Environmental Science, 2020, 7(6): 486-504. doi: 10.3934/environsci.2020031 |
[5] | Frank Abimbola Ogundolie, Olorunfemi Oyewole Babalola, Charles Oluwaseun Adetunji, Christiana Eleojo Aruwa, Jacqueline Njikam Manjia, Taoheed Kolawole Muftaudeen . A review on bioremediation by microbial immobilization-an effective alternative for wastewater treatment. AIMS Environmental Science, 2024, 11(6): 918-939. doi: 10.3934/environsci.2024046 |
[6] | Li Ma, Likun Zhan, Qingdan Wu, Longcheng Li, Xiaochen Zheng, Zhihua Xiao, Jingchen Zou . Optimization of liquefaction process based on global meta-analysis and machine learning approach: Effect of process conditions and raw material selection on remaining ratio and bioavailability of heavy metals in biochar. AIMS Environmental Science, 2024, 11(3): 342-359. doi: 10.3934/environsci.2024016 |
[7] | Sanjeev Sharma, Vinay Kandpal, Tanupriya Choudhury, Ernesto D.R. Santibanez Gonzalez, Naveen Agarwal . Assessment of the implications of energy-efficient technologies on the environmental sustainability of rail operation. AIMS Environmental Science, 2023, 10(5): 709-731. doi: 10.3934/environsci.2023039 |
[8] | Arriya Mungsunti, Kevin A. Parton . The sustainability of the muang fai irrigation system of northern Thailand. AIMS Environmental Science, 2019, 6(2): 77-93. doi: 10.3934/environsci.2019.2.77 |
[9] | M.A. Pardo, A.Riquelme1, J. Melgarejo . A tool for calculating energy audits in water pressurized networks. AIMS Environmental Science, 2019, 6(2): 94-108. doi: 10.3934/environsci.2019.2.94 |
[10] | Tamara S. Wilson, Benjamin M. Sleeter, Jason Sherba, Dick Cameron . Land-use impacts on water resources and protected areas: applications of state-and-transition simulation modeling of future scenarios. AIMS Environmental Science, 2015, 2(2): 282-301. doi: 10.3934/environsci.2015.2.282 |
The necessity for food, clean drinking water, and energy is the basis of the research at the nexus of food-energy-water (FEW) [1,2]. As society progresses and the population steadily grows [2], there will continue to be a strain on FEW systems (FEWS) [3]. The challenges facing the FEWS are that of limiting the pressure on a branch of the nexus when working to improve the other branches [1,4]. The all-encompassing qualities of FEWS solutions are what differentiate it from other renewable solutions, in which there is a resource that ails from the process [1]. For example, the production of ethanol from biomass (e.g., corn or sugarcane) reduces the necessity for crude oil-derived transportation fuels (e.g., gasoline and diesel), however, the production of ethanol places tremendous strain on land use, agricultural markets, and water use [5]. Then the question arises as to what should be done to mitigate the trade-offs and what should be used for the mutual benefit of the FEWS [6]. Conducted studies reported the significant effects of bioproducts (e.g., biochar and bio-oil) and their applications on the FEWS [7,8,9], as well as the existing challenges of biomass to value-added products supply chains [10,11,12].
Biochar from biomass feedstocks has been shown to improve crop productivity, mitigate carbon emissions, contribute to the filtration of wastewater, and subsequently benefit FEWS [7,9]. The challenge of creating a sustainable biochar system is limiting the environmental footprint, catering to the needs of the market [14], and being able to produce at large enough scale to make the production cost-efficient [15]. Evaluation of biochar production is impeded by restricted access to proprietary data and inadequate real-time heterogeneous/high-volume data extraction [16]. This can be linked to the lack of standardized post-processing techniques that enable sharing information among stakeholders and identifying why an operation failed or why productivity was lost through data analytics, diagnostic and prognostic assessment, and adaptive predictive models [16]. Prior studies reported that technology breakthroughs (e.g., wireless sensors and intelligent logic controllers) and data-influenced decision making are key solutions to addressing biomass to bioproducts supply chain challenges (e.g., resilience, efficiency, and productivity) at multiple spatiotemporal scales [16,17,18,19,20].
Recent interest in FEWS has stimulated research efforts to address current and future environmental challenges and FEW shortage crises in the United States (U.S.) and World [4]. Modernizing FEWS will enhance sustainability benefits, such as promoting FEW security, climate change solutions, and the livelihood of affected communities [14,15]. Also, there is a critical need for further databases and information to develop an adaptive decision support tool and potential products (e.g., unblended and blended biochar-based products) that do not strain any of the FEW branches. With the current research into the benefits of unblended and blended biochar-based products, it is evident that biochar could be a viable product to ease pressure on the FEWS [7].
The global biochar market is projected to reach $3.14 billion by 2025 [23], largely due to increasing consumption of organic food and increased awareness regarding the overall advantages of biochar across the FEWS [24,25,26], as well as carbon materials, wood polymer composites, nanomaterials, and as a reducing agent in steel production [27,28,29,30]. Delaney (2015) reported that biochar applications have significant economic and environmental impacts in the Pacific Northwest region due to the established agribusiness industry, such as crops production (e.g., potato, wheat, and barley) and food processing (e.g., milk, chess, and yogurt). The national biochar market potential is estimated at approximately $5.2 billion dollars annually within agriculture ($2.6 billion), including horticulture ($1.1 billion), environmental remediation ($800 million), potting mixes and soil conditioners ($66 million), compost ($60 million), and stormwater management ($510 million) sectors [31,32,33].
Current studies in the renewable and sustainable energy field have shown that there is a clear interlinking between the FEW branches [34]. The focus of the research into the FEWS has turned to the synergies and trade-offs in the nexus of the three branches. The study being conducted on the FEW nexus has led to many different viewpoints on how to address the challenge of improving each branch without adding strain on the other two branches [4,14]. The connections between biochar and the FEW nexus are quite clearly drawn when analyzing the biomass-to-biochar supply chains. Biochar (also referred as black carbon) is a solid by-product of the thermochemical conversion processes (e.g., pyrolysis and gasification) of biomass feedstocks, such as forest harvest residues, invasive plant species, and animal manure [35]. Its properties are similar to traditional charcoal, produced from organic products [36]. However, biochar from biomass feedstocks has a higher water-nutrient holding capacity, organic-matter efficiency, and carbon sequestration capability, which leads to addressing sustainability concerns, such as mitigating greenhouse gas (GHG) emissions, water use and reuse, energy use, and land use [9,29]. In other words, biochar is an adsorbent or soil amendment and manufactured with optimal physicochemical properties for these purposes, but charcoal is a fuel source and manufactured with optimal fuel properties. Biochar, activated carbon, and charcoal have several common features, however, biochar also has some unique properties (e.g., density, cation exchange capacity, bulk density, and mechanical hardness) that can distinguish it from other products for FEW treatment purposes.
Unblended biochar products and biochar blended with other materials (e.g., nutrients) have widespread applications across FEW sectors, as a soil conditioner and additive in organic fertilizers [35], for water filtration and adsorption of contaminants in soil and water [30,31], in livestock farming and animal feeds [32,33], as a food additive and a pharmaceutical [41], as a fuel for electricity generation, heating, and cooking [9,35], among others. The uses of biochar as an additive to fertilizers show the ability to significantly benefit both food and water resources [43]. It benefits food by aiding in the growth of the plants and providing macronutrients, such as nitrogen, phosphorus, and potassium [8,37]. Biochar-based soil amendment, also known as an organic soil conditioner, leads to reducing fertigation (i.e., injection of fertilizers into the irrigation systems) and enhancing soil quality and crop health growth and yields [36,38,39]. Additionally, biochar application in water filtration systems is much more effective than charcoal due to high adsorption capability [47], which leads to entrapping unwanted contaminants in the water due to the porous nature of biochar [13]. The necessity for clean water is quintessential in the future of the population and standard of living [48].
Apart from the stated benefits, the existing biochar production process has not been implemented commercially in comparison to artificial, non-organic products with similar applications, such as conventional fertilizers or water filters [49]. A cost-competitive biochar production process is what the market needs to fully adopt this product [43]. Market-responsive biochar has applications in various industries, such as soil, food, chemicals, water, and energy [50]. Based on prior biochar techno-economic studies, the first and second major cost-drivers are the direct production process (over 50% of total cost) and biomass feedstock collection and transportation (over 30% of total cost), respectively [51]. In the U.S. Northwest region, feedstock suppliers are from timber companies (e.g., Weyerhaeuser, Georgia-Pacific, and West Fraser supply wood residues) with forest-based products, and from dairy companies (e.g., Magic Valley Dairies in Idaho) with livestock products. Delaney (2015) reported that biochar market value was approximately $400,000 annually in this region, and the biochar prices ranged from $90 to $600 per cubic yard [33].
Among thermochemical production processes, pyrolysis technology is expected to witness rapid growth due to higher yield processes and high-quality products in terms of carbon content and stability [23]. Pyrolysis process is one of the most efficient ways of producing high-quality biochar and bio-oil from biomass; mainly, slow pyrolysis is reported as a cost-effective conversion process for biochar production due to high operation yield and end product quality [52]. The competing gasification technology has witnessed increased demand due to the growing need for electricity in distributed energy systems [53]. However, gasification is expected to lose its share over the forecast period, as it does not produce biochar that is stable enough to be used in agriculture for soil amendment and enhancement purposes due to the formation of soot and tar [46,47]. The pyrolysis and gasification conversion environments (e.g., residence time and temperature) affect biochar physicochemical properties (e.g., surface area and polycyclic aromatic hydrocarbon content) that require further investigation. The main drawbacks of gasification are high energy requirement and low production yield in comparison to pyrolysis that increase the total biochar cost.
Currently, the key knowledge gaps in manufacturing and application of biochar-derived products are (a) elucidation of impacts of different biochars with similar/diverse properties on soil fertility, crop yields, and wastewater treatment, (b) understanding biochar complexity and heterogeneity to design and optimize biochar-based FEWS treatments, (c) exploration of potential risks of biochar utilization on crop growth and yields, as well as biochar-facilitated contaminant transport via water treatment or the food chain, and (d) investigation of economic implications and environmental footprints of biochar-based materials [48,49]. Therefore, this review study investigates the key concepts and connections between FEW branches and biochar applications.
As the focus on the intersection of FEWS is relatively new, a literature review of prior studies will assist in the compilation of persuasive data and information to form a better understanding of the existing linkages and to maximize effective management of FEWS. The methodology applied in this study consists of the narrative and systematic literature review techniques to explore the state-of-the-science and the possible solutions and products that have the capability to improve FEWS, as well as to identify prospective directions for enhancing sustainability benefits across FEWS. The narrative review was conducted by comparing existing FEWS methodologies, along with exploring the potential impacts of biochar-derived products to FEWS. The systematic review was performed using qualitative and quantitative approaches to studies published from January 1, 2008, to January 1, 2019.
The narrative review in this study (1) identifies the key concepts and advancements of research in FEWS, exploring the purpose of current FEW studies, defining the challenges facing FEWS as reported in existing articles, and discussing the possible solutions to overcome these challenges, and (2) identifies the evolution of research on the biochar and its application in various sectors (e.g., energy and agriculture) with a focus on sustainability aspects, i.e., economic, environmental, and social. The existing linkages in the effective management of FEWS are identified through key concepts and major contributions discussed in the current literature. Several studies discuss that the advancement of FEWS will be critical in the future of sustainable living as each branch of FEW nexus is co-dependent and necessary to sustain current standards of living.
Systematic literature reviews are essential to efficiently keep scholars up-to-date on current research and development [57]. The systematic review (SR, presented herein and Supplementary Materials) utilizes a series of strategies, evaluations, and analyses to classifying previous studies based on their key attributes [58,59]. Reducing the author bias is one of SR benefits in the analysis of literature compared to a narrative review, which avoids picking information and examples from the narrative review to support the author's point of view [58]. The SR conducted herein includes qualitative and quantitative methods to provide a classification for available studies from leading journals and scholars. The quantitative method analyzes recently published studies, citation data, and keywords. The qualitative approach comprehensively characterizes current literature to classify the research methodologies of the most-cited publications. Traditionally, qualitative literature reviews are classified into two main categories (i.e., analytical and empirical) and several subcategories, which have been identified in recent studies [58].
The FEWS acronym and definition is presented in different ways across the literature. Some reports rearrange the acronym to be WEF, which easily gets confusing, for example, when presented at the World Economic Forum (WEF) in 2011 [60]. This forum presents the nexus as Water-Energy-Food-Climate in other studies, and Agriculture and Land are also interchanged with Food. When analyzing the literature of FEW research, it is first important to examine the nexus thinking [61]. The nexus definition could vary significantly based on the supply, demand, and resources of the area of interest; for example, natural water resources can be used in the generation of hydroelectric energy or irrigation of the land to produce different crops, such as potato or wheat [61]. The utilization of these resources is dependent on the short-, middle-, and long-term goals of the decision makers. A fundamental understanding of FEW nexus is useful in addressing the complex, interdisciplinary nature of existing challenges in this field and stimulate interactions to meet often-conflicting objectives [62].
The connection between three branches is the key to the FEW nexus (Figure 1). The understanding of the interlinkages in FEWS is critical in decision-making processes as it relates to managing stressors on each branch [6]. The primary goals of FEW focused research are maximizing mutually beneficial outcomes, as well as reducing system-level costs [51], environmental impacts, and risks through improved process-/system-level efficiency and productivity. The analysis of the interlinkages provides a blueprint on how to approach environmental needs and maintain competitiveness in the marketplace [63]. Additionally, the analysis of FEWS is much like a life cycle assessment, in which the facilitation of complex decision making in terms of socio-environmental responsibility and techno-economic aspects can be assessed with an emphasis on the connection of all three branches [7].
Earlier FEWS efforts focused mostly on the acknowledgment of FEW nexus and the need for new strategies and decision support systems that show potentials to address existing limitations and challenges [64]. FEW nexus thinking was conceived by the World Economic Forum in 2011, which led to an unprecedented discussion on the future of water security and a call to action on water awareness [14,53]. Population growth was established as the primary concern facing the nexus, as well as the role climate change plays in contributing to the difficulties facing FEW nexus.
Recent studies centered on integrating the existing methods to develop a holistic approach and address FEW issues as a whole [65]. Al-Saidi and Elagib (2017) reported that there is currently no consistent scientific approach or policy to overcome FEWS barriers [66]. They reviewed the integration of the smaller connections (i.e., food-water, food-energy, and water-energy systems) to guide decision makers (e.g., researchers, managers, and policy makers) to meet the global needs instead of reviewing FEWS as a whole.
The growth of the human population is expected to increase the food demand globally by 60% by 2050 [5], which is coupled with the loss of available farmlands (due to urbanization) and the shortage of available water resources (due to projected climate change and water contamination). Without the addition of organic and non-organic fertilizers, the current agricultural production cannot sustain the needs of humanity [43]. Food and crop production levels are dependent on water availability [67]. The agricultural sector accounts for approximately 71% of global water withdrawals and consumes approximately 6.6% of the renewable water resources available, generated through hydrological cycles that flow into lakes, rivers, and streams [3,61].
➢ A couple of major concerns in food-water systems that have to be monitored and managed to minimize health risk and degradation of water resources are:
➢ The use of wastewater for irrigation purposes in the areas without freshwater resources [61,62],
➢ Erosion of land resources and runoff from the inefficient irrigation and fertigation of agricultural lands [70],
➢ Eutrophication and water pollutants due to the oversupply of nutrients (e.g., nitrates and phosphates), synthetic fertilizers, and pesticides [71], and
➢ Salt level of soils and salinization of water resources that, in turn, inhibits water uptake by plants [62,65].
Thus, the evaluation of surface and groundwater used in irrigation/fertigation is critical to managing global water consumption and food supply. Traditional irrigation practices are no longer effective and viable to address challenges arising from water scarcity and consumption, as well as contamination. Besides, new water district restrictions, policies, and jurisdictions across several U.S. states (e.g., Idaho, California, and Texas) play an essential role in the nation's agricultural vulnerability, and crop productivity as 80% of global agriculture is rain-fed [60,66]. These restrictions increase the production risks in rain-fed cropping systems when the precipitation is unpredictable, especially in semi-arid zones [74].
Irrigation technologies (e.g., drip and sprinkler systems) and management strategies stabilize water supplies for crop production and agriculture-driven economy through promoting irrigation efficiency and 'crop per drop' measurements, using adaptive conservation operations [68,69]. Deficit irrigation (DI) is one of the promising irrigation management strategies that utilizes collected data about water stored in the soil, evaporate losses, crop water use dynamics, and precipitation throughout the growing season [68,70]. DI determines the effects of the mentioned parameters on crop productivity and quality. On-farm irrigation technologies poorly evaluate crop water demand to match the supplies and incorporate conservation measures into practice in real time [73]. Ultimately, monitoring of soil moisture content provides useful insights into agriculture infrastructures (e.g., water distribution and management) for determining sustainable, optimal irrigation strategies to meet national priorities and food-water security.
Crop and food production has a profound effect on the energy branch of FEWS as currently, agriculture accounts for nearly 30% of global energy consumption and 20% of GHG emissions [5]. The majority of the energy needed in the food sector comes from the processing, distribution, preparation, and cooking [34]. In the U.S., the food industry is the fifth largest energy consumer in the manufacturing sector [78]. The food sector relies heavily on fossil fuels and volatility in the market has adverse effects on food prices; this dependence leaves the food sector very vulnerable to fluctuation and makes sustainable development more difficult [79].
Over the past 50 years, the need to advance food-energy systems to make them more reliable, efficient, and productive has been fueled by the rapidly growing population (expected to reach nine billion by 2050) and the expectation of doubling the global food demands in the next 30 years across the World [73,74]. Precision agriculture and other advanced production practices play a key role in food-energy security. The utilization of cyber-physical initiatives for big data analytics allows for better irrigation/fertigation, as well as forecasting crop yield under a wide variety of conditions [82].
