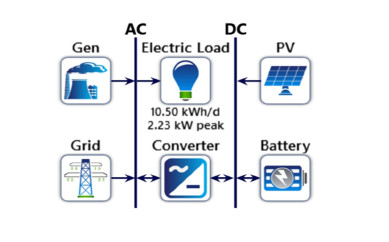
In the rural areas of Saudi Arabia, which are not connected to the national grid, electricity is supplied mainly from diesel generators. This is not just a non-renewable energy source, but it has also resulted in environmental damage and may be hazardous to human health. In order to mitigate the problem, integration with a solar photovoltaic system is proposed. A Photovoltaic-Diesel Hybrid System (PvDHS) was designed, analyzed, and optimized based on the climate data of Yanbu, Saudi Arabia. Measured local solar insolation and climate data were used in the Hybrid Optimization Model for Electric Renewables (HOMER) software with different system components and configurations in order to optimize the design that yields the best energy cost. A system consisting of a 3 kW photovoltaic system, a 2 kW diesel engine, a 1 kW converter, and 14 kWh batteries were identified to be the most cost-effective for the average daily electricity demand of 10.5 kWh. The total Net Present Cost (NPC) of this system is $17, 800, a reduction of 50% over the $35, 770 cost of the diesel-only system. The PvDHS useful electrical energy is found to be $0.36/kWh, while the Cost of Energy (COE) of the diesel-only system is $0.72/kWh. The system is expected to pay for itself in 2.8 years and reduce CO2 emissions by 8110 kg per year.
Citation: Abshir Ashour, Taib Iskandar Mohamad, Kamaruzzaman Sopian, Norasikin Ahmad Ludin, Khaled Alzahrani, Adnan Ibrahim. Performance optimization of a photovoltaic-diesel hybrid power system for Yanbu, Saudi Arabia[J]. AIMS Energy, 2021, 9(6): 1260-1273. doi: 10.3934/energy.2021058
[1] | Mulualem T. Yeshalem, Baseem Khan . Design of an off-grid hybrid PV/wind power system for remote mobile base station: A case study. AIMS Energy, 2017, 5(1): 96-112. doi: 10.3934/energy.2017.1.96 |
[2] | S. Vinoth John Prakash, P.K. Dhal . Cost optimization and optimal sizing of standalone biomass/diesel generator/wind turbine/solar microgrid system. AIMS Energy, 2022, 10(4): 665-694. doi: 10.3934/energy.2022032 |
[3] | Yassine Charabi, Sabah Abdul-Wahab . The optimal sizing and performance assessment of a hybrid renewable energy system for a mini-gird in an exclave territory. AIMS Energy, 2020, 8(4): 669-685. doi: 10.3934/energy.2020.4.669 |
[4] | Aaron St. Leger . Demand response impacts on off-grid hybrid photovoltaic-diesel generator microgrids. AIMS Energy, 2015, 3(3): 360-376. doi: 10.3934/energy.2015.3.360 |
[5] | Humaid Abdullah ALHinai, Azrul Mohd Ariffin, Miszaina Osman . Revolutionizing Oman's energy network with an optimal mixture renewable energy source. AIMS Energy, 2023, 11(4): 628-662. doi: 10.3934/energy.2023032 |
[6] | Tilahun Nigussie, Wondwossen Bogale, Feyisa Bekele, Edessa Dribssa . Feasibility study for power generation using off- grid energy system from micro hydro-PV-diesel generator-battery for rural area of Ethiopia: The case of Melkey Hera village, Western Ethiopia. AIMS Energy, 2017, 5(4): 667-690. doi: 10.3934/energy.2017.4.667 |
[7] | Nadwan Majeed Ali, Handri Ammari . Design of a hybrid wind-solar street lighting system to power LED lights on highway poles. AIMS Energy, 2022, 10(2): 177-190. doi: 10.3934/energy.2022010 |
[8] | Agus Sugiyono, Irawan Rahardjo, Prima Trie Wijaya, Afri Dwijatmiko, Aminuddin, Erwin Siregar, Silvy Rahmah Fithri, Nona Niode, Ira Fitriana . Transitioning from coal to solar: A cost-benefit analysis for sustainable power generation in Indonesia. AIMS Energy, 2024, 12(1): 152-166. doi: 10.3934/energy.2024007 |
[9] | Badr Ouhammou, Fatima Zohra Gargab, Samir El idrissi kaitouni, Slimane Smouh, Rachid El mrabet, Mohammed Aggour, Abdelmajid Jamil, Tarik Kousksou . Energy saving potential diagnosis for Moroccan university campuses. AIMS Energy, 2023, 11(3): 576-611. doi: 10.3934/energy.2023030 |
[10] | Virendra Sharma, Lata Gidwani . Optimistic use of battery energy storage system to mitigate grid disturbances in the hybrid power system. AIMS Energy, 2019, 7(6): 688-709. doi: 10.3934/energy.2019.6.688 |
