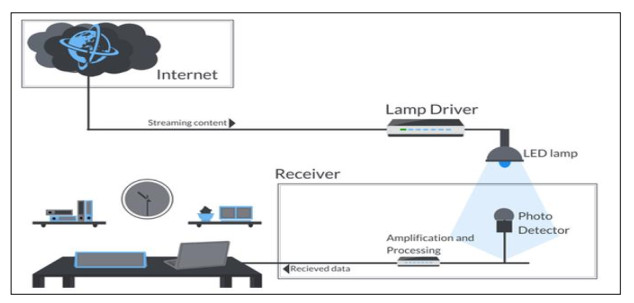
The Internet of Things (IoT) has an ever more significant function in many areas of our modern world. It improves the overall quality of life, where almost everything, including objects and devices, can be linked to the cloud and thus be hyper-connected. Within this changing IoT system, this paper focuses on data transfer, emphasizing the critical role of light fidelity (LiFi) technology. LiFi technology is an up-and-coming technology that makes use of light-emitting diodes (LEDs) and photodetectors (PDs) as transmitters and receivers (RX), respectively, for concurrent data communications and lighting. This paper proposes a single-input multiple-output IoT-based LiFi system using an LED array, phototransistor, and ambient light sensor-based RXS for simultaneous data transmission. DHT11 sensor is used to measure the environmental conditions and store collected data via Wi-Fi in the cloud server using HTTP protocol. Through indoor LiFi communication, the cloud streaming data is transferred using pulse position modulation (PPM) at a frequency of 2 KHz. The experimental results illustrate transferring and receiving data successfully at a communication distance of 1 meter. MATLAB software is used to evaluate the performance of the spatial radiance pattern and illuminance intensity of an LED Array used and received power depending on a line-of-sight (LOS) channel.
Citation: Mostafa A.R. Eltokhy, Mohamed Abdel-Hady, Ayman Haggag, Hisham A. Hamad, Tarek Hosny, Ahmed A. F. Youssef, Ali M. El-Rifaie. An indoor IoT-based LiFi system using LEDs for sensor data transfer[J]. AIMS Electronics and Electrical Engineering, 2025, 9(2): 118-138. doi: 10.3934/electreng.2025007
[1] | Lawrence O. Aghenta, M. Tariq Iqbal . Design and implementation of a low-cost, open source IoT-based SCADA system using ESP32 with OLED, ThingsBoard and MQTT protocol. AIMS Electronics and Electrical Engineering, 2020, 4(1): 57-86. doi: 10.3934/ElectrEng.2020.1.57 |
[2] | Cherechi Ndukwe, M. Tariq Iqbal, Xiaodong Liang, Jahangir Khan, Lawrence Aghenta . LoRa-based communication system for data transfer in microgrids. AIMS Electronics and Electrical Engineering, 2020, 4(3): 303-325. doi: 10.3934/ElectrEng.2020.3.303 |
[3] | B Naresh Kumar, Jai Sukh Paul Singh . Intelligence-based optimized cognitive radio routing for medical data transmission using IoT. AIMS Electronics and Electrical Engineering, 2022, 6(3): 223-246. doi: 10.3934/electreng.2022014 |
[4] | Edwin Collado, Euribiel Valdés, Antony García, Yessica Sáez . Design and implementation of a low-cost IoT-based agroclimatic monitoring system for greenhouses. AIMS Electronics and Electrical Engineering, 2021, 5(4): 251-283. doi: 10.3934/electreng.2021014 |
[5] | Efe Francis Orumwense, Khaled Abo-Al-Ez . Internet of Things for smart energy systems: A review on its applications, challenges and future trends. AIMS Electronics and Electrical Engineering, 2023, 7(1): 50-74. doi: 10.3934/electreng.2023004 |
[6] | Sanjay Dubey, M. C. Chinnaiah, I. A. Pasha, K. Sai Prasanna, V. Praveen Kumar, R. Abhilash . An IoT based Ayurvedic approach for real time healthcare monitoring. AIMS Electronics and Electrical Engineering, 2022, 6(3): 329-344. doi: 10.3934/electreng.2022020 |
[7] | K.V. Dhana Lakshmi, P.K. Panigrahi, Ravi kumar Goli . Machine learning assessment of IoT managed microgrid protection in existence of SVC using wavelet methodology. AIMS Electronics and Electrical Engineering, 2022, 6(4): 370-384. doi: 10.3934/electreng.2022022 |
[8] | Lidong Wang, Cheryl Ann Alexander . Cyber security during the COVID-19 pandemic. AIMS Electronics and Electrical Engineering, 2021, 5(2): 146-157. doi: 10.3934/electreng.2021008 |
[9] | Minglong Zhang, Iek Cheong Lam, Arun Kumar, Kin Kee Chow, Peter Han Joo Chong . Optical environmental sensing in wireless smart meter network. AIMS Electronics and Electrical Engineering, 2018, 2(3): 103-116. doi: 10.3934/ElectrEng.2018.3.103 |
[10] | Simon Bateson . A USB high resolution lock-in photometer. AIMS Electronics and Electrical Engineering, 2019, 3(1): 1-15. doi: 10.3934/ElectrEng.2019.1.1 |
The Internet of Things (IoT) has an ever more significant function in many areas of our modern world. It improves the overall quality of life, where almost everything, including objects and devices, can be linked to the cloud and thus be hyper-connected. Within this changing IoT system, this paper focuses on data transfer, emphasizing the critical role of light fidelity (LiFi) technology. LiFi technology is an up-and-coming technology that makes use of light-emitting diodes (LEDs) and photodetectors (PDs) as transmitters and receivers (RX), respectively, for concurrent data communications and lighting. This paper proposes a single-input multiple-output IoT-based LiFi system using an LED array, phototransistor, and ambient light sensor-based RXS for simultaneous data transmission. DHT11 sensor is used to measure the environmental conditions and store collected data via Wi-Fi in the cloud server using HTTP protocol. Through indoor LiFi communication, the cloud streaming data is transferred using pulse position modulation (PPM) at a frequency of 2 KHz. The experimental results illustrate transferring and receiving data successfully at a communication distance of 1 meter. MATLAB software is used to evaluate the performance of the spatial radiance pattern and illuminance intensity of an LED Array used and received power depending on a line-of-sight (LOS) channel.