Alternative and renewable energy sources arise in the industrial sector to overcome major challenges (e.g., energy security and environmental emissions) regarding high energy consumption and energy footprint [83,84,85]. One of the main attributes of food-energy nexus is bioenergy production from agricultural leftovers and residues (e.g., corn stover, wheat straw, and diseased crops) by using uncultivated lands [86]. Bioenergy production from food resources (also known as first-generation biofuels) puts pressure on the food industry and increases food demand and price, which is one of socio-economic concerns of food-energy nexus [5,80]. Additional to the stated shortcomings, energy production from water resources via thermal power and hydropower generation places a burden on fisheries and freshwater resources [34].
Water and energy are critical resources in economic growth and have a unique interdependence on each other [81,82]. The water-energy nexus is quantified by either kilowatt-hour consumed per cubic meter of water supplied, or alternatively, by the cubic meters of water used per kilowatt-hour produced [90]. The links that are drawn to the usage of these respective quantities are mostly broken down into production and transportation. A few examples of the production of water and electricity resources include water collection, water use for electricity generation, water use for bioenergy production, and electricity use for wastewater treatment and water desalination, purification and transmission/distribution [91]. Transportation of water resources includes pumping or other forms used for groundwater extraction, surface water transfers, retail distribution, and wastewater collection [91]. The energy-water nexus can also be broken down into multiple scales, for example, end-users and individuals (small scale) who consume large amounts of energy in the heating of water and large amounts of water in the cooling of their households; or water and energy supplies for an entire country (large scale) for various purposes, such as food processing and crops production, as well as waste and wastewater treatment [90].
Most electricity production requires water in different phases, steam for electricity generation in nuclear and thermoelectric, or liquid for hydroelectric [82,85]. Energy production consumes (without the ability to reuse) approximately 66 billion cubic meters of fresh water each year and requires 580 billion cubic meters of water across energy production methods globally [34]. In the U.S., energy accounted for 27% of water consumption in 2010 [92] and rose to 37% by 2014 [93]. Each energy production technology has unique water consumption needs. Thermoelectric power plant cooling is responsible for around 4% of all U.S. water consumption, with nuclear energy being the highest consumer [92]. Thermoelectric water consumption will likely increase due to the introduction of closed-loop steam turbines and increased nuclear power generation [92]. Biofuel produced from corn ethanol is one of the most water-intensive energy sources due to the amount of water needed to irrigate corn fields, particularly in the low-precipitation areas [94]. Water usage in fossil fuel energy production is dependent on the fossil fuel source, with coal and oil being more water-dependent than shale gas and natural gas, which requires the least amount of water [92]. The occurring shift in the U.S. and World will change energy generation sources, utilizing more closed-loop systems to both decrease CO2 emissions and impacts on aquatic species. However, such a shift will increase water consumption levels [95].
There is increasing recognition that FEWS are tightly intertwined and interdependent, such that activities within one of the three sectors (e.g., food, energy, or water) will affect the other sector(s), which is the main reason behind the 'nexus' concept [96]. Since global challenges are interconnected, nexus approaches (if well implemented) can detect trade-offs and interactions among various sectors, mitigate process-level impacts, and promote sustainable development strategies. Table 1 represents a number of FEW studies published between 2011 and 2018.
Study | Research overview | Year |
Bazilian et al. | An overview of the linkages across FEW nexus was provided to develop a framework and address policy making at a national level. | 2011 |
Siddiqi and Anadon | A country-level quantitative analysis of FEW nexus was conducted to suggest possible considerations for food and water policy in the Middle East and North Africa regions. | 2011 |
Jägerskog et al. | An overview was reported to determine how regional FEW nexus centered in collaboration could benefit the economic and environmental aspects in the Central Asian and Aral Sea Basin. | 2012 |
Villarroel Walker et al. | A pros and cons analysis of adopting FEW perspectives was conducted for addressing the needs of urban areas based on case studies performed in Atlanta, GA, USA and London, UK. | 2012 |
Ringler et al. | A review and analysis of FEW Land nexus were provided, in which the resource use efficiency is the main focus. This review lays out the connections between FEW branches and gives examples of FEW Land improvement methods. | 2013 |
Lawford et al. | A summary of major factors influencing the security of FEW nexus in the Lake Winnipeg and Southern Asia region was presented. This study focused on the importance of water resources and water security across FEW nexus and nexus decisions. | 2013 |
Rasul | A literature review of FEW nexus research being conducted in South Asia region was provided. This study focused on the synergies and trade-offs, as well as the regional FEW challenges. | 2014 |
Stein et al. | This study included a quantitative method of social networks, linked to FEW nexus in Tana and Beles subbasins. | 2014 |
Villarroel Walker et al. | A quantitative analysis of FEW nexus was conducted in urban areas, utilizing a multi-sectoral system analysis to quantify resource use, synergies and antagonisms, and monetary value of FEW nexus in the London, UK region. | 2014 |
Flammini et al. | A qualitative and quantitative analysis of FEW nexus was conducted that defines FEWS interactions and evaluates the performance of FEW related policies. | 2014 |
Biggs et al. | A critical review of FEW nexus thinking was presented, which the livelihood is taken into consideration for FEW nexus decision making. This study reviewed the current literature on sustainable livelihoods and how they can be applied to improve FEWS. | 2015 |
Conway et al. | A review study with empirical data was provided to examine FEW nexus in the southern Africa region, which highlighted the climate change implications of nexus decision making. | 2015 |
Daher and Mohtar | A FEW nexus modeling tool was proposed to identify sustainable national resource allocation approaches and evaluate case studies in Qatar, Middle East. | 2015 |
Jeswani et al. | This study explored the environmental sustainability issues in FEW nexus, using life cycle assessment for breakfast cereals. | 2015 |
Kraucunas et al. | This study proposed a platform to stimulate interactions among natural and human systems at a regional scale. The conducted experiments focused on the eastern U.S. | 2015 |
Mukuve and Fenner | This study explored the stresses over FEW physical resources in Uganda's food system. It is concluded that the results help food security policy and management, particularly in Uganda. | 2015 |
Ozturk | This study examined FEW nexus for long-term sustainability in the Russian Federation, China, Brazil, South Africa, and India. | 2015 |
Endo et al. | This study collected the existing method to examine FEW nexus and classified them as quantitative or qualitative approaches. It is also discussed case studies in Japan and Philippines. | 2015 |
Middleton et al. | This study defined FEW nexus as a concept and narrative, and applied the concept to South Asia through an environmental justice lens. | 2015 |
Villamayor-Tomas et al. | The development of a FEW nexus framework was presented by combining the insights fo the Institutional Analysis and Development framework and value chain analysis. | 2015 |
Keskinen et al. | This study focused on the definition of FEW nexus, employing this definition, and using a study of the Tonle Sap system to fill a research gap in the understanding of the nexus. | 2015 |
Garcia and You | A literature review of FEW nexus was reported, highlighting FEW challenges and identifying process engineering research opportunities. | 2016 |
Rasul | A conceptual analysis of FEW nexus with emphasis on climate change was presented. This study analyzed FEW nexus in South Asia region and provided FEW decision making suggestions. | 2016 |
De Laurentiis et al. | This study reviewed food security challenges and proposed three pathways to address consequences. The concluded FEW approach is prerequisite for using the proposed pathways. | 2016 |
Cairns and Krzywoszynska | This study discussed the importance of FEW nexus research and development. It is concluded social sciences have major roles to play and may provide opportunities. | 2016 |
Yang et al. | A scenario analysis under FEW nexus thinking was presented to identify the sustainability challenges and conflicts in Brahmaputra River. Their proposed method is expected to diagnose the water resources deficiencies due to various reason, e.g., climate change. | 2016 |
de Strasser et al. | This study proposed a nexus approach for assessing a transboundary basin, impacts across sectors, and policy measures at the national level to reduce intersectoral tensions. | 2016 |
Fasel | This study focused on how FEW nexus thinking gives an innovative approach to mitigate water scarcity of the Black Sea region. | 2016 |
Perrone and Hornberger | An analysis of FEW nexus in Sri Lanka was provided to establish trade-off frontiers and illustrate the system-level tradeoffs of water allocation. | 2016 |
Mortensen et al. | A proposal was provided to employ FEW nexus policies to nutrient management in the Rio Grande region, as well as a quantitative analysis of trade-offs associated with wastewater irrigation. | 2016 |
Wichelns | A critical review was conducted to analyze FEW nexus and reported the necessity for FEW nexus thinking and decision making. | 2017 |
Endo et al. | A review study was presented on FEW related research to determine the state of nexus research in each Asia, Europe, South America, Oceania, Middle East, North America, and Africa regions. | 2017 |
Howarth and Monasterolo | This study characterized FEW nexus to understand externalities and evaluate a bottom-up, participative approach in UK, including 78 stakeholders from academia, industry, and government. | 2017 |
El Gafy et al. | This study proposed a dynamic model approach to incorporate uncertainties to FEW nexus policy making and analyze the dynamic behavior. | 2017 |
Wicaksono et al. | A literature review study focusing on the global FEW nexus was provided, including a review of nexus models and opportunities for future modeling efforts. | 2017 |
Dhaubanjar et al. | The development of a spatially explicit framework for FEW decision making was provided, in which a comparative review of Nepalese power development is analyzed. | 2017 |
Hussien et al. | The development of a bottom-up dynamic system model was presented to quantify FEW nexus at an end-use level. | 2017 |
Johnson and Karlberg | An analysis of FEW nexus tools to develop a decision making framework was conducted, including the presentation of how scenario-building creates space for dialog. | 2017 |
White et al. | A quantitative analysis of FEW nexus was performed in the East Asia region with a focus on the effect of inter-regional trade across FEWS. | 2018 |
Albrecht et al. | A systematic review of FEW nexus assessment methods was provided to analyze current FEW assessment literature to determine the repeatability of the studies, the attention to the interlinkages of the three branches and breaks down the focus of FEW assessment studies. | 2018 |
Pahl-Wostl | An analytical framework was proposed to show the potential of Sustainable Development goals in benefitting FEW nexus and analyze the governance of FEW nexus. | 2019 |
Biochar-based products (unlike charcoal) have many socio-environmental sustainability benefits. When applied to soil, biochar reduces the amount of harmful gases (e.g., NO, N2O, NH3, and CH4) released into the air via (a) immobilizing inorganic nitrogen, (b) affecting enzyme activities relevant to gas production, and (c) modifying soil physical properties (e.g., texture/structure, porosity, density, and temperature) that can increase water-nutrient holding capacity and reduce bulk density of soil [143,144,145,146]. Besides, if produced from wood residues, the natural carbon stored in the plant matter is retained in the biochar, which is proven to degrade at a much slower rate than standard organic waste (e.g., forest harvest residues) [139,140]. Biochar-based soil conditioner can improve both physical and biological soil properties, including the soil moisture and nutrient retention, along with the various benefits to plant growth, including disease suppression [148]. Some of the known biochar benefits to soil are: (a) soil toxicity reduction due to immobilization and/or transformation of heavy and toxic metals, (b) soil pH and structure improvements, (c) soil tensile strength reduction, and (d) the fertilizer use efficiency enhancement, which overcomes the aforementioned FEWS barriers [8,9,142].
This study focuses on the thermal decomposition of biomass feedstocks for biochar production, particularly the pyrolysis conversion pathway (Figure 2), due to high process efficiency and product quality [150]. Pyrolysis process yield is dependent on a few critical parameters, such as temperature, pressure, and exposure time of biomass feedstocks to the heating source [13]. The primary products of biomass pyrolysis process are biochar, bio-oil, and syngas (non-condensable gas) [151]. Syngas has the potential to be recycled to pyrolysis reactors for heating purposes and for reducing biomass moisture content [152]. Until recently, the main focus of biomass pyrolysis was the optimization of the conversion process to produce pyrolysis-oil (bio-oil) and upgrade it to transportation fuels (biofuels or blended fuels). Prior studies reported that biochar production, using a slow pyrolysis process at temperatures below 300 ℃ (572 °F) has high process yields [20,146].
On the other hand, biochar quality and chemical properties with respect to its applications as a soil amendment product have similarities to the charcoal [143,147]. The effects of charcoal use in agriculture for promoting soil health and crop yields have been reported in earlier studies in the U.S. and South America [148,149]. Jeffery et al. (2013) reported that charcoal plays an essential role in the fertilization of unusable lands alongside the Amazon River [157]. Taha et al. (2014) conducted a study that shows the differences in the total pore volume and specific surface area of biochar versus charcoal [159]. Their analyses reported the specific surface area (m2/g) of two representative biochar samples to be on average 1.56 times larger, and the total pore volume (ml/g) to be 2 to 5 times larger, than charcoal. It was also concluded that these greater properties of biochar could lead to higher absorption of pesticides in the aqueous phase [158].
When used for soil amendment, biochar effects on the food-water nexus (agricultural productivity and water security) are a direct result of the modification of soil properties, e.g., the nutrient-water holding capacity due to biochar's porous nature [160]. Biochar-based fertilizer applications for soil productivity and crop yields have been shown to increase plants' nitrogen intake by 400% and the soil pH from 4 to 6 [161], and the plant growth by 250% [7,28]. Biochar effects are dependent on various factors, such as feedstocks used (e.g., forest/agricultural residues, advanced feedstocks, or animal manure), conversion process configurations (e.g., reactor types, temperature/pressure, or residence time/heat transfer rate), and soil conditions (e.g., soil texture, amount of micronutrients or pH) and crop types [136,155,156]. Different biochar-based soil amendment products are produced to cater to the needs of specific crops and soil conditions [164,165,166]. The specific elements of concentration and the soil cation exchange capacity are some of the properties that can be controlled [7].
Biochar-based soil conditioners change the consistency, packing, density, and porosity of soil, and have direct effects on water and air penetration that can benefit the root zone of plants [37,145]. Since biochar is inherently more porous than soil, the influence of biochar to water-nutrient holding capacity is measurable, along with the workability, permeability, and swelling-shrinking dynamics [37,160]. The changes to the soil's physical nature and biochemical properties are also seen in the increase of soil-specific surface area, which improves the structure and aeration of soil [37,161]. Biochar also improves the amount of soil cation and anion exchange capacity, which has a direct impact on increasing soil pH, nitrogen, and phosphorus levels, as well as to decrease the availability of heavy metals [37,162]. These properties lead to the encouragement of root development of the plants and the reduction of the available aluminum in soil [161]. A meta-analysis based on 371 independent studies found that soil amendments of biochar generally resulted in increased above-ground productivity, crop yield, plant potassium tissue concentration, soil microbial biomass, rhizobia nodulation, soil potassium, total soil carbon, soil phosphorus, total soil nitrogen, and soil pH [44].
Additionally, soil amendment of biochar-based products has been shown to increase the microbial health of the soil [170], with significant effects on soil microbial biomass, community composition, and enzymatic activities [164,165]. Particularly, biochar additions resulted in an increase in bacterial diversity, a shift in the composition of the rhizosphere microbiota, and stimulation of microbial metabolism in the rhizosphere, which together could lead to enhanced plant performance [173,174]. Moreover, biochar has been shown to suppress soil-borne plant pathogens due to its influence on the soil-rhizosphere-pathogen-host system [168,169]. Furthermore, biochar may affect microbial decomposers and thereby the turnover and availability of soil organic matter, e.g., carbon, nitrogen, phosphorus [44]. Additionally, biochar-microbe interactions contribute to soil improvement (including carbon sequestration) and pollution remediation [170,171].
Biochar-treated soil has a higher water-holding capacity, which decreases nutrient leaching and soil erosion and reduces the effects of drought and environmental stresses on crops [136,172,173]. Soil moisture content is a critical factor to consider in linkages among all three FEW sections because soil with higher water retention requires less water and energy to deliver the necessary resources to crops, subsequently relieving irrigation levels [76]. The high water content is also one of the key factors to increase the crops/plants yield obtained by biochar amended fields [149]. Biochar adds a physiochemically active aspect of soil that is capable of removing heavy metals and toxic compounds from soil [149]. Some of the metals that can be absorbed include aluminum, manganese, arsenic, nickel, copper, and lead [181]. Specifically, biochar leachate was shown to facilitate microbially-mediated dissimilatory metal reduction, likely due to the electron shuttling capacity of biochar-associated semiquinone functional groups and adsorption of reduced metals on biochar [182].
Carbon sequestration (i.e., the process of long-term storing carbon) has become essential to prevent GHG emissions into the atmosphere [148,176]. In order to be properly stored, carbon must be transformed from an active state to an inert passive state [184]. When biochar has been applied as a soil amendment, the carbon sequestration takes place within biochar for several decades [28,40]. Storage of carbon in soil through biochar application can significantly reduce atmospheric CO2 as the uptake of CO2 by plants is significantly greater than current anthropogenic CO2 emissions [136,176,178]. Therefore, compared to the natural decomposition of organic materials, biochar amendment serves as a significant carbon sink.
The use of fertilizers is necessary to secure the global food supply; however, the production process of fertilizers is highly energy-intensive and results in releasing significant GHG emissions [186]. Replacing current non-organic fertilizers with biochar-derived soil conditioners has been identified to not only improve soil conditions but also stabilize organic carbon and reduce GHG emissions [187]. Soil contains low amounts of unstable carbon, and when biochar is added to soil, it improves the stability of organic carbon in soil [37,176]. Additionally, biochar-treated soil adds the resistance of global carbon to chemical and biological degradation by increasing terrestrial carbon stock or soil carbon content. In the biochar production process, the carbon contained in biomass is either captured in biochar or other co-products of the pyrolysis process, e.g., bio-oil. Latest studies reported that 20% to 35% of the carbon stored in biomass feedstock is captured in biochar [143].
Biomass pyrolysis process has the potential to be a net-zero or even a net negative carbon emission process because the carbon released during plant bioproducts combustion is part of nature from biogenic substances and nearly equal to carbon absorbed by plants in farms or by trees in forests [188,189,190]. Carbon is naturally found in biochar produced from carbon-based feedstocks and organic waste streams, which is a natural derivative of the photosynthesis process. In other words, soil and plants absorb carbon dioxide from the atmosphere through the photosynthesis process, which later unused feedstocks can be utilized to produce biochar, and return and trap the carbon in soil through the use of biochar-derived soil conditioners. Biochar releases carbon at a much slower pace than the organic carbon cycle, therefore, a net negative or carbon neutrality occurs [136,176,177].