In the rural areas of Saudi Arabia, which are not connected to the national grid, electricity is supplied mainly from diesel generators. This is not just a non-renewable energy source, but it has also resulted in environmental damage and may be hazardous to human health. In order to mitigate the problem, integration with a solar photovoltaic system is proposed. A Photovoltaic-Diesel Hybrid System (PvDHS) was designed, analyzed, and optimized based on the climate data of Yanbu, Saudi Arabia. Measured local solar insolation and climate data were used in the Hybrid Optimization Model for Electric Renewables (HOMER) software with different system components and configurations in order to optimize the design that yields the best energy cost. A system consisting of a 3 kW photovoltaic system, a 2 kW diesel engine, a 1 kW converter, and 14 kWh batteries were identified to be the most cost-effective for the average daily electricity demand of 10.5 kWh. The total Net Present Cost (NPC) of this system is $17, 800, a reduction of 50% over the $35, 770 cost of the diesel-only system. The PvDHS useful electrical energy is found to be $0.36/kWh, while the Cost of Energy (COE) of the diesel-only system is $0.72/kWh. The system is expected to pay for itself in 2.8 years and reduce CO2 emissions by 8110 kg per year.
Abbreviations: PvDHS: Photovoltaic-Diesel hybrid system; NPC: Net present cost; COE: Cost of energy; PV: Photovoltaic; DC: Direct current; AC: Alternating current; GHI: Global horizontal irradiance; CO2: Carbon dioxide; CF: Capacity factor; YF: Yield factor; PR: Performance ratio; CO: Carbon monoxide; SO2: Sulphur dioxide; NOx: Nitrogen oxides
Over 6 million people, or 17 percent of the population in Saudi Arabia, live in rural areas that depend on diesel generators for electricity [1]. These regions have a limited connection to the national grid. Expanding the grid to these regions is impractical due to low energy consumption, limited economic activity, and a sparse population. On the other hand, diesel generators utilization has to overcome challenges of high fuel and maintenance costs and greenhouse gas emissions over a short life span [2,3]. Numerous studies have been performed to determine the financial, technical, and environmental feasibility of utilizing renewable energy to power rural and off-grid communities [4]. Some of these studies were carried out in Jordan [5], the east coast of Saudi Arabia [6], and Nigeria [7], which all point to significant penetrations of PV systems for electricity demands. A critical review of the state-of-art PV hybrid system shows that arid climate is the most studied region when it comes to applying PV hybrid systems [8]. Solar photovoltaic systems may be installed and configured in a variety of configurations, including stand-alone, grid-connected, or hybrid designs.
Grid-connected or interactive PV systems are connected to the electrical power grid of a utility through an inverter, which converts the direct current (DC) of the power produced by the photovoltaic array to the alternating current (AC) [9,10]. Any surplus energy produced by the array is sent into the power grid, where it is credited to the consumer's account by the utility provider. When a grid-tied system has a net-metering policy in place, energy may flow both ways.
A study on stand-alone systems conducted in Iran [11] is just one example of the numerous research conducted worldwide. Stand-alone photovoltaic (PV) systems generate electricity without the need for a power grid. It is often used when a grid connection is not economically viable and accessible [12]. The photovoltaic array, inverter, charge controller, battery, and load controller are the main components of a stand-alone photovoltaic system. Solar energy becomes more cost-effective and reliable when coupled with backup power sources or integrated with another power source (hybrid system) [13,14].