The Internet of Things (IoT) is a platform that facilitates the connection of numerous devices, including sensors, wearables, and equipment, to a network connection, enabling communication with data centers, and devices. IoT represents a state of hyper-connectivity wherein virtually all objects and devices can extend the reach of Internet access beyond traditional computing devices, such as computers, tablets, and smartphones, allowing for communication and interaction with the external environment around the advanced wireless communication network while supporting the existence of smart things around us. The information and advanced technology open up many ways to access this information [1]. IoT frameworks are widely used in many applications, including smart transportation systems, intelligent environments, intelligent medical care, intelligent homes, smart agriculture, smart offices, and supply chain logistics IoT [2]. Every IoT device is cemented with a Wi-Fi module to transmit data through the internet. Due to the explosive growth in the amount of wireless data to be transmitted, the radio wave spectrum is quickly reaching its limits and causing problems, increasingly causing high-frequency electromagnetic interference and not having enough spectrum capacity to send huge amounts of data types and difficulty of data security. Because of these limitations of wireless technologies that use radio waves to transmit signals, many researchers have turned their attention to other parts of the electromagnetic spectrum to find new ways to solve this problem [3]. Therefore, light fidelity (LiFi), proposed by Professor Harald Haas in 2011, is considered one of the most robust and trustworthy technologies and is widely known as a complementary solution to wireless technologies, especially Wi-Fi. Specifically, visible light possesses a spectrum range that surpasses radio frequency by roughly 10000 times, and it operates without the need for regulation, meaning that LiFi does not require a license to function [4,5]. Additionally, the LiFi network system provides the advantage of dual functionality, serving both as a source of illumination and a means of communication [6,7]. Figure 1 summarizes the operation of this technology. Li-Fi has the potential to leverage multiple regions of the optical spectrum, including visible light, infrared (IR), and ultraviolet (UV), for information transmission [8,9]. In addition to sending information, Li-Fi also uses light as a source of illumination. It uses light-emitting diode (LED) lights to exchange information and photodetectors such as photodiodes, phototransistors, and ambient light sensors (ALS) to receive light signals. LiFi uses signal processing technology to feed information into LED light bulbs. A photodetector is then used on the receiver side to acquire the light signal. Signal processing components then convert the optical signal into streaming data [10,11].
Moreover, LiFi holds the potential for compatibility with IoT cloud networks using physical layer protocols, including modulation, coding, and framing techniques, thereby opening up vast opportunities for its future development and widespread adoption [12,13]. A cloud platform refers to a virtual environment where users can store, manage, and access their data remotely via the internet. It provides access when needed to different computing capabilities like storage space, processing power, and applications [14]. It is important to explore the feasibility of integrating the advantages of both LEDs and IoT technology to establish an indoor wireless network. As IoT adoption keeps growing, sensors are appended to more and more things, necessitating the need for a quicker and more reliable data transmission source. This can only be accomplished with a widespread and reasonably priced communication infrastructure that strives to regulate the behavior of the devices and offer communication reliability.
The continuous pursuit of improved LiFi performance has driven significant technological advancements in recent years. To address exploring the potential of LiFi for IoT networks, several works have been suggested in the literature. The paper [15] discusses the use of Li-Fi technology, which employs visible light for data transmission instead of conventional radio frequency (RF) methods. This involves the use of light-emitting diodes (LEDs) that flicker at high speeds to encode data in binary form. The implementation of Li-Fi systems includes the use of photodetector-equipped receivers that can decipher the encoded data transmitted through the rapid on-and-off flashing of LEDs, allowing for high-speed data transfer that can reach up to 100 Gbps in laboratory settings.
The paper [16] highlights the potential of Li-Fi technology, utilizing LEDs for high-speed, short-range wireless data transmission, particularly beneficial for the IoT. By leveraging visible light communication (VLC), Li-Fi can facilitate real-time data transfer in various environments, enhancing connectivity in homes and offices. The rapid frequency modulation of LEDs allows for greater data capacity compared to traditional Wi-Fi, making it an innovative solution for IoT applications where reliable and efficient communication is essential.
The study in [17] presents Li-Fi-IoT, a Li-Fi-based system specifically designed for efficient and secure management of IoT devices within smart home automation systems (SHAs). This system utilizes visible light for wireless data transmission to control various home devices remotely. A series of experiments were conducted to demonstrate the potential of the Li-Fi technology in IoT device control, highlighting improvements in data transfer speed, energy efficiency, and data security within the context of smart home automation. The authors in [18] propose LiRF, a light-based wireless communication system that enables smooth cooperation between VLC and RF networks, achieving near-Gbps data rates for IoT devices and paving the way for seamless integration of VLC in IoT networks.
The paper [19] highlights the potential of VLC technology, specifically LiFi, for high-speed wireless data transmission using LEDs. By leveraging the visible light spectrum, LiFi can facilitate efficient communication between IoT devices. The methodology outlined ensures optimized performance through careful selection of components and modulation techniques, making it suitable for various applications, including indoor wireless communication. This integration of LiFi with IoT can enhance data transfer rates and reliability in smart environments.