Animal manure-based biochar production has the potential to reduce up to 10% release of CH4, a GHG with a significantly higher warming potential than CO2, to the atmosphere in relation to livestock [143]. N2O is the main contributor to climate change as it absorbs 300 times more thermal tropospheric radiation [144]. N2O can be emitted through the process of soil nitrification and denitrification. When applied to the soil, biochar is also effective in the trapping and immobilization of nitrogen from inorganic sources and decreases the volatilization of ammonia, which is due to the high carbon to nitrogen ratio of biochar [144]. Manure-based biochar can not only add micronutrients and carbon to the soil, but also control the release of odor and amine gases from the animal manure [191,192,193].
The runoff from agricultural leftovers and animal wastes can become harmful and pollute ground and surface water due to the overloading of biohazards (e.g., microbial pathogens) and nutrients (e.g., nitrogen and phosphorus) [194]. Fresh animal manure has been used as a fertilizer because it can add nutrients and organic matter to soil [195]. Prior studies reported that chicken manure is more beneficial due to high nutrient value in comparison to cattle manure [189,190]. However, there are various environmental, public health, and biosafety concerns linking to the use of untreated animal wastes and manure [198,199,200]. For example, microflora associated with animal manure, such as bacteria Escherichia coli O157:H7, Listeria, Mycobacterium, Salmonella, Campylobacter, protozoal Cryptosporidia, and Giardia, could pose a public health risk [201,202,203]. Thus, adding manure of both livestock and poultry to soil is risky because it can (a) burn the plant roots, (b) inhibit seed germination, and (c) transfer bacteria and disease from animal manure to humans [199].
Biochar production from these organic wastes will alleviate the stress on the environment, as well as provide environmentally beneficial bioproducts [197,198]. The utilization of wastes (especially near the collection fields) for bioenergy and biochar production could not only provide an economic opportunity but also reduce the digestion and decomposition of the organic matter that contributes a significant amount of GHG emissions [42]. The carbon contained in the wastes is unstable, meaning that it will be returned to the atmosphere quickly. Biochar has the ability to lock the carbon in the soil for long-term storage or be utilized in a combustion process as a low-emission energy source with either syngas or co-fired in coal plants [199,200]. Biochar benefits over untreated biomass include increased biosafety, energy density, and carbon stability [208]. Biochar is also beneficial in the reduction of cost and environmental footprint of the bioenergy production process by burning both biochar and syngas for heat and electricity generation [206]. Moreover, biochar co-firing with coal reduces the emissions of coal-fired power plants, although biochar energy density is low, which requires more biochar to be combusted to obtain the same amount of energy as coal (Table 2) [207].
Density kg/m3 | Moisture content w.b.%* | Energy density MJ/kg | |
Green whole tree chips | 350 | 45 | 11 |
Solid wood, low density (Douglas-fir) | 400 | 12 | 17 |
Solid wood, high density (Oak) | 865 | 12 | 17 |
Bio-oil | 1200 | 25 to 30 | 18 |
Biochar (pine wood) | 350 to 500 | 5 | 28 |
Charcoal (oak) | 700 | 5 to 7 | 29 |
Coal (anthracite) | 1100–1400 | 3 to 16 | 33 |
* wet base |
Availability of clean water resources is essential to promote the sustainability of all ecosystems, as no living organism can survive without access to water. For humans and terrestrial plants, clean water is critical, but only around 3% of the Earth's water is freshwater; and out of 3%, only 0.6% is available for human use [212]. When focusing on the purification and decontamination of water for consumption by humans, there are many factors to take into account [213]. Particularly, the sources of drinking water contamination include, but are not limited to, pathogenic organisms, toxic inorganics, radionuclides, and synthetic/organic materials. Even groundwater that is usually more pristine than surface water may contain harmful chemicals, such as arsenic and high levels of fluoride [214]. Especially in poverty-stricken areas, there is a need for a cost-effective water filtration process. It has been found that biochar produced from forest and agricultural residues has the ability to be utilized in cost-competitive water treatment systems [205,206,208].
Biochar can provide a cost-effective addition to filtration systems due to its ability to be created from unused organics and wastes [55]. Unlike other filtration methods that are expensive and only target certain organic materials or heavy metals, feedstocks-based biochar is able to remove both metals and organic materials [55]. Other than filtration by boiling water, each different filtration approach requires the use of outside materials and chemicals [216].
Biochar filtration approach is similar to the traditional charcoal approach and is used as an effective contaminant removal system due to biochar ability to absorb heavy metals and other chemical contaminants [55]. Pyrolysis process is able to activate the naturally occurring celluloses, hemicelluloses, sugars, and proteins in the organic waste to absorb unwanted chemicals. The porous carbon-structure of biochar has a large surface area with an affinity for toxic chemicals and heavy metals [13]. The removal of heavy metals depends on the pore size, porosity, and cation exchange capacity of biochar, which correlates directly to the molecular sieving capability and selectivity of biochar [48,136].
Wastewater treatment, especially for the food processing and manufacturing industry, has gained particular attention [42,210]. In order to avoid penalties, companies must first treat their wastewater to contain no more than 1000 mg/L of biodegradable organic compounds [49]. This process currently is expensive and generates significant GHG emissions [42,206]. The sorption of aqueous contaminants including heavy metals by biochar produced from animal manure and crop residues has been found to be quite successful, allowing compliance with stringent discharge limits at low economic costs and GHG emissions [218]. A benefit of biochar-based filters is the absorption of lead in drinking water, which is toxic to humans and causes long-term health problems [212,213]. Cao et al. (2009) conducted an experiment where lead sorption was tested by mixing biochar with a sodium nitrite solution containing lead. They reported after half an hour the sorption reaction reached equilibrium and the lead levels in the solution decreased to near the limit of the detector at 0.05mg/L from the original concentrations of up to 1 mm (0.39 inches). Biochar is also able to treat water contaminated by agricultural runoff from croplands receiving phosphorus-rich fertilizer. Excessive aqueous phosphate is harmful to both humans and the ecosystem in short- and long-term aspects and is responsible for increased eutrophication in aquatic environments [212].
Recent studies investigated the GHG mitigation potential of biochar soil conditioners in crop production, using environmental sustainability assessment and life cycle analysis [222,223,224,225,226]. Uusitalo and Leino (2019) explored possibilities to neutralize global warming impacts of crop production, using biochar [227]. They concluded that biochar production from side flows and buffer zones biomass is able to neutralize CO2 eq. emissions of oat production [227]. Thers et al. (2019) conducted a life cycle analysis to determine the potentials for GHG mitigation, particularly carbon sequestration, using winter oilseed rape for biodiesel and biochar production via pyrolysis [228]. Their results show that biochar has the potential to improve carbon sequestration of oilseed rape biodiesel production by 83% [228]. Several other studies investigated biochar application to various soils (e.g., tropical, temperate, and desert) and crops to improve environmental sustainability and agricultural productivity [222,223]. A review study on the biochar impacts and benefits to soil properties is given by Ding et al. (2017) [231].
In addition to biochar life cycle analysis, several studies evaluated the crop productivity and economic profitability, and implications of blended biochar-based products [232,233,234]. Shahzad et al. (2018) evaluated the impacts of biochar from green and poultry waste blended with inorganic nitrogen on wheat productivity [233]. Their results suggested that blended biochar increases wheat grain yield, protein content, and nitrogen uptake [233]. Mohammadi et al. (2019) explored the potential economic benefits of biochar production from rice residues for soil application, and their results show 12% increase in net present value of rice production, using biochar [235]. Further details are provided in the review study on biochar applications to improve soil fertility by retaining carbon and nutrients and to maximize biochar effectiveness, mixing with other additives, along with economic feasibility of biochar application by El-Naggar et al. (2019) [236].
There are few studies that evaluated both life cycle analysis and economic assessment of biochar-based products, mainly when biochar was applied for improving soil fertility and crop productivity [237,238,239,240]. Li (2018) investigated biochar life cycle analysis and regional techno-economic study in three U.S. counties/states (i.e., California, Iowa, Florida), using three crops (i.e., rice, corn, and peanuts) [241]. Their results indicate biochar application can increase the crop yield up to 10% over 20 years [241]. Ji et al. (2018) developed a combined life cycle analysis and cost-benefit analysis to assess the sustainability benefits (economic and environmental) of biochar [242]. They reported that biochar is one of the most promising products for GHG mitigation and straw utilization in China [242]. Barber et al. (2018) explored both economic and environmental sustainability of biochar filtration system (harvesting and monetizing nutrients from wastewater) and reuse it as soil amendment (synthetic fertilizers) to improve lettuce and basil quality and yield [49]. Aller et al. (2019) conducted a study on corn yield and soil quality, evaluating the long-term (11 years) effects of biochar in the U.S. Midwest (Iowa) in terms of productivity and environmental performance [243]. They also investigated the cost-benefits of different biochar application rates, and concluded that biochar could reduce nitrate leaching, and increase soil carbon level [243].
Advanced search is conducted, using the following keyword sets in WEB OF SCIENCETM (Thomson Reuters – ISI Web of Knowledge) to collect archival journals and peer-reviewed conference publications based on abstracts, titles, and keywords published (i.e., TS field tag) between Jan. 1, 2008, and Jan. 1, 2019. The keyword sets are used to develop two databases, including publications relating biochar applications with the food-energy-water systems (FEWS).
➢ Keyword Set 1. TS = ((Food OR Agriculture OR Land OR Soil) AND (Energy OR Carbon) AND Water AND (Sustainable OR Sustainability OR Renewable) AND Biochar).
➢ Keyword Set 2. TS = ((Food OR Agriculture OR Soil) AND (Energy OR Water OR Carbon) AND Pyrolysis AND Biochar AND (Sustainable OR Sustainability)).
The period Jan. 1, 2008, to Jan. 1, 2019 is chosen for this systematic review (SR) based on the low number of studies concerning FEWS published outside of the past ten years. Prior to 2008, there are only six and three articles published in Keyword Set 1 and Set 2, respectively. These records are dismissed from this review as prior to the World Economic Forum 2011, the nexus thinking was limited [21]. Directly searching for FEWS and biochar resulted in a database of only 17 publications that all published in 2009 or later. The query of Keyword Set 1 run through the WEB OF SCIENCE™ produced a database of 157 publications and Keyword Set 2 query produced a database of 181 publications. A comparison was conducted to find 60 papers in common between the two datasets. The results of SR for the selected keyword sets show that the research in this field is accelerating with the most rapid publication growth being seen in the last three years (Figure 3).
Table 3 presents the top ten countries in this field based on the number of publications. In both cases, the U.S. and China were the most and second-most productive, respectively, where Germany and Italy rounded out the top four of Keyword Set 1, and Australia and Italy in Keyword Set 2. The data recorded was based on the publication origin, not the author's country of origin.
Keyword set 1 | Keyword set 2 | ||||
Countries/regions | Records | % of 157 | Countries/regions | Records | % of 181 |
USA | 50 | 31.85 | USA | 51 | 28.18 |
China | 30 | 19.11 | China | 32 | 17.68 |
Germany | 18 | 11.47 | Australia | 18 | 9.95 |
Italy | 15 | 9.55 | Italy | 14 | 7.74 |
Australia | 14 | 8.92 | Germany | 13 | 7.18 |
South Korea | 10 | 6.37 | Spain | 12 | 6.63 |
India | 10 | 6.37 | Greece | 11 | 6.08 |
Scotland | 9 | 5.73 | Scotland | 10 | 5.53 |
England | 7 | 4.46 | England | 9 | 4.97 |
Greece | 6 | 3.82 | India | 9 | 4.97 |
Table 4 reports the most common research areas in each data set. The top four research areas are Environmental Sciences and Ecology with 63 and 81 publications for both sets, followed by Agriculture with 54 and 51 publications, engineering with 37 and 57 publications, and Energy Fuels with 26 and 43 publications in Set 1 and Set 2, respectively. The top nine research areas for each keyword set are the same areas, the difference being the order and number of publications in each research area.
Keyword set 1 | Keyword set 2 | ||||
Research areas | Records | % of 157 | Research areas | Records | % of 181 |
Environmental Sciences Ecology | 63 | 40.13 | Environmental Sciences Ecology | 81 | 44.75 |
Agriculture | 54 | 34.40 | Engineering | 57 | 31.49 |
Engineering | 37 | 23.57 | Agriculture | 51 | 28.18 |
Energy Fuels | 26 | 16.56 | Energy Fuels | 43 | 23.76 |
Science Technology other Topics | 16 | 10.19 | Science Technology other Topics | 31 | 17.13 |
Biotechnology Applied Microbiology | 14 | 8.92 | Biotechnology Applied Microbiology | 18 | 9.95 |
Water Resources | 13 | 8.28 | Chemistry | 17 | 9.39 |
Chemistry | 8 | 5.10 | Plant Sciences | 9 | 4.97 |
Plant Sciences | 7 | 4.46 | Water Resources | 8 | 4.42 |
Food Science Technology | 5 | 3.19 | Geology | 3 | 1.66 |
The combined keyword sets contain 278 papers, and they have been cited by 5642 articles from Jan. 1, 2008, to Jan. 1, 2019. The sum of the total times the papers have been cited each year is shown in Figure 1, in total the combined sets have been cited a sum of 8760 times. The number of publications and citations per year has increased greatly in the last three years as seen in Figures 3 and 4 with 54% of the records and 64% of the citations occurring from Jan. 2016 to Jan. 2019.
The majority of the citations for the top ten most-cited journals are attributed to the top-cited publications in the combined datasets. Table 5 lists the top-cited articles in the combined datasets. Over 95% of the total cumulative citations of the top-cited journals are from the top-cited article published by that journal. The three most cited articles are by Woolf et al. in Nature Communications with 650 citations [244], Mohan et al. in Bioresource Technology Journal with 563 citations has the highest citations per year of the top-cited papers [245], and Beesley et al. with 558 citations in Environmental Pollution [149]. Also, not far behind is Van Zwieten et al. with 536 citations published in Plant and Soil [161].
Author | Source title | Citations | Year |
Woolf et al. | Nature Communications | 650 | 2010 |
Mohan et al. | Bioresource Technology | 563 | 2014 |
Beesley et al. | Environmental Pollution | 558 | 2011 |
Van Zwieten et al. | Plant and Soil | 536 | 2010 |
Laird et al. | Geoderma | 411 | 2010 |
Steinbeiss et al. | Soil Biology and Biochemistry | 398 | 2009 |
Laird et al. | Biofuels Bioproducts and Biorefining-Biofpr | 278 | 2009 |
Kookana et al. | Advances in Agronomy | 210 | 2011 |
Regmi et al. | Journal of Environmental Management | 169 | 2012 |
Méndez et al. | Chemosphere | 166 | 2012 |
Figure 5 depicts the bibliometric map illustrating the frequency of keywords found in the top 50 most cited publications the combined datasets. VOSviewer software was used to create a density map that clustered keywords based on their relevance. The map clusters the keywords based on their frequency of use together in the publications, with the fingers of the map showing the separation of certain keywords that are less frequently used together. The map also uses color to illustrate the number of occurrences of each keyword, for this illustration a rainbow color density was used with warmer colors (red and orange), correlating to higher usage and transitions to cooler colors (green and blue) corresponding to lower usage. For the top 50 used articles in the datasets, black carbon, sustainable agriculture, soil, organic-matter, activated carbon, chemical properties, greenhouse gas emission, life-cycle assessment, and biomass were some of the most frequently used keywords. The less frequently used keywords are related to supplementary research in the biochar and FEW fields [43]. The study of the keyword occurrence was limited to the top 50 cited articles due to software limitations. Keyword length was also limited to 25 characters to clean up the visualization. Most of the keywords are related to agriculture and GHG emissions, possibly showing the increased emphasis in applications of biochar in these areas.
With the recent wave of interest in the FEW research, there are opportunities to identify potential pathways, products, and systems for future FEW research, as well as enhance the resilience and sustainability of the integrated forest-fuel-food (F3) industries across the U.S. that will benefit the current state-of-the-art in FEWS [260,261]. The earlier studies focused on each of the three FEW branches for addressing the tensions and to respond to the growing global needs. Later studies centered on life cycle assessment and integrated either two of the three FEW branches, which are well-suited for global-scale studies to respond to the FEW crises. Recent studies established field-based models to optimize nutrient-energy-water systems. A suggested form of integrated F3 processes and products for FEW complexity mitigations would be the development of biofuels and biochar from organic wastes and agricultural residues. Bioproducts creation would reduce the dependence of agriculture production on the energy sector and provide a product that can be applied to improve soil quality and crop yields, mitigating the necessity for excessive irrigation/fertigation by increasing moisture content and nutrients [262].
Biochar has yet to realize its full potential across FEWS, and it is staged to become a key player in the organic food/crop industry. Since biochar production is not an established industry in the U.S., there is a need to expand market demand for the product by educating farmers about its benefits compared to traditional crop applications and non-organic fertilizers. Commercializing biochar addresses market priorities and end-user needs, particularly in the agribusiness industry. Additionally, sustainable biochar serves as a valuable carbon sink by holding carbon in soil and displacing fossil fuels used in energy production. Sufficient cost reduction will be necessary before biochar can become a viable option to replace current synthetic products, mainly as a soil amendment for organic farming [46].
Biochar is primarily utilized in agriculture to improve overall crop productivity, necessary for supporting the livestock sector in the form of animal feed. This is generally true in North America where meat is viewed as an essential product for the human diet. Depending on the properties, biochar has widespread applications across various sectors [263]. Property variables include the heating value, energy content, carbon content, water-nutrient holding capacity, and organic-matter efficiency. Additionally, manure-based biochar is an emerging product, and the industry is in its nascent phase, but growing gradually with increased awareness and improved production processes.
Biochar developing market utilizes biomass from forest and agriculture treatments, designed to increase F3 resilience to disease and wildfire while improving water quality and quantity, and to convert biomass feedstocks into biochar that is delivered to dry land farms to boost food production, using a sustainable, energy-efficient life cycle. Ultimately, advancing cyber-human-physical systems will enable the extensive sharing of information among stakeholders and lead to emergent business-to-business and business-to-consumer relationships. Adaptive cyber-physical systems will more effectively capture systemic intricacies than traditional empirical techniques and provide more accurate ways to analyze and visualize performance characteristics.