In off-grid rural areas, a photovoltaic-diesel hybrid system is one of the most cost-effective options [15]. An example of a study on this can be found in [16]. Computer modeling is one technique for designing and optimizing the performance of solar photovoltaic systems. Among these modeling tools are TRANSYS [17] and Homer. HOMER modeling and simulation assists in finding the optimum design for a renewable energy system. Numerous studies have used the HOMER software to determine the optimal system confirmation and component sizing in order to improve the economics of the system [18]. HOMER has been used to predict and optimize the performance of photovoltaic systems in a variety of locales and climatic groups throughout the world, including the tropical climate of Malaysia [18], the dry climates of Libya [19] and Pakistan [20], and the humid tropical island of Sri Lanka [21]. With HOMER, the sensitivity analysis is used to evaluate a variety of operating conditions and factors, including fuel price, solar resource quality, and a variety of load sizes. Cai et al, the study of [22] examined the size of an off-grid hybrid system that included photovoltaics, a diesel generator, and a battery. The solar PV-diesel system costs 22.2 percent less than the diesel-only system and emits nearly 60 percent less greenhouse gas. In [23], the author developed the Hybrid Optimization technique, which designs and optimizes photovoltaic-diesel hybrid systems, by utilizing Genetic Algorithms. The PV and the diesel systems alone were compared, and the findings suggest that PV-diesel hybrid systems are more cost-effective and reliable. Rehman and Al-Hadhrami [24] conducted an optimization and economic analysis of a Saudi Arabian hybrid solar photovoltaic–diesel–battery system. This research demonstrates that it is technically feasible to convert some diesel generators to solar energy and positively affect rural areas.
The climatic region has significant effects on the efficiency of PV and PV hybrid systems [25]. The purpose of this research is to design and optimize a site-specific photovoltaic-diesel hybrid system (PvDHS) for usage as a power source in Yanbu, Saudi Arabia. A number of previous studies described in this section were done in Saudi Arabia, but none were done in this location. Measured local climate data fed to HOMER software is used to simulate and optimize stand-alone photovoltaic-diesel hybrid systems. The aim is to identify the best system architecture to meet the typical energy demand of small residential buildings in the remote areas surrounding Yanbu.
HOMER software is used with the input data of Yanbu, Saudi Arabia's climate information to optimize PV-diesel hybrid electrification. A search space sub-program was utilized to find the best number of batteries and the optimal PV, converter, and diesel generator size. The startup cost and operation are based on the International Renewable Energy Agency's 2018 Renewable Power Generation Costs. The designers and solar industry specialists recommended that the PV panels have a 25-year lifespan [26]. The deterioration rate of the PV cells is estimated to be 0.5 percent per year, and the system derating factor is 90 percent [27]. The simulation was based on a discount rate of 5% and an inflation rate of 2%.
Figure 1 shows a typical PV-diesel hybrid system in which PV arrays and batteries are linked to the system's DC side through an AC converter. The AC generator and grid extension are connected to the system AC side through the AC bus. The model also has a battery storage backup system aside from the PV and diesel generator power sources.
Figure 2 illustrates the monthly average solar Global Horizontal İrradiance (GHI) clearness index data. The highest radiation occurs during the June to September period with nearly 7.5 kWh/m2/day, while low radiation occurs between November and January. The average yearly radiation is 6.56 kWh/m2/day. Figures 3 (a) through (d) depicts the yearly weather conditions in the Yanbu area, which include (a) the average high and low temperatures, (b) the amount of rainfall, (c) the number of sunlight hours, and (d) the relative humidity of Saudi Arabia's western region.
The solar insolation in this location is among the greatest in the country, averaging 2400 kWh/m2/year [28]. The highest and lowest air temperatures are 40.4 ℃ and 14.2 ℃, respectively. Rainfall is very rare, with about eight days a year. Most rains happen during the cold seasons. Yanbu experiences daylight hours between 10.7 and 13.6 hours year-round. The average humidity is 545. However, during the months of high irradiation, the temperature rises, which reduces the efficiency of the PV array. As a result, the temperature of the solar cells can rise much over the standard test settings of 25 ℃ and can approach 70 ℃, as indicated in Figure 4. This high temperature causes the voltage to drop precipitously while the current increases slightly, resulting in a reduction in the amount of power produced.