The integration of LiFi with IoT and Wi-Fi confronts several critical challenges, including the energy inefficiency of maintaining separate infrastructures for illumination and data transmission, and the need for secure, high-speed data transfer in resource-constrained environments. Traditional systems often rely on dedicated networking hardware, which escalates energy consumption and deployment costs while limiting scalability. Further, the presence of additional light sources in a LiFi system, particularly from ambient light sources, raises significant challenges that can impact the system's performance [20]. This paper addresses these challenges by leveraging a dual-function LED lighting system that reduces hardware redundancy and energy consumption. It uses pulse position modulation (PPM) for efficient communication in variable lighting, incorporates smartphone-based light sensors to streamline receiver design, and facilitates cloud compatibility. The approach enhances security via encrypted LiFi streaming, bridges interoperability with Wi-Fi/IoT networks, and promotes sustainable, energy-efficient indoor wireless solutions. The proposed system mitigates ambient noise from dedicated ambient LEDs via low-pass filtering. The key contributions of the study are as follows:
● Integration of LiFi, IoT: A novel hybrid system architecture is proposed that combines LiFi, IoT, and Wi-Fi technologies to enable energy-efficient indoor wireless communication. This approach leverages existing lighting infrastructure for simultaneous data transmission and illumination, reducing reliance on standalone networking devices.
● IoT-cloud integration for real-time monitoring: The proposed system as shown in Figure 2 incorporates an IoT cloud platform alongside ALS and photodetector (PD) in smartphones, enabling real-time environmental monitoring and adaptive data streaming based on observed conditions.
● Practical implementation: A prototype demonstrates the feasibility of merging IoT analytics with LiFi-enabled data transmission using PPM, offering a scalable solution for smart indoor environments that prioritizes energy efficiency and interoperability.
The paper is organized as follows. Section 2 discusses the Li-Fi in IoT applications. Section 3 discusses the modulation techniques for LiFi. In Section 4, an IoT-based LiFi system architecture is presented. In Section 5, an experimental setup is performed. Section 6 presents the analysis of experimental and simulation results. Finally, in Section 7 we conclude the paper.
This section reviews literature focusing on specific IoT applications that can effectively leverage LiFi technology. The selected studies primarily address indoor scenarios where LiFi can be applied within IoT frameworks. Various application scenarios are discussed concisely, to highlight the potential of LiFi technology for real-world implementation. Furthermore, numerous LiFi applications are identified as being particularly compatible with IoT networks.
The study presented in [21] explores the integration of LiFi use cases within IoT environments. It operates on the premise that LiFi solutions are typically customized to their respective ecosystems. The primary objective of this research is to introduce and discuss a LiFi system concept tailored to the requirements of IoT applications. The study delineates the most significant LiFi use cases and their corresponding requirements for IoT, highlighting the flexibility and versatility afforded by various hardware and software components. In [22], a proposal is introduced for a system utilizing LiFi to support IoT nodes, with a focus on indoor application scenarios. This research provides a comprehensive overview of the LiFi access point and associated IoT devices. The proposed system is specifically designed for LiFi in IoT contexts, featuring an in-depth analysis of system components and structure, particularly regarding positioning and power delivery. The research offers practical solutions for IoT nodes that necessitate only intermittent data delivery through modulation techniques, leveraging available LEDs to facilitate the IoT environment. Additionally, the study explores critical research directions in the domain of LiFi for IoT.
The integration of LiFi within the e-health scenario is analyzed in [23]. This study leverages both LiFi and IoT technologies to enhance health monitoring systems, allowing physicians to promptly update patients' health statuses in the cloud. In this context, the cloud functions as the IoT system, while the LiFi network ensures rapid and interference-free connectivity. This enables physicians to assess patient data and deliver real-time feedback. The study accurately identifies the essential components of the health monitoring system and elucidates their interactions, supplemented by flow diagrams illustrating the system. LiFi technology is also explored in mining environments, as detailed in [24]. This study addresses critical situations where the dissemination of emergency information is hampered by inadequate WiFi coverage. LiFi is employed to monitor hazardous conditions detected by IoT sensors, and a decision-making system is developed to identify abnormal circumstances related to dangerous gases. The performance of the proposed system is evaluated in an authentic testbed, showcasing the utilized hardware components.
In [25], a prototype of a LiFi system designed to transmit analog audio signals is examined for its applicability in IoT. The audio signal is sourced from a mobile phone, which is modulated and converted into light using blinking LEDs. A solar cell then captures the light signal and transmits it to a speaker. The system utilizes genuine hardware components, validating its effectiveness and underscoring the primary advantages of incorporating LiFi into IoT applications. A more extensive application scenario for indoor use is proposed in [22], wherein LiFi serves IoT nodes. This scenario is suitable for delivering various services, and the scheme is thoroughly described, including its components and performance characteristics, such as power consumption and data rate. A comparative analysis between LiFi and RF transmission is also presented, emphasizing the limitations and challenges associated with LiFi. Finally, the study discusses key research directions to promote the integration of LiFi and IoT. In [26], LiFi technology is utilized for local advertising within the context of public transportation. This research primarily focuses on outdoor scenarios, where LiFi transmits local advertisements to devices in proximity to LED sources. Nonetheless, the proposed model is deemed appropriate for indoor IoT communications, enhancing data reliability and addressing bandwidth limitations. The paper provides a comprehensive examination of both the transmitter and receiver sections of LiFi communication, presenting a hardware-based implementation model and evaluating its performance regarding signal attenuation and bit error rate.
The analyzed paper indicates that LiFi technology can be effectively integrated into a diverse array of IoT application scenarios. In these contexts, LiFi offers notable advantages, including high energy efficiency, enhanced security measures, substantial bandwidth availability, the use of low-cost components, and strong resistance to electromagnetic interference. However, the studies also suggest that LiFi technology has not yet matured sufficiently to fully replace existing WiFi connections in IoT applications. Instead, a hybrid approach that combines WiFi and LiFi is recommended for optimal performance. LiFi is particularly valuable for IoT applications that prioritize cost-effective optimization while ensuring high throughput, reliability, and low latency, especially in indoor environments. Furthermore, the analysis presented in these articles suggests that LiFi is poised to become a leading technology for the IoT in the future.