Understanding the ramifications of nutrient-energy-water systems (e.g., complex compounds, mechanisms, multifunctional performance, and commercial viability) and elucidation of the effects of various operational parameters and variables are essential to provide a base of knowledge to enhance FEWS sustainability benefits. The need for further investigation is increasing not only in the creation of conceptual platforms, but also in empirical work for specific applications that will increase industrial growth. Narrative and systematic literature reviews have been conducted to investigate the state-of-the-art within FEW disciplines and explore the socio-economic resilience and ecological integrity of regional FEWS by coupling recent improvements. This study examines the linkages among bioproducts (particularly biochar) and FEWS to identify potential solutions that will bridge existing research gaps.
With the current focus of renewable and sustainable processes and systems research, there is an essential need for a connection to be drawn between the challenges facing the FEWS and bio-products. Therefore, an in-depth review of biochar-related prospects is presented, and the significant impacts of biochar- derived products on improving FEWS and mitigating carbon footprints are explored. The viability and implications of biochar-derived products to FEWS and associated factors (e.g., soil amendment and crop growth, water treatment, energy generation, and reduction of GHG emissions) have been discussed within the narrative review. However, questions remain on the long-term viability of biochar-amended soils, as well as the possible competition for land space if crops are being grown for biochar production. Over the last ten years, the interdisciplinary FEW research has been a fast-growing field of study due to the dearth of literature for effective strategies to improve FEWS efficiency and productivity. The systematic review used WEB OF SCIENCETM to generate two databases, including 146 and 164 articles in each database and 57 articles shared by both databases, and analyzed the various factors based on the number of publications, citations, and keywords. From both narrative and systematic reviews, it is concluded that there is an essential need for solutions-oriented projects at the FEW security nexus at both domestic and global levels.
Moving beyond current practices and techniques to promote FEWS and further breakthroughs offers the opportunity to advance existing FEW infrastructures, streamline the information, and support sustainable FEWS. Advanced FEWS play a crucial role in addressing national priorities, however, based on the disparate nature of operations and inherent complexity associated with FEW entities, it is not surprising that little work has been done to integrate all three branches. The following potential paths are defined for further investigation to advance existing FEWS at various spatiotemporal scales:
➢ Establishment of standard metrics, indicators, approaches, and computational tools for FEW life cycle analyses.
➢ Development of bioproducts classification tools by determining market opportunities and end-users.
➢ Development of techno-economic and socio-environmental studies for solutions with the potential to improve FEWS.
➢ Exploration of the biochar market and sustainability impacts associated with biochar-derived products.
The authors wish to acknowledge Ms. Debbie Lacroix for helping in biochar analysis, and financial support from University of Idaho (Equipment and Infrastructure Support Grant) and Idaho State University (Developing Collaborative Partnerships Grant), as well as the Center for Advanced Energy Studies and Idaho National Laboratory for resources and facilities, and UPM Lappeenranta, Finland for their support and resources.
All authors declare no conflict of interest.
[1] | DOE (2016) Strategic Plan for a Thriving and Sustainable Bioeconomy. 56. |
[2] |
Lin L, Xu F, Ge X, et al. (2018) Improving the sustainability of organic waste management practices in the food-energy-water nexus: A comparative review of anaerobic digestion and composting. Renew Sustain Energy Rev 89: 151-167. doi: 10.1016/j.rser.2018.03.025
![]() |
[3] | Daher B, Mohtar R (2012) Water, energy, and food: The Ultimate Nexus, Encyclopedia of Agricultural, Food, and Biological Engineering, Second Edition. |
[4] |
Albrecht TR, Crootof A, Scott CA (2018) The Water-Energy-Food Nexus: A systematic review of methods for nexus assessment. Environ Res Lett 13: 043002. doi: 10.1088/1748-9326/aaa9c6
![]() |
[5] |
Popp J, Lakner Z, Harangi-Rákos M, et al. (2014) The effect of bioenergy expansion: Food, energy, and environment. Renew Sustain Energy Rev 32: 559-578. doi: 10.1016/j.rser.2014.01.056
![]() |
[6] | Gomo FF, Macleod C, Rowan J, et al. (2018) Supporting better decisions across the nexus of water, energy and food through earth observation data: \hack\break case of the Zambezi basin. Proc Int Assoc Hydrol Sci 376: 15-23. |
[7] | Belmonte BA, Benjamin MFD, Tan RR (2017) Biochar systems in the water-energy-food nexus: the emerging role of process systems engineering. Biotechnol Bioprocess Eng Process Syst Eng 18: 32-37. |
[8] | Hansen S, Mirkouei A (2019) Bio-Oil Upgrading Via Micro-Emulsification And Ultrasound Treatment: Examples For Analysis And Discussion, ASME 2019 International Design Engineering Technical Conferences & Computers and Information in Engineering Conference. |
[9] | Hansen S, Mirkouei A, Diaz LA (2020) A Comprehensive State-of-Technology Review for Upgrading Bio-oil to Renewable or Blended Hydrocarbon Fuels. Renew Sustain Energy Rev in press. |
[10] |
Roy P, Dias G (2017) Prospects for pyrolysis technologies in the bioenergy sector: A review. Renew Sustain Energy Rev 77: 59-69. doi: 10.1016/j.rser.2017.03.136
![]() |
[11] | Mirkouei A, Haapala KR (2015) A network model to optimize upstream and midstream biomass-to-bioenergy supply chain costs, ASME 2015 International Manufacturing Science and Engineering Conference (MSEC), MSEC2015-9355. |
[12] | Mirkouei A (2016) Techno-Economic Optimization and Environmental Impact Analysis for a Mixed-Mode Upstream and Midstream Forest Biomass to Bio-Products Supply Chain. |
[13] |
Cha JS, Park SH, Jung S-C, et al. (2016) Production and utilization of biochar: A review. J Ind Eng Chem 40: 1-15. doi: 10.1016/j.jiec.2016.06.002
![]() |
[14] |
Krajačić G, Vujanović M, Duić N, et al. (2018) Integrated approach for sustainable development of energy, water and environment systems. Energy Convers Manag 159: 398-412. doi: 10.1016/j.enconman.2017.12.016
![]() |
[15] | Hugh McLaughlin (2016) An Overview of the current Biochar and Activated Carbon Markets : Biofuels Digest, 2016. Available from: https://www.biofuelsdigest.com/bdigest/2016/10/11/an-overview-of-the-current-biochar-and-activated-carbon-markets/. |
[16] | Mirkouei A (2019) Cyber-Physical Real-time Monitoring and Control for Biomass-based Energy Production, Emerging Frontiers in Industrial and Systems Engineering: Growing Research and Practice, Taylor & Francis. |
[17] | Hansen S, Mirkouei A (2018) Past Infrastructures and Future Machine Intelligence (MI) for Biofuel Production: A Review and MI-Based Framework. ASME 2018 Int Des Eng Tech Conf Comput Inf Eng Conf V004T05A022. |
[18] | Mirkouei A, Kardel K (2017) Enhance Sustainability Benefits Through Scaling-up Bioenergy Production from Terrestrial and Algae Feedstocks, Proceedings of the 2017 ASME IDETC/CIE: 22nd Design for Manufacturing and the Life Cycle Conference. |
[19] | Mirkouei A, Haapala KR, Murthy GS, et al. (2017) Evolutionary Optimization of Bioenergy Supply Chain Cost with Uncertain Forest Biomass Quality and Availability, Proceedings of the 2016 Industrial and Systems Engineering Research Conference H. Yang, Z. Kong, and MD Sarder, eds., May 21-24, Anaheim, California, USA. |
[20] | Mirkouei A, Haapala K (2014) Integration of machine learning and mathematical programming methods into the biomass feedstock supplier selection process, Proc. 24th Int. Conf. Flex. Autom. Intell. Manuf. FAIM May, 20-23. |
[21] |
Biggs EM, Bruce E, Boruff B, et al. (2015) Sustainable development and the water-energy-food nexus: A perspective on livelihoods. Environ Sci Policy 54: 389-397. doi: 10.1016/j.envsci.2015.08.002
![]() |
[22] | Wallington K, Cai X (2017) The Food-Energy-Water Nexus: A Framework to Address Sustainable Development in the Tropics. Trop Conserv Sci 10: 1940082917720665. |
[23] | GVR (2017) Biochar Market Size, 2017. Available from: https://www.marketwatch.com/press-release/biochar-market-size-worth-31461-million-by-2025-cagr-132-grand-view-research-inc-2018-05-30. |
[24] |
Jeffery S, Verheijen FGA, van der Velde M, et al. (2011) A quantitative review of the effects of biochar application to soils on crop productivity using meta-analysis. Agric Ecosyst Environ 144: 175-187. doi: 10.1016/j.agee.2011.08.015
![]() |
[25] |
Atkinson CJ, Fitzgerald JD, Hipps NA (2010) Potential mechanisms for achieving agricultural benefits from biochar application to temperate soils: a review. Plant Soil 337: 1-18. doi: 10.1007/s11104-010-0464-5
![]() |
[26] |
Ahmad M, Rajapaksha AU, Lim JE, et al. (2014) Biochar as a sorbent for contaminant management in soil and water: A review. Chemosphere 99: 19-33. doi: 10.1016/j.chemosphere.2013.10.071
![]() |
[27] |
Liu W-J, Jiang H, Yu H-Q (2015) Development of Biochar-Based Functional Materials: Toward a Sustainable Platform Carbon Material. Chem Rev 115: 12251-12285. doi: 10.1021/acs.chemrev.5b00195
![]() |
[28] |
Das O, Sarmah AK, Bhattacharyya D (2015) A sustainable and resilient approach through biochar addition in wood polymer composites. Sci Total Environ 512-513: 326-336. doi: 10.1016/j.scitotenv.2015.01.063
![]() |
[29] |
Carpenter AW, de Lannoy C-F, Wiesner MR (2015) Cellulose Nanomaterials in Water Treatment Technologies. Environ Sci Technol 49: 5277-5287. doi: 10.1021/es506351r
![]() |
[30] |
Mousa E, Wang C, Riesbeck J, et al. (2016) Biomass applications in iron and steel industry: An overview of challenges and opportunities. Renew Sustain Energy Rev 65: 1247-1266. doi: 10.1016/j.rser.2016.07.061
![]() |
[31] | Nemati M, Simard F, Fortin J-P, et al. (2015) Potential Use of Biochar in Growing Media. Soil Sci Soc Am J 14. |
[32] | Berry M, Seppalaomem O (2014) OSUAA_Biochar_MarketStudy_6_6_14.pdf. |
[33] | Delaney M (2015) Northwest Biochar commercialization strategy paper, U.S. Forest Service and the Oregon Department of Forestry. |
[34] | Ferroukhi R, Nagpal D, Alvaro Lopez-Peña, et al. (2015) Renewable Energy in the Water, Energy and Food Nexus, IREA. |
[35] | Jha P, Biswas AK, Lakaria BL, et al. (2010) Biochar in agriculture - prospects and related implications. Curr Sci 99: 1218-1225. |
[36] | Jeffery S, Bezemer TM, Cornelissen G, et al. (2013) The way forward in biochar research: targeting trade-offs between the potential wins. GCB Bioenergy 7: 1-13. |
[37] |
Gwenzi W, Musarurwa T, Nyamugafata P, et al. (2014) Adsorption of Zn2+ and Ni2+ in a binary aqueous solution by biosorbents derived from sawdust and water hyacinth (Eichhornia crassipes). Water Sci Technol 70: 1419. doi: 10.2166/wst.2014.391
![]() |
[38] |
Yoo G, Kim H, Chen J, et al. (2014) Effects of Biochar Addition on Nitrogen Leaching and Soil Structure following Fertilizer Application to Rice Paddy Soil. Soil Sci Soc Am J 78: 852-860. doi: 10.2136/sssaj2013.05.0160
![]() |
[39] |
Bucheli TD, Bachmann HJ, Blum F, et al. (2014) On the heterogeneity of biochar and consequences for its representative sampling. J Anal Appl Pyrolysis 107: 25-30. doi: 10.1016/j.jaap.2014.01.020
![]() |
[40] | Gerlach A, Schmidt HP (2014) The use of biochar in cattle farming. Biochar J 2014. |
[41] |
Xu R, Ferrante L, Hall K, et al. (2011) Thermal self-sustainability of biochar production by pyrolysis. J Anal Appl Pyrolysis 91: 55-66. doi: 10.1016/j.jaap.2011.01.001
![]() |
[42] |
Han J, Elgowainy A, Dunn JB, et al. (2013) Life cycle analysis of fuel production from fast pyrolysis of biomass. Bioresour Technol 133: 421-428. doi: 10.1016/j.biortech.2013.01.141
![]() |
[43] |
Agegnehu G, Srivastava AK, Bird MI (2017) The role of biochar and biochar-compost in improving soil quality and crop performance: A review. Appl Soil Ecol 119: 156-170. doi: 10.1016/j.apsoil.2017.06.008
![]() |
[44] | Biederman LA, Harpole WS (2012) Biochar and its effects on plant productivity and nutrient cycling: a meta-analysis. GCB Bioenergy 5: 202-214. |
[45] |
Agegnehu G, Nelson PN, Bird MI (2016) Crop yield, plant nutrient uptake and soil physicochemical properties under organic soil amendments and nitrogen fertilization on Nitisols. Soil Tillage Res 160: 1-13. doi: 10.1016/j.still.2016.02.003
![]() |
[46] |
Aldridge CA, Baker BH, Omer AR (2019) Investigation of short-term effects of winter cover crops on compaction and total soil carbon in a long-term no-till agricultural system. J Soil Water Conserv 74: 77-84. doi: 10.2489/jswc.74.1.77
![]() |
[47] |
Boateng AA, Mullen CA, Goldberg NM, et al. (2010) Sustainable production of bioenergy and biochar from the straw of high-biomass soybean lines via fast pyrolysis. Environ Prog Sustain Energy 29: 175-183. doi: 10.1002/ep.10446
![]() |
[48] |
Ali I (2014) Water Treatment by Adsorption Columns: Evaluation at Ground Level. Sep Purif Rev 43: 175-205. doi: 10.1080/15422119.2012.748671
![]() |
[49] |
Barber ST, Yin J, Draper K, et al. (2018) Closing nutrient cycles with biochar- from filtration to fertilizer. J Clean Prod 197: 1597-1606. doi: 10.1016/j.jclepro.2018.06.136
![]() |
[50] |
Shackley S, Hammond J, Gaunt J, et al. (2011) The feasibility and costs of biochar deployment in the UK. Carbon Manag 2: 335-356. doi: 10.4155/cmt.11.22
![]() |
[51] |
Sessions J, Smith D, Trippe KM, et al. (2019) Can biochar link forest restoration with commercial agriculture? Biomass Bioenergy 123: 175-185. doi: 10.1016/j.biombioe.2019.02.015
![]() |
[52] |
Rosas JG, Gómez N, Cara J, et al. (2015) Assessment of sustainable biochar production for carbon abatement from vineyard residues. J Anal Appl Pyrolysis 113: 239-247. doi: 10.1016/j.jaap.2015.01.011
![]() |
[53] | Shackley S, Carter S, Knowles T, et al. (2012) Sustainable gasification-biochar systems? A case-study of rice-husk gasification in Cambodia, Part I: Context, chemical properties, environmental and health and safety issues. Energy Policy 42: 49-58. |
[54] |
Qin K, Jensen PA, Lin W, et al. (2012) Biomass gasification behavior in an entrained flow reactor: gas product distribution and soot formation. Energy Fuels 26: 5992-6002. doi: 10.1021/ef300960x
![]() |
[55] |
Gwenzi W, Chaukura N, Noubactep C, et al. (2017) Biochar-based water treatment systems as a potential low-cost and sustainable technology for clean water provision. J Environ Manage 197: 732-749. doi: 10.1016/j.jenvman.2017.03.087
![]() |
[56] |
Zygourakis K (2017) Biochar soil amendments for increased crop yields: How to design a "designer" biochar. AIChE J 63: 5425-5437. doi: 10.1002/aic.15870
![]() |
[57] |
Suter GW (2013) Review papers are important and worth writing. Environ Toxicol Chem 32: 1929-1930. doi: 10.1002/etc.2316
![]() |
[58] |
Mirkouei A, Haapala KR, Sessions J, et al. (2017) A review and future directions in techno-economic modeling and optimization of upstream forest biomass to bio-oil supply chains. Renew Sustain Energy Rev 67: 15-35. doi: 10.1016/j.rser.2016.08.053
![]() |
[59] | Taticchi P, Garengo P, Nudurupati SS, et al. (2014) A review of decision-support tools and performance measurement and sustainable supply chain management. Int J Prod Res 1-22. |
[60] | WEF (2011) Water Security: the water-food-energy-climate nexus, Island Press. |
[61] | Franz M, Schlitz N, Schumacher KP (2017) Globalization and the water-energy-food nexus - Using the global production networks approach to analyze society-environment relations. Environ Sci Policy. |
[62] | Endo A, Tsurita I, Burnett K, et al. (2017) A review of the current state of research on the water, energy, and food nexus. Water Energy Food Nexus Asia-Pac Reg 11: 20-30. |
[63] | Venghaus S, Hake J-F (2018) Nexus thinking in current EU policies - The interdependencies among food, energy and water resources. Environ Sci Policy. |
[64] |
Bazilian M, Rogner H, Howells M, et al. (2011) Considering the energy, water and food nexus: Towards an integrated modelling approach. Energy Policy 39: 7896-7906. doi: 10.1016/j.enpol.2011.09.039
![]() |
[65] |