The optimized sizing of solar PV systems necessitates the execution of several critical processes. The energy demand, or the quantity of energy required to power the daily load, must be thoroughly analyzed. The simulation requires a number of input data, including solar radiation, energy demand, peak load, system components, and efficiency. The electrical load is the average amount of electricity consumed by a home in this area, which is 10.5 kWh per day with a peak demand of 2.21 kW. This community's load changes from month to month, as seen in Figure 5 below.
The 3 kW solar PV system powers all loads during normal operating conditions and maintains the battery at full charge using an inverter/charger or conventional charger controller. When there is no solar PV output on cloudy days or at night, the inverter disconnects from PV and uses the energy stored in the batteries to power the load. If the battery has to be charged and the solar PV produces insufficient power, electricity is supplied by the diesel generator. The suggested PV module peak power output and efficiency are 335 W and 21.0%, respectively. The module specifications are listed in Table 1. The initial capital cost is $1300/kWh, whereas the replacement and maintenance costs are $1200 and $15. Table 1 shows the specifications of the solar panels utilized. An inverter is required to convert the DC power produced by the PV arrays to AC power. The inverter is stand-alone and has a 15-year lifespan with 95% efficiency for the inverter and 90% efficiency for the rectifier. This inverter's capital cost was $750/kW, while the replacement cost was $700. The operating and maintenance costs associated with the inverters were considered because they are so negligible.
Electrical data at standard testing conditions | |
Power (Pnom) | 335 W |
Panel efficiency | 21.0% |
Power tolerance | +5/–0% |
Rated voltage (Vmpp) | 57.3 V |
Rated current (Impp) | 5.85 A |
Open-circuit voltage (Voc) | 67.9 V |
Short-circuit current (Isc) | 6.23 A |
Power temp coefficient | –0.30%/℃ |
Voltage temp coefficient | –167.4 mV/℃ |
Current temp coefficient | 3.5 mA/℃ |
As a backup supply, the diesel generator supplements the PV power source. The generator capacity was set at 2 kW in this simulation. The initial cost was $1000/kWh, with an $800/kWh replacement cost and a $0.040/kWh maintenance cost.This is because a solar PV power generation is intermittent and a generator capacity is limited, hybrid solar PV-diesel systems work best when combined with energy storage devices. This model uses Trojan 6 V deep-cycle lead-acid batteries. The nominal maximum capacity of this battery is 2.37 kWh and 396 Ah. The stage of charge is in the range of 30% to 100%, and it has a round-trip efficiency of 85%. The lifetime of the battery is ten years, and the lifetime throughput is 1075 kWh.
The PV panels must be perpendicular to the sun and clear of shadows to capture the most energy. As shown in Figure 6, several different tilt angles and azimuth angles were compared. This study employed an azimuth angle of 0° due south and a tilted angle of 25°, which is nearly equivalent to the project site's latitude (Yanbu).
The simulation results show that a hybrid solar PV-diesel with battery storage is the best solution for supplying the desired load. Three configurations were simulated; PV-diesel generator battery (PV-G-B), PV battery (PV-B), and diesel generator alone (G). In order to meet the required load, the sizes of each component were varied, and the energy cost and renewable energy were calculated. As shown in Table 2, the optimum combination with the lowest net present cost consists of a 3-kW PV, 2-kW generator, six batteries, and a 1-kW converter. The optimum PV-diesel-battery hybrid system costs $7, 450.00, which includes the PV, generator, converter, and related design installations. Operational cost is $7.60/kWh, and NPC is $17270. These results in the lowest COE of $0.366/kWh, while PV contributes 84% of the load demand. The annual production of the PV-G-B system summed up to 4, 716 kWh, with a capacity factor of around 18%.