Modulation techniques are essential components of LiFi systems as they allow for the encoding and decoding of information into light signals. These techniques enable efficient utilization of the available spectrum and enhance the overall performance of LiFi systems [27]. Modulation techniques are integral to visible light communication systems, offering immense potential for high-speed data transfer in various applications ranging from indoor wireless networks to vehicular communication, monitoring, and beyond. Figure 3 shows the overall modulation schemes utilized in LiFi systems.
This paper utilized PPM, a widely used digital modulation technique in communication systems, to efficiently transmit information through noisy channels. Unlike amplitude or frequency modulations, PPM encodes data in the timing of pulses within a signal. In PPM, each pulse denotes discrete symbols representing bits of data, where the position of the pulse within a fixed timeframe determines the symbol being transmitted [28]. By altering the pulse locations based on binary patterns that represent the data bits, multiple symbols can be sent successively. PPM provides benefits in bandwidth efficiency, enabling higher data rates within a given bandwidth compared to other modulation techniques. It is also more robust to noise and interference because of the discrete nature of the symbols [29,30]. As shown in Figure 4, PPM signal formats are created by altering the location of a pulse.
Where x(t) represents the intensity of the optical PPM signal, and the transmission pulse form is presented by P(t). Further, x(t) may be expressed [31]:
x(t)=LP∑KK=0Plk(t−kT) | (1) |
Where LP is an operator at x(t), T is a symbol period, and Plk(t) expresses the set of pulse waveforms.
plk(t)={1fort∈[l−1t,lTL],forl∈1,2,…,L0elsewhere | (2) |
Where T is partitioned into L separate intervals and the symbol l denotes the location within a particular interval during a k′th symbol period.
The performance of the PPM technique in the LiFi system can be set by calculating the bit error rate (BER) for PPM as expressed in Eq (3):
PPPM=12erfc(12√2√SNRL2log2L) | (3) |
Where, SNR and L indicate the signal-to-noise ratio and order of modulation, respectively.
IoT devices are made up of energy-efficient intelligent sensors that need smaller amounts of data compared to their traditional counterparts [32]. However, With the increasing interconnectivity of devices through the Internet, both Li-Fi and Wi-Fi can supply the needed frequency range for transferring large amounts of data. In this paper, we propose to use the Li-Fi communication medium to transfer IoT cloud data from a sensor for receiving with photodetectors.
The proposed IoT-based LiFi System architecture diagram is shown in Figure 5, on the transmitter side the IoT has been strengthened through the integration of Li-Fi and Wi-Fi. Including various sensors can form a matrix that facilitates data interaction and communication in actual time by using Li-Fi connections. These things also connect to Wi-Fi networks create a connection and facilitate the exchange of information between cloud organisms and services. The sensory device in the proposed system discovers and supervises environmental factors such as temperature, and humidity, and provides status updates. The main function of the IoT gate is to convert field protocols into cloud protocols, such as message queuing telemetry transport (MQTT), and hypertext transfer protocol (HTTP) protocols to enable communication between a sensor and the cloud [33].
The NodeMCU used is connected to the Wi-Fi network to upload and download processes with the cloud. Sensor data monitored and stored in the cloud can be requested at any time using one of the protocols for transfer through an indoor Li-Fi system. The content data is modulated and converted into binary data using the PPM technique for transfer via an LED array. From the receiver side, there are two types of detection used, a phototransistor in the first receiver (RX1) and an ALS in a smartphone in the second receiver (RX2) to capture the incoming light with sensor data included and process data to display.
In the transmitter circuit schematically illustrated in Figure 6, we used a DHT11 sensor to monitor changes in the environment, which is a digital sensor that measures the humidity and temperature levels as in Eq (4):
RH=PWPS∗100% | (4) |
Where PW is the actual water steam intensity and PS is the water steam intensity at saturation.
These values are also continuously monitored and stored on the ThinkSpeak cloud through NodeMCU ESP32. In this prototype, the Arduino UNO MCU is fed with the cloud data by sensor data stored in the cloud using ESP32. The LED driver circuit uses N-channel MOSFET IRF520. To sense the gate input of the MOSFET, the output of the microcontroller is programmed using C language to produce a modulated PPM level signal. An additional series diode is connected to the circuit to protect the output pin of the microcontroller from the bias voltage. When the gate voltage level fluctuates in response to the modulation signal, the MOSFET draws current corresponding to the gate voltage fluctuation.
In the system, there are two LiFi receivers for the detection process. The first receiver (RX1) uses a phototransistor to convert incoming light into electrical energy. For digital signals, the circuit between the phototransistor and the Arduino must be processed to correctly interpret the electrical signal. Therefore, the current is converted into voltage to be amplified and compared. To amplify the signal, we used a variable resistor to maintain a constant, stable voltage scale for the voltage signal. The output signal is routed to the microcontroller unit's (MCU) analog-to-digital converter (ADC) for subsequent sampling and demodulation processes. The received data will be exhibited on an LCD module. The second receiver (RX2), encompasses a light sensor that enables the identification of light emanating from the LED array by utilizing one of the pre-installed sensors present in smartphones, commonly referred to as the ALS. We have developed an Android application through the utilization of Android Studio Software, which utilizes the ALS to receive sensor data transferred via a modulated LED array light. The receiver's circuit schematic is indicated in Figure 7.
The propagation model in a proposed IoT-based LiFi system is the line-of-sight channel whereby the system based on optical technology uses LED arrays and PDs with large surface areas. On the transmitter side, the radiation intensity pattern for the LED's angular distribution [34], is shown in Figure. 8, is based on a Lambertian pattern and is defined by Eq (5).
Ro(φ)={m1+12πcosm1(φ),Φϵ[−π/2,π/2]0,φ≥π/2 | (5) |
Where φ=0 is the angle at which the maximum power is radiated, Փ is the half-angle of the LED's beam, and m1 can be obvious of the LED's half-power semiangle using Eq (6) [35].
m1=ln(2)ln(cos(Φ1/2)) | (6) |
The radiant intensity of an LED, which is described in terms of the angle of irradiance and the total power output of the LED, can be calculated using Eq (7).