Leck H, Conway D, Bradshaw M, et al. (2015) Tracing the Water-Energy-Food Nexus: Description, Theory and Practice. Geogr Compass 9: 445-460. doi: 10.1111/gec3.12222
![]() |
[66] |
Al-Saidi M, Elagib NA (2017) Towards understanding the integrative approach of the water, energy and food nexus. Sci Total Environ 574: 1131-1139. doi: 10.1016/j.scitotenv.2016.09.046
![]() |
[67] | Bates B, Kundzewicz ZW, Wu S, et al. (Eds.) (2008) Climate Change and Water. Technical Paper of the Intergovernmental Panel on Climate Change, Geneva, IPCC Secretariat. |
[68] | Alexandratos N, Bruinsma J (2012) World agriculture towards 2030/2050: the 2012 revision. 160. |
[69] | Mateo-Sagasta J, Burke J (2008) Agriculture and water quality interactions: a global overview. |
[70] | Ongley ED (1996) Control of water pollution from agriculture; Chapter 1: Introduction to agricultural water pollution. |
[71] |
Wiedmann T (2018) Eutrophication's neglected drivers. Nat Sustain 1: 273-274. doi: 10.1038/s41893-018-0084-2
![]() |
[72] | Yue Y, Guo WN, Lin QM, et al. (2016) Improving salt leaching in a simulated saline soil column by three biochars derived from rice straw (Oryza sativa L.), sunflower straw (Helianthus annuus), and cow manure. J Soil Water Conserv 71: 467-475. |
[73] |
Bell JM, Schwartz R, McInnes KJ, et al. (2018) Deficit irrigation effects on yield and yield components of grain sorghum. Agric Water Manag 203: 289-296. doi: 10.1016/j.agwat.2018.03.002
![]() |
[74] | Wagner K (2012) Status and trends of irrigated agriculture in Texas. Spec Rep Tex Water Resour Inst Tex AM Univ Coll Stn Tex Tex Water Resour Inst. |
[75] |
Bordovsky JP, Mustian JT, Cranmer AM, et al. (2011) Cotton-grain sorghum rotation under extreme deficit irrigation conditions. Appl Eng Agric 27: 359-371. doi: 10.13031/2013.37079
![]() |
[76] |
Grafton RQ, Williams J, Perry CJ, et al. (2018) The paradox of irrigation efficiency. Science 361: 748-750. doi: 10.1126/science.aat9314
![]() |
[77] | Vadez V, Kholová J, Yadav RS, et al. (2013) Small temporal differences in water uptake among varieties of pearl millet (Pennisetum glaucum (L.) R. Br.) are critical for grain yield under terminal drought. Plant Soil 371: 447-462. |
[78] |
Compton M, Willis S, Rezaie B, et al. (2018) Food processing industry energy and water consumption in the Pacific northwest. Innov Food Sci Emerg Technol 47: 371-383. doi: 10.1016/j.ifset.2018.04.001
![]() |
[79] | Ralph E. H. Sims (2011) Energy-Smart Food for People and Climate. UN Food and Agriculture Organisation. |
[80] | FAO (2018) Food and Agriculture Organization of the United Nations, 2018.Available from: http://www.fao.org/home/en/. |
[81] |
Reganold JP, Wachter JM (2016) Organic agriculture in the twenty-first century. Nat Plants 2: 15221. doi: 10.1038/nplants.2015.221
![]() |
[82] |
Dong X, Vuran MC, Irmak S (2013) Autonomous precision agriculture through integration of wireless underground sensor networks with center pivot irrigation systems. Ad Hoc Netw 11: 1975-1987. doi: 10.1016/j.adhoc.2012.06.012
![]() |
[83] | Helu M, Hedberg T, Barnard Feeney A (2017) Reference architecture to integrate heterogeneous manufacturing systems for the digital thread. CIRP J Manuf Sci Technol. |
[84] | Vogl GW, Weiss BA, Helu M (2016) A review of diagnostic and prognostic capabilities and best practices for manufacturing. J Intell Manuf 1-17. |
[85] |
Wolfe ML, Ting KC, Scott N, et al. (2016) Engineering solutions for food-energy-water systems: it is more than engineering. J Environ Stud Sci 6: 172-182. doi: 10.1007/s13412-016-0363-z
![]() |
[86] |
Lehmann J, Gaunt J, Rondon M (2006) Bio-char Sequestration in Terrestrial Ecosystems - A Review. Mitig Adapt Strateg Glob Change 11: 403-427. doi: 10.1007/s11027-005-9006-5
![]() |
[87] | Napolitano G, Isaac J, Bizzarri G, et al. (2010) Bioenergy and Food Security. Food and Agriculture Organization of the United Nations. |
[88] | Khan Z, Linares P, García-González J (2017) Integrating water and energy models for policy driven applications. A review of contemporary work and recommendations for future developments. Renew Sustain Energy Rev 67: 1123-1138. |
[89] | Pate R, Hightower M, Cameron C, et al. (2007) Overview of Energy-Water Interdependencies and the emerging energy demands on Water Resources. |
[90] | Scott CA, Pierce SA, Pasqualetti MJ, et al. (2011) Policy and institutional dimensions of the water-energy nexus. Sustain Biofuels 39: 6622-6630. |
[91] |
Hamiche AM, Stambouli AB, Flazi S (2016) A review of the water-energy nexus. Renew Sustain Energy Rev 65: 319-331. doi: 10.1016/j.rser.2016.07.020
![]() |
[92] | Erik Mielke, Laura Diaz Anadon, Vankatesh Narayanamurti (2010) Water Consumption of Energy Resource Extraction, Processing, and Conversion, A review of the literature for estimates of water intensity of energy-resource extraction, processing to fuels,and conversion to electricity, Belfer Center for Science and International Affairs, Harvard University. |
[93] |
Bartos MD, Chester MV (2014) The Conservation Nexus: Valuing Interdependent Water and Energy Savings in Arizona. Environ Sci Technol 48: 2139-2149. doi: 10.1021/es4033343
![]() |
[94] |
Wu M, Mintz M, Wang M, et al. (2009) Water Consumption in the Production of Ethanol and Petroleum Gasoline. Environ Manage 44: 981. doi: 10.1007/s00267-009-9370-0
![]() |
[95] | Ernst KM, Preston BL (2017) Adaptation opportunities and constraints in coupled systems: Evidence from the U.S. energy-water nexus. Environ Sci Policy 70: 38-45. |
[96] |
Liu J, Hull V, Godfray HCJ, et al. (2018) Nexus approaches to global sustainable development. Nat Sustain 1: 466. doi: 10.1038/s41893-018-0135-8
![]() |
[97] |
Jägerskog A, Lindström A, Björklund G, et al. (2012) Regional Options for Addressing the Water, Energy and Food Nexus in Central Asia and the Aral Sea Basin AU - Granit, Jakob. Int J Water Resour Dev 28: 419-432. doi: 10.1080/07900627.2012.684307
![]() |
[98] |
Villarroel Walker R, Beck MB, Hall JW (2012) Water - and nutrient and energy - systems in urbanizing watersheds. Front Environ Sci Eng 6: 596-611. doi: 10.1007/s11783-012-0445-4
![]() |
[99] | Siddiqi A, Anadon LD (2011) The water-energy nexus in Middle East and North Africa. Crossroads Pathw Renew Nucl Energy Policy North Afr 39: 4529-4540. |
[100] | Ringler C, Bhaduri A, Lawford R (2013) The nexus across water, energy, land and food (WELF): potential for improved resource use efficiency? Aquat Mar Syst 5: 617-624. |
[101] | Lawford R, Bogardi J, Marx S, et al. (2013) Basin perspectives on the Water-Energy-Food Security Nexus. Aquat Mar Syst 5: 607-616. |
[102] |
Rasul G (2014) Food, water, and energy security in South Asia: A nexus perspective from the Hindu Kush Himalayan region☆. Environ Sci Policy 39: 35-48. doi: 10.1016/j.envsci.2014.01.010
![]() |
[103] | Stein C, Barron J, Nigussie L, et al. (2014) Advancing the water-energy-food nexus: social networks and institutional interplay in the Blue Nile, International Water Management Institute (IWMI). CGIAR Research Program. |
[104] |
Villarroel R, Beck MB, Hall JW, et al. (2014) The energy-water-food nexus: Strategic analysis of technologies for transforming the urban metabolism. J Environ Manage 141: 104-115. doi: 10.1016/j.jenvman.2014.01.054
![]() |
[105] |
Biggs EM, Bruce E, Boruff B, et al. (2015) Sustainable development and the water-energy-food nexus: A perspective on livelihoods. Environ Sci Policy 54: 389-397. doi: 10.1016/j.envsci.2015.08.002
![]() |
[106] |
Conway D, van Garderen EA, Deryng D, et al. (2015) Climate and southern Africa's water-energy-food nexus. Nat Clim Change 5: 837. doi: 10.1038/nclimate2735
![]() |
[107] | Daher BT, Mohtar RH (2015) Water-energy-food (WEF) Nexus Tool 2.0: guiding integrative resource planning and decision-making. Water Int 40: 748-771. |
[108] |
Jeswani HK, Burkinshaw R, Azapagic A (2015) Environmental sustainability issues in the food-energy-water nexus: Breakfast cereals and snacks. Sustain Prod Consum 2: 17-28. doi: 10.1016/j.spc.2015.08.001
![]() |
[109] |
Kraucunas I, Clarke L, Dirks J, et al. (2015) Investigating the nexus of climate, energy, water, and land at decision-relevant scales: the Platform for Regional Integrated Modeling and Analysis (PRIMA). Clim Change 129: 573-588. doi: 10.1007/s10584-014-1064-9
![]() |
[110] |
Mukuve FM, Fenner RA (2015) Scale variability of water, land, and energy resource interactions and their influence on the food system in Uganda. Sustain Prod Consum 2: 79-95. doi: 10.1016/j.spc.2015.07.009
![]() |
[111] |
Ozturk I (2015) Sustainability in the food-energy-water nexus: Evidence from BRICS (Brazil, the Russian Federation, India, China, and South Africa) countries. Energy 93: 999-1010. doi: 10.1016/j.energy.2015.09.104
![]() |
[112] |
Endo A, Burnett K, Orencio P, et al. (2015) Methods of the water-energy-food nexus. Water 7: 5806-5830. doi: 10.3390/w7105806
![]() |
[113] | Middleton C, Allouche J, Gyawali D, et al. (2015) The Rise and Implications of the Water-Energy-Food Nexus in Southeast Asia through an Environmental Justice Lens. |
[114] | Villamayor-Tomas S, Grundmann P, Epstein G, et al. (2015) The Water-Energy-Food Security Nexus through the Lenses of the Value Chain and the Institutional Analysis and Development Frameworks. 8: 21. |
[115] | Keskinen M, Someth P, Salmivaara A, et al. (2015) Water-Energy-Food Nexus in a Transboundary River Basin: The Case of Tonle Sap Lake, Mekong River Basin. |
[116] | Garcia DJ, You F (2016) The water-energy-food nexus and process systems engineering: A new focus. 12th Int Symp Process Syst Eng 25th Eur Symp Comput Aided Process Eng PSE-2015ESCAPE-25 31 May - 4 June 2015 Cph Den 91: 49-67. |
[117] |
Rasul G (2016) Managing the food, water, and energy nexus for achieving the Sustainable Development Goals in South Asia. Environ Dev 18: 14-25. doi: 10.1016/j.envdev.2015.12.001
![]() |
[118] |
De Laurentiis V, Hunt D, Rogers C (2016) Overcoming food security challenges within an energy/water/food nexus (EWFN) approach. Sustainability 8: 95. doi: 10.3390/su8010095
![]() |
[119] |
Cairns R, Krzywoszynska A (2016) Anatomy of a buzzword: The emergence of 'the water-energy-food nexus' in UK natural resource debates. Environ Sci Policy 64: 164-170. doi: 10.1016/j.envsci.2016.07.007
![]() |
[120] |
Yang YE, Wi S, Ray PA, et al. (2016) The future nexus of the Brahmaputra River Basin: climate, water, energy and food trajectories. Glob Environ Change 37: 16-30. doi: 10.1016/j.gloenvcha.2016.01.002
![]() |
[121] |
de Strasser L, Lipponen A, Howells M, et al. (2016) A methodology to assess the water energy food ecosystems nexus in transboundary river basins. Water 8: 59. doi: 10.3390/w8020059
![]() |
[122] |
Fasel M, Bréthaut C, Rouholahnejad E, et al. (2016) Blue water scarcity in the Black Sea catchment: Identifying key actors in the water-ecosystem-energy-food nexus. Environ Sci Policy 66: 140-150. doi: 10.1016/j.envsci.2016.09.004
![]() |
[123] |
Perrone D, Hornberger G (2016) Frontiers of the food-energy-water trilemma: Sri Lanka as a microcosm of tradeoffs. Environ Res Lett 11: 014005. doi: 10.1088/1748-9326/11/1/014005
![]() |
[124] |
Mortensen JG, González-Pinzón R, Dahm CN, et al. (2016) Advancing the Food-Energy-Water Nexus: Closing Nutrient Loops in Arid River Corridors. Environ Sci Technol 50: 8485-8496. doi: 10.1021/acs.est.6b01351
![]() |
[125] |
Wichelns D (2017) The water-energy-food nexus: Is the increasing attention warranted, from either a research or policy perspective? Environ Sci Policy 69: 113-123. doi: 10.1016/j.envsci.2016.12.018
![]() |
[126] | Endo A, Tsurita I, Burnett K, et al. (2017) A review of the current state of research on the water, energy, and food nexus. Water Energy Food Nexus Asia-Pac Reg 11: 20-30. |
[127] |
Howarth C, Monasterolo I (2017) Opportunities for knowledge co-production across the energy-food-water nexus: Making interdisciplinary approaches work for better climate decision making. Environ Sci Policy 75: 103-110. doi: 10.1016/j.envsci.2017.05.019
![]() |
[128] | Flammini A, Puri M, Pluschke L, et al. (2014) Walking the nexus talk: assessing the water-energy-food nexus in the context of the sustainable energy for all initiative, Rome, Climate, Energy and Tenure Division (NRC), Food and Agriculture Organization of the United Nations. |
[129] |
Pahl-Wostl C (2019) Governance of the water-energy-food security nexus: A multi-level coordination challenge. Environ Sci Policy 92: 356-367. doi: 10.1016/j.envsci.2017.07.017
![]() |
[130] |
El Gafy I, Grigg N, Reagan W (2017) Dynamic Behaviour of the Water-Food-Energy Nexus: Focus on Crop Production and Consumption. Irrig Drain 66: 19-33. doi: 10.1002/ird.2060
![]() |
[131] | Wicaksono A, Jeong G, Kang D (2017) Water, energy, and food nexus: Review of global implementation and simulation model development. |
[132] | Dhaubanjar S, Davidsen C, Bauer-Gottwein P (2017) Multi-Objective Optimization for Analysis of Changing Trade-Offs in the Nepalese Water-Energy-Food Nexus with Hydropower Development. Water 9. |
[133] |
Hussien WA, Memon FA, Savic DA (2017) An integrated model to evaluate water-energy-food nexus at a household scale. Environ Model Softw 93: 366-380. doi: 10.1016/j.envsoft.2017.03.034
![]() |
[134] |
Johnson OW, Karlberg L (2017) Co-exploring the Water-Energy-Food Nexus: Facilitating Dialogue through Participatory Scenario Building. Front Environ Sci 5: 24. doi: 10.3389/fenvs.2017.00024
![]() |
[135] |
White DJ, Hubacek K, Feng K, et al. (2018) The Water-Energy-Food Nexus in East Asia: A tele-connected value chain analysis using inter-regional input-output analysis. Appl Energy 210: 550-567. doi: 10.1016/j.apenergy.2017.05.159
![]() |
[136] | Albrecht TR, Crootof A, Scott CA (2018) The water-energy-food nexus: A comprehensive review of nexus-specific methods. Environ Res Lett. |
[137] | Siddiqi A, Anadon LD (2011) The water-energy nexus in Middle East and North Africa. Crossroads Pathw Renew Nucl Energy Policy North Afr 39: 4529-4540. |
[138] |
Villarroel Walker R, Beck MB, Hall JW (2012) Water - and nutrient and energy - systems in urbanizing watersheds. Front Environ Sci Eng 6: 596-611. doi: 10.1007/s11783-012-0445-4
![]() |
[139] | Ringler C, Bhaduri A, Lawford R (2013) The nexus across water, energy, land and food (WELF): potential for improved resource use efficiency? Aquat Mar Syst 5: 617-624. |
[140] |
Rasul G (2016) Managing the food, water, and energy nexus for achieving the Sustainable Development Goals in South Asia. Environ Dev 18: 14-25. doi: 10.1016/j.envdev.2015.12.001
![]() |
[141] |
Wichelns D (2017) The water-energy-food nexus: Is the increasing attention warranted, from either a research or policy perspective? Environ Sci Policy 69: 113-123. doi: 10.1016/j.envsci.2016.12.018
![]() |
[142] |
White DJ, Hubacek K, Feng K, et al. (2018) The Water-Energy-Food Nexus in East Asia: A tele-connected value chain analysis using inter-regional input-output analysis. Appl Energy 210: 550-567. doi: 10.1016/j.apenergy.2017.05.159
![]() |
[143] |
Qambrani NA, Rahman MdM, Won S, et al. (2017) Biochar properties and eco-friendly applications for climate change mitigation, waste management, and wastewater treatment: A review. Renew Sustain Energy Rev 79: 255-273. doi: 10.1016/j.rser.2017.05.057
![]() |
[144] |
Bruun EW, Müller-Stöver D, Ambus P, et al. (2011) Application of biochar to soil and N2O emissions: potential effects of blending fast-pyrolysis biochar with anaerobically digested slurry. Eur J Soil Sci 62: 581-589. doi: 10.1111/j.1365-2389.2011.01377.x
![]() |
[145] |
Laird DA (2008) The Charcoal Vision: A Win-Win-Win Scenario for Simultaneously Producing Bioenergy, Permanently Sequestering Carbon, while Improving Soil and Water Quality. Agron J 100: 178-181. doi: 10.2134/agronj2007.0161
![]() |
[146] |
Kuppusamy S, Thavamani P, Megharaj M, et al. (2016) Agronomic and remedial benefits and risks of applying biochar to soil: Current knowledge and future research directions. Environ Int 87: 1-12. doi: 10.1016/j.envint.2015.10.018
![]() |
[147] | Vanholme B, Desmet T, Ronsse F, et al. (2013) Towards a carbon-negative sustainable bio-based economy. Front Plant Sci 4: 174. |
[148] |
Tan Z, Lin CSK, Ji X, et al. (2017) Returning biochar to fields: A review. Appl Soil Ecol 116: 1-11. doi: 10.1016/j.apsoil.2017.03.017
![]() |
[149] |
Beesley L, Moreno-Jiménez E, Gomez-Eyles JL, et al. (2011) A review of biochars' potential role in the remediation, revegetation and restoration of contaminated soils. Environ Pollut 159: 3269-3282. doi: 10.1016/j.envpol.2011.07.023
![]() |
[150] | Lehmann J, Joseph S (2009) Biochar for Environmental Management: An Introduction. 12. |