PV (kW) | Gen (kW) | Battery (kWh) | Converter (kW) | Initial cost ($) | Operating cost ($/kWh) | Total NPC ($) | COE ($/kWh) | Renewable % | |
PV-G-B | 3 | 2 | 6 | 1 | 7450 | 7.60 | 17270 | 0.366 | 84 |
PV-B | 5 | 18 | 2 | 13400 | 5.90 | 21, 024 | 0.451 | 100 | |
G | 2 | 1000 | 2.68 | 35, 635 | 0.755 | 0 |
Figure 7 shows the average monthly electric load share of the PV and diesel generator. This graph indicated that the PV components could provide all power demand in January, February, March, April, and November. The diesel generator is needed to supplement the PV in order to meet the need for power during the other months.The renewable fraction is 84% out of the 5318 kWh produced in a year.
Figure 8 shows the monthly PV electric production versus electrical load. Between January and April, and in November, the power production from PV exceeds the load. These are due to the fact that during the cold months, power demand by air-conditioning is reduced significantly, while solar radiation remains relatively high. To provide a more detailed insight of the power production-consumption on a weekly basis, Figure 9 depicts a solar PV output and consumption in the last week of April and early May, where the load starts to surpass the PV power output. Additionally, it demonstrates that throughout the early and late hours of daylight, the PV power output exceeds the instantaneous loads.
Figure 10 demonstrates the system's Present Net Cost, separated down by cost category. According to this graph, the battery has the greatest net present cost of the system, followed by the PV and the diesel generator. Capital and replacement cost made up the majority of NPC. The PV panels take about 50% of the capital cost, but the replacement cost is 70% battery-related. The operating cost is less than a quarter of capital and replacement costs and mainly constitute battery and diesel generators. Fuel cost is about $2000, and the salvage cost is at the negative spectrum. The sum of NPC for this PV-DHS is $17800.
Figure 11 presents a cash flow comparison of the hybrid and conventional systems over the expected 25 years life span. It shows that during the infancy stage, even though the initial cost of a diesel-only system is way lower than the PVDHS, the later system is significantly less costly to run throughout the simulated period. The hybrid system's operating costs increase significantly on the fifth, tenth, and twenty-third years of life of the system due to battery and photovoltaic module replacement, although this is still less than the running expenses of a diesel-only system.
Figure 12 displays a cash flow and revenue projection of the hybrid system for a period of 25 years, grouped by component and cost type. The net present cost and energy cost of the PV-diesel hybrid system are determined to be 50% cheaper than that of diesel alone. These results pointed out that the payback period is 2.8 years with a 30% internal rate of return.
Sensitivity analysis and repeated optimization were used to detect uncertainties and evaluate the simulation's unexpected behavior when fuel prices, photovoltaic efficiency losses, equipment prices, and environmental factors varied [29]. For example, high ambient temperatures decrease PV output voltage by 10%, while soiling can reduce output current by 10–30% [30]. On the other hand, Diesel generators have a cheap capital cost but a high fuel cost, which significantly impacts their adoption. In Saudi Arabia, diesel fuel prices have varied from $0.25/Liter to $0.58/liter [31]. A sensitivity analysis was conducted on the gasoline price, with values ranging from $0.25/Liter to $1.0/Liter in increments of $0.25/Liter examined. The grid expansion costs are estimated in this study using the software's default parameters. The initial construction cost, operating, and maintenance expenses per kilometer are $8, 000 and $45 per year, respectively, assuming a grid power purchase rate of $0.048/kWh. A 15% tax is being considered. According to the model's assumptions, the predicted photovoltaic hybrid system will have a CF of between 18 and 25%. This means the 3 kW PV Plant would generate between 4716 and 5, 524 kWh of energy per year. The optimization indicated that grid extension is preferable or break-even when the grid connecting point is within 1.07 kilometers, as shown in Figure 13.
When fossil fuels are burned, greenhouse gases are released into the Earth's atmosphere. Diesel generators use the combustion of fossil fuel. The simulation yields that PVDHS can avoid between 8110 and 11050 kg of CO2 per year. Table 3 compares the PVDHS emissions with those of a dedicated diesel generator system.