Pi=(m1+1)Ptcosm1(∅)2π | (7) |
where Pt represents the total power that is transmitted by an LED.
The LOS channel DC gain can be determined by:
HLOS(0)={Ar(m1+1)2πd2∗Cosm1(∅)Ts(ѱ)g(ѱ)Cos(ѱ),0≤ѱ≤ѱc0,ѱ≥ѱc | (8) |
where Ar represents the photodetector surface area, ѱ is the angle of incidence. Ts(ѱ), g(ѱ) represents the optical filter's and an optical concentrator's gain, correspondingly. ѱc is a FOV at the receiver, and d is the space between the receiver and sender.
In the receiver, the optical power Pr received at the PD is derived from the transferred Pt, the photodetector responsivity R and may be expressed:
Pr−LOS=HLOS(0)∗R∗Pt | (9) |
The system includes the DHT11 sensor for computing the environmental conditions. The sensor is linked to the NodeMCU ESP32 that is used to uplink and downlink processes with a cloud. The data is accumulated in the microcontroller after being picked up by the sensor and then sent to the IoT cloud through Wi-Fi. The IoT cloud platform functions as a centralized hub for storing and processing this sensor data. We used the HTTP protocol to connect sensor data with the cloud server which facilitates data transmissions between the server and web platforms and considerably reduced the cross-platform problem. The ThingSpeak cloud server was used to store the data in private or public channels and perform analysis on the data. To activate the connection between the ThingSpeak cloud and the LiFi system, we used the API keys of a ThingSpeak channel to establish a secure connection and request specific data from the cloud to the indoor LiFi system.
The LiFi transmitter consists of an LED driver connected to the ATmega 328p microcontroller to drive the white LED Array that provides the benefits of low energy exhaustion and high intensity to achieve a lengthy distance between the transmitter and receiver up to 1 meter using PPM. MCU can produce the 2 kHz carrier wave with precision in the transmission. On the receiver side, we have used two LiFi receivers and a phototransistor 3DU33 in the RX1 that can receive light signals in the shape of an electric flow that holds various values. After converting it to digital, the signal is passed to the Arduino UNO for demodulation processes and the outcome received is exhibited on LCD. The other receiver RX2 includes an ALS in the Alcatel smartphone to detect incoming light. When the level of ambient light fluctuates, our developed Android application can preserve lighting intensity and receive the data. Table 1 shows the ALS specifications in an Android app used. Figure 9 and Figure 10 illustrate the transmitter and receiver hardware implementation and the experimental setup of the proposed system, respectively.
Sensibility | Delay time [µS] |
Sensor delay speedy | 0 |
Sensor UI | 50.000 |
Sensor delays Normal | 100.000 |
As a part of the work, we built a prototype based on IoT for an indoor Li-Fi system to send data to PDs. An experiment was performed to sensor data transmission and reception using a photodetector and mobile-developed application. The DHT11 sensor is employed to detect humidity levels and obtain temperature information. The sensor is linked with Wi-Fi to transfer the collected data to the ThinkSpeak cloud, the sensing values graphs are shown in Figure 11.
Additionally, Li-Fi technology is incorporated to transmit the humidity conditions and temperature data from cloud storage. To facilitate the data transmission, PPM is utilized. The utilization of PPM in VLC systems effectively minimizes flickering effects since it employs a high level to represent both bit 0 and bit 1. The PPM signal is generated following the sensor data bit, whereby the filled slot is positioned to the left slot if a bit is logical 0. Conversely, if the bit is logical 1, the filled slot is positioned to the right slot. The entire system functions efficiently with the LED array of the transmitter, and the receivers are capable of receiving the data transmitted. The space between the sender and receivers, as measured for the prototype, is approximately 1 meter. Even when the angle between the LED array and phototransistor exceeds 60 degrees, the data can still be received relatively quickly in the phototransistor of the first receiver. The difference in received voltage between logic 1 and logic 0 was minimal, so the signal was amplified using a 741 op-amp to the Arduino UNO and subsequently demodulated to display the original data. The output waveforms using a DSO for the transmitted and received data are indicated in Figure 12.
The monitoring sensor data changes over time. Figure 13 shows the output of RX1 using a phototransistor on the LCD. The information regarding the sensor used is sent to the end user Li-Fi data monitoring display.
For the RX2 result based on the Android app using an ALS, we chose the fastest level and experimented. From the results, the least time interval is around 7 ms, indicating that the light sensor can expose the signal with a high sensitivity. The result shown in Figure 14 indicates the illumination level value as a lux meter and the received data.
The receiving process was done successfully with the phototransistor and ALS used in the LiFi receivers. Depending on the amount of light that the photodetectors receive on the reception side and their specifications, Figure 15 compares two types of photodetectors a phototransistor and an ALS in which there is a descending trend in both sensors' response to the light. The phototransistor response is more sensitive than the ALS response which means the phototransistor can detect faster changes in light intensity. The phototransistor is suitable for applications that require high data rates.
The probability of errors for PPM with a given BER can be calculated using the relationship shown in Eq (3). Figure 16(a) displays the theoretical and simulated error probability curve for PPM across different BER values.
Figure 16(b) presents a logarithmic plot showcasing the relationship between the BER and communication distance in an experimental setup utilizing an LED array, phototransistor, and ALS-based RXS for simultaneous data transmission. The plot reveals a single data point at a communication distance of 20 cm, where the BER is measured at 10-3. As the communication distance extends towards 1 meter, there is a notable increase in the BER.
In the LiFi system, the radiation paradigm of the LED is comparable to the Lambertian model. The optical medium is used to spread the light that the LEDs emit. The simulation parameters used in MATLAB software are illustrated in Table 2.