[151] |
Mohan D, Pittman Charles U, Steele PH (2006) Pyrolysis of Wood/Biomass for Bio-oil: A Critical Review. Energy Fuels 20: 848-889. doi: 10.1021/ef0502397
![]() |
[152] |
Kong S-H, Loh S-K, Bachmann RT, et al. (2014) Biochar from oil palm biomass: A review of its potential and challenges. Renew Sustain Energy Rev 39: 729-739. doi: 10.1016/j.rser.2014.07.107
![]() |
[153] |
Qian K, Kumar A, Zhang H, et al. (2015) Recent advances in utilization of biochar. Renew Sustain Energy Rev 42: 1055-1064. doi: 10.1016/j.rser.2014.10.074
![]() |
[154] |
Shea EC (2014) Adaptive management: The cornerstone of climate-smart agriculture. J Soil Water Conserv 69: 198A-199A. doi: 10.2489/jswc.69.6.198A
![]() |
[155] | Tenenbaum DJ (2009) Biochar: Carbon Mitigation from the Ground Up. Environ Health Perspect 117: A70-A73. |
[156] | Liang B, Lehmann J, Solomon D, et al. (2006) Black Carbon Increases Cation Exchange Capacity in Soils. Soil Sci Soc Am J 70. |
[157] | Jeffery S, Bezemer TM, Cornelissen G, et al. (2013) The way forward in biochar research: targeting trade-offs between the potential wins. GCB Bioenergy 7: 1-13. |
[158] |
Taha SM, Amer ME, Elmarsafy AE, et al. (2014) Adsorption of 15 different pesticides on untreated and phosphoric acid treated biochar and charcoal from water. J Environ Chem Eng 2: 2013-2025. doi: 10.1016/j.jece.2014.09.001
![]() |
[159] |
Taha SM, Amer ME, Elmarsafy AE, et al. (2014) Adsorption of 15 different pesticides on untreated and phosphoric acid treated biochar and charcoal from water. J Environ Chem Eng 2: 2013-2025. doi: 10.1016/j.jece.2014.09.001
![]() |
[160] |
Liu J, Schulz H, Brandl S, et al. (2012) Short-term effect of biochar and compost on soil fertility and water status of a Dystric Cambisol in NE Germany under field conditions. J Plant Nutr Soil Sci 175: 698-707. doi: 10.1002/jpln.201100172
![]() |
[161] |
Van Zwieten L, Kimber S, Morris S, et al. (2010) Effects of biochar from slow pyrolysis of papermill waste on agronomic performance and soil fertility. Plant Soil 327: 235-246. doi: 10.1007/s11104-009-0050-x
![]() |
[162] | Kookana RS, Sarmah AK, Van Zwieten L, et al. (2011) Chapter three - Biochar Application to Soil: Agronomic and Environmental Benefits and Unintended Consequences, In: Sparks DL (Ed.), Advances in Agronomy, Academic Press, 103-143. |
[163] |
Major J, Rondon M, Molina D, et al. (2010) Maize yield and nutrition during 4 years after biochar application to a Colombian savanna oxisol. Plant Soil 333: 117-128. doi: 10.1007/s11104-010-0327-0
![]() |
[164] |
Mohammadi A, Cowie AL, Mai TLA, et al. (2017) Climate-change and health effects of using rice husk for biochar-compost: Comparing three pyrolysis systems. J Clean Prod 162: 260-272. doi: 10.1016/j.jclepro.2017.06.026
![]() |
[165] |
Mohammadi A, Cowie A, Mai TLA, et al. (2016) Biochar use for climate-change mitigation in rice cropping systems. J Clean Prod 116: 61-70. doi: 10.1016/j.jclepro.2015.12.083
![]() |
[166] |
Mohammadi A, Cowie A, Mai TLA, et al. (2016) Quantifying the greenhouse gas reduction benefits of utilising straw biochar and enriched biochar. Energy Procedia 97: 254-261. doi: 10.1016/j.egypro.2016.10.069
![]() |
[167] |
Agegnehu G, Bass AM, Nelson PN, et al. (2016) Benefits of biochar, compost and biochar-compost for soil quality, maize yield and greenhouse gas emissions in a tropical agricultural soil. Sci Total Environ 543: 295-306. doi: 10.1016/j.scitotenv.2015.11.054
![]() |
[168] |
Schimmelpfennig S, Glaser B (2012) One Step Forward toward Characterization: Some Important Material Properties to Distinguish Biochars. J Environ Qual 41: 1001-1013. doi: 10.2134/jeq2011.0146
![]() |
[169] | Kloss S, Zehetner F, Wimmer B, et al. (2013) Biochar application to temperate soils: Effects on soil fertility and crop growth under greenhouse conditions. J Plant Nutr Soil Sci 177: 3-15. |
[170] |
Zhou H, Zhang D, Wang P, et al. (2017) Changes in microbial biomass and the metabolic quotient with biochar addition to agricultural soils: A Meta-analysis. Agric Ecosyst Environ 239: 80-89. doi: 10.1016/j.agee.2017.01.006
![]() |
[171] |
Burns RG, DeForest JL, Marxsen J, et al. (2013) Soil enzymes in a changing environment: current knowledge and future directions. Soil Biol Biochem 58: 216-234. doi: 10.1016/j.soilbio.2012.11.009
![]() |
[172] |
Lehmann J, Rillig MC, Thies J, et al. (2011) Biochar effects on soil biota - A review. Soil Biol Biochem 43: 1812-1836. doi: 10.1016/j.soilbio.2011.04.022
![]() |
[173] |
Caroline A, Debode J, Vandecasteele B, et al. (2016) Biological, physicochemical and plant health responses in lettuce and strawberry in soil or peat amended with biochar. Appl Soil Ecol 107: 1-12. doi: 10.1016/j.apsoil.2016.05.001
![]() |
[174] |
Kolton M, Graber ER, Tsehansky L, et al. (2017) Biochar-stimulated plant performance is strongly linked to microbial diversity and metabolic potential in the rhizosphere. New Phytol 213: 1393-1404. doi: 10.1111/nph.14253
![]() |
[175] |
Graber ER, Frenkel O, Jaiswal AK, et al. (2014) How may biochar influence severity of diseases caused by soilborne pathogens? Carbon Manag 5: 169-183. doi: 10.1080/17583004.2014.913360
![]() |
[176] |
Jaiswal AK, Frenkel O, Elad Y, et al. (2015) Non-monotonic influence of biochar dose on bean seedling growth and susceptibility to Rhizoctonia solani: the "Shifted R max-Effect". Plant Soil 395: 125-140. doi: 10.1007/s11104-014-2331-2
![]() |
[177] |
Zhu X, Chen B, Zhu L, et al. (2017) Effects and mechanisms of biochar-microbe interactions in soil improvement and pollution remediation: a review. Environ Pollut 227: 98-115. doi: 10.1016/j.envpol.2017.04.032
![]() |
[178] |
Lal R, Smith P, Jungkunst HF, et al. (2018) The carbon sequestration potential of terrestrial ecosystems. J Soil Water Conserv 73: 145A-152A. doi: 10.2489/jswc.73.6.145A
![]() |
[179] |
Karhu K, Mattila T, Bergström I, et al. (2011) Biochar addition to agricultural soil increased CH4 uptake and water holding capacity - Results from a short-term pilot field study. Agric Ecosyst Environ 140: 309-313. doi: 10.1016/j.agee.2010.12.005
![]() |
[180] |
Bu XL, Su J, Xue JH, et al. (2019) Effect of rice husk biochar addition on nutrient leaching and microbial properties of Calcaric Cambisols. J Soil Water Conserv 74: 172-179. doi: 10.2489/jswc.74.2.172
![]() |
[181] |
Uchimiya M, Lima IM, Klasson KT, et al. (2010) Contaminant immobilization and nutrient release by biochar soil amendment: Roles of natural organic matter. Chemosphere 80: 935-940. doi: 10.1016/j.chemosphere.2010.05.020
![]() |
[182] |
Xu S, Adhikari D, Huang R, et al. (2016) Biochar-facilitated microbial reduction of hematite. Environ Sci Technol 50: 2389-2395. doi: 10.1021/acs.est.5b05517
![]() |
[183] |
Downie A, Munroe P, Cowie A, et al. (2012) Biochar as a Geoengineering Climate Solution: Hazard Identification and Risk Management. Crit Rev Environ Sci Technol 42: 225-250. doi: 10.1080/10643389.2010.507980
![]() |
[184] |
Campbell JL, Sessions J, Smith D, et al. (2018) Potential carbon storage in biochar made from logging residue: Basic principles and Southern Oregon case studies. PLOS ONE 13: e0203475. doi: 10.1371/journal.pone.0203475
![]() |
[185] |
Creamer AE, Gao B, Zhang M (2014) Carbon dioxide capture using biochar produced from sugarcane bagasse and hickory wood. Chem Eng J 249: 174-179. doi: 10.1016/j.cej.2014.03.105
![]() |
[186] |
Hasler K, Bröring S, Omta SWF, et al. (2015) Life cycle assessment (LCA) of different fertilizer product types. Eur J Agron 69: 41-51. doi: 10.1016/j.eja.2015.06.001
![]() |
[187] |
Duku MH, Gu S, Hagan EB (2011) Biochar production potential in Ghana-A review. Renew Sustain Energy Rev 15: 3539-3551. doi: 10.1016/j.rser.2011.05.010
![]() |
[188] |
Lee JW, Hawkins B, Day DM, et al. (2010) Sustainability: the capacity of smokeless biomass pyrolysis for energy production, global carbon capture and sequestration. Energy Environ Sci 3: 1695-1705. doi: 10.1039/c004561f
![]() |
[189] |
Yang Q, Han F, Chen Y, et al. (2016) Greenhouse gas emissions of a biomass-based pyrolysis plant in China. Renew Sustain Energy Rev 53: 1580-1590. doi: 10.1016/j.rser.2015.09.049
![]() |
[190] | Mirkouei A, Haapala KR, Sessions J, et al. (2016) Reducing greenhouse gas emissions for sustainable bio-oil production using a mixed supply chain, ASME 2016 International Design Engineering Technical Conferences and Computers and Information in Engineering Conference, American Society of Mechanical Engineers, V004T05A031. |
[191] | Dougherty B (2016) Biochar as a cover for dairy manure lagoons: reducing odor and gas emissions while capturing nutrients, Oregon State University. |
[192] | Stetina K (2017) Control of Fecal Malodor by Adsorption onto Biochar. |
[193] | Tsutomu I, Asada T, Kuniaki K, et al. (2004) Comparison of Removal Efficiencies for Ammonia and Amine Gases between Woody Charcoal and Activated Carbon. J Health Sci - J Health SCI 50: 148-153. |
[194] |
Parham J, Deng S, Raun W, et al. (2002) Long-term cattle manure application in soil. Biol Fertil Soils 35: 328-337. doi: 10.1007/s00374-002-0476-2
![]() |
[195] | Gasser JKR (1987) The future of animal manures as fertilizer or waste, In: Van Der Meer HG, Unwin RJ, Van Dijk TA, et al. (Eds.), Animal Manure on Grassland and Fodder Crops. Fertilizer or Waste? Proceedings of an International Symposium of the European Grassland Federation, Wageningen, The Netherlands, 31 August-3 September 1987, Dordrecht, Springer Netherlands, 259-278. |
[196] |
Kleinman PJ, Sharpley AN, Wolf AM, et al. (2002) Measuring water-extractable phosphorus in manure as an indicator of phosphorus in runoff. Soil Sci Soc Am J 66: 2009-2015. doi: 10.2136/sssaj2002.2009
![]() |
[197] | Sharpley A, Moyer B (2000) Phosphorus forms in manure and compost and their release during simulated rainfall. J Environ Qual 29: 1462-1469. |
[198] |
Heuer H, Schmitt H, Smalla K (2011) Antibiotic resistance gene spread due to manure application on agricultural fields. Curr Opin Microbiol 14: 236-243. doi: 10.1016/j.mib.2011.04.009
![]() |
[199] | Spencer JL, Guan J (2004) Public Health Implications Related to Spread of Pathogens in Manure From Livestock and Poultry Operations, In: Spencer JFT, Ragout de Spencer AL (Eds.), Public Health Microbiology: Methods and Protocols, Totowa, NJ, Humana Press, 503-515. |
[200] | You Y, Silbergeld EK (2014) Learning from agriculture: understanding low-dose antimicrobials as drivers of resistome expansion. Front Microbiol 5: 284. |
[201] |
Casey JA, Curriero FC, Cosgrove SE, et al. (2013) High-density livestock operations, crop field application of manure, and risk of community-associated methicillin-resistant Staphylococcus aureus infection in Pennsylvania. JAMA Intern Med 173: 1980-1990. doi: 10.1001/jamainternmed.2013.10408
![]() |
[202] |
Manyi-Loh CE, Mamphweli SN, Meyer EL, et al. (2016) An Overview of the Control of Bacterial Pathogens in Cattle Manure. Int J Environ Res Public Health 13: 843. doi: 10.3390/ijerph13090843
![]() |
[203] |
Nicholson FA, Groves SJ, Chambers BJ (2005) Pathogen survival during livestock manure storage and following land application. Bioresour Technol 96: 135-143. doi: 10.1016/j.biortech.2004.02.030
![]() |
[204] | Qiu M, Sun K, Jin J, et al. (2014) Properties of the plant- and manure-derived biochars and their sorption of dibutyl phthalate and phenanthrene. Sci Rep 4: 5295. |
[205] |
Batista EMCC, Shultz J, Matos TTS, et al. (2018) Effect of surface and porosity of biochar on water holding capacity aiming indirectly at preservation of the Amazon biome. Sci Rep 8: 10677. doi: 10.1038/s41598-018-28794-z
![]() |
[206] |
Brown TR, Thilakaratne R, Brown RC, et al. (2013) Techno-economic analysis of biomass to transportation fuels and electricity via fast pyrolysis and hydroprocessing. Fuel 106: 463-469. doi: 10.1016/j.fuel.2012.11.029
![]() |
[207] |
Huang Y-F, Syu F-S, Chiueh P-T, et al. (2013) Life cycle assessment of biochar cofiring with coal. Bioresour Technol 131: 166-171. doi: 10.1016/j.biortech.2012.12.123
![]() |
[208] | Bergman PCA, Boersma AR, Zwart RWR, et al. (2005) Torrefaction for biomass co-firing in existing coal-fired power stations, Energy research Centre of the Netherlands. |
[209] | Abdullah H, Wu H (2009) Biochar as a Fuel: 1. Properties and Grindability of Biochars Produced from the Pyrolysis of Mallee Wood under Slow-Heating Conditions. Energy Fuels 23: 4174-4181. |
[210] |
Yang X, Wang H, Strong P, et al. (2017) Thermal properties of biochars derived from waste biomass generated by agricultural and forestry sectors. Energies 10: 469. doi: 10.3390/en10040469
![]() |
[211] | Engineering ToolBox (2003) Classification of Coal, 2003.Available from: https://www.engineeringtoolbox.com/classification-coal-d_164.html. |
[212] |
Vikrant K, Kim K-H, Ok YS, et al. (2018) Engineered/designer biochar for the removal of phosphate in water and wastewater. Sci Total Environ 616-617: 1242-1260. doi: 10.1016/j.scitotenv.2017.10.193
![]() |
[213] |
Tan X, Liu Y, Gu Y, et al. (2016) Biochar-based nano-composites for the decontamination of wastewater: A review. Bioresour Technol 212: 318-333. doi: 10.1016/j.biortech.2016.04.093
![]() |
[214] | Sizmur T, Fresno T, Akgül G, et al. (2017) Biochar modification to enhance sorption of inorganics from water. Spec Issue Biochar Prod Charact Appl - Soil Appl 246: 34-47. |
[215] |
Husk BR, Sanchez JS, Anderson BC, et al. (2018) Removal of phosphorus from agricultural subsurface drainage water with woodchip and mixed-media bioreactors. J Soil Water Conserv 73: 265-275. doi: 10.2489/jswc.73.3.265
![]() |
[216] |
Inyang MI, Gao B, Yao Y, et al. (2016) A review of biochar as a low-cost adsorbent for aqueous heavy metal removal. Crit Rev Environ Sci Technol 46: 406-433. doi: 10.1080/10643389.2015.1096880
![]() |
[217] |
Jing X-R, Wang Y-Y, Liu W-J, et al. (2014) Enhanced adsorption performance of tetracycline in aqueous solutions by methanol-modified biochar. Chem Eng J 248: 168-174. doi: 10.1016/j.cej.2014.03.006
![]() |
[218] |
Regmi P, Garcia Moscoso JL, Kumar S, et al. (2012) Removal of copper and cadmium from aqueous solution using switchgrass biochar produced via hydrothermal carbonization process. J Environ Manage 109: 61-69. doi: 10.1016/j.jenvman.2012.04.047
![]() |
[219] |
Ahmed MB, Zhou JL, Ngo HH, et al. (2016) Progress in the preparation and application of modified biochar for improved contaminant removal from water and wastewater. Bioresour Technol 214: 836-851. doi: 10.1016/j.biortech.2016.05.057
![]() |
[220] |
DeBoe G, Bock E, Stephenson K, et al. (2017) Nutrient biofilters in the Virginia Coastal Plain: Nitrogen removal, cost, and potential adoption pathways. J Soil Water Conserv 72: 139-149. doi: 10.2489/jswc.72.2.139
![]() |
[221] |
Cao X, Ma L, Gao B, et al. (2009) Dairy-Manure Derived Biochar Effectively Sorbs Lead and Atrazine. Environ Sci Technol 43: 3285-3291. doi: 10.1021/es803092k
![]() |
[222] |
Xu X, Cheng K, Wu H, et al. (2019) Greenhouse gas mitigation potential in crop production with biochar soil amendment-a carbon footprint assessment for cross-site field experiments from China. GCB Bioenergy 11: 592-605. doi: 10.1111/gcbb.12561
![]() |
[223] | Wijitkosum S, Jiwnok P, UNISEARCH C (2019) Effect of biochar on Chinese kale and carbon storage in an agricultural area on a high rise building. |
[224] |
Shaheen A, Turaib Ali Bukhari S (2018) Potential of sawdust and corn cobs derived biochar to improve soil aggregate stability, water retention, and crop yield of degraded sandy loam soil. J Plant Nutr 41: 2673-2682. doi: 10.1080/01904167.2018.1509092
![]() |
[225] |
Sarma B, Farooq M, Gogoi N, et al. (2018) Soil organic carbon dynamics in wheat-Green gram crop rotation amended with vermicompost and biochar in combination with inorganic fertilizers: A comparative study. J Clean Prod 201: 471-480. doi: 10.1016/j.jclepro.2018.08.004
![]() |
[226] | Hersh B, Mirkouei A (2019) Life Cycle Assessment of Pyrolysis-Derived Biochar from Organic Wastes and Advanced Feedstocks, Proceedings of the ASME 2019 International Design Engineering Technical Conferencesand Computers and Information in Engineering Conference, IDETC2019-97896. |
[227] |
Uusitalo V, Leino M (2019) Neutralizing global warming impacts of crop production using biochar from side flows and buffer zones: A case study of oat production in the boreal climate zone. J Clean Prod 227: 48-57. doi: 10.1016/j.jclepro.2019.04.175
![]() |
[228] |