Emissions | Hybrid system (kg/year) | Diesel system (kg/year) |
carbon dioxide | 1740 | 9850 |
carbon monoxide | 4.29 | 23.84 |
unburned hydrocarbons | 0.476 | 2.66 |
particulate matter | 0.324 | 17.94 |
sulfur dioxide | 4.24 | 23.55 |
nitrogen oxides | 38.3 | 212.77 |
A photovoltaic-diesel hybrid electrification system was developed based on Yanbu, Saudi Arabia's climate data, to serve the grid-disconnected rural areas of this region, in which electricity is supplied mainly by diesel generators. The aim is to decrease reliance on diesel generators and increase the use of green buildings, which minimize air pollution associated with diesel combustion and provide a more reliable power system. HOMER software was utilized in the design, analysis, and optimization. The system should serve a daily electrical load of 10.5 kWh with a peak demand of 2.21 kW. The architecture of the optimized PV hybrid system incorporates 3 kW solar PV, 2 kW diesel generators, a 1 kW power converter, and 14.2 kWh batteries. The system produces 5957 kWh per year. The solar photovoltaic component can produce 80% of total energy, leaving the diesel generator component to provide 20%. Although the hybrid system has a greater initial capital cost of $7450 than the diesel-only system ($1000), the NPC of $17, 800 is much less than the diesel-only system NPC of $35, 770. The system will pay for itself in less than three years, and it will reduce CO2 emissions by 8110 kg per year, which is a significant reduction.
Financial support by the UKM through research funding of Fundamental Research Grant Scheme (FRGS) (FRGS/1/2019/TK07/UKM/02/4) is gratefully acknowledged.
[1] | Saudi Arabia-Urban Issues (2020) Available from: https://unhabitat.org/saudi-arabia-urban-issues. |
[2] |
Bawah U, Addoweesh KE, Eltamaly AM (2013) Comparative study of economic viability of rural electrification using renewable energy resources versus the diesel generator option in Saudi Arabia. J Renewable Sustainable Energy 5: 042701. doi: 10.1063/1.4812646
![]() |
[3] |
Ghenai C, Bettayeb M, Brdjanin B, et al. (2019) Hybrid solar PV/PEM fuel Cell/Diesel generator power system for cruise ships: A case study in Stockholm, Sweden. Case Stud in Therm Eng 14: 100497. doi: 10.1016/j.csite.2019.100497
![]() |
[4] |
Post HN, Thomas MG (1988) Photovoltaic systems for current and future applications. Sol Energy 41: 465-473. doi: 10.1016/0038-092X(88)90020-5
![]() |
[5] |
Mahmoud M (1990) Experience results and techno-economic feasibility of using photovoltaic generators instead of diesel motors for water pumping from rural desert wells in Jordan. IEE Proc C Gener, Transm Distrib 137: 391-394. doi: 10.1049/ip-c.1990.0053
![]() |
[6] |
Shaahid SM, Elhadidy MA (2007) Technical and economic assessment of grid-independent hybrid photovoltaic‑diesel‑battery power systems for commercial loads in desert environments. Renewable Sustainable Energy Rev 11: 1794-1810. doi: 10.1016/j.rser.2006.03.001
![]() |
[7] |
Oparaku OU (2002) Assessment of the cost-effectiveness of photovoltaic systems for telecommunications in Nigeria. Int J Sol Energy 22: 123-129. doi: 10.1080/0142591031000094551
![]() |
[8] |
Mazzeo D, Matera N, De Luca P, et al. (2021) A literature review and statistical analysis of photovoltaic-wind hybrid renewable system research by considering the most relevant 550 articles: An upgradable matrix literature database. J Cleaner Prod 295: 126070. doi: 10.1016/j.jclepro.2021.126070
![]() |