Parameters | Values |
LED arrays | 1 |
LEDs per array | 25 |
All LEDs power | 2W |
LED Type | 3528 SMD |
Luminous Flux | 400 lm (at 100 mA, 25℃) |
Center Luminous Intensity | 0.73 [cd] |
Receiver's Field of View 𝜃𝑐 | 60 [deg] |
Photodetector Area APD | 1 [Cm2] |
Photodetector responsivity R | 0.4 [A/W] |
The results focus on the LOS channel used in the LiFi system. The results in Figure 17(a) illustrate the spatial radiance pattern of an LED as a light source in polar coordinates. It visualizes how the intensity of light disperses in different directions. The pattern indicates varying intensities of light emission-specific angles at which the intensity is measured. Figure 17(b) illustrates the illumination distribution with LED array lights where the coverage area had enough light, and the average illuminance level was 350 lux, which was considered adequate.
The considerable contribution to the used lighting LED array structure is the reality that it expends low energy in downlink connection. Figure 18 illustrates the LiFi receivers' received optical power distribution values. It is observed that light and optical power are concentrated in the middle.
Where the square of the distance between the LED array and photodetector is inversely proportionate to received power as expressed in Eq (9). Therefore, as the detector moves gradually from the center position toward the corners, the received power value decreases.
The rapid proliferation of the IoT necessitates innovative solutions for reliable, high-speed data transmission in environments saturated with connected devices. LiFi technology has emerged as a transformative approach, leveraging visible light for simultaneous illumination and communication. This analysis evaluates recent advancements in LiFi systems for IoT applications, comparing the experimental framework proposed in our work with existing works in the field.
Our system employs a single-input multiple-output (SIMO) architecture featuring an LED array transmitter and phototransistor-based receivers augmented with an ALS. This design contrasts with Ref [17] smart home implementation, which uses point-to-point LiFi links without spatial diversity. The proposed approach enhances signal reliability through spatial redundancy, addressing occlusion challenges common in IoT environments where devices may operate in shadowed areas.
In Ref [19] a visible light communication model using discrete LEDs at a 0.8 m communication distance. While their system prioritizes bandwidth, our LED array configuration optimizes coverage uniformity, achieving high illuminance consistency across 1 m according to MATLAB radiation pattern simulations. This spatial optimization proves critical for IoT deployments requiring stable connectivity for distributed sensors.
Unlike in Ref [18] framework that integrates RF signals directly into LiFi modulation, our system adopts a dual-layer architecture: LiFi handles device-to-device communication while Wi-Fi manages cloud uplink via HTTP. This separation leverages LiFi's interference-free operation for local data aggregation while utilizing existing Wi-Fi infrastructure for wide-area connectivity, reducing deployment costs compared to fully hybrid systems. In Ref [21] flexible LiFi concept shares this pragmatic approach, advocating for the complementary use of optical and RF technologies.
This comparative analysis positions our proposed system LiFi system as a pragmatic advancement in IoT connectivity, balancing performance with deployment feasibility. Our architecture demonstrates unique strengths in adaptive reception and hybrid network integration. The results validate LiFi's potential as a complementary technology in the IoT communication ecosystem, particularly for applications requiring electromagnetic interference immunity and spatial-diverse connectivity.
This paper introduced the experimental implementation of an IoT-based LiFi system. The LiFi scheme in an indoor static environment that a white LED Array-based Tx was used for the simultaneous transmission of signals. The DHT11 sensor was used to measure the humidity and temperature conditions where the data was stored in the ThinkSpeak cloud channel using HTTP protocol. We can request the cloud data using API keys for transfer via a secure LiFi system using a PPM technique and receive it through two LiFi receivers. A phototransistor was employed as the primary receiver, while a smartphone-based Android application leveraging the integrated ALS was implemented as a secondary receiver for data receiving and illuminance measurement. The data is received successfully at the communication distance of 1 meter between the transmitter and receiver. The integration of Wi-Fi-based IoT and LiFi technology holds significant implications for future communication systems. As demonstrated in recent studies, hybrid LiFi-WiFi architectures, underscore the potential of these systems for scalable, high-speed data transmission, such as in [36]. Recent advancements in mobility-aware blockage management, as demonstrated by [37] in multi-user hybrid architectures, further validate the robustness of such integrated frameworks. By leveraging the strengths of both systems, faster wireless data transfer can be achieved, enabling applications across diverse sectors, including smart environments and industrial IoT.
All authors contributed equally to this work.
The authors declare they have not used Artificial Intelligence (AI) tools in the creation of this article.
The authors declare no conflicts of interest in this paper.