Thers H, Djomo SN, Elsgaard L, et al. (2019) Biochar potentially mitigates greenhouse gas emissions from cultivation of oilseed rape for biodiesel. Sci Total Environ 671: 180-188. doi: 10.1016/j.scitotenv.2019.03.257
![]() |
[229] |
Alotaibi KD, Schoenau JJ (2019) Addition of Biochar to a Sandy Desert Soil: Effect on Crop Growth, Water Retention and Selected Properties. Agronomy 9: 327. doi: 10.3390/agronomy9060327
![]() |
[230] |
Wang D, Li C, Parikh SJ, et al. (2019) Impact of biochar on water retention of two agricultural soils-A multi-scale analysis. Geoderma 340: 185-191. doi: 10.1016/j.geoderma.2019.01.012
![]() |
[231] | Ding Y, Liu Y, Liu S, et al. (2017) Potential Benefits from Biochar Application for Agricultural Use: A Review. Pedosphere. |
[232] |
Wang L, Li L, Cheng K, et al. (2018) An assessment of emergy, energy, and cost-benefits of grain production over 6 years following a biochar amendment in a rice paddy from China. Environ Sci Pollut Res 25: 9683-9696. doi: 10.1007/s11356-018-1245-6
![]() |
[233] |
Shahzad K, Abid M, Sintim HY (2018) Wheat productivity and economic implications of biochar and inorganic nitrogen application. Agron J 110: 2259-2267. doi: 10.2134/agronj2018.01.0055
![]() |
[234] |
Jalal F, Arif M, Ahmad I, et al. (2018) Increasing Farm Productivity and Soil Fertility on Sustainable Basis Through "Summer Gap" Utilization with Biochar and Legumes. Gesunde Pflanz 70: 45-53. doi: 10.1007/s10343-017-0412-x
![]() |
[235] |
Mohammadi A, Cowie AL, Cacho O, et al. (2017) Biochar addition in rice farming systems: Economic and energy benefits. Energy 140: 415-425. doi: 10.1016/j.energy.2017.08.116
![]() |
[236] |
El-Naggar A, Lee SS, Rinklebe J, et al. (2019) Biochar application to low fertility soils: A review of current status, and future prospects. Geoderma 337: 536-554. doi: 10.1016/j.geoderma.2018.09.034
![]() |
[237] |
Al-Wabel MI, Hussain Q, Usman AR, et al. (2018) Impact of biochar properties on soil conditions and agricultural sustainability: a review. Land Degrad Dev 29: 2124-2161. doi: 10.1002/ldr.2829
![]() |
[238] |
Robb S, Dargusch P (2018) A financial analysis and life-cycle carbon emissions assessment of oil palm waste biochar exports from Indonesia for use in Australian broad-acre agriculture. Carbon Manag 9: 105-114. doi: 10.1080/17583004.2018.1435958
![]() |
[239] |
Dai L, Li H, Tan F, et al. (2016) Biochar: a potential route for recycling of phosphorus in agricultural residues. Gcb Bioenergy 8: 852-858. doi: 10.1111/gcbb.12365
![]() |
[240] | Munoz C, Gongora S, Zagal E (2016) Use of biochar as a soil amendment: a brief review. Chil J Agric Anim Sci Ex Agro-Cienc 32: 37-47. |
[241] | Li W (2018) Investigating the development of the bioproducts production platform from a techno-economic and environmental perspective. |
[242] |
Ji C, Cheng K, Nayak D, et al. (2018) Environmental and economic assessment of crop residue competitive utilization for biochar, briquette fuel and combined heat and power generation. J Clean Prod 192: 916-923. doi: 10.1016/j.jclepro.2018.05.026
![]() |
[243] |
Aller DM, Archontoulis SV, Zhang W, et al. (2018) Long term biochar effects on corn yield, soil quality and profitability in the US Midwest. Field Crops Res 227: 30-40. doi: 10.1016/j.fcr.2018.07.012
![]() |
[244] |
Woolf D, Amonette JE, Street-Perrott FA, et al. (2010) Sustainable biochar to mitigate global climate change. Nat Commun 1: 56. doi: 10.1038/ncomms1053
![]() |
[245] | Mohan D, Sarswat A, Ok YS, et al. (2014) Organic and inorganic contaminants removal from water with biochar, a renewable, low cost and sustainable adsorbent - A critical review. Spec Issue Biosorption 160: 191-202. |
[246] |
Woolf D, Amonette JE, Street-Perrott FA, et al. (2010) Sustainable biochar to mitigate global climate change. Nat Commun 1: 56. doi: 10.1038/ncomms1053
![]() |
[247] | Mohan D, Sarswat A, Ok YS, et al. (2014) Organic and inorganic contaminants removal from water with biochar, a renewable, low cost and sustainable adsorbent - A critical review. Spec Issue Biosorption 160: 191-202. |
[248] |
Beesley L, Moreno-Jiménez E, Gomez-Eyles JL, et al. (2011) A review of biochars' potential role in the remediation, revegetation and restoration of contaminated soils. Environ Pollut 159: 3269-3282. doi: 10.1016/j.envpol.2011.07.023
![]() |
[249] |
Van Zwieten L, Kimber S, Morris S, et al. (2010) Effects of biochar from slow pyrolysis of papermill waste on agronomic performance and soil fertility. Plant Soil 327: 235-246. doi: 10.1007/s11104-009-0050-x
![]() |
[250] |
Laird DA, Fleming P, Davis DD, et al. (2010) Impact of biochar amendments on the quality of a typical Midwestern agricultural soil. Geoderma 158: 443-449. doi: 10.1016/j.geoderma.2010.05.013
![]() |
[251] |
Steinbeiss S, Gleixner G, Antonietti M (2009) Effect of biochar amendment on soil carbon balance and soil microbial activity. Soil Biol Biochem 41: 1301-1310. doi: 10.1016/j.soilbio.2009.03.016
![]() |
[252] |
Laird DA, Brown RC, Amonette JE, et al. (2009) Review of the pyrolysis platform for coproducing bio-oil and biochar. Biofuels Bioprod Biorefining 3: 547-562. doi: 10.1002/bbb.169
![]() |
[253] | Kookana RS, Sarmah AK, Van Zwieten L, et al. (2011) Chapter three - Biochar Application to Soil: Agronomic and Environmental Benefits and Unintended Consequences, In: Sparks DL (Ed.), Advances in Agronomy, Academic Press, 103-143. |
[254] |
Regmi P, Garcia Moscoso JL, Kumar S, et al. (2012) Removal of copper and cadmium from aqueous solution using switchgrass biochar produced via hydrothermal carbonization process. J Environ Manage 109: 61-69. doi: 10.1016/j.jenvman.2012.04.047
![]() |
[255] |
Méndez A, Gómez A, Paz-Ferreiro J, et al. (2012) Effects of sewage sludge biochar on plant metal availability after application to a Mediterranean soil. Chemosphere 89: 1354-1359. doi: 10.1016/j.chemosphere.2012.05.092
![]() |
[256] |
Laird DA, Fleming P, Davis DD, et al. (2010) Impact of biochar amendments on the quality of a typical Midwestern agricultural soil. Geoderma 158: 443-449. doi: 10.1016/j.geoderma.2010.05.013
![]() |
[257] |
Steinbeiss S, Gleixner G, Antonietti M (2009) Effect of biochar amendment on soil carbon balance and soil microbial activity. Soil Biol Biochem 41: 1301-1310. doi: 10.1016/j.soilbio.2009.03.016
![]() |
[258] |
Laird DA, Brown RC, Amonette JE, et al. (2009) Review of the pyrolysis platform for coproducing bio-oil and biochar. Biofuels Bioprod Biorefining 3: 547-562. doi: 10.1002/bbb.169
![]() |
[259] |
Méndez A, Gómez A, Paz-Ferreiro J, et al. (2012) Effects of sewage sludge biochar on plant metal availability after application to a Mediterranean soil. Chemosphere 89: 1354-1359. doi: 10.1016/j.chemosphere.2012.05.092
![]() |
[260] | Hansen S, Mirkouei A (2019) Prototyping of a Laboratory-scale Cyclone Separator for Biofuel Production from Biomass Feedstocks Using a Fused Deposition Modeling Printer, The Minerals, Metals & Materials Society (TMS) Conference Proceedings. |
[261] | Hansen S, Mirkouei A, Xian M (2019) Cyber-Physical Control and Optimization for Biofuel 4.0.Proc 2019 IISE Annu Conf H.E. Romeijn, A Schaefer, R. Thomas, eds. |
[262] |
Tripathi M, Sahu JN, Ganesan P (2016) Effect of process parameters on production of biochar from biomass waste through pyrolysis: A review. Renew Sustain Energy Rev 55: 467-481. doi: 10.1016/j.rser.2015.10.122
![]() |
[263] |
Bis Z, Koby\lecki R, Ścis\lowska M, et al. (2018) Biochar-Potential tool to combat climate change and drought. Ecohydrol Hydrobiol 18: 441-453. doi: 10.1016/j.ecohyd.2018.11.005
![]() |
1. | Liuwei Wang, Yong Sik Ok, Daniel C. W. Tsang, Daniel S. Alessi, Jörg Rinklebe, Hailong Wang, Ondřej Mašek, Renjie Hou, David O'Connor, Deyi Hou, Fiona Nicholson, New trends in biochar pyrolysis and modification strategies: feedstock, pyrolysis conditions, sustainability concerns and implications for soil amendment, 2020, 36, 0266-0032, 358, 10.1111/sum.12592 | |
2. | Elvir Tenic, Rishikesh Ghogare, Amit Dhingra, Biochar—A Panacea for Agriculture or Just Carbon?, 2020, 6, 2311-7524, 37, 10.3390/horticulturae6030037 | |
3. | Samuel Hansen, Amin Mirkouei, Luis A. Diaz, A comprehensive state-of-technology review for upgrading bio-oil to renewable or blended hydrocarbon fuels, 2020, 118, 13640321, 109548, 10.1016/j.rser.2019.109548 | |
4. | Ethan Struhs, Amin Mirkouei, Yaqi You, Amir Mohajeri, Techno-economic and environmental assessments for nutrient-rich biochar production from cattle manure: A case study in Idaho, USA, 2020, 279, 03062619, 115782, 10.1016/j.apenergy.2020.115782 | |
5. | Nor Adilla Rashidi, Suzana Yusup, 2020, Chapter 2, 978-1-78985-895-2, 10.5772/intechopen.92621 | |
6. | Érika Cristina Francisco, Paulo Sérgio de Arruda Ignácio, Alessandro Luis Piolli, Maria Ester Soares Dal Poz, Food-energy-water (FEW) nexus: Sustainable food production governance through system dynamics modeling, 2023, 386, 09596526, 135825, 10.1016/j.jclepro.2022.135825 | |
7. | Jean-Philippe Harvey, William Courchesne, Minh Duc Vo, Kentaro Oishi, Christian Robelin, Ugo Mahue, Philippe Leclerc, Alexandre Al-Haiek, Greener reactants, renewable energies and environmental impact mitigation strategies in pyrometallurgical processes: A review, 2022, 9, 2329-2229, 212, 10.1557/s43581-022-00042-y | |
8. | Yang Goh, Samantha Lauro, Steven T. Barber, Scott A. Williams, Thomas A. Trabold, Cleaner production of flexographic ink by substituting carbon black with biochar, 2021, 324, 09596526, 129262, 10.1016/j.jclepro.2021.129262 | |
9. | Matthew A. Thompson, Amir Mohajeri, Amin Mirkouei, Comparison of pyrolysis and hydrolysis processes for furfural production from sugar beet pulp: A case study in southern Idaho, USA, 2021, 311, 09596526, 127695, 10.1016/j.jclepro.2021.127695 | |
10. | Fanghua Li, Daniel J. Sweeney, Yanjun Dai, Chi-Hwa Wang, Effect of novel Ni2P-loaded catalysts on algal pyrolysis bio-oil, 2021, 151, 13640321, 111575, 10.1016/j.rser.2021.111575 | |
11. | Gricelda Herrera-Franco, Harry Alberto Bollmann, Janaina Camile Pasqual Lofhagen, Lady Bravo-Montero, Paúl Carrión-Mero, Approach on water-energy-food (WEF) nexus and climate change: A tool in decision-making processes, 2023, 46, 22114645, 100858, 10.1016/j.envdev.2023.100858 | |
12. | Zhong Hu, Lin Wei, Review on Characterization of Biochar Derived from Biomass Pyrolysis via Reactive Molecular Dynamics Simulations, 2023, 7, 2504-477X, 354, 10.3390/jcs7090354 | |
13. | Aggarwal Mohit, Neelancherry Remya, Upasana Priyadarshini, Multifaceted application of modified biochar for water and wastewater treatment, 2024, 33, 1088-1913, 945, 10.1002/tqem.22170 | |
14. | 2024, 9781119982166, 238, 10.1002/9781119982203.app2 | |
15. | Saroj Kumar Chapagain, Vishnu Prasad Pandey, Sabin Dangol, Edeltraud Guenther, Operationalizing Resource Nexus: a systematic review from water perspective, 2024, 32, 2948-1627, 10.1007/s00550-024-00553-x | |
16. | William F. Rance Bare, Ethan Struhs, Amin Mirkouei, Kenneth Overturf, Martha L. Chacón-Patiño, Amy M. McKenna, Huan Chen, Krishnan S. Raja, Controlling Eutrophication of Aquaculture Production Water Using Biochar: Correlation of Molecular Composition with Adsorption Characteristics as Revealed by FT-ICR Mass Spectrometry, 2023, 11, 2227-9717, 2883, 10.3390/pr11102883 | |
17. | Patricia Kerner, Ethan Struhs, Amin Mirkouei, Ken Aho, Kathleen A. Lohse, Robert S. Dungan, Yaqi You, Microbial Responses to Biochar Soil Amendment and Influential Factors: A Three-Level Meta-Analysis, 2023, 57, 0013-936X, 19838, 10.1021/acs.est.3c04201 | |
18. | Cristina Calderón-Tapia, Daniel Chuquín-Vasco, Alex Guambo-Galarza, Soledad Núñez-Moreno, Cristina Silva-Cisneros, Bioelectricity production from anaerobically treated leachate in microbial fuel cell using Delftia acidovorans spp., 2023, 10, 2372-0352, 847, 10.3934/environsci.2023046 | |
19. | Kishan Nandi Shoudho, Tausif Hasan Khan, Ummay Rifat Ara, Moshiur Rahman Khan, Zayed Bin Zakir Shawon, Md Enamul Hoque, Biochar in global carbon cycle: Towards sustainable development goals, 2024, 8, 26660865, 100409, 10.1016/j.crgsc.2024.100409 | |
20. | Hanyue Yang, Patricia Kerner, Xi Liang, Ethan Struhs, Amin Mirkouei, Yaqi You, Biochar amendment modulates root metabolome and rhizosphere microbiome of wheat, 2025, 7, 2524-7867, 10.1007/s42773-025-00434-6 | |
21. | Israel Valencia Quiroz, 2025, 9789815322439, 322, 10.2174/9789815322439125010017 |
Study | Research overview | Year |
Bazilian et al. | An overview of the linkages across FEW nexus was provided to develop a framework and address policy making at a national level. | 2011 |
Siddiqi and Anadon | A country-level quantitative analysis of FEW nexus was conducted to suggest possible considerations for food and water policy in the Middle East and North Africa regions. | 2011 |
Jägerskog et al. | An overview was reported to determine how regional FEW nexus centered in collaboration could benefit the economic and environmental aspects in the Central Asian and Aral Sea Basin. | 2012 |
Villarroel Walker et al. | A pros and cons analysis of adopting FEW perspectives was conducted for addressing the needs of urban areas based on case studies performed in Atlanta, GA, USA and London, UK. | 2012 |
Ringler et al. | A review and analysis of FEW Land nexus were provided, in which the resource use efficiency is the main focus. This review lays out the connections between FEW branches and gives examples of FEW Land improvement methods. | 2013 |
Lawford et al. | A summary of major factors influencing the security of FEW nexus in the Lake Winnipeg and Southern Asia region was presented. This study focused on the importance of water resources and water security across FEW nexus and nexus decisions. | 2013 |
Rasul | A literature review of FEW nexus research being conducted in South Asia region was provided. This study focused on the synergies and trade-offs, as well as the regional FEW challenges. | 2014 |
Stein et al. | This study included a quantitative method of social networks, linked to FEW nexus in Tana and Beles subbasins. | 2014 |
Villarroel Walker et al. | A quantitative analysis of FEW nexus was conducted in urban areas, utilizing a multi-sectoral system analysis to quantify resource use, synergies and antagonisms, and monetary value of FEW nexus in the London, UK region. | 2014 |
Flammini et al. | A qualitative and quantitative analysis of FEW nexus was conducted that defines FEWS interactions and evaluates the performance of FEW related policies. | 2014 |
Biggs et al. | A critical review of FEW nexus thinking was presented, which the livelihood is taken into consideration for FEW nexus decision making. This study reviewed the current literature on sustainable livelihoods and how they can be applied to improve FEWS. | 2015 |
Conway et al. | A review study with empirical data was provided to examine FEW nexus in the southern Africa region, which highlighted the climate change implications of nexus decision making. | 2015 |
Daher and Mohtar | A FEW nexus modeling tool was proposed to identify sustainable national resource allocation approaches and evaluate case studies in Qatar, Middle East. | 2015 |
Jeswani et al. | This study explored the environmental sustainability issues in FEW nexus, using life cycle assessment for breakfast cereals. | 2015 |
Kraucunas et al. | This study proposed a platform to stimulate interactions among natural and human systems at a regional scale. The conducted experiments focused on the eastern U.S. | 2015 |
Mukuve and Fenner | This study explored the stresses over FEW physical resources in Uganda's food system. It is concluded that the results help food security policy and management, particularly in Uganda. | 2015 |
Ozturk | This study examined FEW nexus for long-term sustainability in the Russian Federation, China, Brazil, South Africa, and India. | 2015 |
Endo et al. | This study collected the existing method to examine FEW nexus and classified them as quantitative or qualitative approaches. It is also discussed case studies in Japan and Philippines. | 2015 |
Middleton et al. | This study defined FEW nexus as a concept and narrative, and applied the concept to South Asia through an environmental justice lens. | 2015 |
Villamayor-Tomas et al. | The development of a FEW nexus framework was presented by combining the insights fo the Institutional Analysis and Development framework and value chain analysis. | 2015 |
Keskinen et al. | This study focused on the definition of FEW nexus, employing this definition, and using a study of the Tonle Sap system to fill a research gap in the understanding of the nexus. | 2015 |