[9] | Ball GJ (2016) Grid-Connected PV systems, In Reinders A., Verlinden P., Sark W. and Freundlich A., Photovoltaic Solar Energy. Wiley Online Library, 511-529. Available from: https://doi.org/10.1002/9781118927496.ch46. |
[10] | Stapleton G, Neill S (2012) Grid-connected solar electric systems: The earthscan expert handbook for planning, design and ınstallation, 1st Ed., Routledge, ISBN 9780367787486. |
[11] |
Gharibi M, Askarzadeh A (2019) Size and power exchange optimization of a grid-connected diesel generator-photovoltaic-fuel cell hybrid energy system considering reliability, cost and renewability. Int J Hydrogen Energy 44: 25428-25441. doi: 10.1016/j.ijhydene.2019.08.007
![]() |
[12] | Hankins M (2010) Stand-alone solar electric systems: The earthscan expert handbook for planning, design and ınstallation, 1st Ed. ISBN 9780367787509. |
[13] |
Hocaoğlu FO, Gerek ÖN, Kurban M (2019) A novel hybrid (wind-photovoltaic) system sizing procedure. Sol Energy 83: 2019-2028. doi: 10.1016/j.solener.2009.07.010
![]() |
[14] |
Smaoui M, Abdelkafi A, Krichen L (2015) Optimal sizing of stand-alone photovoltaic/wind/hydrogen hybrid system supplying a desalination unit. Sol Energy 120: 263-276. doi: 10.1016/j.solener.2015.07.032
![]() |
[15] |
Bahramara S, Parsa Moghaddam M, Haghifam MR (2016) Optimal planning of hybrid renewable energy systems using HOMER: A review. Renewable Sustainable Energy Rev 62: 609-620. doi: 10.1016/j.rser.2016.05.039
![]() |
[16] |
Kaabeche A, Ibtiouen R (2014) Techno-economic optimization of hybrid photovoltaic/wind/diesel/battery generation in a stand-alone power system. Sol Energy 103: 171-182. doi: 10.1016/j.solener.2014.02.017
![]() |
[17] |
Mazzeo D, Matera N, Bevilacqua P, et al. (2015) Energy and economic analysis of solar photovoltaic plants located at the university of calabria. Int J Heat Tech 33: 41-50. doi: 10.18280/ijht.330406
![]() |
[18] | Abdul Razak N, Othman M, Musirin I (2010) Optimal sizing and operational strategy of hybrid renewable energy system using homer. 2010 4th International Power Engineering and Optimization Conference (PEOCO), Shah Alam, Malaysia: IEEE, 495-501. Available from: https://doi.org/10.1109/PEOCO.2010.5559240. |
[19] | Fulzele JB, Dutt S (2011) Optimium planning of hybrid renewable energy system using HOMER. Int J Electrical Com Eng 2: 68-74. |
[20] | Abbas MK, Qadeer-ul-Hasan (2015) Economic power generation for an off-grid site in Pakistan. Power Gener Syst Renewable Energy Tech: 1-6. |
[21] | Givler T, Lilienthal P (2005) Using HOMER software, NREL's micropower optimization model, to explore the role of gen-sets in small solar power systems; case study: Sri Lanka. |
[22] |
Cai W, Li X, Maleki A, et al. (2020) Optimal sizing and location based on economic parameters for an off-grid application of a hybrid system with photovoltaic, battery and diesel technology. Energy 201: 117480. doi: 10.1016/j.energy.2020.117480
![]() |
[23] |
Dufo-López R, Bernal-Agustín JL (2005) Design and control strategies of PV-Diesel systems using genetic algorithms. Sol Energy 79: 33-46. doi: 10.1016/j.solener.2004.10.004
![]() |
[24] |
Rehman S, Al-Hadhrami LM (2010) Study of a solar PV-diesel-battery hybrid power system for a remotely located population near Rafha, Saudi Arabia. Energy 35: 4986-4995. doi: 10.1016/j.energy.2010.08.025
![]() |
[25] |
Mazzeo D, Matera N, Luca PD, et al. (2020) Worldwide geographical mapping and optimization of stand-alone and grid-connected hybrid renewable system techno-economic performance across Köppen-Geiger climates. Appl Energy 276: 115507. doi: 10.1016/j.apenergy.2020.115507