[1] | Priyadarsini K, Karthik S, Sonia JJ, Anbazhagu UV, Saranya VG (2025) An IoT-based structure for development management using radio frequency identification system. In Responsible AI for Digital Health and Medical Analytics, 327‒350. IGI Global Scientific Publishing. https://doi.org/10.4018/979-8-3693-6294-5.ch013 |
[2] |
Nawaz U, Rehman F, Ullah KA, Raza UA, Sharif H, Junaid M (2025) Analysis of Device-to-Device Communication in IoT System. Securing the Digital Realm: Advances in Hardware and Software Security, Communication, and Forensics, 48. https://doi.org/10.1201/9781003497851-5 doi: 10.1201/9781003497851-5
![]() |
[3] | Ankitha HM, Paul IS, Rao SS (2025) Simulation and Implementation of Li-Fi Communication System. International Journal of Artificial Intelligence of Things (AIoT) in Communication Industry 1: 13‒21. |
[4] |
Morales-Céspedes M, Pérez-Aracil J, García-Armada A, Salcedo-Sanz S (2025) Learning for Visible Light Communications: Potential Scenarios and Applications. IEEE Consum Electr Mag. https://doi.org/10.1109/MCE.2025.3525584 doi: 10.1109/MCE.2025.3525584
![]() |
[5] |
Eltokhy MAR, Abdel-Hady M, Haggag A, El-Bendary MAM, Ali H, Hosny T (2023) Audio SIMO system based on visible light communication using cavity LEDs. Multimed Tools Appl 82: 46371–46385. https://doi.org/10.1007/s11042-023-15680-8 doi: 10.1007/s11042-023-15680-8
![]() |
[6] | Kaur T, Kaur A, Sharma Y (2024) Li-Fi: An Approach to the New Era of Optical Wireless Technology. In Latest Trends in Engineering and Technology, 244‒249. CRC Press. https://doi.org/10.1201/9781032665443-35 |
[7] |
Singh A, Pandey R (2025) Exploring visible light communication for real-time indoor metaverse connectivity. Journal of Optical Communications. https://doi.org/10.1515/joc-2024-0261 doi: 10.1515/joc-2024-0261
![]() |
[8] |
Chen C, Basnayaka DA, Purwita AA, Wu X, Haas H (2020) Wireless infrared-based LiFi uplink transmission with link blockage and random device orientation. IEEE T Commun 69: 1175‒1188. https://doi.org/10.1109/TCOMM.2020.3035405 doi: 10.1109/TCOMM.2020.3035405
![]() |
[9] |
Yilmaz AF, Myderrizi I (2024) Increasing indoor Li-Fi system efficiency using light reflective materials and lenses. Journal of Engineering Research. https://doi.org/10.1016/j.jer.2024.10.014 doi: 10.1016/j.jer.2024.10.014
![]() |
[10] |
Alfattani S (2021) Review of LiFi Technology and Its Future Applications. Journal of Optical Communications 42: 121–132. https://doi.org/10.1515/joc-2018-0025 doi: 10.1515/joc-2018-0025
![]() |
[11] |
Yarbrough P, Welker T, Silva D, Islam R, Patamsetti VV, Abiona O (2022) Analyzing Li-Fi Communication Benefits Compared to Wi-Fi. International Journal of Communications, Network and System Sciences 15: 67–77. https://doi.org/10.4236/ijcns.2022.156006 doi: 10.4236/ijcns.2022.156006
![]() |
[12] |
Petrosino A, Striccoli D, Romanov O, Boggia G, Grieco LA (2023) Light Fidelity for Internet of Things: A survey. Opt Switch Netw 48: 100732. https://doi.org/10.1016/j.osn.2023.100732 doi: 10.1016/j.osn.2023.100732
![]() |
[13] |
Al Hwaitat AK (2020) A Survey on Li-Fi Technology and Internet of Things (IOT). International Journal of Advanced Trends in Computer Science and Engineering 9: 225–232. https://doi.org/10.30534/ijatcse/2020/34912020 doi: 10.30534/ijatcse/2020/34912020
![]() |
[14] |
Qaisar F, Shahab H, Iqbal M, Sargana HM, Aqeel M, Qayyum MA (2023) Recent Trends in Cloud Computing and IoT Platforms for IT Management and Development: A Review. Pakistan Journal of Engineering and Technology 6: 98–105. https://doi.org/10.51846/vol6iss1pp98-105 doi: 10.51846/vol6iss1pp98-105
![]() |
[15] |
Khan KA, Dahiya A, Sharma G (2024) LiFi Luminescence: Illuminating the Future of Wireless. Available at SSRN 5013214. https://doi.org/10.2139/ssrn.5013214 doi: 10.2139/ssrn.5013214
![]() |
[16] |
Lokumarambage MU, Gowrisetty VSS, Rezaei H, Sivalingam T, Rajatheva N, Fernando A (2023) Wireless end-to-end image transmission system using semantic communications. IEEE Access 11: 37149‒37163. https://doi.org/10.1109/ACCESS.2023.3266656 doi: 10.1109/ACCESS.2023.3266656
![]() |
[17] |
Aydin H, Aydın GZG, Aydın MA (2024) The potential of light fidelity in smart home automation. Bulletin of Electrical Engineering and Informatics 13: 3155‒3166. https://doi.org/10.11591/eei.v13i5.7199 doi: 10.11591/eei.v13i5.7199
![]() |
[18] |
Zhang R, Li M, Zhang Y, Xiong J, Lu L (2024) LiRF: Light-based Wireless Communications Supporting Ubiquitous Radio Frequency Signals. IEEE Photonics Journal. https://doi.org/10.1109/JPHOT.2024.3449326 doi: 10.1109/JPHOT.2024.3449326
![]() |
[19] | Balaram A, Jadhav RD, Kumar N, Muthukumaran D, Nalini C, Kumar YD (2024) Empowering LiFi Technology: Experimental Design of Light Assisted Data Communication using Visible Light Communication Model. In 2024 5th International Conference on Image Processing and Capsule Networks (ICIPCN), 941‒947. https://doi.org/10.1109/ICIPCN63822.2024.00161 |
[20] |
Chatterjee S, Roy B (2024) An approach to ensure joint illumination & communication performance of a forward error corrected indoor visible light communication (VLC) system in presence of ambient light interference. Journal of Optical Communications 44: s1767‒s1776. https://doi.org/10.1515/joc-2019-0212 doi: 10.1515/joc-2019-0212
![]() |