Garcia and You | A literature review of FEW nexus was reported, highlighting FEW challenges and identifying process engineering research opportunities. | 2016 |
Rasul | A conceptual analysis of FEW nexus with emphasis on climate change was presented. This study analyzed FEW nexus in South Asia region and provided FEW decision making suggestions. | 2016 |
De Laurentiis et al. | This study reviewed food security challenges and proposed three pathways to address consequences. The concluded FEW approach is prerequisite for using the proposed pathways. | 2016 |
Cairns and Krzywoszynska | This study discussed the importance of FEW nexus research and development. It is concluded social sciences have major roles to play and may provide opportunities. | 2016 |
Yang et al. | A scenario analysis under FEW nexus thinking was presented to identify the sustainability challenges and conflicts in Brahmaputra River. Their proposed method is expected to diagnose the water resources deficiencies due to various reason, e.g., climate change. | 2016 |
de Strasser et al. | This study proposed a nexus approach for assessing a transboundary basin, impacts across sectors, and policy measures at the national level to reduce intersectoral tensions. | 2016 |
Fasel | This study focused on how FEW nexus thinking gives an innovative approach to mitigate water scarcity of the Black Sea region. | 2016 |
Perrone and Hornberger | An analysis of FEW nexus in Sri Lanka was provided to establish trade-off frontiers and illustrate the system-level tradeoffs of water allocation. | 2016 |
Mortensen et al. | A proposal was provided to employ FEW nexus policies to nutrient management in the Rio Grande region, as well as a quantitative analysis of trade-offs associated with wastewater irrigation. | 2016 |
Wichelns | A critical review was conducted to analyze FEW nexus and reported the necessity for FEW nexus thinking and decision making. | 2017 |
Endo et al. | A review study was presented on FEW related research to determine the state of nexus research in each Asia, Europe, South America, Oceania, Middle East, North America, and Africa regions. | 2017 |
Howarth and Monasterolo | This study characterized FEW nexus to understand externalities and evaluate a bottom-up, participative approach in UK, including 78 stakeholders from academia, industry, and government. | 2017 |
El Gafy et al. | This study proposed a dynamic model approach to incorporate uncertainties to FEW nexus policy making and analyze the dynamic behavior. | 2017 |
Wicaksono et al. | A literature review study focusing on the global FEW nexus was provided, including a review of nexus models and opportunities for future modeling efforts. | 2017 |
Dhaubanjar et al. | The development of a spatially explicit framework for FEW decision making was provided, in which a comparative review of Nepalese power development is analyzed. | 2017 |
Hussien et al. | The development of a bottom-up dynamic system model was presented to quantify FEW nexus at an end-use level. | 2017 |
Johnson and Karlberg | An analysis of FEW nexus tools to develop a decision making framework was conducted, including the presentation of how scenario-building creates space for dialog. | 2017 |
White et al. | A quantitative analysis of FEW nexus was performed in the East Asia region with a focus on the effect of inter-regional trade across FEWS. | 2018 |
Albrecht et al. | A systematic review of FEW nexus assessment methods was provided to analyze current FEW assessment literature to determine the repeatability of the studies, the attention to the interlinkages of the three branches and breaks down the focus of FEW assessment studies. | 2018 |
Pahl-Wostl | An analytical framework was proposed to show the potential of Sustainable Development goals in benefitting FEW nexus and analyze the governance of FEW nexus. | 2019 |
Density kg/m3 | Moisture content w.b.%* | Energy density MJ/kg | |
Green whole tree chips | 350 | 45 | 11 |
Solid wood, low density (Douglas-fir) | 400 | 12 | 17 |
Solid wood, high density (Oak) | 865 | 12 | 17 |
Bio-oil | 1200 | 25 to 30 | 18 |
Biochar (pine wood) | 350 to 500 | 5 | 28 |
Charcoal (oak) | 700 | 5 to 7 | 29 |
Coal (anthracite) | 1100–1400 | 3 to 16 | 33 |
* wet base |
Keyword set 1 | Keyword set 2 | ||||
Countries/regions | Records | % of 157 | Countries/regions | Records | % of 181 |
USA | 50 | 31.85 | USA | 51 | 28.18 |
China | 30 | 19.11 | China | 32 | 17.68 |
Germany | 18 | 11.47 | Australia | 18 | 9.95 |
Italy | 15 | 9.55 | Italy | 14 | 7.74 |
Australia | 14 | 8.92 | Germany | 13 | 7.18 |
South Korea | 10 | 6.37 | Spain | 12 | 6.63 |
India | 10 | 6.37 | Greece | 11 | 6.08 |
Scotland | 9 | 5.73 | Scotland | 10 | 5.53 |
England | 7 | 4.46 | England | 9 | 4.97 |
Greece | 6 | 3.82 | India | 9 | 4.97 |
Keyword set 1 | Keyword set 2 | ||||
Research areas | Records | % of 157 | Research areas | Records | % of 181 |
Environmental Sciences Ecology | 63 | 40.13 | Environmental Sciences Ecology | 81 | 44.75 |
Agriculture | 54 | 34.40 | Engineering | 57 | 31.49 |
Engineering | 37 | 23.57 | Agriculture | 51 | 28.18 |
Energy Fuels | 26 | 16.56 | Energy Fuels | 43 | 23.76 |
Science Technology other Topics | 16 | 10.19 | Science Technology other Topics | 31 | 17.13 |
Biotechnology Applied Microbiology | 14 | 8.92 | Biotechnology Applied Microbiology | 18 | 9.95 |
Water Resources | 13 | 8.28 | Chemistry | 17 | 9.39 |
Chemistry | 8 | 5.10 | Plant Sciences | 9 | 4.97 |
Plant Sciences | 7 | 4.46 | Water Resources | 8 | 4.42 |
Food Science Technology | 5 | 3.19 | Geology | 3 | 1.66 |
Author | Source title | Citations | Year |
Woolf et al. | Nature Communications | 650 | 2010 |
Mohan et al. | Bioresource Technology | 563 | 2014 |
Beesley et al. | Environmental Pollution | 558 | 2011 |
Van Zwieten et al. | Plant and Soil | 536 | 2010 |
Laird et al. | Geoderma | 411 | 2010 |
Steinbeiss et al. | Soil Biology and Biochemistry | 398 | 2009 |
Laird et al. | Biofuels Bioproducts and Biorefining-Biofpr | 278 | 2009 |
Kookana et al. | Advances in Agronomy | 210 | 2011 |
Regmi et al. | Journal of Environmental Management | 169 | 2012 |
Méndez et al. | Chemosphere | 166 | 2012 |
Study | Research overview | Year |
Bazilian et al. | An overview of the linkages across FEW nexus was provided to develop a framework and address policy making at a national level. | 2011 |
Siddiqi and Anadon | A country-level quantitative analysis of FEW nexus was conducted to suggest possible considerations for food and water policy in the Middle East and North Africa regions. | 2011 |
Jägerskog et al. | An overview was reported to determine how regional FEW nexus centered in collaboration could benefit the economic and environmental aspects in the Central Asian and Aral Sea Basin. | 2012 |
Villarroel Walker et al. | A pros and cons analysis of adopting FEW perspectives was conducted for addressing the needs of urban areas based on case studies performed in Atlanta, GA, USA and London, UK. | 2012 |
Ringler et al. | A review and analysis of FEW Land nexus were provided, in which the resource use efficiency is the main focus. This review lays out the connections between FEW branches and gives examples of FEW Land improvement methods. | 2013 |
Lawford et al. | A summary of major factors influencing the security of FEW nexus in the Lake Winnipeg and Southern Asia region was presented. This study focused on the importance of water resources and water security across FEW nexus and nexus decisions. | 2013 |
Rasul | A literature review of FEW nexus research being conducted in South Asia region was provided. This study focused on the synergies and trade-offs, as well as the regional FEW challenges. | 2014 |
Stein et al. | This study included a quantitative method of social networks, linked to FEW nexus in Tana and Beles subbasins. | 2014 |
Villarroel Walker et al. | A quantitative analysis of FEW nexus was conducted in urban areas, utilizing a multi-sectoral system analysis to quantify resource use, synergies and antagonisms, and monetary value of FEW nexus in the London, UK region. | 2014 |
Flammini et al. | A qualitative and quantitative analysis of FEW nexus was conducted that defines FEWS interactions and evaluates the performance of FEW related policies. | 2014 |
Biggs et al. | A critical review of FEW nexus thinking was presented, which the livelihood is taken into consideration for FEW nexus decision making. This study reviewed the current literature on sustainable livelihoods and how they can be applied to improve FEWS. | 2015 |
Conway et al. | A review study with empirical data was provided to examine FEW nexus in the southern Africa region, which highlighted the climate change implications of nexus decision making. | 2015 |
Daher and Mohtar | A FEW nexus modeling tool was proposed to identify sustainable national resource allocation approaches and evaluate case studies in Qatar, Middle East. | 2015 |
Jeswani et al. | This study explored the environmental sustainability issues in FEW nexus, using life cycle assessment for breakfast cereals. | 2015 |
Kraucunas et al. | This study proposed a platform to stimulate interactions among natural and human systems at a regional scale. The conducted experiments focused on the eastern U.S. | 2015 |
Mukuve and Fenner | This study explored the stresses over FEW physical resources in Uganda's food system. It is concluded that the results help food security policy and management, particularly in Uganda. | 2015 |
Ozturk | This study examined FEW nexus for long-term sustainability in the Russian Federation, China, Brazil, South Africa, and India. | 2015 |
Endo et al. | This study collected the existing method to examine FEW nexus and classified them as quantitative or qualitative approaches. It is also discussed case studies in Japan and Philippines. | 2015 |
Middleton et al. | This study defined FEW nexus as a concept and narrative, and applied the concept to South Asia through an environmental justice lens. | 2015 |
Villamayor-Tomas et al. | The development of a FEW nexus framework was presented by combining the insights fo the Institutional Analysis and Development framework and value chain analysis. | 2015 |
Keskinen et al. | This study focused on the definition of FEW nexus, employing this definition, and using a study of the Tonle Sap system to fill a research gap in the understanding of the nexus. | 2015 |
Garcia and You | A literature review of FEW nexus was reported, highlighting FEW challenges and identifying process engineering research opportunities. | 2016 |
Rasul | A conceptual analysis of FEW nexus with emphasis on climate change was presented. This study analyzed FEW nexus in South Asia region and provided FEW decision making suggestions. | 2016 |
De Laurentiis et al. | This study reviewed food security challenges and proposed three pathways to address consequences. The concluded FEW approach is prerequisite for using the proposed pathways. | 2016 |
Cairns and Krzywoszynska | This study discussed the importance of FEW nexus research and development. It is concluded social sciences have major roles to play and may provide opportunities. | 2016 |
Yang et al. | A scenario analysis under FEW nexus thinking was presented to identify the sustainability challenges and conflicts in Brahmaputra River. Their proposed method is expected to diagnose the water resources deficiencies due to various reason, e.g., climate change. | 2016 |
de Strasser et al. | This study proposed a nexus approach for assessing a transboundary basin, impacts across sectors, and policy measures at the national level to reduce intersectoral tensions. | 2016 |
Fasel | This study focused on how FEW nexus thinking gives an innovative approach to mitigate water scarcity of the Black Sea region. | 2016 |
Perrone and Hornberger | An analysis of FEW nexus in Sri Lanka was provided to establish trade-off frontiers and illustrate the system-level tradeoffs of water allocation. | 2016 |
Mortensen et al. | A proposal was provided to employ FEW nexus policies to nutrient management in the Rio Grande region, as well as a quantitative analysis of trade-offs associated with wastewater irrigation. | 2016 |
Wichelns | A critical review was conducted to analyze FEW nexus and reported the necessity for FEW nexus thinking and decision making. | 2017 |
Endo et al. | A review study was presented on FEW related research to determine the state of nexus research in each Asia, Europe, South America, Oceania, Middle East, North America, and Africa regions. | 2017 |
Howarth and Monasterolo | This study characterized FEW nexus to understand externalities and evaluate a bottom-up, participative approach in UK, including 78 stakeholders from academia, industry, and government. | 2017 |
El Gafy et al. | This study proposed a dynamic model approach to incorporate uncertainties to FEW nexus policy making and analyze the dynamic behavior. | 2017 |
Wicaksono et al. | A literature review study focusing on the global FEW nexus was provided, including a review of nexus models and opportunities for future modeling efforts. | 2017 |
Dhaubanjar et al. | The development of a spatially explicit framework for FEW decision making was provided, in which a comparative review of Nepalese power development is analyzed. | 2017 |
Hussien et al. | The development of a bottom-up dynamic system model was presented to quantify FEW nexus at an end-use level. | 2017 |
Johnson and Karlberg | An analysis of FEW nexus tools to develop a decision making framework was conducted, including the presentation of how scenario-building creates space for dialog. | 2017 |
White et al. | A quantitative analysis of FEW nexus was performed in the East Asia region with a focus on the effect of inter-regional trade across FEWS. | 2018 |
Albrecht et al. | A systematic review of FEW nexus assessment methods was provided to analyze current FEW assessment literature to determine the repeatability of the studies, the attention to the interlinkages of the three branches and breaks down the focus of FEW assessment studies. | 2018 |
Pahl-Wostl | An analytical framework was proposed to show the potential of Sustainable Development goals in benefitting FEW nexus and analyze the governance of FEW nexus. | 2019 |
Density kg/m3 | Moisture content w.b.%* | Energy density MJ/kg | |
Green whole tree chips | 350 | 45 | 11 |
Solid wood, low density (Douglas-fir) | 400 | 12 | 17 |
Solid wood, high density (Oak) | 865 | 12 | 17 |
Bio-oil | 1200 | 25 to 30 | 18 |
Biochar (pine wood) | 350 to 500 | 5 | 28 |
Charcoal (oak) | 700 | 5 to 7 | 29 |
Coal (anthracite) | 1100–1400 | 3 to 16 | 33 |
* wet base |
Keyword set 1 | Keyword set 2 | ||||
Countries/regions | Records | % of 157 | Countries/regions | Records | % of 181 |
USA | 50 | 31.85 | USA | 51 | 28.18 |
China | 30 | 19.11 | China | 32 | 17.68 |
Germany | 18 | 11.47 | Australia | 18 | 9.95 |
Italy | 15 | 9.55 | Italy | 14 | 7.74 |
Australia | 14 | 8.92 | Germany | 13 | 7.18 |
South Korea | 10 | 6.37 | Spain | 12 | 6.63 |
India | 10 | 6.37 | Greece | 11 | 6.08 |
Scotland | 9 | 5.73 | Scotland | 10 | 5.53 |
England | 7 | 4.46 | England | 9 | 4.97 |
Greece | 6 | 3.82 | India | 9 | 4.97 |
Keyword set 1 | Keyword set 2 | ||||
Research areas | Records | % of 157 | Research areas | Records | % of 181 |
Environmental Sciences Ecology | 63 | 40.13 | Environmental Sciences Ecology | 81 | 44.75 |
Agriculture | 54 | 34.40 | Engineering | 57 | 31.49 |
Engineering | 37 | 23.57 | Agriculture | 51 | 28.18 |
Energy Fuels | 26 | 16.56 | Energy Fuels | 43 | 23.76 |
Science Technology other Topics | 16 | 10.19 | Science Technology other Topics | 31 | 17.13 |
Biotechnology Applied Microbiology | 14 | 8.92 | Biotechnology Applied Microbiology | 18 | 9.95 |
Water Resources | 13 | 8.28 | Chemistry | 17 | 9.39 |
Chemistry | 8 | 5.10 | Plant Sciences | 9 | 4.97 |
Plant Sciences | 7 | 4.46 | Water Resources | 8 | 4.42 |
Food Science Technology | 5 | 3.19 | Geology | 3 | 1.66 |
Author | Source title | Citations | Year |
Woolf et al. | Nature Communications | 650 | 2010 |
Mohan et al. | Bioresource Technology | 563 | 2014 |
Beesley et al. | Environmental Pollution | 558 | 2011 |
Van Zwieten et al. | Plant and Soil | 536 | 2010 |
Laird et al. | Geoderma | 411 | 2010 |
Steinbeiss et al. | Soil Biology and Biochemistry | 398 | 2009 |
Laird et al. | Biofuels Bioproducts and Biorefining-Biofpr | 278 | 2009 |
Kookana et al. | Advances in Agronomy | 210 | 2011 |
Regmi et al. | Journal of Environmental Management | 169 | 2012 |
Méndez et al. | Chemosphere | 166 | 2012 |