![]() |
[26] |
Jordan DC, Kurtz SR (2013) Photovoltaic degradation rates—an analytical review. Prog in Photovoltaics: Res Appl 21: 12-29. doi: 10.1002/pip.1182
![]() |
[27] | Jordan, D. C., and S. R. Kurtz. 2013. "Photovoltaic Degradation Rates-an Analytical Review." Prog in Photovoltaics: Res Appl. Available from: https://doi.org/10.1002/pip.1182. |
[28] |
Zell E, Gasim S, Wilcox S, et al. (2015) Assessment of solar radiation resources in Saudi Arabia. Sol Energy 119: 422-438. doi: 10.1016/j.solener.2015.06.031
![]() |
[29] |
Rezzouk H, Mellit A (2015) Feasibility study and sensitivity analysis of a stand-alone photovoltaic‑diesel‑battery hybrid energy system in the north of Algeria. Renewable Sustainable Energy Rev 43: 1134-1150. doi: 10.1016/j.rser.2014.11.103
![]() |
[30] | Benghanem M, Almohammedi A, Khan MT, et al. (2018) Effect of dust accumulation on the performance of photovoltaic panels in desert countries: A case study for Madinah, Saudi Arabia. Int J Power Electronics Drive Syst 9: 1356-1366. |
[31] | Saudi Arabia Gasoline Prices. Ministry of Energy, Industry and Mineral Resources, 2021. Available from: https://tradingeconomics.com/saudi-arabia/gasoline-prices. |
1. | Richard Cartland, Al-Mas Sendegeya, Jean de Dieu Khan Hakizimana, Performance Analysis of a Hybrid of Solar Photovoltaic, Genset, and Hydro of a Rural-Based Power Mini-Grid: Case Study of Kisiizi Hydro Power Mini-Grid, Uganda, 2023, 11, 2227-9717, 175, 10.3390/pr11010175 |
Electrical data at standard testing conditions | |
Power (Pnom) | 335 W |
Panel efficiency | 21.0% |
Power tolerance | +5/–0% |
Rated voltage (Vmpp) | 57.3 V |
Rated current (Impp) | 5.85 A |
Open-circuit voltage (Voc) | 67.9 V |
Short-circuit current (Isc) | 6.23 A |
Power temp coefficient | –0.30%/℃ |
Voltage temp coefficient | –167.4 mV/℃ |
Current temp coefficient | 3.5 mA/℃ |
PV (kW) | Gen (kW) | Battery (kWh) | Converter (kW) | Initial cost ($) | Operating cost ($/kWh) | Total NPC ($) | COE ($/kWh) | Renewable % | |
PV-G-B | 3 | 2 | 6 | 1 | 7450 | 7.60 | 17270 | 0.366 | 84 |
PV-B | 5 | 18 | 2 | 13400 | 5.90 | 21, 024 | 0.451 | 100 | |
G | 2 | 1000 | 2.68 | 35, 635 | 0.755 | 0 |
Emissions | Hybrid system (kg/year) | Diesel system (kg/year) |
carbon dioxide | 1740 | 9850 |
carbon monoxide | 4.29 | 23.84 |
unburned hydrocarbons | 0.476 | 2.66 |
particulate matter | 0.324 | 17.94 |
sulfur dioxide | 4.24 | 23.55 |
nitrogen oxides | 38.3 | 212.77 |
Electrical data at standard testing conditions | |
Power (Pnom) | 335 W |
Panel efficiency | 21.0% |
Power tolerance | +5/–0% |
Rated voltage (Vmpp) | 57.3 V |
Rated current (Impp) | 5.85 A |
Open-circuit voltage (Voc) | 67.9 V |
Short-circuit current (Isc) | 6.23 A |
Power temp coefficient | –0.30%/℃ |
Voltage temp coefficient | –167.4 mV/℃ |
Current temp coefficient | 3.5 mA/℃ |
PV (kW) | Gen (kW) | Battery (kWh) | Converter (kW) | Initial cost ($) | Operating cost ($/kWh) | Total NPC ($) | COE ($/kWh) | Renewable % | |
PV-G-B | 3 | 2 | 6 | 1 | 7450 | 7.60 | 17270 | 0.366 | 84 |
PV-B | 5 | 18 | 2 | 13400 | 5.90 | 21, 024 | 0.451 | 100 | |
G | 2 | 1000 | 2.68 | 35, 635 | 0.755 | 0 |
Emissions | Hybrid system (kg/year) | Diesel system (kg/year) |
carbon dioxide | 1740 | 9850 |
carbon monoxide | 4.29 | 23.84 |
unburned hydrocarbons | 0.476 | 2.66 |
particulate matter | 0.324 | 17.94 |
sulfur dioxide | 4.24 | 23.55 |
nitrogen oxides | 38.3 | 212.77 |