[21] | Bober KL, Jungnickel V, Emmelmann M, Riegel M, Tangdiongga E, Koonen AMJ, et al.(2020) A Flexible System Concept for LiFi in the Internet of Things. In Proceedings of the 2020 22nd International Conference on Transparent Optical Networks (ICTON), 1–4. https://doi.org/10.1109/ICTON51198.2020.9203185 |
[22] |
Demirkol I, Camps-Mur D, Paradells J, Combalia M, Popoola W, Haas H (2019) Powering the Internet of Things through Light Communication. IEEE Commun Mag 57: 107–113. https://doi.org/10.1109/MCOM.2019.1800429 doi: 10.1109/MCOM.2019.1800429
![]() |
[23] | Mohammed J, Lung CH, Ocneanu A, Thakral A, Jones C, Adler A (2014) Internet of Things: Remote Patient Monitoring Using Web Services and Cloud Computing. In Proceedings of the 2014 IEEE International Conference on Internet of Things(iThings), and IEEE Green Computing and Communications (GreenCom) and IEEE Cyber, Physical and Social Computing (CPSCom), 256–263. https://doi.org/10.1109/iThings.2014.45 |
[24] |
Hu Y, Leus G (2015) Selp-Estimation of Path-Loss Exponent in Wireless Networks and Applications. IEEE Trans Veh Technol 64: 5091–5102. https://doi.org/10.1109/TVT.2014.2380823 doi: 10.1109/TVT.2014.2380823
![]() |
[25] | Othman RAA, Sagaran DA, Mokayef M, Nasir WIIRBWM (2018) Effective LiFi Communication for IoT Applications. In Proceedings of the 2018 IEEE 4th International Symposium in Robotics and Manufacturing Automation (ROMA), 1–4. https://doi.org/10.1109/ROMA46407.2018.8986698 |
[26] | Dinesh Kumar JR, Priyadharsini K, Srinithi K, Samprtiha RV, Ganesh Babu C (2021) An Experimental Analysis of Lifi and Deployment on Localization Based Services & Smart Building. In Proceedings of the 2021 International Conference on Emerging Smart Computing and Informatics (ESCI), 92–97. https://doi.org/10.1109/ESCI50559.2021.9396889 |
[27] |
Geng Z, Khan FN, Guan X, Dong Y (2022) Advances in Visible Light Communication Technologies and Applications. Photonics 9: 893. https://doi.org/10.3390/photonics9120893 doi: 10.3390/photonics9120893
![]() |
[28] | Farhat ZA, Ahfayd MH, Mather PJ, Sibley MJN (2019) Improved BER for Offset Pulse Position Modulation Using Priority Decoding over VLC System. In Proceedings of the 2019 Wireless Days (WD), 1–4. https://doi.org/10.1109/WD.2019.8734159 |
[29] |
Loureiro PA, Guiomar FP, Monteiro PP (2023) Visible Light Communications: A Survey on Recent High-Capacity Demonstrations and Digital Modulation Techniques. Photonics 10: 993. https://doi.org/10.3390/photonics10090993 doi: 10.3390/photonics10090993
![]() |
[30] |
Afroj M (2023) Li-Fi Concept in Terms of Modulation Techniques. International Journal of Research and Innovation in Applied Science 8: 01–05. https://doi.org/10.51584/IJRIAS.2023.8601 doi: 10.51584/IJRIAS.2023.8601
![]() |
[31] |
Sönmez M (2022) Performance Analysis of FSK-PPM Technique in Visible-Light Communication Systems. Journal of Optical Communications 43: 447–455. https://doi.org/10.1515/joc-2019-0009 doi: 10.1515/joc-2019-0009
![]() |
[32] |
Khanna A, Kaur S (2020) Internet of Things (IoT), Applications and Challenges: A Comprehensive Review. Wireless Pers Commun 114: 1687–1762. https://doi.org/10.1007/s11277-020-07446-4 doi: 10.1007/s11277-020-07446-4
![]() |
[33] |
Gupta BB, Quamara M (2020) An Overview of Internet of Things (IoT): Architectural Aspects, Challenges, and Protocols. Concurrency and Computation 32: e4946. https://doi.org/10.1002/cpe.4946 doi: 10.1002/cpe.4946
![]() |
[34] |
Yahia S, Meraihi Y, Ramdane-Cherif A, Gabis AB, Acheli D, Guan H (2021) A Survey of Channel Modeling Techniques for Visible Light Communications. J Netw Computer Appl 194: 103206. https://doi.org/10.1016/j.jnca.2021.103206 doi: 10.1016/j.jnca.2021.103206
![]() |
[35] | Gismalla MSM, Abdullah MFL, Mabrouk WA, Das B, Niass MI (2019) Data Rate and BER Analysis for Optical Attocells Configuration Model in Visible Light Communication. In Proceedings of the 2019 International Conference on Information Science and Communication Technology (ICISCT), 1–6. https://doi.org/10.1109/CISCT.2019.8777433 |
[36] |
Wu X, Soltani MD, Zhou L, Safari M, Haas H (2021) Hybrid LiFi and WiFi networks: A survey. IEEE Commun Surv Tut 23: 1398‒1420. https://doi.org/10.1109/COMST.2021.3058296 doi: 10.1109/COMST.2021.3058296
![]() |
[37] |
Chatterjee S, Sabui D, Roy B (2024) Mobility aware blockage management of a multi-user multi-cell hybrid Li-Fi Wi-Fi system with freeform diversity receiver. Opt Commun 560: 130487. https://doi.org/10.1016/j.optcom.2024.130487 doi: 10.1016/j.optcom.2024.130487
![]() |
Sensibility | Delay time [µS] |
Sensor delay speedy | 0 |
Sensor UI | 50.000 |
Sensor delays Normal | 100.000 |
Parameters | Values |
LED arrays | 1 |
LEDs per array | 25 |
All LEDs power | 2W |
LED Type | 3528 SMD |
Luminous Flux | 400 lm (at 100 mA, 25℃) |
Center Luminous Intensity | 0.73 [cd] |
Receiver's Field of View 𝜃𝑐 | 60 [deg] |
Photodetector Area APD | 1 [Cm2] |
Photodetector responsivity R | 0.4 [A/W] |
Sensibility | Delay time [µS] |
Sensor delay speedy | 0 |
Sensor UI | 50.000 |
Sensor delays Normal | 100.000 |
Parameters | Values |
LED arrays | 1 |
LEDs per array | 25 |
All LEDs power | 2W |
LED Type | 3528 SMD |
Luminous Flux | 400 lm (at 100 mA, 25℃) |
Center Luminous Intensity | 0.73 [cd] |
Receiver's Field of View 𝜃𝑐 | 60 [deg] |
Photodetector Area APD | 1 [Cm2] |
Photodetector responsivity R | 0.4 [A/W] |